- Department of Spatial Planning, Urban and Rural Design, Faculty of Architecture, Cracow University of Technology, Kraków, Poland
Environmental challenges, such as climate change and resource depletion, are driving the search for alternative energy sources like wind energy. This study explores the ecological effects of installing wind turbines in Małopolska, Poland. The goals are to find suitable wind power locations, analyse the impact of distance-to-building criteria, and assess carbon emission reduction. The study was carried out in two stages: identification of possible sites for wind farms, taking into account the two criteria of distance from residential buildings of 700 m and 500 m, and estimation of potential carbon emission reductions. Results show optimal locations in north and south of Małopolska. Reducing the distance criteria doubles suitable areas, potentially decreasing Poland’s annual carbon emissions by 0.44%–1.03% and generating up to 1.49 TWh of wind energy, comparable to combined heat and power (CHP) plants in the region.
1 Introduction
Contemporary environmental issues such as climate change, air pollution and the depletion of traditionally used non-renewable natural resources are driving the search for sustainable energy sources. A major environmental challenge is to reduce greenhouse gas emissions, particularly carbon dioxide (CO2), which is the main cause of global warming. One of the main solutions to these problems is renewable energy, including wind power, which is playing an increasingly significant role in the global energy mix. Reducing the production of energy from fossil fuel combustion in favor of wind energy, one of the cleanest and greenest energy sources, contributes to a significant reduction in greenhouse gas emissions. A study conducted by the Global Wind Energy Council (GWEC) revealed that the installation of 1 GW of wind energy worldwide in 2020 will result in a reduction of approximately 1.5 million tonnes of CO2 emissions per year (Global Wind Energy Council, 2012; Global Wind Energy Council, 2023). The illustrative case is the development of wind energy in Denmark, which by 2020 was generating up to 48% of its domestic electricity needs from windmills, making a significant contribution to reducing greenhouse gas emissions (United Nations Framework Convention on Climate Change, 2023). Wind power plants are assumed to be emission-free, thus contributing to improved air quality. The findings of foreign and Polish studies (e.g., conducted by the Institute of Environmental Protection) confirm that investments in wind farms can contribute to the reduction of smog and improve the health of the inhabitants of areas previously dependent on coal. Wind power, important for mitigating global warming and supporting sustainable development, has gained governmental support. Countries are planning major wind energy expansions to address climate change. In 2021, 837 GW of wind capacity in 31 countries produced 2,186 TWh of electricity and mitigated 1,311 Mt of CO2. By mid-century, wind power could generate over 30% of global electricity, potentially reducing CO2 emissions by up to 14,871 Mt by 2050 and 32,864 Mt by 2,100 (Long et al., 2023). In addition to the absence of atmospheric pollution, wind energy has a minimal impact on water resources. Traditional thermal power plants require substantial amounts of water for cooling, leading to overuse of water resources and water quality problems. As documented in a report by the International Renewable Energy Agency (IRENA), the development of wind power, along with solar power, contributes to a significant reduction in global water demand (IRENA, 2018; Johst and Rothstein, 2014; Kara and Şahin, 2023). In Poland, where many regions are experiencing water scarcity, especially in summer, the development of wind energy use can significantly reduce the pressure on local water resources. It is also worth noting that investments in wind energy contribute to the development of technologies and innovations in energy management, which further increases the positive impact on the environment.
Wind energy is one of important and frequently addressed research problems today. It is of interest to scientists in many different fields. Their research covers many different issues. Among them are issues related to: the impact of wind farms on the natural environment, including climate (Abbasi et al., 2016), the impact of atmospheric conditions on the design of wind turbines (Guo et al., 2021), or their impact on atmospheric conditions such as wind speed or turbulence (Akhtar et al., 2024; Bodini et al., 2021; Platis et al., 2018; Raghukumar et al., 2022). The research also encompasses the design of wind turbines (Gasch and Twele, 2012), or the social impacts of wind farms (Gawande and Chaudhry, 2019; Glasson et al., 2022; Klok et al., 2023). Research focuses on both onshore and offshore wind farms. Although there is an increasing focus on offshore wind farms, partly due to more stable wind conditions offshore and the ability to build larger turbines (Akhtar et al., 2024; Glasson et al., 2022), onshore wind farms are an equally important solution, particularly for landlocked countries. The planning of investments such as wind power plants necessitates meticulous prior analysis, encompassing spatial, environmental, economic, and social considerations. This is associated with a number of factors that exert an influence on, inter alia, their location and design, and thus their nominal power output. The current scientific research on wind farm location selection addresses various criteria and methodologies to ensure optimal siting. An increasing number of researchers are utilising multi-criteria decision support tools in conjunction with Geographic Information Systems (GIS) to assess and prioritise potential sites (Josimović et al., 2023; Spyridonidou and Vagiona, 2020; Yegizaw and Mengistu, 2023). The issue of optimal location of wind power plants in Poland is also a developing research topic. Researchers also employ multi-criteria analysis and geographic information systems to select suitable sites (Amsharuk and Łaska, 2024; Zarębski et al., 2024). Part of the research focusses on determining the potential electricity production of wind turbines. Diverse types of software are used for this purpose; for example, Windographer software was used for analyses in Nagpur, India (Roga et al., 2024). The study showed that the Windtec DD 3000-125 can achieve a capacity factor of 9.27%. Despite low wind potential, it can produce approximately 2,412,379 kW per year, demonstrating viable performance in resource-constrained environments. In turn, the other study, which examines the feasibility of wind energy as an alternative to solar and other energy systems, focused on four sites in Ethiopia, uses HOMER software (Hybrid Optimisation Model for Electric Renewables) to analyse and simulate the wind energy potential (Bekele and Palm, 2009). Another example is a study of the potential for wind energy in Bangladesh, with 5 years of wind speed data from fifty sites. In this case, the power density method was found to be the most accurate for this study. As a result, Enercon E-40 and E-48 were identified as the most suitable models for the Sandwip region due to their high efficiency and adaptability to local wind conditions (Hasan et al., 2024). A different statistical approach was presented by scientists from China. They proposed a methodology using finite mixture statistical distributions and a joint angular-linear modelling approach for improved accuracy (Wang and Liu, 2021). Additionally, some research demonstrates the usefulness of combining remote sensing and statistical modelling to assess wind energy potential (Lázár et al., 2024).
In a similar vein, some researchers focus on evaluating the impact of wind turbines on the reduction of carbon dioxide (CO2) emissions. An example is a study that explores the impact of technological advances in wind turbines and land use policies on the potential of wind energy and the reduction of CO2 emissions in China (Liu et al., 2023). This study models how these advances affect wind energy onshore in China. Newer 2.5 MW turbines can boost energy potential. These technological gains improve capacity in regions with poor wind resources, making energy generation economically viable. By 2030, China’s wind potential could reach 20.1 PWh with advanced technology, reducing CO2 emissions by up to 21.7 Gt. Research emphasises the influence of advanced turbines (higher hub heights, larger rotors, and higher rated power) on expanding wind energy viability, especially in regions with poor wind resources. Several studies examine the full-life cycle (FLC) emissions of wind turbines, encompassing stages from manufacturing through operation to decommissioning. For example, studies demonstrate that the CO2 emissions associated with wind turbines are compensated for within approximately two years of operation, indicating that wind farms contributes substantially to net reductions in greenhouse gas emissions shortly after deployment (Pincelli et al., 2024). On the other hand, while wind energy is generally considered to be a clean source, stages such as equipment manufacturing and decommissioning generate significant emissions and environmental impacts. Manufacturing, particularly steel-intensive processes, accounts for up to 90% of life-cycle emissions. However, research confirms that, despite some emissions at certain stages, wind farms remain a low-impact energy source compared to fossil fuels (Mello et al., 2020). In turn, studies conducted for the European Union area emphasise that wind energy has the potential to mitigate up to 13,100 Mt of CO2 in the EU by 2050, representing a critical element in the transition to a carbon-neutral energy system (Vázquez Hernández et al., 2019). This makes wind energy a key player in global efforts to decarbonize the power sector. The research indicates that the correlation between wind energy and CO2 reduction is differentiated across different countries (Ali and Meo, 2024). This is why it is important to do local research - for a specific region.
It seems that the current research on the environmental impact of wind farms, particularly in terms of CO₂ emission reduction, may not fully account for the local siting possibilities and the unique climatic conditions specific to Poland. Poland is currently exploring the potential for amending the legal framework concerning the minimum distance between wind turbines and residential buildings. Among the distance options under review are 700 m and 500 m. Therefore, this study addresses the issue of the potential impact of the realisation of wind farms on the reduction of carbon dioxide emissions by considering two scenarios: one in which the minimum distance from buildings is set at 700 m and the other in which it is set at 500 m.
The study area covers the region of Małopolska, one of Poland’s 16 voivodeships, located in the south of the country. Małopolska is one of the smaller voivodeships. It covers an area of 15,182 km2. Compared to other voivodeships, Małopolska, together with Śląsk, has some of the highest annual levels of air pollution in Poland, especially PM2.5 dust - data for 2021 (Szwagrzyk, 2021). Therefore, it seems important to consider measures that could improve air quality. At the same time, by focusing on a smaller area, it is possible to obtain detailed data on the cadastral division, which is an important aspect when selecting suitable plots for the location of wind turbines.
The aim of the study is to determine:
• Where in Małopolska could wind turbines be located?
• How does the change in the criterion of distance from buildings affect the location possibilities?
• To what extent could the location of new wind power plants in Małopolska contribute to the reduction of carbon dioxide emissions in Poland?
According to the Wind Power database (https://www.thewindpower.net/index.php, n.d.) in 2024 there are 27,753 wind farms in the world with a capacity of 2,393.3 GW. Wind farms generate the largest electrical capacity in China (559 562 MW), Brazil (250 304 MW) and the United States (223 919) (Table 1). On the other hand, the largest number of wind turbines is in Germany (6,443), China (4,791) and Denmark (1,807). From the point of view of the extent to which the demand for electricity from renewable energy sources is met, it is also important to compare the capacity of wind farms per number of inhabitants (Figure 1). In this case, the highest capacity per 10,000 inhabitants has: Ireland (143.55 MW), Estonia (134.23 MW) and United States of America (131.19 MW) (Table 1).
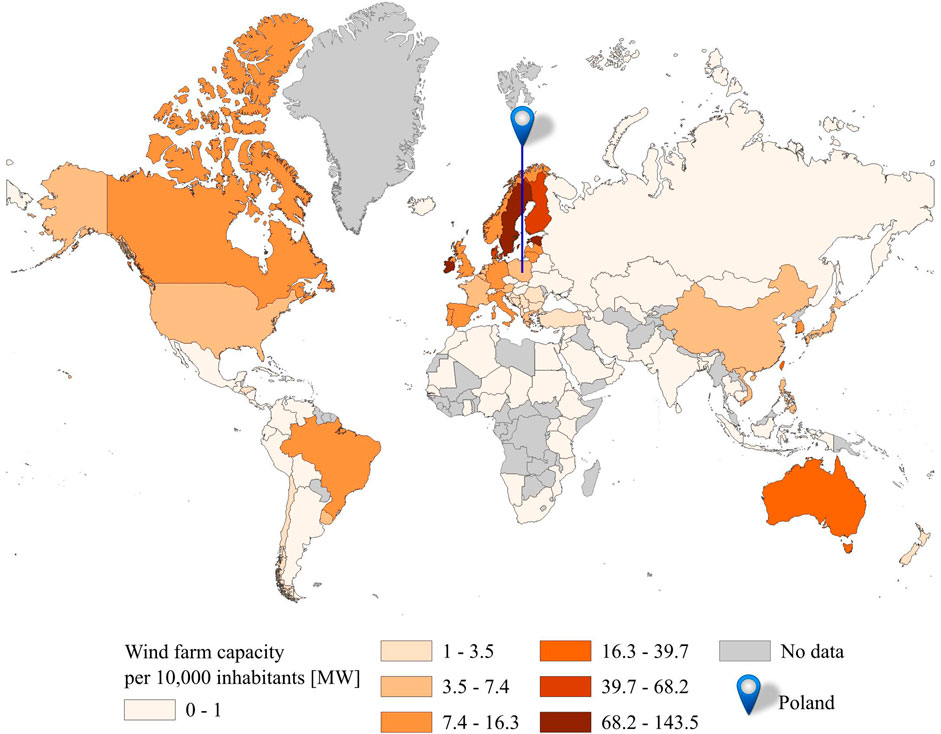
Figure 1. Comparison of wind farm capacity per 10,000 inhabitants [MW] between countries. Own elaboration based on Wind Power database (https://www.thewindpower.net/index.php, n.d.) and World Bank Group data (https://data.worldbank.org/indicator/SP.POP.TOTL, n.d.).
Against this background, Poland is situated among the top thirty countries in terms of wind farm capacity, with a total capacity of 18,379 MW and a wind farm capacity per 10,000 inhabitants of 5.01 MW. According to data from the Database of Topographic Objects (BDOT10k), the largest number of wind turbines are situated in the following voivodeships Zachodniopomorskie, Kujawsko-Pomorskie, Wielkopolskie and Pomorskie (Table 2). These are voivodeships located in northern and central Poland (Figure 2) in the most favourable wind energy zones (Katolik, 2023; Zarębski et al., 2024). The fewest wind turbines are in the border provinces. The smallest number of turbines is thirty-nine in Małopolska.
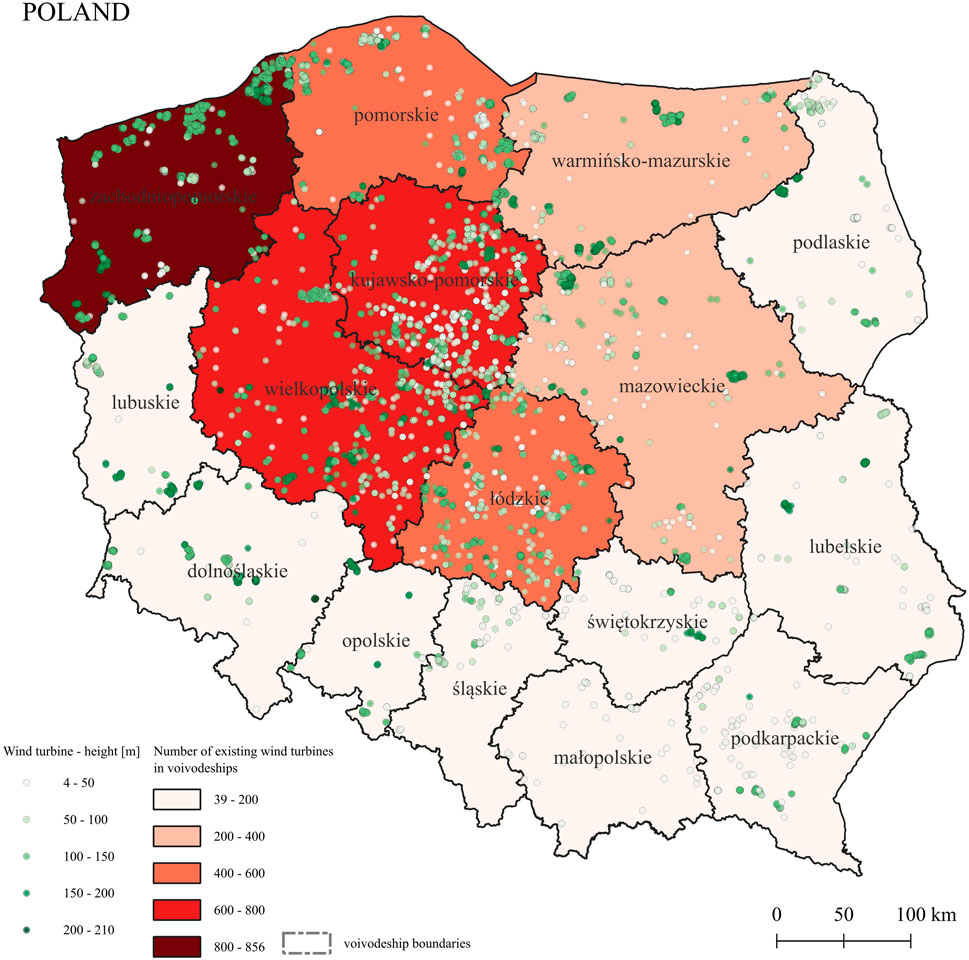
Figure 2. Wind turbines in Poland in 2024. Own elaboration based on Database of Topographic Objects (BDOT10k) (Główny Urząd Geodezji i Kartografii, 2021).
Currently in Poland, the implementation of investments such as wind power plants is regulated by various legal acts. Among the key ones are:
• Act of 20 May 2016 on investments in wind power plants (ISAP, 2016)
• Act of 9 March 2023 amending the Act on investments in wind power plants and certain other acts (ISAP, 2023)
• Act of 27 March 2003 on spatial planning and development (ISAP, 2003)
• Law of 3 February 1995 on the protection of agricultural and forest land (ISAP, 1995)
• Act of 10 April 1997. - Energy law (ISAP, 1997).
• Act of 3 October 2008 on the provision of information on the environment and its protection, public participation in environmental protection and environmental impact assessments (ISAP, 2024).
From the point of view of spatial constraints, the first three acts mentioned introduce the most location-specific conditions in Poland, in particular Act of 20 May 2016 on investments in wind power plants (ISAP, 2016). In 2023, the above law was amended - Act of 9 March 2023 amending the Act on investments in wind power plants and certain other acts, according to which the distance of a wind power plant ‘from a residential building or a building with a mixed function shall be greater than or equal to ten times the total height of the wind power plant, unless the local plan specifies a different distance, expressed in metres, but not less than 700 m’ (Article 4(1)). In light of the aforementioned issues, legislative changes are currently being considered in both industry and legal circles with the aim of reducing the distance to 500 m (Wind energy in reinvented, 2024; PSEW, 2024; Wind energy reinvented. Necessary legislative changes and analysis of onshore potential/Energetyka wiatrowa na nowo. Niezbędne zmiany legislacyjne i analiza potencjału onshore/, 2024). The results presented in this article consider two scenarios: the location of the wind turbines if the 700-meter restriction from development is applied, and the location of the wind turbines in the event that the 500-meter restriction from development is applied. It should be noted that this is a hypothetical assumption and does not consider other local development plan arrangements or the lack thereof.
In Poland, it is widespread practice to classify wind turbines according to electrical output. In this article, the following breakdown by generated power is adopted (Table 3).
Own elaboration based on (ISAP, 2016; Chyła, 2022; Katolik, 2023).
In Poland, for micro-installations of renewable energy sources, including wind energy, there are separate legal regulations for their location and construction than for wind power plants. According to the Law of 20 February 2015 on renewable energy sources, a micro-installation is ‘an installation of a renewable energy source with a total installed electrical capacity of not more than 50 kW, connected to an electricity grid with a rated voltage of less than 110 kV or with a cogeneration heat output of not more than 150 kW, where the total installed electrical capacity is not more than 50 kW’ (ISAP, 2015). On the other hand, according to the Law of 20 May 2016 on wind power investments, the provisions on distances from buildings or forms of nature conservation do not apply to micro-installations, but to wind power plants. In accordance with the adopted classification of wind turbines, this study only concerns wind power plants.
2 Materials and methods
The study was divided into two phases:
• The first concerns the identification of areas in the Małopolska Voivodeship where the spatial conditions allow the realisation of medium, large, and professional wind power plants (3.1 Location).
• The second phase provides an indication of the possible ecological effects of realising this type of investment in the defined areas by limiting the production of electricity from fuel burning installations and thus reducing carbon dioxide emissions (3.2 Ecological effects).
Multi-Criteria Evaluations (MCEs) (Hejmanowska and Hnat, 2009), or otherwise Multi-Criteria Decision Making (MCDM) (Uzun et al., 2021), are often used in studies aimed at decision-making, such as choosing a suitable location given various factor. These analyses are used to help make decisions that depend on multiple determinants, including some that are contradictory. In the case of spatial determinants, GIS tools are sometimes used for this purpose. Various methods are used in multi-criteria analyses, such as Analytic Hierarchy Process (AHP) (Wiącek and Malinowski, 2023), Technique for Order of Preference by Similarity to Ideal Solution (TOPSIS) (Amsharuk and Łaska, 2024), PROMETHEE methods (Brans and De Smet, 2016; Josimović et al., 2023), hard methods - Boolean, or soft methods - Weighted Linear Combination (WLC), Ordered Weighted Average (Hejmanowska and Hnat, 2009).
In the present study, QGIS software (version 3.34.7) was used to perform multi-criteria spatial analyses to be carried out in order to identify areas predisposed for the location of wind power plants.
The first step was to identify the factors that have a significant impact on the choice of wind turbine sites. In Poland, the most common ones found in the literature are: distance from existing settlements, presence of nature protection forms, forests, surface waters, transport areas and overhead power lines, terrain (slope), presence of flood plains, mining areas and location of existing wind turbines (Amsharuk and Łaska, 2024; Hektus, 2016). The necessary data were then collected, processed, and stored in a suitable format for implementation in GIS software. Table 4 summarises the collected data with an indication of the source used.
The above criteria are hard Boolean and indicate which sites are excluded from wind turbine siting. For this reason, a hard Boolean method was used in this study. In this case, the resulting map shows which areas are excluded (not useful) or useful in terms of wind turbine investment.
The authors have detailed the above conditions, considering the legal regulations in force in Poland, including those related to wind turbine investments. For this reason, appropriate buffers were added to the excluded areas and additional parameters of the individual criteria were indicated. Table 5 shows the detailed factors that prevent the siting of wind turbines in Poland according to the applicable legislation.
The analyses were carried out in two ways because of the need to distinguish the criterion of distance from residential areas: for sites within 700 m of buildings and for sites within 500 m of buildings. This division is related to the legislative changes under consideration in Poland, which would reduce the possible required distance from 700 m to 500 m. Taking into account these two criteria will allow answering the question of the impact of this criterion on siting possibilities and environmental impacts.
Then, within the areas identified as unsuitable for wind turbines, areas predisposed for their siting were identified (as a difference). Using the data for each parcel, those parcels that were within the predisposed areas were selected. Subsequently, adjacent parcels were merged. This was done in order to identify larger areas that could potentially be used for the siting of wind turbines with the highest possible capacity, assuming that ownership issues could be resolved. Subsequently, supplementary criteria were incorporated into the evaluation of the merged parcels. These included a minimum parcel size of 0.5 ha, a maximum distance of 800 m from medium-voltage power lines, and the potential for access via a public road within a 10-metre radius. This resulted in the generation of maps delineating the predisposed areas (selected parcels).
The second part of the study dealt with the potential impact of the siting of wind turbines on the identified sites on the reduction of carbon dioxide emissions. In this case, the method used by the authors in another study on the environmental impact of installing photovoltaic panels in residential areas (Blazy et al., 2021) was modelled. However, different conversion factors were used.
The first step was to assume average turbine capacities, which depend, among other things, on the size of the site. Industry literature suggests that a wind farm typically requires between 2 and 40 acres per megawatt of capacity, i.e., approximately 0.80 ha–16 ha (Our World of Energy, 2023). The minimum plot size for a wind farm is therefore assumed to be 0.5 ha. NREL researchers found a rough average of 4 MW per square kilometre. So, a 2-MW wind turbine would require a total area of about half a square kilometre (about 50 ha) (Brenner, 2018). In view of the above, the following averaged values of nominal power versus site size were assumed (Table 6).
Based on these relationships and the criteria for selecting medium, large, and professional power plants, plots larger than 1 ha were accepted for the CO2 reduction analysis. The rest were excluded from further analysis.
The average annual electricity production was then calculated for each parcel on which the wind turbines could be located, based on the formula (Jasiūnas and Teleszewski, 2019):
where:E–average annual energy produced from the wind turbine/power plants [MWh]P–nominal power expressed in megawatts [MW]h–number of hours per year [hours] (87,600 h)CF–capacity factor (0.20)
In Poland, the real wind turbine capacity factor (CF) is from 0.20 to 0.30 (Jasiūnas and Teleszewski, 2019). In this study, the CF was assumed to be 0.20 due to the location of Małopolska in less favourable wind energy zones. The calculations resulted in a potential average annual electricity production from wind power plants that could be built at the indicated sites.
It is assumed that the generation of electricity from wind turbines will result in a reduction in the demand for electricity produced by fuel-burning installations, thereby having an environmental impact in the form of a decrease in greenhouse gas emissions. This article presents the findings of a study investigating the potential reduction of CO2 emissions resulting from the implementation of wind turbines in the Małopolska region. In order to ascertain the potential annual reduction of CO2 emissions in Poland as a consequence of the installation of wind turbines in Małopolska, the average annual production of electricity from wind turbines in Małopolska (E) and the emission factor for electricity produced in fuel combustion plants in Poland (w) were used to calculate the amount of CO2 that could be reduced (ΔCO2) in kilograms. The potential annual reduction of CO2 emissions in Małopolska was estimated according to the following formula:
where:ΔCO2 – potential annual reduction in CO2 emissions [kg]
E–average annual electricity production from wind turbine/power plants [MWh]w–CO2 emission factor for electricity produced in combustion installations in Poland (788 kg/MWh in 2022) [kg/MWh].
Emission factors are employed in the calculation of the environmental impact of retrofit or realisation of new projects that reduce greenhouse gas emissions. They illustrate the burden of CO2 emissions, as well as those of SO₂, NOx, CO, and total dust, per MW hour of electricity generated (Krajowy Ośrodek Bilansowania i Zarządzania Emisjami/Instytut Ochrony Środowiska, 2023).
The result was then compared with the annual CO2 emissions from fuel combustion installations producing electricity or electricity and heat in 2022 in the country. As a result, the size of the contribution of the realisation of wind power plants in Małopolska to the reduction of annual CO2 emissions from fuel combustion installations producing electricity or electricity and heat in Poland was obtained.
3 Results
3.1 Location
The first stage of the study identified the spatial barriers in Małopolska that prevent wind turbines from being located in a given place (Figure 3):
• Forms of nature protection: national parks, landscape parks, nature reserves and Natura 2000 (SAC and SPAs)
• Forests
• Surface waters (flowing and stagnant waters)
• Flood hazard areas
• Areas with a slope of more than 15 degrees
• Mining terrain and mining area
• Transport areas
• Overhead power lines
• Buildings with buffer 700 m or 500 m.
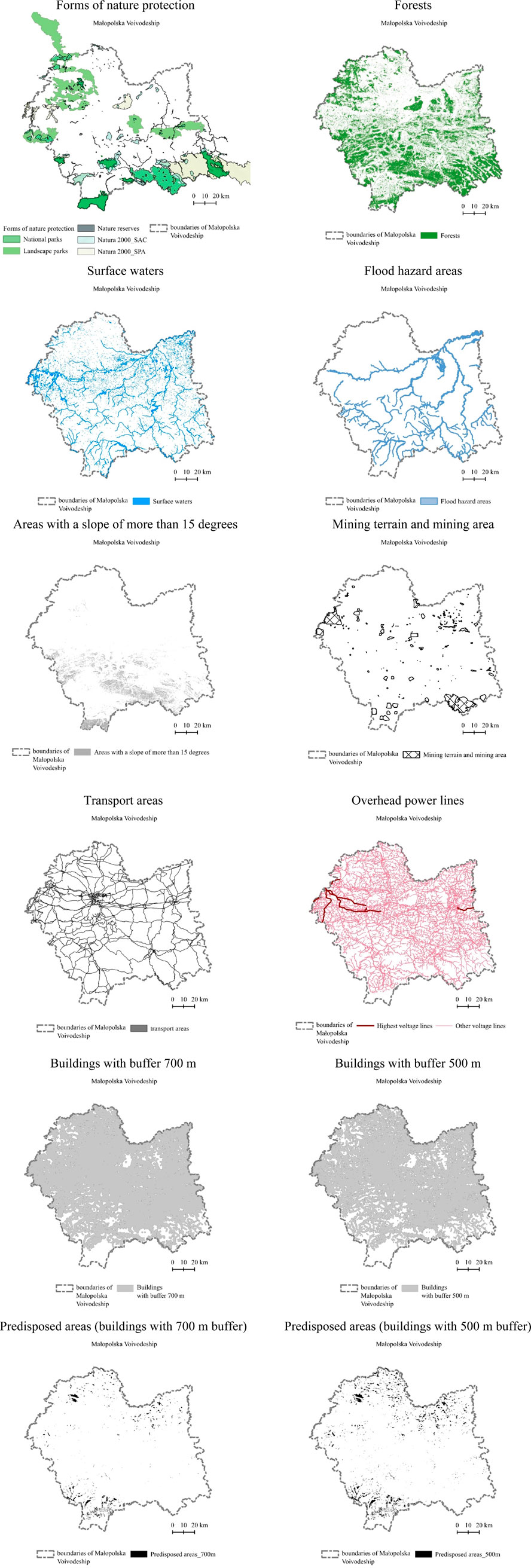
Figure 3. Graphic presentation of different areas, where wind turbines cannot be located. Own elaboration.
As a result, it was found that in Małopolska the criterion that most restricts investment opportunities for wind turbines is existing buildings (Table 7). In the case of a 700-metre buffer zone, this factor effectively excludes 85.2% of the voivodeship’s total area. In the case of a 500-metre buffer, the exclusion rate rises to 76.8%. The next most significant impediment is the presence of forests, which cover an area of 66% of Małopolska, including a 100-metre buffer zone. Overhead power lines can be further specified if we consider all lines regardless of their type. The other criteria already account for a much smaller percentage. Nevertheless, these are major areas that cannot be excluded from the analysis. Moreover, these areas do not always overlap.
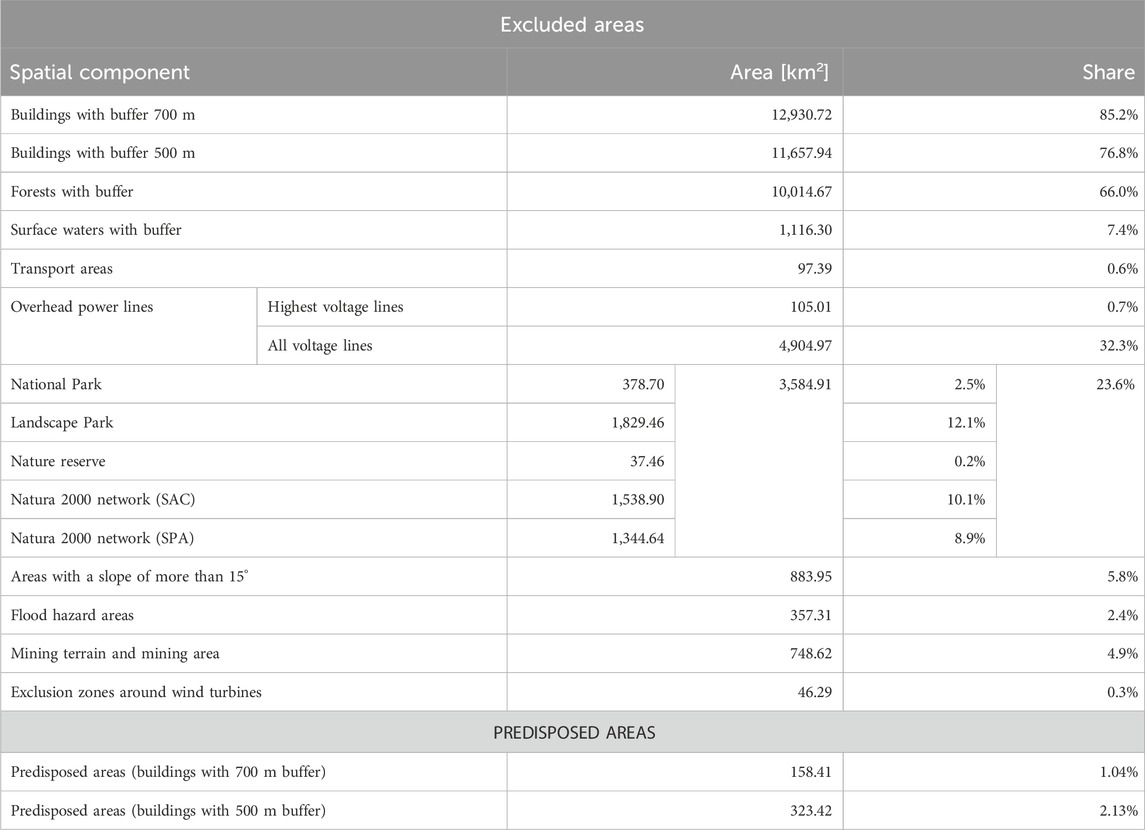
Table 7. The area of the Małopolska region excluded from the location of wind turbines due to spatial barriers.
In the end, the study showed that only 158.41 km2 of land in Małopolska is suitable for wind turbines, provided that a minimum distance of 700 m from buildings is maintained. They occupy 1.04% of the area under consideration. At a distance of 500 m, this area increases to 323.42 km2, which is 2.13% of the area of the voivodeship.
Once the areas initially suitable for the siting of wind turbines had been determined, a further selection was made by taking into account the cadastral plots that fell within the area indicated and then identifying those with an area of at least 0.5 ha and with access to a public road and a medium-voltage power line. Finally, the selected evidential plots were corrected considering the excluded areas to obtain only those parts where the wind turbine can be built (Figure 4).
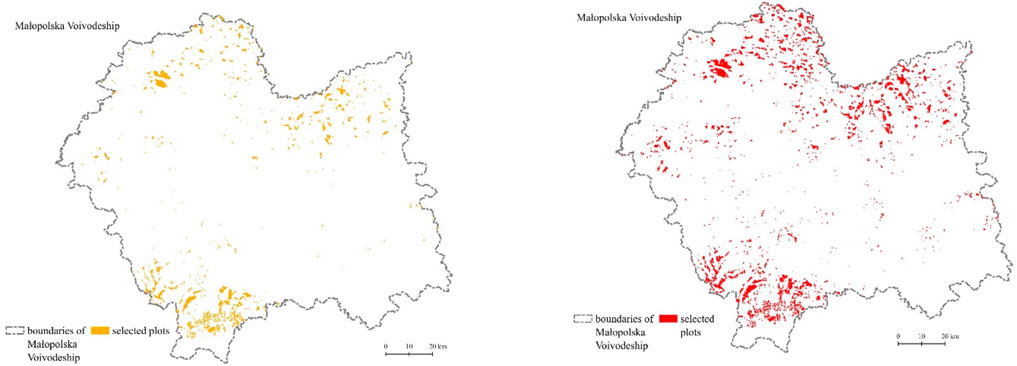
Figure 4. Location of selected plots against the borders of Małopolska voivodeship: on the left for the criterion of distance of 700 m from buildings, on the right for the criterion of distance of 500 m from buildings. Own elaboration.
The results are as follows (Table 8):
• For the criterion of a minimum distance of 700 m from buildings, 1,656 registered plots are suitable for the installation of wind turbines. Their area represents 94.92 km2 or 0.63% of the voivodeship.
• For the criterion of a minimum distance of 500 m from buildings, 3,136 parcels of land have been selected for wind turbines. They cover a total area of 218.82 km2, which is 1.44% of the area of Małopolska.

Table 8. Number and area of parcels of land selected to the location of wind turbines for buffers of 500 and 700 m minimum distance from buildings.
The analysis of the location of the selected plots within the voivodeship showed that most of them are in the northern and southern parts of Małopolska (Figure 5). In the case of 700 m distance from buildings, the largest area of plots (over 1,000 ha) is available in the following poviats: nowotarski, tatrzański, miechowski and krakowski. On the other hand, for the criterion of 500 m from built-up areas, these are the following poviats: nowotarski, miechowski, krakowski, brzeski, tatrzański, proszowicki, dąbrowski and olkuski (Table 9).
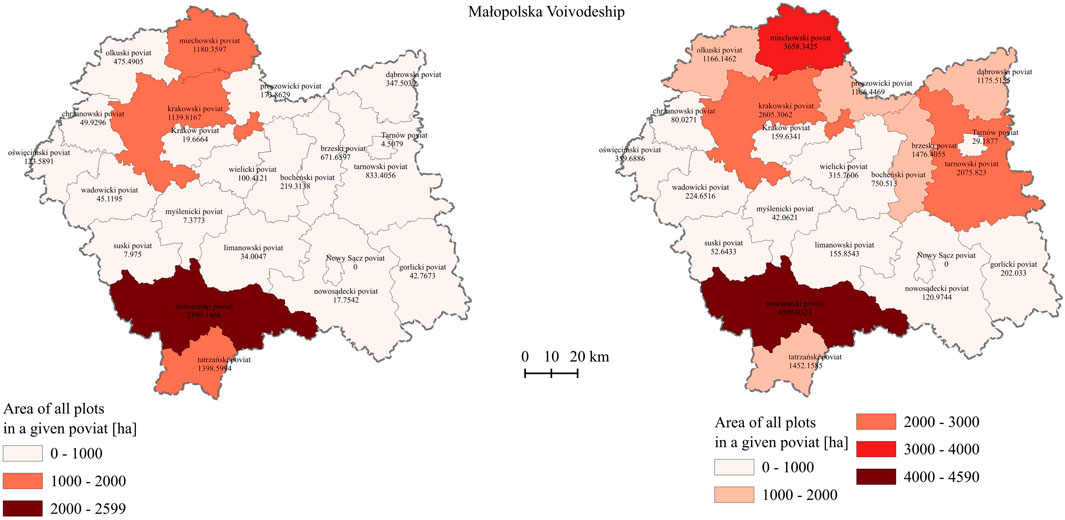
Figure 5. Cartogram showing the total area of selected plots of land in individual poviats of Małopolska. Own elaboration.
3.2 Ecological effect
To calculate the potential ecological impact of the deployment of wind turbines in Małopolska, it was assumed that a wind power plant with a nominal capacity of 40 kW could be constructed on a plot of land measuring 1 ha. The plots of land obtained in the initial phase of the analysis were assigned nominal powers corresponding to the potential wind power plants that could be constructed on them, with consideration given to their respective areas. Plots of less than 1 ha were excluded from the subsequent analysis. Thereafter, the potential annual electricity production was calculated for each of the remaining plots. These analyses were conducted for two variants, namely, plots determined based on the criterion of distance of 700 m and 500 m from existing buildings.
Regarding the 700 m distance criterion, the final survey encompassed 815 plots with an average area of 11.24 ha. The mean nominal power assigned to the plots was 0.45 MW. The final calculation of the potential total annual production of electricity from the wind power plants in the Małopolska region, based on all plots, reached 641,818 MWh (Table 10).

Table 10. Characteristics of plots selected for wind turbine locations for 500 and 700 m minimum distance buffers from buildings in Małopolska.
In the case of the 500 m distance criterion, 1,591 plots with an average area of 13.37 ha were studied. The average nominal power allocated to the plots was 0.53 MW. Ultimately, the potential total (all plots) annual wind power production in the Małopolska area reached 1,490,508 MWh (Table 10).
The results obtained subsequently permitted the calculation of the annual reduction in CO₂ emissions resulting from the implementation of wind power plants on the selected plots.
In the case of the 700 m criterion, the potential annual reduction of CO2 emissions in Małopolska was calculated to be 505,752,584 kg, representing 0.44% of the annual CO2 emissions in Poland from fuel combustion installations producing electricity or electricity and heat in 2022 (Table 11).
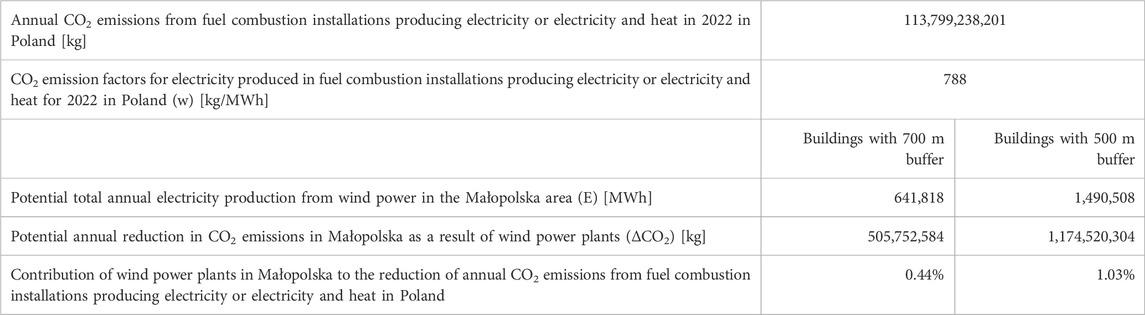
Table 11. Impact of wind energy use in the Małopolska region on the reduction of CO2 emissions in the country.
With regard to the 500 m criterion, the potential annual reduction of CO2 emissions in Małopolska was 1,174,520,304 kg, representing 1.03% of the annual CO2 emissions in Poland from fuel combustion installations producing electricity or electricity and heat in 2022 (Table 11).
4 Discussion
In different countries, the distance regulations between wind turbines and residential areas vary significantly, depending largely on public acceptance, environmental considerations, and land availability. In Poland, the '10H' rule was introduced in 2016, which requires wind turbines to be placed at a distance at least ten times the height of the turbine from residential buildings, usually equating to approximately 2 km. Although intended to protect local residents from noise and visual impacts, this regulation effectively restricted new wind turbine projects almost in 98% of Polish territory. However, recent proposals advocate for reducing the distance to 500 m, which is more aligned with regulations in other European countries (European Platform Against Windfarms, 2009; Stede et al., 2021). On the contrary, countries like Germany have region-specific rules. For example, Bavaria follows a ‘10H’ rule, but in Germany, the required distance can range between 400 and 1,000 m depending on local legislation. The United Kingdom typically mandates minimum distances of 500–600 m, though these can vary by region and turbine height, but minimum distance is 350 m. In Denmark, the rule is around four times the turbine height or approximately 500 m from residential areas. These regulations often balance the need to expand renewable energy with concerns about noise, shadow flicker, and aesthetic impact on communities. A preliminary investigation of the potential for the wind turbine installation in Małopolska has revealed that the main obstacle to its deployment is the requirement to maintain a specified distance from existing buildings. It is important and necessity to guarantee appropriate living conditions and to prevent any deterioration thereof through investments such as wind power plants. To date, the Polish legislature has considered two distances with respect to the aforementioned criterion: distances of 700 m and 500 m, respectively. The present study has shown that a reduction in these distances would allow the doubling the suitable area for the location of wind turbines, from 0.63% to 1.44% of the Małopolska Voivodeship. Moreover, an examination of the cadastral division revealed that nearly twice as many plots could be designated for this category of investment. It is important to note that these findings are not definitive. It is probable that the availability of plots is lower, as limitations resulting from detailed and local restrictions, such as the soil quality of the plot (value class), still need to be considered. However, the objective of this study was to indicate the maximum potential investment opportunities. A comparison of the regulations used in the countries mentioned above reveals that a distance of 500 m is permitted in the majority of them. Considering the findings of this study and the aforementioned considerations, it may be beneficial to explore the possibility of implementing a minimum distance rule of 500 m.
Prior research on the location of wind farms in Poland has demonstrated that the facilities with the highest generation capacity are situated at distances of 30–80 km from larger urban centres, defined as those with a population exceeding 100,000 (Zarębski et al., 2024). The present study revealed that in the Małopolska region, the mean distance between potential wind farms and the nearest towns, in accordance with the 700-metre distance criterion from development, would be 8.36 km. A reduction in the criterion to 500 m results in a decrease in the distance to 8.03 km. When considering only cities with more than 100,000 inhabitants (Kraków and Tarnów), the distance is 51.13 km for 700 m and 44.81 km for 500 m. These results are comparable to those of the aforementioned studies. It is also important to support rural and sparsely populated areas in implementing renewable energy projects, taking advantage of their unique settlement structures (Śleszyński et al., 2021).
Analyses of the ecological effect have demonstrated the efficacy of installing new wind turbines in the context of reducing carbon dioxide emissions. Modifying the distance criterion of buildings from 700 m to 500 m has the potential to double the total annual production of electricity from wind farms in the Małopolska area. This consequently results in a double reduction in CO2 emissions. This is the result of the assumption that there is a linear relationship between the area of a plot of land and the nominal power of a wind power plant. However, it is important to note that on a Polish scale, the change is relatively minor. It represents an increase from 0.44% of the total annual CO2 emissions from fuel combustion installations producing electricity or electricity and heat in 2022 in Poland to 1.03%. It should be noted that these figures represent the maximum potential values, which will likely be lower in practice.
The largest city in Małopolska, Kraków, is home to important CHP plants operated by PGE Energia Ciepła S.A., which produce both electricity and heat for the city and surrounding areas. The CHP Kraków plant is one of the largest in the region. Based on available data: the CHP Kraków Plant produces approximately 450 MW of electricity and 1,080 MW of heat. In 2020, the total electricity generated by PGE Energia Ciepła in Małopolska was around 1,400 GWh (or 1.4 TWh) annually (Ciepło dla Krakowa, n.d.). Potential wind energy production (1.49 TWh) from the plots analysed in Małopolska could match or even slightly surpass the electricity produced by CHP plants in the region (1.4 TWh). However, it is important to note that CHP plants also provide substantial heat, which wind turbines do not.
This paper presents one of the key considerations when planning investments in wind turbines and thus renewable energy sources. It might be useful to make decisions about the establishment of criteria for the location of wind turbines, as well as their impact on the reduction of CO2 emissions. However, when making a final decision, it is also essential to consider a number of additional factors, including economic and social impacts, for example the impact of the location of wind turbines on property prices and the health of residents (Jensen et al., 2018).
5 Conclusion
Our research was focused on three key areas of inquiry:
1. Where in Małopolska could wind turbines be located?
2. How does the change in the criterion of distance from buildings affect the location possibilities?
3. To what extent could the location of new wind power plants in Małopolska contribute to the reduction of carbon dioxide emissions in Poland?
We next develop three key insights in response to the research questions:
1. In Małopolska, with the current legal regulations, a maximum of 0.63% of the land allows the location of wind farms. These areas are located in the northern and southern parts of the voivodeship.
The study revealed, that in Małopolska only 94.92 km2 of land is suitable for wind turbines if a minimum distance of 700 m from buildings is maintained, representing for 0.63% of the area under review. Plots more than 1,000 ha are available in nowotarski, tatrzański, miechowski, and krakowski poviats.
2. The reduction the distance to 500 m would permit the doubling of the area suitable for the location of wind turbines, from 0.63% to 1.44% of the Małopolska voivodeship.
Reducing the distance to 500 m increases the suitable area to 218.82 km2, or 1.44% of the voivodeship’s total area. For a 500 m distance, plots over 1,000 ha are available in nowotarski, miechowski, krakowski, brzeski, tatrzański, proszowicki, dąbrowski, and olkuski poviats.
3. The potential annual reduction of CO2 emissions in Małopolska was calculated to be 505,752,584 kg, representing 0.44% of the annual CO2 emissions in Poland from fuel combustion installations producing electricity or electricity and heat in 2022.
Changing the distance criterion from 700 m to 500 m can double CO2 emission reductions. With the 700 m criterion, Małopolska could reduce CO2 emissions by 505,752,584 kg, accounting for 0.44% of Poland’s 2022 emissions from fuel-based electricity production. At 500 m, the reduction potential is 1,174,520,304 kg, or 1.03% of Poland’s total.
The findings of the study suggest that the specific local conditions prevalent in the Małopolska region restrict the potential for establishing wind farms. The principal constraint identified is however the nationwide requirement for wind turbines to maintain a certain distance from residential structures. Modifying the minimum distance requirement from these buildings, could potentially double the available land area and, consequently, contribute to a reduction in carbon dioxide emissions by facilitating the construction of additional wind farms.
Data availability statement
The original contributions presented in the study are included in the article/supplementary material, further inquiries can be directed to the corresponding author.
Author contributions
RB: Conceptualization, Project administration, Resources, Supervision,Validation, Writing–original draft, Writing–review and editing. RŁ: Conceptualization, Data curation, Formal Analysis, Investigation, Methodology, Resources, Visualization, Writing–original draft, Writing–review and editing.
Funding
The author(s) declare that no financial support was received for the research, authorship, and/or publication of this article.
Conflict of interest
The authors declare that the research was conducted in the absence of any commercial or financial relationships that could be construed as a potential conflict of interest.
Generative AI statement
The author(s) declare that no Generative AI was used in the creation of this manuscript.
Publisher’s note
All claims expressed in this article are solely those of the authors and do not necessarily represent those of their affiliated organizations, or those of the publisher, the editors and the reviewers. Any product that may be evaluated in this article, or claim that may be made by its manufacturer, is not guaranteed or endorsed by the publisher.
Abbreviations
CHP plants, Combined heat and power plants; GWEC, Global Wind Energy Council; HOMER software, Hybrid Optimisation Model for Electric Renewables; IRENA, International Renewable Energy Agency; GIS, Geographic Information Systems; FLC, Full-life cycle; MCEs, Multi-Criteria Evaluations; MCDM, Multi-Criteria Decision-Making; AHP, Analytic Hierarchy Process; TOPSIS, Technique for Order of Preference by Similarity to Ideal Solution; WLC, Weighted Linear Combination; BDOT10k, National Database of Topographic Objects in scale 1:10 000; DEM, Digital Elevation Model; E, Average annual energy produced from the wind turbine/wind farms; P, Nominal power; H, Number of hours per year; CF, Capacity factor; CO2, Carbon dioxide; ΔCO2, Potential annual reduction in CO2 emissions; w, CO2 emission factor for electricity produced in combustion installations; SAC, Special Areas of Conservation; SPAs, Special Protection Areas.
References
Abbasi, S. A., Tabassum-Abbasi, , and Abbasi, T. (2016). Impact of wind-energy generation on climate: a rising spectre. Renew. Sustain. Energy Rev. 59, 1591–1598. doi:10.1016/j.rser.2015.12.262
Akhtar, N., Geyer, B., and Schrum, C. (2024). Larger wind turbines as a solution to reduce environmental impacts. Sci. Rep. 14 (1), 6608. doi:10.1038/s41598-024-56731-w
Ali, S., and Meo, M. S. (2024). How wind-based renewable energy contribute to CO2 emissions abatement? Evidence from Quantile-on-Quantile estimation. Int. J. Environ. Sci. Technol. 21 (9), 6583–6596. doi:10.1007/s13762-023-05409-3
Amsharuk, A., and Łaska, G. (2024). Site selection of wind farms in Poland: combining theory with reality. Energies 17 (11), 2635. doi:10.3390/en17112635
Bekele, G., and Palm, B. (2009). Wind energy potential assessment at four typical locations in Ethiopia. Appl. Energy 86 (3), 388–396. doi:10.1016/j.apenergy.2008.05.012
Blazy, R., Błachut, J., Ciepiela, A., Łabuz, R., and Papież, R. (2021). Renewable energy sources vs. an air quality improvement in urbanized areas - the metropolitan area of Kraków case. Front. Energy Res. 9. doi:10.3389/fenrg.2021.767418
Bodini, N., Lundquist, J. K., and Moriarty, P. (2021). Wind plants can impact long-term local atmospheric conditions. Sci. Rep. 11 (1), 22939. doi:10.1038/s41598-021-02089-2
Chyła, T. (2022). Types of wind turbines/Rodzaje turbin wiatrowych. Available at: https://www.energetyka.plus/rodzaje-turbin-wiatrowych/(Accessed September 23, 2024).
European Platform Against Windfarms (2009). European Setbacks (minimum distance between wind turbines and habitations).
Gasch, R., and Twele, J. (2012). in Wind power plants. Fundamentals, design, construction and operation (Berlin, Heidelberg: Springer Berlin Heidelberg).
Gawande, A., and Chaudhry, P. (2019). Environmental and social impacts of wind energy: a view point with reference to India. Ecol. Quest. 30 (2), 39. doi:10.12775/eq.2019.009
Generalna Dyrekcja Ochrony Środowiska (GDOŚ) (2024). Forms of nature protection in Poland. Available at: https://www.gov.pl/web/gdos/dostep-do-danych-geoprzestrzennych.
Glasson, J., Durning, B., Welch, K., and Olorundami, T. (2022). The local socio-economic impacts of offshore wind farms. Environ. Impact Assess. Rev. 95, 106783. doi:10.1016/j.eiar.2022.106783
Global Wind Energy Council (2012). Wind power is crucial for combating climate change. Available at: https://gwec.net/wp-content/uploads/2012/06/Wind-climate-fact-sheet-low-res.pdf (Accessed September 25, 2024).
Global Wind Energy Council (2023). Global wind report 2023. Available at: https://gwec.net/wp-content/uploads/2023/03/GWR-2023_interactive.pdf (Accessed September 25, 2024).
Główny Urząd Geodezji i Kartografii (2021). Digital elevation model (DEM). Available at: https://dane.gov.pl/pl/dataset/792,numeryczny-model-terenu-o-interwale-siatki-co-najmniej-100-m.
Główny Urząd Geodezji i Kartografii (2022). Exclusion zones around wind turbines. Available at: https://www.geoportal.gov.pl/pl/usluga/uslugi-pobierania-wfs/.
Główny Urząd Geodezji i Kartografii (2023). Land and property register. Available at: https://www.geoportal.gov.pl/pl/usluga/uslugi-przegladania-wms-i-wmts/.
Główny Urząd Geodezji i Kartografii (2024). National database of topographic objects-BDOT10k. Available at: https://dane.gov.pl/pl/dataset/2030,dane-obiektow-topograficznych-o-szczegolowosci-zap.
Guo, N., Zhang, M., Li, B., and Cheng, Y. (2021). Influence of atmospheric stability on wind farm layout optimization based on an improved Gaussian wake model. J. Wind Eng. Industrial Aerodynamics 211, 104548. doi:10.1016/j.jweia.2021.104548
Hasan, M., Dey, P., Janefar, S., Salsabil, N. A., Khan, I. J., Chowdhury, N. U. R., et al. (2024). A critical analysis of wind energy generation potential in different regions of Bangladesh. Energy Rep. 11, 2152–2173. doi:10.1016/j.egyr.2024.01.061
Hejmanowska, B., and Hnat, E. (2009). Multi-factoral evaluation of residential area locations: case Study of Podegrodzie local authority/Wielokryterialna analiza lokalizacji zabudowy na przykładzie gminy Podegrodzie. Arch. Fotogram. Kartogr. i Teledetekcji 20, 109–121.
Hektus, P. (2016). “Czynniki lokalizacji oraz rozmieszczenie przestrzenne energetyki wiatrowej w Polsce,” in Interdyscyplinarne zagadnienia w inżynierii i ochronie środowiska 8. Politechnika Wrocławska (Wrocław, Poland: Oficyna Wydawnicza Politechniki Wrocławskiej), 120–130. Available at: https://www.thewindpower.net/index.php.
IRENA (2018). “Global energy transformation. A roadmap to 2050,”. Abu Dhabi. Available at: https://www.irena.org/-/media/Files/IRENA/Agency/Publication/2018/Apr/IRENA_Report_GET_2018.pdf (Accessed September 25, 2024).
ISAP (1995). Law of 3 February 1995 on the protection of agricultural and forest land (n.d.) Dz.U. 2024 poz. 82. Poland. Available at: https://isap.sejm.gov.pl/isap.nsf/DocDetails.xsp?id=WDU20240000082.
ISAP (1997). Act of 10 April 1997. - energy law (n.d.) dz.U. 2024 poz. 266. Available at: https://isap.sejm.gov.pl/isap.nsf/DocDetails.xsp?id=WDU20240000266.
ISAP (2003). Act of 27 March 2003 on spatial planning and development (n.d.) Dz.U. 2024 poz. 1130. Available at: https://isap.sejm.gov.pl/isap.nsf/DocDetails.xsp?id=WDU20240001130.
ISAP (2015). Law of February 20, 2015 on renewable energy sources. Available at: https://isap.sejm.gov.pl/isap.nsf/DocDetails.xsp?id=WDU20240001361.
ISAP (2016). Act of 20 May 2016 on investments in wind power plants. Available at: https://isap.sejm.gov.pl/isap.nsf/DocDetails.xsp?id=WDU20240000317.
ISAP (2023). Act of 9 March 2023 amending the Act on investments in wind power plants and certain other acts. Available at: https://isap.sejm.gov.pl/isap.nsf/DocDetails.xsp?id=WDU20230000553.
ISAP (2024). October 2008 on the provision of information on the environment and its protection, public participation in environmental protection and environmental impact assessments. Dz.U. 2024 poz 1112. Available at: https://isap.sejm.gov.pl/isap.nsf/DocDetails.xsp?id=WDU20240001112.
Jasiūnas, K., and Teleszewski, T. J. (2019). “Wind energy,” in Buildings 2020+. Energy sources. Politechnika Białostocka (Białystok, Poland: Oficyna Wydawnicza Politechniki Białostockiej), 99–132.
Jensen, C. U., Panduro, T. E., Lundhede, T. H., Nielsen, A. S. E., Dalsgaard, M., and Thorsen, B. J. (2018). The impact of on-shore and off-shore wind turbine farms on property prices. Energy Policy116, 50–59. doi:10.1016/j.enpol.2018.01.046
Johst, M., and Rothstein, B. (2014). Reduction of cooling water consumption due to photovoltaic and wind electricity feed-in. Renew. Sustain. Energy Rev. 35, 311–317. doi:10.1016/j.rser.2014.04.029
Josimović, B., Srnić, D., Manić, B., and Knežević, I. (2023). Multi-criteria evaluation of spatial aspects in the selection of wind farm locations: integrating the GIS and PROMETHEE methods. Appl. Sci. 13 (9), 5332. doi:10.3390/app13095332
Kara, T., and Şahin, A. D. (2023). Implications of climate change on wind energy potential. Sustainability 15 (20), 14822. doi:10.3390/su152014822
Klok, C. W., Kirkels, A. F., and Alkemade, F. (2023). Impacts, procedural processes, and local context: rethinking the social acceptance of wind energy projects in The Netherlands. Energy Res. and Soc. Sci. 99, 103044. doi:10.1016/j.erss.2023.103044
Krajowy Ośrodek Bilansowania i Zarządzania Emisjami/Instytut Ochrony Środowiska (2023). Wskaźniki emisyjności CO2, SO2, NOx, CO i pyłu całkowitego dla energii elektrycznej na podstawie informacji zawartych w Krajowej bazie o emisjach gazów cieplarnianych i innych substancji za 2022 rok. Available at: https://www.kobize.pl/pl/fileCategory/id/28/wskazniki-emisyjnosci (Accessed September 23, 2024).
Lázár, I., Hadnagy, I., Bertalan-Balázs, B., Bertalan, L., and Szegedi, S. (2024). Comparative examinations of wind speed and energy extrapolation methods using remotely sensed data – a case study from Hungary. Energy Convers. Manag. X 24, 100760. doi:10.1016/j.ecmx.2024.100760
Liu, F., Sun, F., and Wang, X. (2023). Impact of turbine technology on wind energy potential and CO2 emission reduction under different wind resource conditions in China. Appl. Energy 348, 121540. doi:10.1016/j.apenergy.2023.121540
Long, Y., Chen, Y., Xu, C., Li, Z., Liu, Y., and Wang, H. (2023). The role of global installed wind energy in mitigating CO2 emission and temperature rising. J. Clean. Prod. 423, 138778. doi:10.1016/j.jclepro.2023.138778
Mello, G., Ferreira Dias, M., and Robaina, M. (2020). Wind farms life cycle assessment review: CO2 emissions and climate change. Energy Rep. 6, 214–219. doi:10.1016/j.egyr.2020.11.104
Państwowe Gospodarstwo Wodne Wody Polskie (2024). Flood hazard map (MZP). Available at: https://dane.gov.pl/pl/dataset/2178.
Państwowy Instytut Geologiczny (2024). Central geological database. Available at: https://dane.gov.pl/pl/dataset?page=10&per_page=20&q=&sort=-date&institution%5Bid%5D%5Bterms%5D=88&isMapOpen=false.
Pincelli, I. P., Hinkley, J., and Brent, A. (2024). Developing onshore wind farms in Aotearoa New Zealand: carbon and energy footprints. J. R. Soc. N. Z., 1–23. doi:10.1080/03036758.2024.2344785
Platis, A., Siedersleben, S. K., Bange, J., Lampert, A., Bärfuss, K., Hankers, R., et al. (2018). First in situ evidence of wakes in the far field behind offshore wind farms. Sci. Rep. 8 (1), 2163. doi:10.1038/s41598-018-20389-y
PSEW (2024). Wind energy in Poland 2024. Available at: https://www.psew.pl/raporty/(Accessed September 23, 2024).
Raghukumar, K., Chartrand, C., Cheung, L., Roberts, J., et al. (2022). Effect of floating offshore wind turbines on atmospheric circulation in California. Front. Energy Res. 10. doi:10.3389/fenrg.2022.863995
Roga, S., Dahiwale, H., Bardhan, S., and Sinha, S. (2024). Wind energy potential assessment: a case study in Central India. Proc. Institution Civ. Eng. - Energy 177 (3), 130–148. doi:10.1680/jener.22.00016
Śleszyński, P., Nowak, M., Brelik, A., Mickiewicz, B., and Oleszczyk, N. (2021). Planning and settlement conditions for the development of renewable energy sources in Poland: conclusions for local and regional policy. Energies14 (7), 1935. doi:10.3390/en14071935
Spyridonidou, S., and Vagiona, D. G. (2020). Systematic review of site-selection processes in onshore and offshore wind energy research. Energies 13 (22), 5906. doi:10.3390/en13225906
Stede, J., Blauert, M., and May, N. (2021). Way off: the effect of minimum distance regulation on the deployment and cost of wind power. SSRN Electron. J. doi:10.2139/ssrn.4005887
United Nations Framework Convention on Climate Change (2023). Wind energy in Denmark case study: good practices and lessons learned on the setup and implementation of national systems of innovation. Available at: https://unfccc.int/ttclear/misc_/StaticFiles/gnwoerk_static/TEC_NSI/63eb6ced5b1e43429a6eccdef95ff61e/85bd141304c5486fb7f2ef71f8d2d45f.pdf (Accessed September 25, 2024).
Uzun, B., Ozsahin, I., and Agbor, V. O. (2021). “Theoretical aspects of multi-criteria decision-making (MCDM) methods,” in Applications of multi-criteria decision-making theories in healthcare and biomedical engineering (Elsevier), 3–40.
Vázquez Hernández, C., Serrano González, J., and Fernández-Blanco, R. (2019). New method to assess the long-term role of wind energy generation in reduction of CO2 emissions – case study of the European Union. J. Clean. Prod. 207, 1099–1111. doi:10.1016/j.jclepro.2018.09.249
Wang, Z., and Liu, W. (2021). Wind energy potential assessment based on wind speed, its direction and power data. Sci. Rep. 11 (1), 16879. doi:10.1038/s41598-021-96376-7
Wiącek, M., and Malinowski, M. (2023). Method of identifying potential locations for wind farms in rural areas/Metoda identyfikacji potencjalnych lokalizacji farm wiatrowych na terenach wiejskich. Infrastructure Ecol. rural areas/Infrastruktura i Ekologia Terenów Wiejskich/18 (1), 205–220. doi:10.14597/INFRAECO.2023.011
Wind energy reinvented (2024). “Necessary legislative changes and analysis of onshore potential/Energetyka wiatrowa na nowo,” in Niezbędne zmiany legislacyjne i analiza potencjału onshore/. Warszawa.
Yegizaw, E. S., and Mengistu, D. A. (2023). Multi-criteria decision analysis for wind farm location selection in Bahir Dar City and its surroundings, Northwestern Ethiopia. Environ. Monit. Assess. 195 (5), 559. doi:10.1007/s10661-023-11114-y
Keywords: wind farms, renewable energy sources, GIS, multicriteria analysis, reduction of CO2 emissions
Citation: Blazy R and Łabuz R (2025) Potential ecological effects of wind farm location on the example of the Małopolska region in Poland. Front. Energy Res. 12:1504371. doi: 10.3389/fenrg.2024.1504371
Received: 02 October 2024; Accepted: 09 December 2024;
Published: 07 January 2025.
Edited by:
Agnimitra Biswas, National Institute of Technology, Silchar, IndiaReviewed by:
Anal Ranjan Sengupta, JIS College of Engineering, IndiaSukanta Roga, Visvesvaraya National Institute of Technology, India
Copyright © 2025 Blazy and Łabuz. This is an open-access article distributed under the terms of the Creative Commons Attribution License (CC BY). The use, distribution or reproduction in other forums is permitted, provided the original author(s) and the copyright owner(s) are credited and that the original publication in this journal is cited, in accordance with accepted academic practice. No use, distribution or reproduction is permitted which does not comply with these terms.
*Correspondence: Rafał Blazy, cmJsYXp5QHBrLmVkdS5wbA==
†These authors have contributed equally to this work and share first authorship