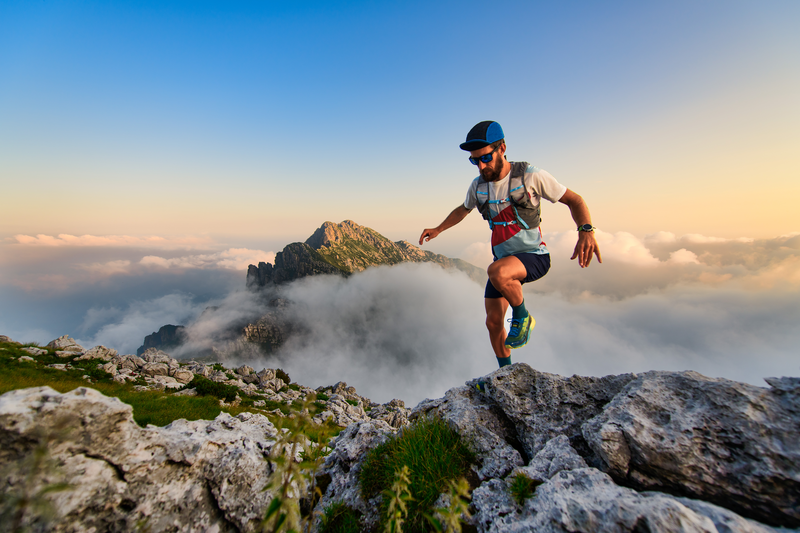
95% of researchers rate our articles as excellent or good
Learn more about the work of our research integrity team to safeguard the quality of each article we publish.
Find out more
OPINION article
Front. Energy Res. , 17 March 2025
Sec. Hydrogen Storage and Production
Volume 13 - 2025 | https://doi.org/10.3389/fenrg.2025.1540396
The concept of sustainability and green energy has become increasingly relevant in our lives, especially in the face of climate change and the growing demand for sustainable solutions in the energy sector. Driven by renewable energies, there is a continuous effort to research and develop alternative energy sources and fuels. In this context, the European Union (EU) Strategy for Hydrogen (H) has emerged, placing this source as one of the central pillars in the fight against climate change. Hydrogen is seen as a potential fuel and energy source of the future. However, in addition to political and structural challenges, this new approach also faces significant technical obstacles.
With the increase in population and human needs, the need for energy continues to grow. The world population is projected to reach ten billion people by the year 2050 (Tarhan and Çil, 2021). To meet this growing demand and promote a transition to clean energies, many countries are incorporating renewable energy sources into their energy mix, while still relying on fossil fuels. Developed countries are gradually reducing their use of fossil fuels in energy production. Considering that 80 per cent of our daily energy needs are still met by these sources, the complete transition is complex and not immediate, but it is an achievable goal.
Hydrogen has the potential to radically transform our reality and is positioned as one of the most promising solutions for the energy future. In the midst of an unprecedented environmental and energy crisis, the need for clean and sustainable alternatives to replace fossil fuels is becoming increasingly urgent (Hassan et al., 2024). Among the various options, H stands out not only for being a clean energy source, but also for its versatility and abundance (Qyyum et al., 2021).
One of the main advantages of hydrogen is its positive environmental impact: when used as a fuel, its only by-product is water vapour, resulting in zero emissions of carbon dioxide (CO2) or other greenhouse gases (Sánchez-Bastardo et al., 2021). This makes it a key element in the fight against global warming, especially in sectors whose decarbonisation is crucial, such as transport and industry. Green hydrogen, produced from renewable sources, has proved to be an ideal solution for driving this transition to low-carbon technologies.
In July 2020, the European Commission (EC) launched the Hydrogen Strategy for a Climate-Neutral Europe, with the aim of accelerating the development of clean hydrogen and ensuring its role as a pillar of a carbon-neutral energy system by 2050. This ambitious plan demonstrates Europe’s commitment to the energy transition and highlights hydrogen as a centrepiece for achieving carbon neutrality targets. Energy generated from hydrogen is extremely versatile and has gained prominence as a promising solution for tackling climate change and driving the transition to a sustainable energy future (Crabtree and Dresselhaus, 2008).
In a report adopted in May 2021, MEPs responded to the EC’s proposal (European Commission, 2020) by emphasising that only green hydrogen - produced from renewable sources - can sustainably contribute to achieving climate neutrality in the long term. This position reflects the growing preference for totally clean energy sources without any dependence on fossil fuels (Gielen et al.,, 2019). The environmental benefits of hydrogen depend directly on how it is produced. Of the 69 million tonnes of H produced globally (excluding hydrogen as a by-product), almost 99% comes from fossil fuels, with 76% derived from natural gas and 23% from coal, which results in significant carbon emissions (International Energy Agency, 2019). There is therefore an urgent need to develop more sustainable H production technologies in order to reduce the associated carbon intensity, as global demand for this energy source continues to rise.
Another crucial point in H’s favour is its abundance (Abdin et al., 2020). Representing around 70 per cent of the matter in the universe, it can be extracted from a variety of sources, including water, which guarantees long-term energy security. In addition, extracting hydrogen from water using renewable energy is a sustainable and environmentally friendly process (Amin et al., 2022). The ability to produce locally also reduces dependence on fossil fuel imports, which is a strategic advantage for many countries. Nations like Portugal could produce their own green hydrogen and export it to countries with less renewable energy generation capacity. This would strengthen global energy security and help create new value chains in the world economy (Santos et al., 2024).
Today, faced with the scarcity of fossil fuels and worsening environmental problems, the need to find new sources of energy that are efficient, environmentally friendly and sustainable has become increasingly evident. In the global context of the transition to a low-carbon energy system, H has emerged as a clean and efficient source. Initially used in vehicles with hydrogen fuel cells, its success has fuelled the development of other sectors related to this emerging technology (Liu et al., 2022).
A key advantage of integrating hydrogen into energy strategies is its potential to decarbonize the sector by serving as a clean, reliable solution for energy storage and transportation. This transition can reduce dependence on fossil fuels and enhance the resilience of energy systems against climate change.
Hydrogen aligns with Environmental, Social, and Governance (ESG) principles, as it promotes environmental sustainability, provides social benefits, and supports economic growth. Additionally, hydrogen plays a crucial role in advancing several United Nations (UN) Sustainable Development Goals (SDGs), including SDG 7 (Affordable and Clean Energy), SDG 13 (Climate Action), and SDG 17 (Partnerships for the Goals) (Zainal et al., 2024).
However, the widespread adoption of hydrogen as a clean energy source in the global transition faces several obstacles, primarily due to the slow development of hydrogen infrastructure. The high cost of producing hydrogen from low-carbon energy sources remains a significant barrier, as current hydrogen supply is still largely dependent on natural gas and coal. Additionally, regulatory challenges are hindering the growth of a clean hydrogen industry. Despite these challenges, various industries and companies are actively working to integrate green hydrogen into their products. As demand for renewable energy rises, green hydrogen is emerging as a key player in the future energy landscape. Growing awareness of its importance has driven advancements in green technology and increased global acceptance. Furthermore, hydrogen production through power-to-gas processes using renewable energy is becoming more economically viable, thanks to the recent sharp decline in renewable energy costs (Zainal et al., 2024).
In the automotive sector, the lack of adequate infrastructure has been one of the main obstacles to the mass adoption of this type of vehicle. However, this reality is changing. In March 2023, the European Parliament and the Council reached an agreement on mandatory national targets for the implementation of H infrastructure. The new rules provide for the installation of refuelling stations at least every 200 km on the main roads of the European Union by 2031. This initiative is key to accelerating the transition to sustainable mobility, especially over long distances, where H-powered vehicles offer advantages in terms of autonomy and refuelling time (Gielen et al., 2019).
Vehicles powered by this chemical element, hydrogen, especially those equipped with fuel cells, offer an energy conversion rate that is two to three times higher than that of internal combustion engines. This means that H-powered vehicles can travel longer distances with lower fuel consumption, making them highly efficient (Halder et al., 2024). In addition, hydrogen combustion does not generate emissions of hydrogen (CO2) or other harmful pollutants such as carbon monoxide (CO) and nitrogen oxides (NOx), which negatively affect health and contribute to air pollution.
Several well-known car manufacturers, such as Toyota and Honda, already have hydrogen-powered vehicles on the road. The Toyota Mirai, for example, is one of the first mass-produced hydrogen cars, offering a range of around 500 km per tank (H2. live). Although hydrogen refuelling infrastructure is still in the development phase in many countries, the rapid growth of refuelling stations in Europe, Japan and California points to a promising future for this technology (Khan et al., 2020). In the motorbike sector, Kawasaki has been one of the pioneers in exploring the potential of hydrogen, taking the first steps in this direction.
In addition to the transport sector, hydrogen has significant applications in industry. It is currently used as a raw material in the production of chemical products such as ammonia and methanol, which are essential for agriculture and the manufacture of plastics. However, the biggest challenge for industry is the transition from fossil-based H to green hydrogen, produced from renewable sources. This change has the potential to substantially reduce hydrogen emissions in industrial sectors that are major energy consumers, such as cement, steel and paper production.
Hydrogen can also be used to provide heat in industrial processes that require high temperatures, such as in refineries and steelworks, offering a clean alternative to industries that traditionally rely on fossil fuels. One promising example is the use of H in the direct reduction of iron ore for steel production, a technology that could drastically reduce CO2 emissions in this sector, which is responsible for around 7% of global emissions (Philibert, 2017).
Steelmaking is a highly energy-intensive process, and current technology is mainly based on the use of coal. This sector is responsible for around 2.7 billion tonnes of CO2 annually. China, South Korea and Japan are the largest emitters, with shares of approximately 15 per cent, 14 per cent and 12 per cent respectively (Hieminga et al., 2023). Replacing coal with green hydrogen in steel production could therefore represent a significant step forward in reducing global greenhouse gas emissions.
Despite all its advantages, hydrogen still faces significant challenges. One of the main obstacles is the cost of producing green hydrogen, which is currently much higher than that of H produced from fossil sources such as natural gas. The development of infrastructure, such as refuelling stations and hydrogen distribution networks, is also a priority in order to make this technology more accessible and viable.
In addition, the issue of storing and transporting H represents a technical challenge. Being an extremely light gas, storage in both a gaseous and liquid state requires special conditions, which can significantly increase costs (Alzohbi et al., 2023). However, research into new storage technologies is progressing rapidly, offering promising solutions to overcome these limitations and enable wider use.
At automotive level, Toyota will unveil portable hydrogen cartridges during the Japan Mobility Show 2024, which represents an important step in the wider adoption of this technology, promoting greater flexibility and accessibility in the use of H as an energy source (ACP, 2024).
Hydrogen has the potential to revolutionise the global energy sector, offering a clean, safe and abundant alternative to fossil fuels. Europe’s commitment, exemplified by the EC Hydrogen Strategy and the recent agreement on mandatory refuelling infrastructure targets, represents an important step towards a climate-neutral economy, with green hydrogen playing a central role in this transition. As countries seek to reduce their carbon emissions and meet climate targets, hydrogen stands out as one of the most promising solutions for ensuring a sustainable future (Delloitte, 2023). With the right investments and continued technological innovation, hydrogen could become an essential tool for mitigating climate change, improving energy security and transforming the global economy. Investing in hydrogen is undoubtedly betting on the future. It offers a unique opportunity to reduce our dependence on fossil fuels, combat climate change and transform the way we produce and consume energy. The transition to an H-based economy requires investment and innovation, but the benefits of a cleaner, more sustainable society are incalculable (Hassan et al., 2024).
Recent data shows that global hydrogen demand has increased fourfold since 1975 and continues to rise. Reports from the International Energy Agency (IEA) reveal that hydrogen production is responsible for 830 million tonnes of CO2 emissions annually comparable to the total yearly emissions of England and Indonesia. Currently, 6% of hydrogen comes from natural gas, 2% from coal, and the remainder from other sources, including hydrocarbons and electrolysis. The worldwide hydrogen demand now stands at 70 million tonnes (Azizimehr et al., 2024).
RC: Writing–original draft. AI-M: Writing–original draft.
The author(s) declare that financial support was received for the research, authorship, and/or publication of this article. This article has not been financed.
Author RC was employed by the CIGEST.
The author declares that the research was conducted in the absence of any commercial or financial relationships that could be construed as a potential conflict of interest.
The authors declare that no Generative AI was used in the creation of this manuscript.
All claims expressed in this article are solely those of the authors and do not necessarily represent those of their affiliated organizations, or those of the publisher, the editors and the reviewers. Any product that may be evaluated in this article, or claim that may be made by its manufacturer, is not guaranteed or endorsed by the publisher.
Abdin, Z., Zafaranloo, A., Rafiee, A., Mérida, W., Lipiński, W., and Khalilpour, K. R. (2020). Hydrogen as an energy vector. Renew. Sustain. Energy Rev. 120, 109620. doi:10.1016/j.rser.2019.109620
ACP, Automóvel Clube de Portugal (2024). Automóvel Clube de Portugal. Available at: https://www.acp.pt/o-clube/revista-acp/atualidade/detalhe/cartuchos-de-hidrogenio-da-toyota-podem-ser-as-baterias-do-futuro.
Alzohbi, G., Almoaikel, A., and AlShuhail, L. (2023). An overview on the technologies used to store hydrogen. Energy Rep. 9 (11), 28–34. doi:10.1016/j.egyr.2023.08.072
Amin, M., Shah, H., Fareed, A., Khan, W., Chung, E., Zia, A., et al. (2022). Hydrogen production through renewable and non-renewable energy processes and their impact on climate change. Int. J. Hydrogen Energy 47 (77), 33112–33134. doi:10.1016/j.ijhydene.2022.07.172
Azizimehr, B., Armaghani, T., Ghasemiasl, R., Nejadian, A. K., and Javadi, M. A. (2024). A comprehensive review of recent developments in hydrogen production methods using a new parameter. Int. J. Hydrogen Energy 72, 716–729. doi:10.1016/j.ijhydene.2024.05.219
Crabtree, G., and Dresselhaus, M. (2008). The hydrogen fuel alternative. Mrs Bull. 33 (4), 421–428. doi:10.1557/mrs2008.84
Gielen, D., Boshell, F., Saygin, D., Bazilian, M. D., Wagner, N., and Gorini, R. (2019). The role of renewable energy in the global energy transformation. Energy strategy Rev. 24, 38–50. doi:10.1016/j.esr.2019.01.006
Halder, P., Babaie, M., Salek, F., Shah, K., Stevanovic, S., Bodisco, T., et al. (2024). Performance, emissions and economic analyses of hydrogen fuel cell vehicles. Renew. Sustain. Energy Rev. 199, 114543. doi:10.1016/j.rser.2024.114543
Hassan, Q., Algburi, S., Sameen, A., Salman, H., and Jaszczur, M. (2024). Green hydrogen: a pathway to a sustainable energy future. Int. J. Hydrogen Energy 50, 310–333. doi:10.1016/j.ijhydene.2023.08.321
Hieminga, G., Dantuma, E., and Stellema, T. (2023). Hydrogen sparks change for the future of green steel production. Future Hydrogen. Available online at: https://think.ing.com/articles/hydrogen-sparks-change-for-the-future-of-green-steel-production/.
International Energy Agency (2019). The Future of Hydrogen. Available online at: https://www.iea.org/reports/the-future-of-hydrogen
Khan, U., Yamamoto, T., and Sato, H. (2020). Consumer preferences for hydrogen fuel cell vehicles in Japan. Transp. Res. Part D Transp. Environ. 87 (3), 102542. doi:10.1016/j.trd.2020.102542
Liu, J., Ma, Y., Yang, J., Sun, L., Guo, D., and Xiao, P. (2022). Recent advance of metal borohydrides for hydrogen storage. Recent Adv. metal borohydrides hydrogen storage. Front. Chem. 10, 945208–945212. doi:10.3389/fchem.2022.945208
Philibert, C. (2017). Renewable energy for industry: from green energy to green materials and fuels. International Energy Agency. Available at: https://iea.blob.core.windows.net/assets/48356f8e-77a7-49b8-87de-87326a862a9a/Insights_series_2017_Renewable_Energy_for_Industry.pdf.
Qyyum, M., Dickson, R., Shah, S., Niaz, H., Khan, A., Liu, J., et al. (2021). Availability, versatility, and viability of feedstocks for hydrogen production: product space perspective. Renew. Sustain. Energy Rev. 145, 1–12. doi:10.1016/j.rser.2021.110843
Sánchez-Bastardo, N., Schlögl, R., and Ruland, H. (2021). Methane pyrolysis for zero-emission hydrogen production: a potential bridge technology from fossil fuels to a renewable and sustainable hydrogen economy. Industrial and Eng. Chem. Res. 60 (32), 11855–11881. doi:10.1021/acs.iecr.1c01679
Santos, B. H., Lopes, J. P., Carvalho, L., Matos, M., and Alves, I. (2024). Public policies to foster green hydrogen seasonal storage: Portuguese study case model until 2040. Energy Strategy Rev. 52101354, 2211–467X. doi:10.1016/j.esr.2024.101354
Tarhan, C., and Çil, M. (2021). A study on hydrogen, the clean energy of the future: hydrogen storage methods. J. Energy Storage 40, 102676. doi:10.1016/j.est.2021.102676
Keywords: hydrogen, climate, energy, mobility, sustainability
Citation: Caldeira R and Infante-Moro A (2025) Strategic hydrogen management: driving a sustainable energy future. Front. Energy Res. 13:1540396. doi: 10.3389/fenrg.2025.1540396
Received: 09 December 2024; Accepted: 25 February 2025;
Published: 17 March 2025.
Edited by:
Simeone Chianese, University of Campania Luigi Vanvitelli, ItalyReviewed by:
Jochelle Laguipo, Dublin City University, IrelandCopyright © 2025 Caldeira and Infante-Moro. This is an open-access article distributed under the terms of the Creative Commons Attribution License (CC BY). The use, distribution or reproduction in other forums is permitted, provided the original author(s) and the copyright owner(s) are credited and that the original publication in this journal is cited, in accordance with accepted academic practice. No use, distribution or reproduction is permitted which does not comply with these terms.
*Correspondence: Rui Caldeira, Y2FsZGVpcmEucmNAZ21haWwuY29t
Disclaimer: All claims expressed in this article are solely those of the authors and do not necessarily represent those of their affiliated organizations, or those of the publisher, the editors and the reviewers. Any product that may be evaluated in this article or claim that may be made by its manufacturer is not guaranteed or endorsed by the publisher.
Research integrity at Frontiers
Learn more about the work of our research integrity team to safeguard the quality of each article we publish.