- China Nuclear Power Technology Research Institute Co., Ltd., Shenzhen, Guangdong, China
Within the context of the long-term development plan of batch loading of accident-tolerant fuel assemblies, an equivalent physical model of lead test assemblies is established based on the requirements of radiation testing and the applicability of analysis software. The quantitative assessment has been conducted on the impacts of parameters including zirconium alloy cladding coating and pellet additives on reactivity of fuel assembly. Concurrently, the fuel management strategy is optimized in alignment with the irradiation test objectives of ATF assemblies, leading to the creation of an evaluation process tailored for ATF assembly loading pattern. Focusing on the irradiation of the lead test assemblies into a nuclear power plant in China, three-cycle nuclear reactor loading pattern are designed, which are taking into account the economic, safety, and irradiation testing goals of the nuclear power plant. Simultaneously, the neutronic characteristics are also evaluated, quantitatively. The results show that the established equivalent physical model can effectively represent the characteristics of the lead test assemblies. The loading patterns designed based on the Optimised fuel management strategy achieve the desired goals in terms of economy, safety and radiation test requirements. The designed loading pattern evaluation process and equivalent model analysis method in this work provide guidance for the subsequent engineering design and application of irradiation testing for lead test assemblies.
1 Introduction
The Accident Tolerant Fuel (ATF) is a new generation of highly secure nuclear fuel developed to enhance the safety performance of nuclear fuel in accident conditions, especially under severe accident scenarios. Compared with traditional fuels, ATF improves the accident tolerance of the fuel by enhancing the thermophysical properties of the nuclear fuel pellets or the oxidation resistance and mechanical properties of the pellet cladding, enabling the nuclear fuel to maintain stability for a certain period of time under harsh accident conditions without causing serious consequences, thus providing nuclear reactor operator with more response time. To increase the safety margin of fuel failure, ATF pellets have set research and development goals in terms of thermal conductivity, fission product containment capacity, mechanical properties, irradiation stability, and whether they are conducive to fuel cycle, aiming to enhance the performance of ATF pellets. Since 2011, the research and development of ATF has become the most important research key in the field of nuclear fuels internationally. In recent years, a large number of research works have been carried out on fuel pellets and cladding materials. Extant research has revealed that the addition of a small amount of Cr2O3 to the fuel pellet UO2 can increase the distance for fission gas to travel to its crystal surface, effectively inhibiting the release of fission gas (Zhu et al., 2011; Dehaudt and Lemaignan, 1988). This is because the introduction of Cr2O3 not only alters the grain size of UO2, which subsequently affects the diffusion distance of fission gas, but also creates point defects that modify the diffusion coefficient of fission gas (Dehaudt and Lemaignan, 1988). Apart from enhancing the capacity for fission gas retention, the incorporation of a small amount of Cr2O3 in UO2 has also demonstrated remarkable capabilities in improving PCI performance, as well as enhancing oxidation resistance and wear resistance (Pramanika et al., 2010). There are two main research directions for cladding materials: one is to enhance the high-temperature oxidation resistance of zirconium alloy cladding, and the other is to develop cladding materials with high strength, excellent oxidation resistance, and corrosion resistance. From the perspective of engineering demonstration and implementation, applying a coating treatment to the surface of zirconium alloy represents a relatively feasible technical approach, which adheres well with a zirconium matrix, improve mechanical properties, have minimal impact on the neutron economy of the cladding tube (Umretiya et al., 2020; Fejt et al., 2019), and provide high oxidation resistance by forming a protective layer of Cr2O3 on the surface (Yook et al., 2022; Yang et al., 2022; Bourdon et al., 2019). The Cr-coated zirconium alloy claddings are near-term approach for accident tolerant fuel (ATF) claddings (Kim et al., 2023).
Apart from fundamental research, numerous research reactor and commercial reactor irradiation tests have been conducted internationally on Accident Tolerant Fuel (ATF): France’s Framatome and the US’s Westinghouse began commercial reactor irradiation testing of large-grain UO2 pellets in the late 1990s, successively conducting performance tests on ATF pellets and cladding materials in the Byron 2 and Vogtle 2 units in the United States (Freebairn, 2017; Champlin, 2018; Cui, 2024). In January 2019, Russia initiated the irradiation testing of the first batch of ATF experimental assemblies in the MIR research reactor at the Research Institute of Atomic Reactors (RIAR), followed by the reloading and restart of the Rostov Nuclear Power Plant’s Unit 2 with the first batch of ATF in September 2021, officially commencing the first irradiation test of ATF in a commercial reactor. In January 2019, China successfully loaded its independently developed and designed ATF pins into a research reactor for irradiation testing, marking the country’s first in-reactor irradiation of ATF fuel. This experiment provided reliable data and strong support for the subsequent loading of pilot rod assemblies. After a series of research and safety evaluations, the ATF pilot rod assemblies were successfully loaded into a commercial reactor for irradiation in 2023, a significant milestone representing a crucial step following the ATF pin irradiation tests in research reactors.
The irradiation testing of lead test assembly (LTA) is an essential stage for new nuclear fuel to transition from research to application, necessitating thorough evaluations during the research phase to ensure the safety of LTA irradiation. Through preliminary exploration and comparison, this study focuses on ATF products with Cr coating on the zirconium alloy cladding surface and the addition of a small amount of Cr2O3 to UO2 pellet. Unlike traditional fuel assemblies, some fuel rods in the ATF assemblies have Cr coating on the zirconium alloy cladding surface, and some fuel pellets contain a small amount of Cr2O3, making them large-grain pellets. The sensitivity analysis is conducted on the influencing factors of the assembly models, and the neutronics characteristics of the LTA are quantitatively evaluated. Based on these characteristics, a generalized equivalent physical model is established. Furthermore, an optimized fuel management strategy is developed based on the irradiation testing objectives and requirements of LTA. A design method for the irradiation testing loading pattern is established, and three-cycle reactor loading pattern are designed in accordance with the core design criteria and the irradiation testing objectives and requirements of LTA. Reasonable suggestions are provided for the location of LTA in each cycle, and the impact of the LTA on the core is analyzed.
2 Analyze objects and methods
2.1 Core overview
The LTA are planned to undergo irradiation testing in the N-th to (N+2)-th cycles of a nuclear power plant, where the N-th cycle is a balanced cycle with an IN-OUT low-leakage loading pattern. The reactor is loaded with 157 fuel assemblies, with the enrichment of the new fuel assemblies at 4.45%. The fuel assembly is arranged in a 17 by 17 square and contains 264 fuel rods, 24 guide tubes for the control rods and neutron source, and one instrument tube. The height of the core active section is 3.658 m. The assemblies are classified into fuel assemblies containing 8, 16, and 20 gadolinium-containing fuel rods based on the number of gadolinium fuel rods they contain. The LTA is a non-gadolinium-containing assembly. Table 1 presents the design parameters for the reactor core.
2.2 LTA assembly model
Several irradiation test assemblies are proposed based on Irradiation testing requirements and feasibility of engineering, considering factors such Cr coating on the zirconium alloy cladding surface, pellet type, and the number of ATF pilot rods. The number of coated rods and large-grain UO2 rods of one assembly in various models is shown in Table 2, and the description of material for LTA is in Table 3. As shown in Figure 1, the ATF pilot rods are arranged at the center of the assembly’s edges, and the coordinates of four fuel rod are (9, 1), (9, 17), (1, 9) and (17, 9).
2.3 Calculation method and code
The deterministic software PCM (Lu et al., 2024) and the Monte Carlo code OpenMC (Romano and Forget, 2013) are used in this work. PCM software package is a 3D nuclear core design software, consisting of assembly cross section calculation code PINE and core design code COCO. The main function of PINE code is to perform 2D transport and burn-up calculations for PWR fuel assemblies, providing a homogenized assembly neutron cross section for core calculations code COCO. The database of 69 group cross sections published by the WLUP project of the International Atomic Energy Agency is adopted. The characteristic line method (MOC) is used for neutron transport calculation, and The energy spectrum of the assembly is modified by B1 approximation model (Li et al., 2022). Based on the neutron cross section library provided by PINE, COCO uses the Green function nodal method (NGFM) to solve the three-dimensional neutron diffusion equation. In the cross section library, the cross section is stored in the form of polynomial expansion coefficients, COCO can obtain the accurate cross-section data according to the actual status of the core. The functions of the COCO include core modeling and fuel depletion calculation, refueling calculation, reactivity coefficient solving, control rod worth differential value calculation, and assembly power reconstruction calculation (Lu et al., 2017; Lu et al., 2013). OpenMC is a community-developed Monte Carlo neutron and photon transport simulation code. It is capable of performing fixed source, k-eigenvalue on models built using constructive solid geometry. And it supports both continuous-energy and multigroup transport, which can solving key problems in the field of reactor physics.
3 Fuel management strategy and radiation testing principles
3.1 Fuel management strategy optimization
The irradiation testing of ATF assemblies in commercial reactors necessitates multiple cycles to achieve the target burnup depth, and it also requires a rational design to acquire irradiation data across multiple burnup steps. Therefore, in addition to meeting the energy demand of the power plant, nuclear design criteria, and safety evaluation criteria for fuel replacement, the design of the loading pattern for ATF assemblies in commercial reactors must also take into account the irradiation testing objectives and plans of the ATF assemblies. The superposition and limitations of multiple factors pose additional challenges to the design of the loading pattern for in-reactor irradiation. Taking into account economic efficiency, safety, and irradiation testing requirements, an evaluation process for the loading pattern suitable for the irradiation testing of ATF assemblies has been designed, as illustrated in Figure 2.
The design of the reactor core loading pattern involves several key technical processes. Firstly, based on the general fuel management strategy, the number of reactor cycles and the energy demand for each cycle are determined, and the appropriate number of batch assemblies and new assembly layout are matched accordingly. Before searching for a fuel management plan, the power plant will formulate the energy demand for each cycle based on the grid requirements. By considering the number and type of new assemblies available, a reasonable new assembly layout is developed in accordance with the requirements of the fuel management strategy, and an initial search for the core loading pattern is conducted. Secondly, the locations for the LTA are determined. According to the reactor loading pattern designed by the first step, suitable placement positions for the ATF assemblies are selected based on their number, type, and irradiation requirements. At the same time, it is necessary to design a reasonable rotational symmetry mode for LTA based on the arrangement of each cycle and burnup data considering to be obtained, which is convenient for the unloading and inspection of assemblies in subsequent cycles. Finally, the design criteria, safety criteria, and irradiation test targets are verified. Once the reactor core loading pattern is determined, it is necessary to check the design criteria, safety criteria, and irradiation test targets, including the nuclear enthalpy rise factor, moderator temperature coefficient, critical boron concentration, burnup of the irradiated assemblies after removal from the reactor, and the maximum discharge burnup of the assemblies. After satisfying all safety limitation of these parameters, the reactor loading pattern can be finalized.
3.2 Principle and objective of irradiation test
In addition to its high safety characteristics, Accident-Tolerant Fuels (ATF) with higher uranium enrichment ratios and burnup have the potential to extend the refueling cycle of pressurized water reactors. Therefore, in order to obtain irradiation test data for higher burnup, the following goals and principles for ATF irradiation testing in the reactor have been established:
(1) Principle of high burnup: Optimize the core loading pattern to make the assembly burnup and rod burnup to higher as much as possible, while avoiding the ATF fuel assembly becoming the hotest assembly in the core during irradiation, ensuring sufficient safety margins.
(2) Principle of thorough testing: Arrange the number of irradiation test rods reasonably to validate their irradiation performance with minimal impact on the core fully and comprehensively, aiming to obtain sufficient irradiation performance data for analyzing and supporting the research on ATF characteristics.
(3) The arrangement of ATF rods in the assembly should facilitate inspection for fuel assemblies poolside inspection: Fuel assemblies poolside inspection (PSI) is an important detection method to obtain data on the appearance and dimensional fuel assemblies at the site. It is a crucial guarantee for smoothly advancing the process of independent fuel assembly design and localization of material manufacturing. Because PSI is during the overhaul of the nuclear power plant, the arrangement of pilot rods within the fuel assembly should minimize the time required for poolside inspection while ensuring sufficient irradiation performance test data.
4 Irradiation scheme design and neutron analysis
4.1 Sensitivity analysis and equivalent physical model designed
The kinf is the infinite medium multiplication factor, commonly used to describe the neutron multiplication of a system without neutron leakage losses. This parameter is very important in nuclear physics and nuclear engineering because it is directly related to the sustainability and efficiency of nuclear reactions. The parameter kinf depends on the composition and structure of the system, independent of the geometry and size of the system. For a reactor with finite size, since the neutron non-leakage probability is always less than 1, kinf must be greater than 1 to maintain the chain reaction.
To quantitatively analyze the differences on kinf between the assemblies that shown in Table 2, deterministic code and Monte Carlo code are used to establish assembly models, and the impact of single influencing factors on the reactivity of the assemblies is analyzed. We set the tradition fuel assembly as the reference model, Table 4 shows the kinf values of each assembly model analyzed by the Monte Carlo code and give the differences compared to the reference model.
From Table 4, the effect of Cr coating on kinf can be summarized from model 2, model 3 and model 4. First, comparing the assembly reactivity results of model 2 and model 3 in Table 2, it can be found that the difference of reactivity between the two assembly models is 5 pcm. According to the description of the assembly model shown in Table 1, there are four Cr coating large-grain UO2 fuel rods in model 2 and four large-grain UO2 fuel rods in model 3, so the total reactivity penalty introduced by the coating on the four fuel rods is 5 pcm. Similarly, Comparing the assembly reactivity of model 2 and model 4, model 4 contains one large-grain UO2 fuel rod and three Cr coating large-grain UO2 fuel rods, so the total reactivity penalty introduced by the Cr coating on the three fuel rods is 4pcm. Therefore, Combined model 2, model 3 and model 4, the total reactivity penalty introduced by one rod with Cr coating is about 1.25 pcm–1.33 pcm. Meanwhile, the effect of large-grain UO2 on kinf can be found by the results of reference model, model 1 and model 2. On the one hand, the total reactivity penalty introduced by the four large-grain UO2 fuel rods due to the addition of Cr2O3 is 5 pcm according to the results of the reference model and model 3. On the other hand, the difference of kinf is 1 pcm by comparing model 1 and model 2, which is due to two large-grain UO2 fuel rod. So, the effect of Cr2O3 added to each fuel rod on kinf is about 0.5pcm–1.25pcm.
Comparing the thermal-neutron capture cross sections of nuclide Cr and Zr in nuclear database, the capture cross section of the nulcide Zr is on the order of 100 barns less than those of Cr-50, Cr-52, Cr-53 and Cr-54 in the thermal energy region (1/v region), respectively (Alrwashdeh and Alameri, 2022). Due to the number of ATF pilot rod is small, the reactivity penalty compared with the reference model is small.
In addition to evaluate the effects of ATF on assembly reactivity, model 2 is selected as an objective to analyze the pin-by-pin radial power distribution of assembly, aiming to determine the influence of coated large-grain UO2 pilot rods on the radial power of the assembly. The deviation in rod power distribution between the LTA and the traditional fuel assembly is shown in Figure 3. Compared to traditional fuel rods, the biggest relative deviation of fuel rod power distribution caused by the combination of Cr coating and large-grain UO2 pellets is 0.52% in the coordinate of (9.17). Numerically, the power of the pilot rod is lower than that of the traditional fuel rod. This is because adding coating to zirconium alloy cladding and large-grain pellets to the UO2 fuel rods will increases neutron absorption, which aligns with physical laws.
Because the deterministic code will used in the core loading pattern and refueling design, the influence of various factors are also analyzed by the deterministic code to verified the reliability of the code. The results are summarized in Table 5. As can be seen from the table, the impacts of large-grain UO2 and coating analyzed by the deterministic code are generally consistent with those obtained from the Monte Carlo code.
Combining the results in Tables 4, 5, we can see that the addition of a small amount of coated fuel rods and large-grain fuel rods in the assemblies has a small effect on the fuel assembly reactivity, and it is initially judged that the neutronics characteristics of the LTA into the reactor are close to those of the traditional fuel assembly into the reactor. Since the deterministic code does not support the definition of two types of cladding materials in one assembly, assuming that the cladding of all the fuel rods in LTA is coated from the perspective of reactivity penalty, and two assembly models are obtained. The one is with the largest reactivity penalty (all fuel rod with coating), and the other one is without reactivity penalty (the traditional assembly model). The two models can be regarded as equivalent physical model, that can be used to indirectly assess the impact level of the LTA into the reactor.
4.2 Design of loading pattern
To comprehensively acquire the poolside inspection data of different burnup step of ATF fuel rods after irradiation in commercial reactors and minimize the impact on the key path during the overhaul period, a stepped burnup strategy is adopted to design the irradiation sequence of four sets of LTA.
During the irradiation testing of LTA, apart from fully considering the core design criteria, it is necessary to consider the irradiation testing objectives and the in-reactor arrangement of the test assemblies for the design of the core loading pattern. The limitation of multiple factors and special in-reactor requirements increase the difficulty of designing the core loading pattern. Therefore, based on the fuel management strategy and the energy demand of the nuclear power plant, along with the irradiation testing goals and principles, a three-cycle loading patterns have been designed, which are shown in Figure 4. And the initial design includes 76, 76, and 64 new assemblies in the N-th, (N+1)-th, and (N+2)-th cycles. Due to the different in-reactor arrangements of the four groups of LTA, the layout needs to follow the principle of 1/4 rotational symmetry according to the requirements of the refueling design. In the first cycle, the LTA are arranged at positions E13, J14, B07, and J02, where E13 adopts an independent layout strategy without a symmetrical relationship with the other three groups. The J14, B07, and J02 assemblies are arranged with 1/8 rotational symmetry, while J14 and B07 are arranged with 1/4 rotational symmetry. This layout ensures that assemblies 3 and 4 have the same testing arrangements in reactor, while the independent irradiation plan for assembly 1 will not be affected. Additionally, to minimize the impact of the LTA, the four groups assemblies are arranged in four quadrants of the core. In the second cycle, the position of the assemblies 2, 3 and 4 in the reactor are L12, D05, and L04. Since the second LTA should be irradiated for three cycles to obtain high burnup data, it is necessary to put it at a reasonable location in the third cycle to reduce the risk of exceeding the burnup limit. Therefore, the No. 1 and No. 2 LTA are arranged in the position of H09 and H01. The usage of assemblies in each cycle is shown in Table 6.
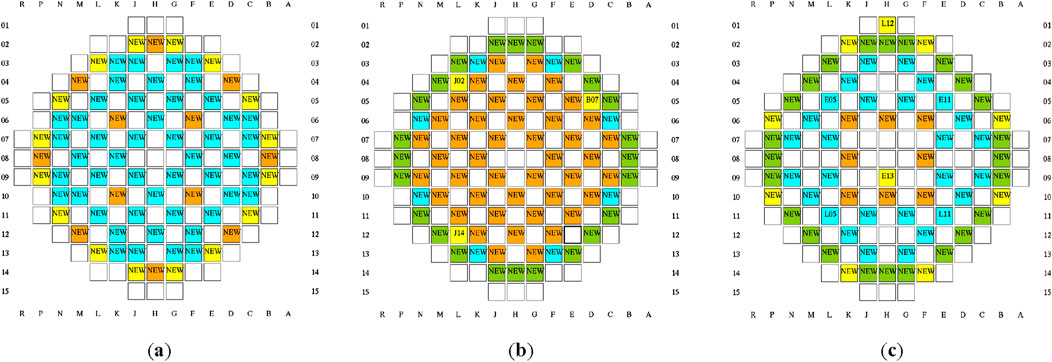
Figure 4. (A) The loading pattern for cycle N; (B) The loading pattern for cycle N+1; (C) The loading pattern for cycle N+2.
4.3 Evaluation of nuclear design criteria
The characteristic neutron parameters of the core loading pattern mainly include cycle length, nuclear enthalpy rise factor (FDH), moderator temperature coefficient (MTC), critical boron concentration (CB), shutdown margin (SDM), etc. These parameters are crucial to the safe and stable operation of the reactor and must be validated during fuel management and loading pattern search. In the field of reactor physic, the definition of cycle length is the duration for which a reactor operates at full power after one fuel loading, and this parameter is directly related to the economy of nuclear power plant. The FDH is closely related to the power distribution of the reactor. The operation state of the reactor can be evaluated and monitored effectively through regular confirmation and supervision of key safety parameters such as the FDH, so as to ensure the safe operation of the reactor. The MTC is the reactivity change per unit temperature change of the reactor moderator, and a negative moderator temperature coefficient endows the reactor with inherent stability, which is a safety feature in reactor design. The CB, as it is defined, is the boron concentration required to keep the reactor in a critical state by adjusting the boric acid concentration during reactor operation. To avoid issues caused by excessively high or low boron concentrations, which can lead to either sub-critical or overcritical, careful control and monitoring are essential. Finally is SDM, defined as the negative reactivity achieved when all control rods are inserted into the reactor core, is a crucial indicator of a reactor’s safety performance. It ensures that even in extreme situations such as control rod failure or other events leading to increased reactivity, the reactor remains within safe limits to prevent uncontrolled chain reactions.
From the previous sensitivity analysis of assembly, we already know that the neutron characteristic of the LTA can be expressed by equivalent physical models. So we evaluate the effect the characteristic neutron parameters by using the equivalent physical model and a core loading pattern. The comparison results of cycle length, FDH, MTC, CB, SDM, and burnup of the irradiated assemblies after removal from the reactor are shown in Table 7. We can see that the difference of numerical value of all neutron parameters is small when the equivalent physical model is loading in reactor. Because the neutron characteristic of LTA is between the two equivalent physical models, but is close to traditional fuel assembly, the influence of the LTA on the neutron parameters compared traditional fuel assembly will be lower than the values in Table 7. In addition, safety margin of distance from design criteria for all characteristic neutron parameters are also presented. Combining the results of the three cycles, the design values of parameters such as FDH, CB, and SDM et al. have at least 11.5%, 3.3%, and 31% safety margins compared to the safety limits, respectively. Therefore, the results from Table 7 demonstrate that the energy demands have been met to satisfy the power plant’s needs, and it is also indicate compliance with safety-related design criteria for the core. For instance, the MTC results demonstrate that the core still possesses negative feedback characteristics, the FDH results show that the core power distribution is well-flattened, and the SDM results indicate that the control rods can provide sufficient negative reactivity margin to ensure reactor shutdown capability during emergency conditions.
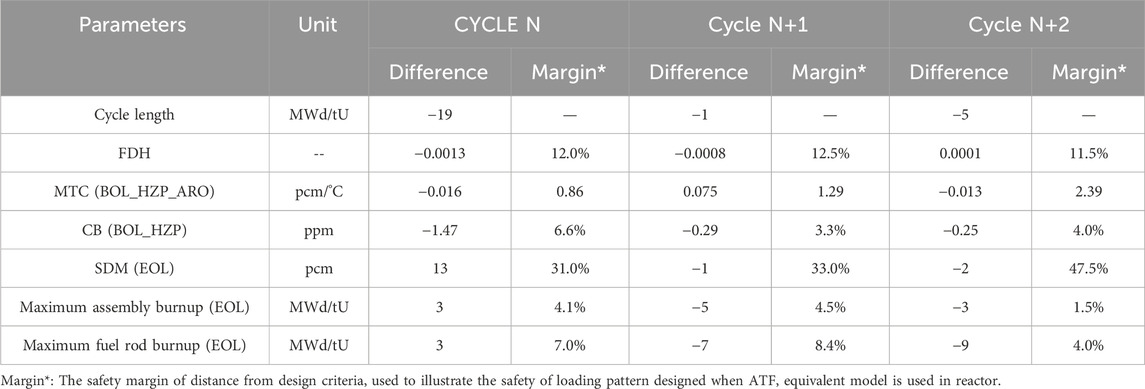
Table 7. Comparison results of characteristic neutron parameters and safety margin of distance from design criteria of three cycle using equivalent physical models in reactor.
The calculation results of the axial burnup distribution and axial power distribution of the maximum burnup assembly in reactor will be used for fuel performance analysis and safety analysis. Therefore, in addition to the above neutron parameters in Table 7, the comparison results of the axial burnup distribution and axial power distribution of the maximum burned-up assembly with equivalent physical model are shown in Figure 5 and Table 8, respectively. Through the calculation results, it is found that the positions of the maximum burned-up assemblies after the irradiation of the equivalent physical models in three cycles are same (position K07 in cycle N, position H08 in cycle N+1, position A08 in cycle N+2). The difference in the axial burnup distribution of the maximum burnup assembly in the reactor is very small, with a difference within the range of −10 to 7 MWd/tU in Figure 5. The comparison results of axial power distribution of some special assemblies are shown in Table 8, and the maximum deviation in the axial power distribution of the maximum burned-up assembly over its entire lifetime is only 0.59%, and the maximum deviation in the axial power distribution of all test assemblies over their entire lifetimes is 2.12%.
5 Conclusion
In this paper, considering the economy, safety and irradiation test requirements, we design a loading pattern evaluation process, which is applicable for the irradiation test of ATF into reactor. In addition, the sensitivity analysis of zirconium alloy cladding coating and pellet additive for ATF are carried out using deterministic code and Monte Carlo code and the impacts of large-grain UO2 and coating on reactivity of assembly are around -1pcm and -2pcm, respectively. Simultaneously, the equivalent physical model of LTA are established based on analysis software applicability and diversity of LTA. Finally, the nuclear reactor loading patterns of three cycle for the irradiation of LTA are designed based on the stepped burnup in-reactor strategy and the loading pattern evaluation process. And the effects of LTA on the nuclear design parameters, the axial burnup distribution and the axial power distribution of the deepest burning assembly are evaluated indirectly by the equivalent physical model based on the same loading pattern. From the results, we can conclude that small number of LTA into reactor has minor impacts on nuclear design parameters and critical safety parameters. And the designed loading pattern evaluation process and equivalent model analysis method of LTA designed in this work are applicable to the irradiation test of ATF into the reactor, which can provide guidance and reference for subsequent engineering demonstrations of the irradiation testing of ATF assemblies.
Data availability statement
The original contributions presented in the study are included in the article/supplementary material, further inquiries can be directed to the corresponding author.
Author contributions
QG: Conceptualization, Data curation, Formal Analysis, Investigation, Methodology, Software, Validation, Visualization, Writing–original draft, Writing–review and editing. YZ: Data curation, Methodology, Supervision, Writing–review and editing. ZL: Data curation, Software, Writing–review and editing. TJ: Validation, Visualization, Writing–review and editing. CZ: Funding acquisition, Project administration, Resources, Supervision, Writing–review and editing.
Funding
The author(s) declare that no financial support was received for the research, authorship, and/or publication of this article.
Conflict of interest
Authors QG, YZ, ZL, TJ, and CZ were employed by China Nuclear Power Technology Research Institute Co., Ltd.
Publisher’s note
All claims expressed in this article are solely those of the authors and do not necessarily represent those of their affiliated organizations, or those of the publisher, the editors and the reviewers. Any product that may be evaluated in this article, or claim that may be made by its manufacturer, is not guaranteed or endorsed by the publisher.
References
Alrwashdeh, M., and Alameri, S. A. (2022). Chromium-coated zirconium cladding neutronics impact for APR-1400 reactor core. Energies 15, 8008. doi:10.3390/en15218008
Bourdon, G., Sevecek, M., Krejcí, J., and Cvrcek, L. (2019). High-temperature steam and air oxidation of chromium-coated optimized Zirlo™. Acta Polytech. CTU Proc. 24, 1–8. doi:10.14311/app.2019.24.0001
Champlin, P. A. (2018). Techno-economic evaluation of cross-cutting technologies for cost reduction in nuclear power plants. Thesis. Massachusetts Institute of Technology. https://dspace.mit.edu/handle/1721.1/119044.
Cui, H. (2024). Fuel combination optimization model of thermal power plant based on new particle swarm optimization algorithm. J. Phys. Conf. Ser. IOP Publ. 2704 (1), 012005. doi:10.1088/1742-6596/2704/1/012005
Dehaudt, P., and Lemaignan, C. (1988). Advances in pellets technology for improved performance at high burnup, IAEA TECDOC-1036. Vienna: IAEA.
Fejt, F., Sevecek, M., Frýbort, J., and Novak, O. (2019). Study on neutronics of VVER-1200 with accident tolerant fuel cladding. Ann. Nucl. Energy 124, 579–591. doi:10.1016/j.anucene.2018.10.040
Freebairn, W. (2017). Areva accident tolerant fuel test assemblies to be loaded at Vogtle-2 in 2019. Platts Nucl. Fuel 42 (15), 2–3.
Kim, D., Steinbrück, M., Grosse, M., Tang, C., and Lee, Y. (2023). Eutectic reaction and oxidation behavior of Cr-coated Zircaloy-4 accident-tolerant fuel cladding under various heating rates. J. Nucl. Mater. 583, 154538. doi:10.1016/j.jnucmat.2023.154538
Li, J., Wang, C., Chen, J., and Peng, J. (2022). Development and verification of fuel assembly bowing model in software package PCM. High Power Laser Part. Beams 34, 026004. doi:10.11884/HPLPB202234.210378
Lu, G., Ding, M., Lan, B., Pan, X., Li, C., Wang, C., et al. (2024). Verification of PCM nuclear design code for whole core calculations. Nucl. Power Eng. 45 (4), 17–23. doi:10.13832/j.jnpe.2024.04.0017
Lu, H. L., Chen, J., Wang, J. L., Cai, L., and Gao, S. N. (2017). Verification and validation of self-reliant core nuclear design code COCO. Atomic Energy Sci. Technol. 51 (8), 1459–1463. doi:10.7538/yzk.2017.51.08.1459
Lu, H. L., Mo, K., Li, W. H., and Li, J. G. (2013). Dedevopment of self-reliant three-dimensional core nuclear design code COCO. Atomic Energy Sci. Technol. 47. doi:10.7538/yzk.2013.47.S0.0327
Pramanika, D., Ravindranb, M., and Rao, G. V. S. H. (2010). IAEA-TECDOC-1654 advanced fuel pellet materials and fuel rod design for water cooled reactors. Vienna: IAEA.
Romano, P. K., and Forget, B. (2013). The OpenMC Monte Carlo particle transport code. Ann. Nucl. Energy 51, 274–281. doi:10.1016/j.anucene.2012.06.040
Umretiya, R. V., Elward, B., Lee, D., Anderson, M., Rebak, R. B., and Rojas, J. V. (2020). Mechanical and chemical properties of PVD and cold spray Cr-coatings on Zircaloy-4. J. Nucl. Mater. 541, 152420. doi:10.1016/j.jnucmat.2020.152420
Yang, J., Steinbrück, M., Tang, C., Große, M., Liu, J., Zhang, J., et al. (2022). Review on chromium coated zirconium alloy accident tolerant fuel cladding. J. Alloys Compd. 895, 162450. doi:10.1016/j.jallcom.2021.162450
Yook, H., Shirvan, K., Phillips, B., and Lee, Y. (2022). Post-LOCA ductility of Cr-coated cladding and its embrittlement limit. J. Nucl. Mater. 558, 153354. doi:10.1016/j.jnucmat.2021.153354
Keywords: accident-tolerant fuel, lead test assemblies, equivalent physical model, fuel management strategy, neutron analysis
Citation: Gao Q, Zhu Y, Li Z, Jiang T and Zhao C (2024) Study on fuel management strategy for accident-tolerant fuel on in-pile irradiation test. Front. Energy Res. 12:1463183. doi: 10.3389/fenrg.2024.1463183
Received: 11 July 2024; Accepted: 30 September 2024;
Published: 09 October 2024.
Edited by:
Turgay Korkut, Sinop University, TürkiyeReviewed by:
Mohammad Alrwashdeh, Emirates Nuclear Technology Center, Khalifa University, United Arab EmiratesChongchong Tang, Karlsruhe Institute of Technology (KIT), Germany
Copyright © 2024 Gao, Zhu, Li, Jiang and Zhao. This is an open-access article distributed under the terms of the Creative Commons Attribution License (CC BY). The use, distribution or reproduction in other forums is permitted, provided the original author(s) and the copyright owner(s) are credited and that the original publication in this journal is cited, in accordance with accepted academic practice. No use, distribution or reproduction is permitted which does not comply with these terms.
*Correspondence: Changyou Zhao, emhhb2NoYW5neW91QGNnbnBjLmNvbS5jbg==