- 1Division of Environmental Science and Dept. of Biosciences, Rajagiri College of Social Sciences, Cochin, Kerala, India
- 2Institute of Bioresources and Sustainable Development, Imphal, India
- 3Microbial Processes and Technology Division, CSIR-National Institute for Interdisciplinary Science and Technology, Trivandrum, India
Bamboo is a perennial rapid-growing plant that is given preference for renewable biosources for biofuels and bio-based chemical conversion. Bamboos are rich in cellulose and have highly recalcitrant biomass due to high lignin. Bamboo is abundantly available in Northeastern India and can be utilized as a feedstock biofuels. Here, we evaluated the pretreatment of bamboo residues Dendrocalamus strictus with different concentrations of alkali, hydrogen peroxide, and alkaline hydrogen peroxide and its influence on biomass digestibility for enhancement of sugar recovery with Celic C cellulase enzyme blend. Enzymatic hydrolysis data indicated untreated raw biomass showed a digestibility of 40% after 48 h of incubation. The biomass pretreated with alkali showed a maximum digestibility of 61% obtained from 10% loaded with 0.5% w/v NaOH. Pretreatment of the bamboo with H2O2 shows a maximum digestibility of 75% from biomass loaded with 1% w/v of H2O2. Combinational pretreatment of alkaline hydrogen peroxide showed a maximum efficiency of biomass digestibility of 83% attained from biomass loaded with 1% w/v NaOH-H2O2. Crystallinity index (CrI) analysis showed that CrI increased from 64% to 70.75% in pretreated biomass. FTIR and SEM analysis show changes in functional groups, morphology, and surface of biomass in pretreated biomass. Compositional analysis shows that 68% of lignin removal is obtained from alkaline hydrogen peroxide pretreatment. Cellulose content increased from 52% to 65%, and hemicellulose decreased from 18.6% to 8.6%. Results indicated that the potential possibility of bamboo waste biomass as feedstock for biorefinery products and alkaline hydrogen peroxide pretreatment methods is an efficient strategy for sugar recovery for bioethanol production.
1 Introduction
The conversion of lignocellulosic biomass (LCB) into biochemicals, biofuels, and materials is a feasible and sustainable approach to reducing dependency on fossil fuels and the concerns associated with the energy crisis and environmental carbon emissions. Accelerated global warming is associated with environmental damage, and increasing pollution, and health issues among people due to the extensive use of fossil fuels. These issues are resolved by encouraging the use of renewable energy sources such as solar, wind, and fuel cells including biofuels (Deshmukh and Pathan, 2024). Biofuels are alternative, extensively preferred fuels against fossil fuels due to their low pollution emissions, oxygenation advantage, and renewable nature. Many countries, including India, blend 10% of biofuels (bioethanol) with gasoline.
Bamboo is a perennial, fast-growing plant that is considered an alternative sustainable source for second-generation biofuels and biobased chemicals (Ummalyma et al., 2022). Bamboo biomass gained interest as a potent raw material for bioethanol due to its rapid growth, no need for extra fertilizer, and resilience to extreme environmental conditions (Chaowana, 2013; Laltha et al., 2021). Generally, the household processing industry uses bamboo for its materials and other purposes. Processing bamboo into furniture items generates a significant amount of bamboo waste. Often, people simply burn the produced bamboo residues to generate heat or landfill them. The waste bamboo residues are enriched with carbohydrates and polymers such as cellulose and hemicellulose; thus, they can be further processed in biological and thermochemical ways to generate biofuels and biomaterials (Hou et al., 2024; Zheng et al., 2021).
The lignocellulosic processing for biorefinery mainly comprises pretreatment, hydrolysis (enzymatic), and fermentation. Lignin, hemicellulose, and cellulose make up the majority of LCBs. Chemically strong bonds enwrap the hemicellulose and lignin together, sealing the cellulose and creating biomass recalcitrance (Huang et al., 2022; Ummalyma et al., 2021; Sahoo et al., 2017). Thus, the recalcitrance and presence of high lignin in bamboo biomass require pretreatment before conversion into any products, especially via biochemical platforms. However, biorefineries consider the pretreatment process as a crucial step, aiming to reveal the cellulose structure of the biomass and enhance the subsequent enzymatic hydrolytic process of the biomass (Huang et al., 2022). Different pretreatment technologies are adapted for bamboo biomass, such as alkali, steam explosion, organosolv, dilute acid, and biological pretreatment processes (Huang et al., 2022; Alam et al., 2019; Lai et al., 2019). Yang et al. (2017) reported that pretreatment costs currently account for approximately 20% of the cost of biofuel processing. Hence, research on low-cost, efficient, and eco-friendly pretreatment of biomass is still ongoing.
Alkali pretreatments aid cellulose saccharification by removing lignin efficiently and providing chemical swelling of cellulose fibers (Li et al., 2014). Hydrogen peroxide is an oxidative green reagent for the pretreatment of biomass, and it has better enzymatic digestibility in biomass due to extraordinary delignification (Alexandropoulou et al., 2023; Zhan et al., 2022). Alkali peroxide pretreatment is similar to sodium hydroxide or Kraft pulping, which is used for the delignification process that helps to solubilize and extract lignin from the biomass (Mittal et al., 2017). During alkaline hydrogen peroxide pretreatment under basic conditions, hydrogen peroxide (H2O2) can break down to release hydroxyl (HO−) and superoxide (OO−) anion radical species, which oxidize and cleave lignin into small monomers (Huang et al., 2022; Zhang et al., 2013; Ali et al., 2019). Simultaneously, hemicellulose is also broken down from the biomass by the action of H2O2 (Klinke et al., 2002). This pretreatment process is efficient for lignin removal under mild conditions with low chemical dose and temperature. Alkaline peroxide is a green reagent system due to its fast break down into oxygen and water molecules; hence, there is no residual H2O2 available in the pretreated biomass (Rabelo et al., 2008). A previous study showed that this pretreatment breaks up lignin’s ester and ether links by a large amount, while keeping cellulose and partially breaking down hemicellulose (Li et al., 2014).
However, the present investigation aims to compare the effect of different pretreatment processes of alkali (NaOH), hydrogen peroxide (H2O2), and alkaline hydrogen peroxide (NaOH-H2O2) on local bamboo residues of Dendrocalamus strictus, locally known as unan. The impact of pretreatment reagents on biomass composition and functional moieties by FTIR, biomass surface change by SEM, and crystallinity index by XRD analysis are performed. The biomass digestibility of differentially pretreated biomass is correlated with its suitability for biofuel applications.
2 Materials and methods
2.1 Materials
Chemicals like sodium hydroxide, H2O2, tween 80, and other chemicals required for the experiments are purchased from Merck. The enzyme cellulase (Cellic CTec2; 250 FPU/ml) from Novozymes was purchased from Sigma, India.
2.1.1 Biomass collection
Bamboo residues belong to D. strictus, obtained from Imphal Market, where they sell bamboo poles for building houses. The collected residues are dried in sunlight. The size reduction of biomass was done with a kitchen blender, and biomass powder was sieved through a 1 mm sieve. The moisture content of the biomass was 8.9%, and it was kept in a plastic container at room temperature (23°C–25°C) until further use.
2.1.2 Biomass pretreatment
The pretreatment of bamboo residues was performed in a 250 mL beaker (Borosil) loaded with 10% biomass and different concentrations ranging from 0.2%–1.2% (w/v) of NaOH, H2O2, and a combination of NaOH- H2O2. The reaction mixture was thoroughly mixed and autoclaved at 121°C for 60 min. After cooling, samples were washed several times using tap water until reached a neutral pH. Solid-liquid separations were performed using a nylon sieve. Recovered solids are air-dried at room temperature and stored in an airtight container until further use.
2.1.3 Compositional analysis
The component analysis of native and pretreated samples was conducted based on the protocol described by the National Renewable Energy Laboratory for analyzing biomass materials (Sluiter et al., 2011). Biomass sugars from final hydrolysis are quantified with a UFLC system with an RI detector (Shimadzu). A monosaccharide column of Rezex® RPM (Phenomenex) with deionized Milli-Q was used for the mobile phase at a flow rate of 0.8 mL/min and an oven temperature maintained at 80°C.
2.1.4 Biomass characterization and analysis
The efficiency of pretreatment chemicals on biomass structure, functional moieties, and crystallinity was analyzed by SEM, FTIR, and XRD.
2.1.4.1 SEM analysis
Scanning electron microscopy (SEM) analysis was conducted to gauge any alterations on the surface of biomass after each pretreatment process. Air-dried biomass samples were sputter coated with gold, and observations were done under an electron microscope of the Zeiss Evo 17 SE (Germany).
2.1.4.2 pXRD analysis
The crystallinity analyses of pretreated and native samples were conducted with an X-ray powder diffractometer (pXRD) with an Empyrean Cu LFF HR X-ray generator containing an X'Celerator detector (PANalytical Empyrean, the Netherlands). Tablets are made from pretreated and untreated samples. X-ray diffractograms are recorded from 10° to 88°, with a difference of 0.01°. The crystallinity index (CrI) of the samples was calculated based on the equations previously reported by Segal et al. (1959).
where 1002 represents the peaks of the crystalline part, which lie at 2 θ 22.5, and IAM is the intensity peak from the non-crystalline material (amorphous), which lies at approximately 18°angle in the valley between the peaks.
2.1.4.3 FTIR analysis
To evaluate the influence of the pretreatment process on biomass functional groups, FTIR analysis was performed. The untreated and pretreated powder samples were pelleted with KBr powder, and analysis was performed on a Bruker Alpha II diamond-ATR FT-IR spectrometer. The spectra are captured from an average of 20 scans in a range of 500–4,000 cm−1 with a 0.8 cm−1 resolution.
2.2 Enzymatic digestibility
The effectiveness of the pretreatment process is correlated with biomass digestibility. This enzymatic hydrolysis is conducted in a 150-mL screw-capped conical flask with 10% (w/w) pretreated biomass loaded with 20 FPU/g commercial cellulase mentioned in Section 2.1. The reaction mix was added with antibiotic solution and tween 80 as per the concentrations mentioned in Sahoo et al. (2017). The final reaction volume was 20 mL. The hydrolyzed samples were collected at regular intervals of 0–48 h, and the sugar released was quantified as per the methodology by Miller (1959). The enzymatic hydrolytic yield equation reported by Huang et al. (2022).
2.3 Fermentation
Hydrolysate obtained from the enzymatic hydrolysis of alkali-hydrogen peroxide pretreatment was used for fermentation studies for bioethanol production. The experiment was performed in a screw-cap conical flask (100 mL) containing 50 mL of hydrolysate. Hydrolysate was inoculated with wild yeast SFAYS (4% wet v/v). Samples are incubated at 30°C ± 2°C under static conditions. Samples are taken at 0–48 h and checked for sugar consumption and ethanol production. Ethanol produced was quantified using HPLC (Thermo Fisher Dionex ultimate 3,000) using Rezex ROA organic acid column as per the methodology reported by Ummalyma et al., 2022. All the experiments are conducted in triplicates and average mean values are represented.
3 Results and discussions
3.1 Impact of pretreatment on composition
Bamboo is an abundantly available bioresource in the northeastern parts of India, mostly used for furniture preparation, house fencing, and other purposes. The region’s bioeconomy can benefit from the sustainable use of bamboo processing residues as feedstock for biofuels and biomaterial conversion (Ummalyma et al., 2022). Hence, this biomass is used to evaluate it as a raw material for biorefinery products. Lignocellulose polymers are tightly intertwined to provide strong support for the cell wall. The entanglement of strong lignocellulose biomass compounds inhibits their enzymatic breakdown into low-molecular-building blocks. Furthermore, the occurrence of lignin inhibits the enzymatic hydrolytic process by binding to the cellulose surface (Tian et al., 2018; Song et al., 2020; Djajadi et al., 2018). For this conversion, an efficient pretreatment process is required to break down the strong bonds of lignocellulose. In the present study, three different pretreatment processes of D. strictus are studied, and their performance is compared in terms of solid recovery after pretreatment, biomass components of raw and differentially pretreated biomass, and enzymatic digestibility.
During the pretreatment process, approximately 80% of the solids are recovered from the alkali-pretreated biomass due to a reduction in lignin content, whereas in the H2O2 pretreatment process, 85% of the solids are recovered, and in the alkali-hydrogen peroxide pretreatment, 76% solids are recovered from the biomass. This could be due to the partial dissolution of lignin and hemicellulose in the pretreated biomass. The compositional analysis of untreated bamboo biomass showed 40% cellulose, 26.8% hemicellulose, and 30.5% hemicellulose, respectively. After alkaline pretreatment, a 25.7% rise in cellulose content and a 40.9% reduction in lignin and hemicellulose are not much affected. In the H2O2 pretreatment process, there was a 46.7% increase in cellulose, a 45.8% reduction in hemicellulose, and a 49.5% reduction in lignin removal, respectively. Cellulose recovery of 56.1%, 43.2% reduction of hemicellulose, and 54% reduction in lignin, respectively, were obtained from the alkaline hydrogen peroxide pretreatment process. This data is in concordance with an earlier report on bamboo pretreatment with different concentrations of H2O2 under alkaline conditions (Huang et al., 2022). Table 1 represents the biomass compositional analysis of D. strictus bamboo. After pretreatment with hydrogen peroxide, the combination of alkaline hydrogen peroxide assists in the degradation of lignin and xylan, while in the case of alkali pretreatment, the xylan content was unchanged. The cellulose content of biomass is increased by hydrogen peroxide and reaches its maximum in the alkaline hydrogen peroxide pretreatment. This is mainly attributed to the selective elimination of hemicellulose and lignin from the biomass, hence the enriched cellulose content in the biomass (Zhan et al., 2022). This observation is consistent with previous studies on the pretreatment of two bamboo species (Phyllostachysedulis and Neosinocalamusaffinis) with alkaline hydrogen peroxide treatment (Zhan et al., 2022). It has been reported that alkali pretreatment breaks the glycosidic and ester bonds in the cell wall matrix, which may lead to cellulose swelling and decrystallization. This further alters the lignin structure, along with the partial solvation of hemicellulose. Moreover, alkalis also assist in the hydrolysis of the ester bond between ferulic acids and hemicellulose, which causes the degradation of lignin and the swelling of lignocellulose particles (Brodeur et al., 2011). Under alkaline hydrogen peroxide conditions, reactive radicals of hydroxyl and superoxide anion are generated, which have very strong oxidant properties and attack lignin (Ho et al., 2019). Balat et al. (2008) show that alkali can dissociate the polymers from cell walls by breaking the hydrogen and covalent bonds.
3.2 Surface morphology analysis
To correlate with the influence of the pretreatment effect on the available area of biomass that affects the subsequent hydrolytic process. The SEM analysis was employed to compare the air-dried, pretreated, and native bamboo samples (Figure 1A). It was shown that the surface of untreated biomass is smooth and does not have visible fibers. The surface of alkali-pretreated bamboo showed cracks and a rough surface (Figure 1B). The surface of H2O2-pretreated biomass showed porousness; particles burst due to fibrillation (Figure 1C). This might be due to the partial degradation of lignin and hemicellulose. Samples pretreated with alkali hydrogen peroxide show the surface is rough and fibers are visible on the surface of the biomass (Figure 1D), which assists enzymes to easily adsorb on the surface of the biomass and enhances the hydrolytic process. The presence of porous fibers in the left-over cellulose-rich area was observed due to the significant removal of hemicellulose and lignin due to the combined action of alkaline hydrogen peroxide, as supported by previous research (Xu et al., 2020). SEM analysis is consistent with compositional analysis, which confirms that alkaline hydrogen peroxide pretreatment effectively disrupts the compact structure of lignocellulose, removes lignin, separates amorphous hemicellulose, and retains crystalline cellulose (Yin et al., 2022).
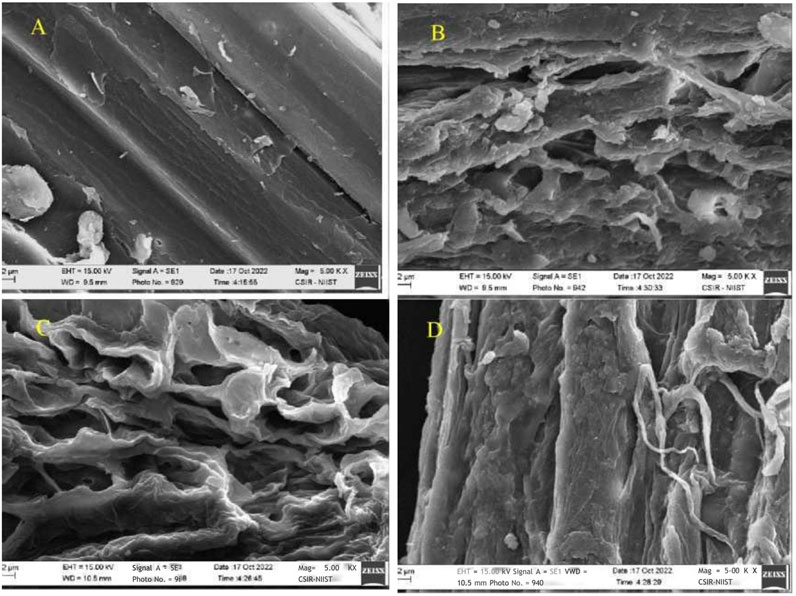
Figure 1. Scanning electron micrographs of morphological changes of pretreated and untreated bamboo residues (A) Untretaed; (B) Alkali; (C) Hydrogen peroxide; (D) Alkali hydrogen peroxide. Magnification of the image is 5.00kx.
3.3 Crystallinity analysis
The crystallinity of biomass is vital to consider for any fluctuations in the crystalline cellulose and its amorphous region of lignocellulosic which is used to access the enzymatic hydrolytic process of biomass. pXRD analyses are performed to correlate the effect of differentially pretreatment on biomass cellulose. XRD plots of pretreated biomass are shown in Figure 2. The expected changes in the crystalline index (CrI) of bamboo biomass are governed by the changes in three building blocks and the breach of plant cell wall structure (Xu et al., 2020). The CrI calculation showed raw bamboo biomass at 64%. During the differential pretreatment process with alkali, H2O2, and akali-H2O2, CrI increased from 64% to 70.75%. The CrI maximum of 70.75% obtained from an alkali-H2O2 pretreatment is probably due to the oxidation, depolymerization, and elimination of lignin and hemicellulose, which is consistent with the biomass chemical composition. The CrI of alkali alone showed a maximum of 68.8%, mainly due to the removal of lignin content from the biomass. Pretreatment with H2O2 showed a CrI of 66.2%; this could be because of the hemicellulose partial removal. The pXRD profile showed that the depolymerization of an amorphous region including both lignin and hemicellulose enhanced the increase in cellulose proportion, which further assisted the exposure of accessible sites for cellulase enzymes in further hydrolytic processes to release the fermentable sugars. This follows previous studies of bamboo biomass pretreated with hydrogen peroxide, acetic acid, alkali, and alkaline hydrogen peroxide (Meng et al., 2022; Xu et al., 2020; Qing et al., 2016). This increase in CrI is mainly due to the removal of non-crystalline parts such as hemicellulose and lignin and a surge in cellulose (Meng et al., 2022), or it could be due to the removal of some amorphous regions of cellulose besides lignin and hemicellulose content.
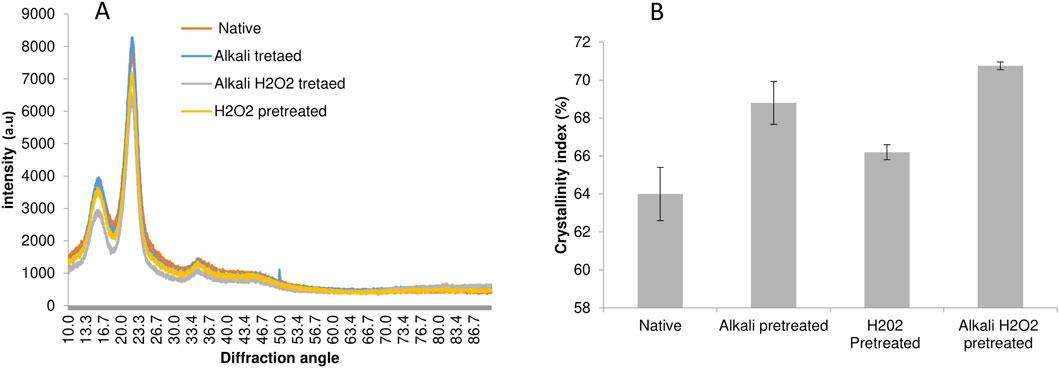
Figure 2. XRD Analysis of pretreated biomass (A) and crystallinity index pretreated biomass samples (B) (Average mean percentage values are expressed).
3.4 FTIR analysis
FTIR spectral analyses were further conducted to gauge the fluctuations in the functional groups of carbohydrates and lignin polymers in pretreated and untreated biomass (see Supplementary Figure S1). The vibration signal at 3,334 cm−1 and 2,916 cm−1 signifies the stretching of hydroxyl and C-H groups, respectively (Pandiyan et al., 2014; Zhan et al., 2022). The peak at 1,604 cm−1 represents the vibrational stretching of C = O from acetyl groups in the hemicelluloses. The intensity signals in the range of 1,450 cm−1, 1,505 cm−1, and 1,599 cm−1 are attributable to the benzene ring skeletal vibrations (Qing et al., 2016; Zhan et al., 2022). The peak shift is observed in the pretreated samples mainly due to the depolymerization of the lignin-carbohydrate complex, which is in agreement with the component analysis of the pretreated biomass. The intensity peak at 1,246 cm−1 was assigned to the ester linkages from lignin and hemicellulose (Sun et al., 2015). This peak is declining in pretreated biomass, which indicates the breakdown of polymers (Qing et al., 2016; Zhan et al., 2022). Peak intensity reduction at 896 cm−1 assigned to β-D glycosidic linkages from cellulose, which is widening in biomass, showing cellulose preserved (Liang et al., 2020). The absorption peaks at 1,635–1,640 cm−1 indicate the bending ambiances of absorbed water molecules. The results show that partial degradation of hemicellulose and lignin and the cellulose are intact during pretreatment, which further assists in the enzymatic hydrolytic process.
3.5 Enzymatic digestibility
Enzymatic digestibility is an important metric to correlate with pretreatment efficiency. Enzymatic hydrolytic efficacy is recognized as a critical gauge for arbitrating biomass recalcitrancy and the holistic process of pretreatment (Shen et al., 2019). The pretreated bamboo biomass was digested using a cellulase enzyme blend loading of 20 FPU/g substrate. Untreated raw biomass showed a digestibility of 40% after 48 h of incubation. The biomass pretreated with alkali showed a maximum digestibility of 61% obtained from 10% loaded with 0.5% w/v NaOH (Figure 3). This could be due to the partial removal of lignin from the alkali-pretreated biomass. The presence of lignin in the substrate restricts and blocks the cellulase’s accessibility to its substrate by steric hindrance, and the cellulase enzymes non-productive adsorption, which is one of the important limiting factors during the cellulose hydrolytic process (Huang et al., 2022; Meng et al., 2022; Lin et al., 2021). Pretreatment of the bamboo with H2O2 shows a maximum digestibility of 75% from biomass loaded with 1% w/v of H2O2 (Figure 4). This could be due to the partial removal of both lignin and hemicellulose from the substrate, which helps in the penetration of cellulase on the available exposed surface of cellulose for releasing fermentable sugars from the substrate. H2O2 is a green liquid that selectively oxidizes lignin and hemicellulose and depolymerizes them into monomeric units and degradation products during the pretreatment process (Teong et al., 2019). Zhang et al. (2013) highlighted that the removal of hemicellulose may help in the creation of pores in the substrate that expose residual cellulose that further assists the cellulose binding. Combinational pretreatment of alkaline hydrogen peroxide showed a maximum efficiency of biomass digestibility of 83% attained from biomass loaded with 1% w/v NaOH-H2O2 (Figure 5). A high concentration does not increase the hydrolytic efficiency. This high efficiency in biomass hydrolysis is mainly due to the removal of recalcitrance from the substrate by solubilizing the lignin and hemicellulose polymers, which facilitate the availability of cellulase enzymes to cellulose (Yao et al., 2022). The present data is in line with the previous report on bamboo biomass with NaOH-H2O2, and efficiency increased from 68.73 to 90 with an increasing concentration of H2O2 from 0%–3%. The study highlighted that 88.3% reported H2O2 in alkaline hydrogen peroxide pretreatment (Zhan et al., 2022). Identical results reported by Yang et al. (2017) showed that alkaline hydrogen peroxide pretreatment of corn stover had an enzymatic digestibility of 83.7%. The present study shows alkaline hydrogen peroxide is a better pretreatment process compared with alkali and hydrogen peroxide alone as a pretreatment chemical. It is a green solvent and effective for the removal of recalcitrance from bamboo biomass by depolymerizing lignin and hemicellulose and assisting in the release of maximum sugars for further application as biofuels and biochemicals.
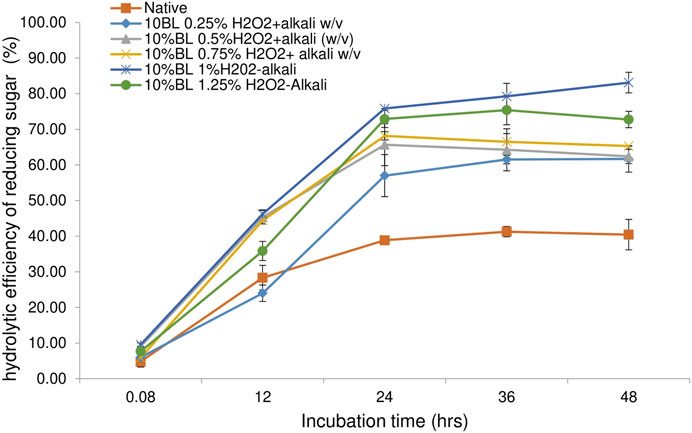
Figure 5. Enzymatic digestibility efficiency of alkaline hydrogen peroxide pretreated bamboo residues.
3.6 Fermentation
Enzymatic hydrolysates obtained from alkaline hydrogen peroxide-pretreated biomass are evaluated for their potential to produce bioethanol. Hydrolysates are used directly without any further modification, like the purification or removal of inhibitory chemicals released during the pretreatment process. After the inoculation of wild yeast cells, samples are withdrawn at regular intervals for sugar consumption, yeast growth, and ethanol concentration. As shown in Figure 6, the ethanol concentration reached a maximum of 2.1% with a fermentation efficiency of 90% ethanol yield at 36 h. When the incubation reached 48 h, the bioethanol yield reached 85.7%. The results agree with previous studies on the pretreatment of bamboo, which also produced a bioethanol yield of 87% (Jin et al., 2021). Hydrogen peroxide pretreatment of Pleioblastus amarus produced a maximum fermentation yield of 83.98% (Meng et al., 2022). Pretreatment of bamboo biomass from Neosinocalamus offinis culms with alkaline hydrogen peroxide produced a maximum ethanol yield of 86.14% (Huang et al., 2022). The pretreatment of the Dendrocalamus strictus biomass study is also in line with previous reports. The present study indicates that the alkaline hydrogen peroxide pretreatment process is an efficient green approach for obtaining sugars for biorefinery biochemical conversions, including bioethanol. The exploitation of bamboo biomass residues is an alternative, feasible, and sustainable solution for meeting biofuel demands.
In this study, we demonstrated the technical feasibility of pretreating bamboo with alkaline hydrogen peroxide. We need to optimize the high-solid loading for enzymatic hydrolysis to enhance its feasibility. For better technical feasibility and bioethanol yield, we need to optimize inhibitor generation during the pretreatment and hydrolysis processes. Bamboo residues from the bamboo processing industry will be suitable feedstock for biofuels from biorefineries. To improve bamboo waste utilization, we could optimize and valorize not only biofuels but also other possible bamboo-based byproducts, including bioethanol production, to improve the utilization of bamboo waste. We need to conduct a techno-economic analysis for a zero-waste biorefinery that aligns with the bamboo waste industry’s bioeconomy.
4 Conclusion
The study highlighted that bamboo residues are a feasible alternative for bioethanol production without compromising food crops. The enzymatic digestibility of pretreated biomass demonstrated that alkaline hydrogen peroxide treatment is a superior pretreatment process that removes lignin and hemicellulose and increases the crystallinity index, further facilitating substrate bioconversion. The morphology of the biomass revealed that the broken bamboo surfaces exposed more cellulose fibers, creating a favorable environment for cellulase enzymes to release sugars. We achieved a maximum sugar conversion of 83% and a fermentation efficiency of the ethanol yield ranging from 85.7% to 90%. The study highlighted that the alkaline hydrogen peroxide pretreatment process is an eco-friendly pretreatment approach for bamboo-based biorefineries.
Data availability statement
The original contributions presented in the study are included in the article/Supplementary Material, further inquiries can be directed to the corresponding author.
Author contributions
SU: Conceptualization, Formal Analysis, Methodology, Project administration, Resources, Supervision, Visualization, Writing–original draft, Writing–review and editing. NH: Methodology, Writing–review and editing. RS: Formal Analysis, Visualization, Writing–review and editing.
Funding
The author(s) declare that financial support was received for the research, authorship, and/or publication of this article. This study was supported by the core fund of Institute of Bioresources and Sustainable Development Core received through Department of Biotechnology, Govt.of India.
Acknowledgments
All authors are thankful to the Electron Microscopy Section and the Chemical Sciences Division of CSIR-National Institute for Interdisciplinary Science and Technology, Trivandrum, for their support for SEM and XRD analysis.
Conflict of interest
The authors declare that the research was conducted in the absence of any commercial or financial relationships that could be construed as a potential conflict of interest.
Publisher’s note
All claims expressed in this article are solely those of the authors and do not necessarily represent those of their affiliated organizations, or those of the publisher, the editors and the reviewers. Any product that may be evaluated in this article, or claim that may be made by its manufacturer, is not guaranteed or endorsed by the publisher.
Supplementary material
The Supplementary Material for this article can be found online at: https://www.frontiersin.org/articles/10.3389/fenrg.2024.1444813/full#supplementary-material
References
Alam, A., Zhang, R., Liu, P., Huang, J. F., Wang, Y. T., Hu, Z., et al. (2019). A finalized determinant for complete lignocellulose enzymatic saccharification potential to maximize bioethanol production in bioenergy Miscanthus. Biotechnol. Biofuels 12, 99. doi:10.1186/s13068-019-1437-4
Alexandropoulou, M., Antonopoulou, G., Ntaikou, I., and Lyberatos, (2023). The impact of alkaline/hydrogen peroxide pretreatment on hydrogen and methane production from biomasses of different origin: the case of willow sawdust and date palm fibers. Sust. Chem. Pharm. 32, 100971. doi:10.1016/j.scp.2023.100971
Ali, N., Giwa, A. S., Abdalla, M., and Liu, X. (2019). Alkaline hydrogen peroxide pretreatment of bamboo culm for improved enzymatic release of reducing sugars using recombinant cellulases. Cellulose 27 (2), 769–779. doi:10.1007/s10570-019-02829-8
Balat, M., Balat, H., and Oz, C. (2008). Progress in bioethanol processing. Prog. Energy Combust. Sci. 34 (5), 551–573. doi:10.1016/j.pecs.2007.11.001
Brodeur, G., Yau, E., Badal, K., Collier, J., Ramachandran, K. B., and Ramakrishnan, S. (2011). Chemical and physicochemical pretreatment of lignocellulosic biomass: a review. Enzyme Res. 2011, 1–17. doi:10.4061/2011/787532
Chaowana, P. (2013). Bamboo: an alternative raw material for wood and wood-based composites. J. Mater. Sci. Res. 2, 90–102. doi:10.5539/jmsr.v2n2p90
Deshmukh, M., and Pathan, A. (2024). Bambusa tulda: a potential feedstock for bioethanol and its blending effects on the performance of spark ignition engine. Rene Sust Energ Rev 192, 114270. doi:10.1016/j.rser.2023.114270
Djajadi, D. T., Pihlajaniemi, V., Rahikainen, J., Kruus, K., and Meyer, A. S. (2018). Cellulases adsorb reversibly on biomass lignin. Biotechnol. Bioeng. 115, 2869–2880. doi:10.1002/bit.26820
Ho, M. C., Ong, V. Z., and Wu, T. Y. (2019). Potential use of alkaline hydrogen peroxide in lignocellulosic biomass pretreatment and valorization-A review. Rene Sust. Energ Rev. 112, 75–86. doi:10.1016/j.rser.2019.04.082
Hou, Q., Liu, Z., Shi, Z., Yang, H., Wang, D., and Yang, J. (2024). A deep eutectic solvent pretreatment with self-cleaning lignin droplets function to efficiently improve the enzymatic saccharification and ethanol production of bamboo residues. Indust Crops Prod. 216, 118730. doi:10.1016/j.indcrop.2024.118730
Huang, C., Zhan, Y., Cheng, J., Wang, J., Meng, X., Fang, G., et al. (2022a). The bamboo delignification saturation point in alkaline hydrogen peroxide pretreatment and its association with enzymatic hydrolysis. Bioresour. Technol. 359, 127462. doi:10.1016/j.biortech.2022.127462
Huang, C., Zhao, X., Zheng, Y., Lin, W., Lai, C., Yong, Q., et al. (2022b). Revealing the mechanism of surfactant-promoted enzymatic hydrolysis of dilute acid pretreated bamboo. Bioresour. Technol. 360, 127524. doi:10.1016/j.biortech.2022.127524
Jin, Y., Liu, J., Yang, H., Shi, Z., Zhao, P., and Yang, J. (2021). Improving enzymatic saccharification and ethanol production of bamboo residues with sulfomethylation-aided phosphoric acid pretreatment. Ind. Crop. Prod. 170, 113733. doi:10.1016/j.indcrop.2021.113733
Klinke, H. B., Ahring, B. K., Schmidt, A. S., and Thomsen, A. B. (2002). Characterization of degradation products from alkaline wet oxidation of wheat straw. Bioresour. Technol. 82 (1), 15–26. doi:10.1016/s0960-8524(01)00152-3
Lai, C., Yang, B., Lin, Z., Jia, Y., Huang, C., Li, X., et al. (2019). New strategy to elucidate the positive effects of extractable lignin on enzymatic hydrolysis by quartz crystal microbalance with dissipation. Biotechnol. Biofuels 12, 57. doi:10.1186/s13068-019-1402-2
Laltha, M., Sewsynker-Sukai, Y., and Gueguim Kana, E. B. (2021). Development of microwave-assisted alkaline pretreatment methods for enhanced sugar recovery from bamboo and corn cobs: process optimization, chemical recyclability and kinetics of bioethanol production. Indus Crop Prod. 174, 114166. doi:10.1016/j.indcrop.2021.114166
Li, M., Pattathil, S., Hahn, M. G., and Hodge, D. B. (2014). Identification of features associated with plant cell wall recalcitrance to pretreatment by alkaline hydrogen peroxide in diverse bioenergy feedstocks using glycome profiling. RSC Adv. 4 (33), 17282–17292. doi:10.1039/c4ra00824c
Liang, Y., Duan, W., An, X., Qiao, Y., Tian, Y., and Zhou, H. (2020). Novel betaine-amino acid based natural deep eutectic solvents for enhancing the enzymatic hydrolysis of corncob. Bioresour. Technol. 310, 123389. doi:10.1016/j.biortech.2020.123389
Lin, W., Yang, J., Zheng, Y., Huang, C., and Yong, Q. (2021). Understanding the effects of different residual lignin fractions in acid-pretreated bamboo residues on its enzymatic digestibility. Biotechnol. Biofuels 14, 143. doi:10.1186/s13068-021-01994-y
Meng, F., Li, N., Yang, H., Shi, Z., Zhao, P., and Yang, J. (2022). Investigation of hydrogen peroxide-acetic acid pretreatment to enhance the enzymatic digestibility of bamboo residues. Bioresour. Technol. 344, 126162. doi:10.1016/j.biortech.2021.126162
Miller, G. M. (1959). Use of dinitrosalicylic acid reagent for determination of reducing sugar. Anal. Chem. 31, 426–428. doi:10.1021/ac60147a030
Mittal, A., Katahira, R., Donohoe, B., Black, B. A., Pattathil, S., Stringer, J. M., et al. (2017). Alkaline peroxide delingnification of corn stover. ACS Sustainable Chem. Eng. 5 (7), 6310–6321. doi:10.1021/acssuschemeng.7b01424
Pandiyan, K., Tiwari, R., Rana, S., Arora, A., Singh, S., Saxena, A. K., et al. (2014). Comparative efficiency of different pretreatment methods on enzymatic digestibility of Parthenium sp. World J. Microbiol. Biotechnol. 30 (1), 55–64. doi:10.1007/s11274-013-1422-1
Qing, Q., Zhou, L., Huang, M., Guo, Q., He, Y., Wang, L., et al. (2016). Improving enzymatic saccharification of bamboo shoot shell by alkalic salt pretreatment with H2O2. Bioresour. Technol. 201, 230–236. doi:10.1016/j.biortech.2015.11.059
Rabelo, S. C., Filho, R. M., and Costa, A. C. (2008). A comparison between lime and alkaline hydrogen peroxide pretreatments of sugarcane bagasse for ethanol production. Appl. Biochem. Biotechnol. 144 (1), 87–100. doi:10.1007/s12010-007-8086-y
Sahoo, D., Ummalyma, S. B., Okram, A. K., Sukumaran, R. K., George, E., and Pandey, A. (2017). Potential of Brachiaria mutica (para grass) for bioethanol production from loktak lake. Bioresour. Technol. 242, 133–138. doi:10.1016/j.biortech.2017.03.047
Segal, L., Creely, J. J., Martin-Jr, A. E., and Conrad, C. M. (1959). An empirical method for estimation the degree of crystallinity of native cellulose using the X-ray diffractometer. Text. Res. J. 29, 786–794. doi:10.1177/004051755902901003
Shen, X. J., Wen, J. L., Mei, Q. Q., Chen, X., Sun, D., Yuan, T. Q., et al. (2019). Facile fractionation of lignocelluloses by biomass-derived deep eutectic solvent (DES) pretreatment for cellulose enzymatic hydrolysis and lignin valorization. Green Chem. 21 (2), 275–283. doi:10.1039/c8gc03064b
Sluiter, A., Hames, B., Ruiz, R., Scralata, C., Sluiter, J., and Templeton, D. (2011). Determination of Structural Carbohydrates and Lignin in Biomass. NREL/TP510-42618. Laboratory Analytical Procedure (LAP). National Renewable Energy Laboratory.
Song, Y., Lee, Y. G., Cho, E. J., and Bae, H. J. (2020). Production of xylose, xylulose, xylitol, and bioethanol from waste bamboo using hydrogen peroxicde-acetic acid pretreatment. Fuel 278, 118247. doi:10.1016/j.fuel.2020.118247
Sun, F. F., Wang, L., Hong, J., Ren, J., Du, F., Hu, J., et al. (2015). The impact of glycerol organosolv pretreatment on the chemistry and enzymatic hydrolyzability of wheat straw. Bioresour. Technol. 187, 354–361. doi:10.1016/j.biortech.2015.03.051
Teong, S. P., Li, X., and Zhang, Y. (2019). Hydrogen peroxide as an oxidant in biomass-to-chemical processes of industrial interest. Green Chem. 21, 5753–5780. doi:10.1039/c9gc02445j
Tian, S. Q., Zhao, R. Y., and Chen, Z. C. (2018). Review of the pretreatment and bioconversion of lignocellulosic biomass from wheat straw materials. Renew. Sust. Energ Rev. 91, 483–489. doi:10.1016/j.rser.2018.03.113
Ummalyma, S. B., Karki, V., and Singh, A. (2022). Enzymatic digestibility of pretreated dewaxed bamboo residues as feedstock for bioethanol production. Syst. Microbiol. Biomanufact 2, 716–722. doi:10.1007/s43393-022-00092-w
Ummalyma, S. B., Sahoo, D., Puthiyamadam, A., Adhrsh, V. P., Sukumaran, R. K., Bhaskar, T., et al. (2021). Sono-assisted alkali and dilute acid pretreatment of Phragmites karka (tall reed grass) to enhance enzymatic digestibility for bioethanol conversion. Front. Energy Res. 8. doi:10.3389/fenrg.2020.594452
Xu, J., Dai, L., Gui, Y., Yuan, L., Ma, J., and Zhang, C. (2020). Towards a waste-free biorefinery: a cascade valorization of bamboo for efficient fractionation, enzymatic hydrolysis, and lithium-sulfur cathode. Ind. Crops Prod. 149, 112364. doi:10.1016/j.indcrop.2020.112364
Yang, B., Tao, L., and Wyman, C. E. (2017). Strengths, challenges, and opportunities for hydrothermal pretreatment in lignocellulosic biorefineries. Biofuels Bioprod. Biorefin 12 (1), 125–138. doi:10.1002/bbb.1825
Yao, L., Yang, H. T., Meng, X. Z., and Ragauskas, A. J. (2022). Toward a fundamental understanding of the role of lignin in the biorefinery process. Front. Energy Res. 9, 804088. doi:10.3389/fenrg.2021.804086
Yin, X., Cai, T., Liu, C., Ma, Y., Hu, J., Jiang, J., et al. (2022). A novel solvothermal biorefinery for production of lignocellulosic xylooligosaccharides, fermentable sugars and lignin nano-particles in biphasic system. Carbohydr. Polym. 295, 119901. doi:10.1016/j.carbpol.2022.119901
Zhan, Y., Cheng, J., Liu, X., Huang, C., Wang, J., Han, S., et al. (2022). Assessing the availability of two bamboo species for fermentable sugars by alkaline hydrogen peroxide pretreatment. Bioresour. Technol. 349, 126854. doi:10.1016/j.biortech.2022.126854
Zhang, C., Zhuang, X. S., Wang, Z. J., Matt, F., John, F. S., and Zhu, J. Y. (2013). Xylanase supplementation on enzymatic saccharification of dilute acid pretreated poplars at different severities. Cellulose 20, 1937–1946. doi:10.1007/s10570-013-9934-2
Keywords: bamboo residues, alkaline hydrogen peroxide, biomass digestibility, pretreatment, biofuels
Citation: Ummalyma SB, Herojit N and Sukumaran RK (2024) Alkaline hydrogen peroxide pretreatment of bamboo residues and its influence on physiochemical properties and enzymatic digestibility for bioethanol production. Front. Energy Res. 12:1444813. doi: 10.3389/fenrg.2024.1444813
Received: 06 June 2024; Accepted: 15 August 2024;
Published: 27 August 2024.
Edited by:
Jürgen Krahl, Technische Hochschule Ostwestfalen-Lippe, GermanyReviewed by:
Mudasir A. Dar, Jiangsu University, ChinaLing-Ping Xiao, Dalian Polytechnic University, China
Copyright © 2024 Ummalyma, Herojit and Sukumaran. This is an open-access article distributed under the terms of the Creative Commons Attribution License (CC BY). The use, distribution or reproduction in other forums is permitted, provided the original author(s) and the copyright owner(s) are credited and that the original publication in this journal is cited, in accordance with accepted academic practice. No use, distribution or reproduction is permitted which does not comply with these terms.
*Correspondence: Sabeela Beevi Ummalyma, c2FiZWVsYS4yNUBnbWFpbC5jb20=