- 1Climate, Health, and Energy Equity Lab (CHEEL Lab), Department of Earth and Environmental Sciences, Vanderbilt University, Nashville, TN, United States
- 2Climate, Health, and Energy Equity Lab (CHEEL Lab), Program in Climate and Environmental Studies, Vanderbilt University, Nashville, TN, United States
- 3Vanderbilt University, School of Nursing, Nashville, TN, United States
- 4Vanderbilt University, Nashville, TN, United States
Energy-inefficient buildings are a major driver of climate change. The aging, energy inefficient housing occupied by low-income households in the US and other high-income countries is a driver of notable environmental and health disparities as well. Public policies for alleviating the financial and health burdens of energy inefficient housing have existed for decades, but fallen short of reaching most households in need. This paper proposes a promising new approach to filling gaps left by public programs — one that relies on mobilizing the tools of private governance, carbon offsetting and the voluntary carbon market (VCM) to finance energy upgrades for low-income households. We develop a new and readily applicable methodology for calculating energy and carbon savings from efficiency upgrades based on readily accessible publicly available data. Tailored to the needs of estimating energy and carbon savings from efficiency upgrades to low-income units, rentals in particular, this methodology can be fruitfully deployed in monetizing the carbon savings from efficiency driven reductions in household energy use. Specifically, we propose packaging the emission reductions generated through energy savings as carbon offsets, then selling these offsets on the voluntary carbon market to generate financing for energy upgrades to low-income homes not served by public energy efficiency programs. Given the multiple economic and health co-benefits from low-income energy upgrades, we expect that carbon offsets generated through such upgrades will be attractive to many corporate and institutional offset buyers, particularly those who seek to fulfill climate commitments while also advancing economic and human development in their host communities.
1 Introduction
As the effects of climate change intensify, marginalized groups face disproportionate exposure to climate and environmental hazards like extreme heat and air pollution (Alizadeh et al., 2022; Khatana et al., 2022). In the US and similarly high-income countries, aging, energy-inefficient housing is a major contributor to such disparities in climate and health vulnerability (Brown et al., 2020; Goldstein et al., 2022; Kolokotsa and Santamouris, 2015). On the one hand, energy inefficient housing accelerates climate forcing through excess emissions from wasteful energy use. On the other hand, it burdens low-income households with high energy costs, leaving them struggling to maintain homes at healthy, seasonally appropriate temperatures, and forcing them to cope with the physical and psychological toll of choosing between buying food and medicine or paying energy bills in order to avoid electric and gas shutoffs (Drehobl et al., 2020; Hernández and Siegel, 2019; Reames et al., 2021).
Energy efficiency retrofits to low-income housing can reduce greenhouse gas (GHG) emissions while improving health outcomes and climate resilience for members of marginalized groups. As such, prioritizing energy efficiency retrofits that benefit low-income households should be a key focus of climate and energy policies. Despite a number of long-standing public programs targeted at improving energy efficiency and affordability for low-income households, however, high levels of energy inefficiency and high energy cost burdens remain the norm for these households in the US and other high-income countries (Antunes et al., 2023; Kontokosta et al., 2020).
Some tools of private climate governance are well-positioned to fill gaps in public policies and financing of low-income energy efficiency upgrades. Carbon offsetting and the voluntary carbon market (VCM) offer one promising and novel approach to financing energy efficiency upgrades in low-income housing, both rental and owner-occupied. Specifically, GHG reductions generated through efficiency-driven reductions in energy use have distinct value to carbon offset buyers on the voluntary carbon market. The estimated reductions in emissions from household energy use can be packaged as carbon offsets and sold to some of the many corporate and institutional buyers who are currently striving to reduce carbon footprints and fulfill corporate climate commitments. Proceeds from the sale of offsets generated over the 25-year lifespan of an energy efficiency upgrade to a low-income housing unit can constitute a new and promising mechanism for financing the up-front cost of such efficiency upgrades (Maine State Housing Authority, 2012). This type of carbon offset generated through low-income efficiency upgrades — an offset that comes with positive economic and health co-benefits (or spillovers) for members of marginalized groups — will be particularly attractive for corporate and institutional actors who seek to decarbonize challenging portions of their operations and value chains, while contributing to economic and human development in the regions where they operate (Lou et al., 2023).
A key practical barrier in mobilizing carbon offsets and the VCM as a finance mechanism for low-income energy efficiency, however, is the technical challenge of quantifying upgrade-enabled reductions in household energy use and carbon emissions (Maine State Housing Authority, 2012). This technical challenge looms especially large in the context of low-income rental housing, where continuous measurements of pre- and post-upgrade energy use and savings are impeded by cost and potentially high tenant turnover (Desmond et al., 2015).
The research presented in this paper introduces a new methodology for quantifying efficiency-driven reduction in household energy use, utility bills, and carbon emissions, and shows how to determine the carbon offset value of such reductions. While designed to address the unique data challenges of measuring energy and climate benefits of efficiency retrofits to low-income rental housing, this new methodology is applicable to other low-income residential contexts as well.
Over 60% of Americans at the bottom quartile of the income distribution are renters, while only 10.5% of Americans in the top quartile of the income distribution rent their homes (DeSilver, 2021). An estimated 32% of renters are low-income households earning less than $30, 000/year in household income, while the median renter household makes a mere $ 47,000/year. Added to the housing cost burdens faced by low-income renters (Mateyka and Yoo, 2023), high energy cost burdens amplify the financial precarity of renter households. The energy cost burdens of these low-income, financially precarious renter households, along with the negative health implications of high energy costs, are a leading motivator for this study.
The goals of the paper are twofold. First, we aim to introduce our new methodology for calculating efficiency-generated carbon offsets and illustrate its ready applicability to even the most data-challenging low-income rental housing contexts in the US. Second, and equally important, we aim to demonstrate that mobilizing the VCM and corporate spending on carbon offsets to finance energy efficiency retrofits to low-income rental housing is a meaningful climate intervention with potentially significant social, health, and energy equity co-benefits in the US and beyond (See Figure 1).
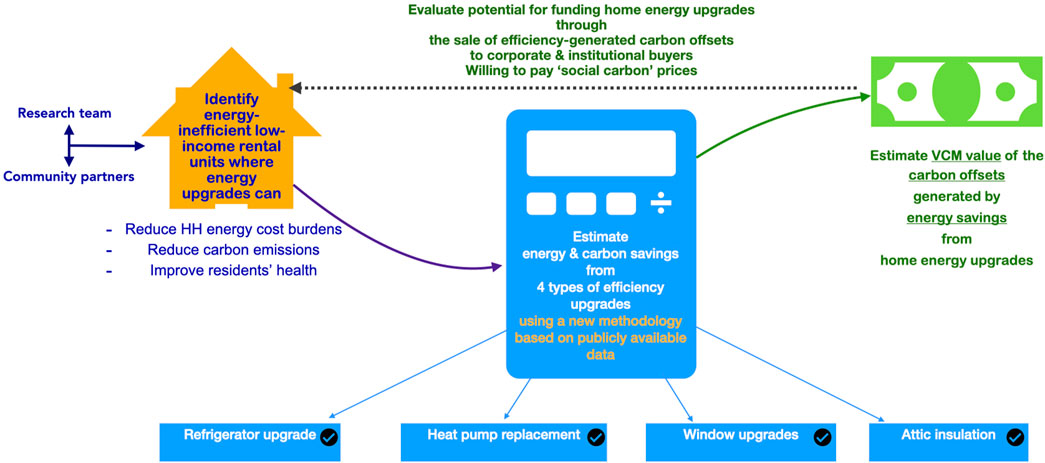
Figure 1. Conceptual and methodological approach to mobilizing carbon offsets and the VCM to advance energy efficiency in low-income rental housing.
In sum, we focus on the substance and development of a new methodology for calculating residential-energy-efficiency-generated carbon offsets, while drawing out the anticipated climate, health equity, and social equity benefits of scaling up its practical implementation in the US and beyond.
2 Background
The health consequences of energy inefficient housing are discussed below, as are the health benefits of home energy upgrades that reduce the energy cost burdens (ECBs) of low-income households. This is followed by a breakdown of shortfalls in public programs for assisting low-income households with energy efficiency upgrades, and introduction of carbon offsets as one promising tool for bringing energy efficiency upgrades to homes and households unreached by public policies and funds.
2.1 Energy-inefficient housing worsens climate change and widens economic and health disparities
Energy inefficiency is a significant concern in the U.S. The residential sector alone consumes about 21% of the nation’s primary energy, largely used for heating and cooling. It also accounts for roughly 21% of annual greenhouse gas emissions in the US (Bradshaw et al., 2016). Enhancing energy efficiency in the residential sector presents a substantial, cost-effective, and immediate method to decrease energy consumption and related emissions (Pierce et al., 2023).
Efficiency upgrades are particularly challenging in low-income housing, however, exposing its residents to disproportionately high energy costs and their associated health risks. A major component of housing affordability, utility costs pose financial challenges for low-income households (Kontokosta et al., 2020). Many low-income residents are renters responsible for paying their utility bills, but have little control over the energy efficiency of the residence, since landlords lack motivation to invest in energy upgrades (Pierce et al., 2023; Vandenbergh, 2018). Lacking financial and other ability to make efficiency improvements, low-income households in the US face some of the highest energy cost burdens (ECBs), meaning they spend a higher-than-average percentage of their annual gross income on utilities such as electric, gas, and other heating fuels (Bradshaw et al., 2016; Drehobl and Ross, 2016). A 2020 study, for example, puts the median energy cost burden for low-income households at 8.1% of annual income, more than double the national median of 3.5% (Drehobl et al., 2020).
Economic stress from high ECBs in turn contributes to a range of physical and mental health problems. Fear and uncertainty about maintaining energy access, obtaining energy assistance, and covering energy costs are documented sources of chronic stress, anxiety, and depression in members of energy-cost-burdened households. High ECBs can further tax mental health through increasing social isolation and lowering the academic performance of students, while parents fear loss of custodial rights due to their perceived inability to provide a healthy home environment (Drehobl et al., 2020; Drehobl and Ross, 2016).
Coping with high ECBs also means foregoing or reducing home heating and cooling – a coping strategy practiced by an estimated 13 million US households (Reames et al., 2021) in a context where living in an unseasonably cold or warm home environment heightens the risk of developing respiratory problems, heart disease, arthritis, and rheumatism, among other conditions. Managing existing respiratory, cardiovascular, and inflammatory disease is also very challenging when living in an energy inefficient or energy insecure home (Drehobl et al., 2020; Hernández and Siegel, 2019). Recent research has broadly confirmed energy poverty and energy burdens as a key social determinant of health, showing that in a majority of US counties, each unit increase in the energy burden of low- and middle-income households is associated with measurable uptick in premature deaths and self-reports of poor health. Each unit increase in low-income energy burden is also associated with a measurable (more than a 5-year) decline in average county-level life expectancy (Reames et al., 2021).
Further exacerbating health disparities, the physical health problems aggravated by high ECBs are more common in marginalized groups most exposed to energy inefficient housing. Asthma is more prevalent among poor, Puerto-Rican and non-Hispanic Black Americans (Celedón et al., 2014), for example, and asthma-related hospitalizations and deaths are more than 3 times higher for African Americans than non-Hispanic whites (AAFA, 2020; CDC, 2021; HHS, 2022; Zanobetti et al., 2022).
Unlike housing and transportation costs, high energy cost burdens are relatively easier to address through energy efficiency measures (Kontokosta et al., 2020). In addition to reducing both ECBs and GHG emissions, weatherization of low-income homes produces direct and indirect health benefits (Evens et al., 2017; Tonn et al., 2024). Significant reductions in symptoms of asthma and COPD, as well as reductions in thermal stress, arthritis symptoms, poor sleep quality, days of poor mental health have all been reported by low-income households following home weatherization. Indirectly, reduced ECBs have helped increase access to nutritious foods and prescribed medications, reduced food spoilage, and reduced need to choose between health promoting behaviors and utility usage (Tonn et al., 2021). Studies of low-income efficiency retrofits in both the US (Illinois and Indiana) and New Zealand find a range of similar energy-efficiency-related improvements to health and quality-of-life outcomes (Francisco et al., 2017; Howden-Chapman et al., 2007).
2.2 Public programs for energy efficiency reach only a fraction of US households in need
Energy poverty and insecurity are insufficiently prioritized as a federal policy problem, as evidenced by the lack of dedicated federal strategies and statutes for mitigating energy insecurity, which affects more than 20 million American households (more than 17% of all households) (Bednar and Reames, 2020). However, the federal government has recognized the narrower issue of energy affordability, largely through two main programs. The smaller of the two, the Weatherization Assistance Program (WAP), was established in 1976 in response to the 1973 oil crisis. It provides federal grants to US states and territories to help them improve energy affordability through funding weatherization of low-income homes (Office of State and Community Energy Programs, 2024). States receiving these grants partner with private sector contractors to provide no-cost retrofits for qualifying households earning below 200% of the federal poverty level. Despite being the largest federal weatherization program, WAP supports retrofits for only a fraction of eligible homes: in 2022, for example, it supported 69,000 retrofits, less than half-percent of all WAP-eligible households (Drehobl, 2020; NASCSP, 2022; US Census Bureau, 2022). Insufficient federal spending limits WAP’s ability to reach a majority of energy cost burdened low-income households, as do shortages in state-level staff and contractors (NASCSP, 2022). WAP’s current administration is leaving behind tens of millions of low-income households, despite the fact that independent program evaluations have clearly demonstrated the multiple energy and non-energy (e.g., health, job creation) benefits of WAP implementation (NASCSP, 2018; Bednar and Reames, 2020; National Academies of Sciences, Engineering, and Medicine, 2023, p. 287).
Housed in the Department of Health and Human Services, the Low-Income Home Energy Assistance Program (LIHEAP) is the second federal policy with some potential to alleviate the high ECBs of low-income families. It offers financial aid towards covering the cost of home energy bills, energy-related repairs, and weatherization (Office of Community Services, 2023). LIHEAP functions best as a source of emergency aid, however, rather than an instrument of systemic and structural change, since its design and operation are based on an empirically incorrect assumption that energy bill struggles are a temporary rather than chronic problem for many American households (Tonn et al., 2003; Bednar and Reames, 2020). Although it is funded at an average level of 3 billion USD annually — more than 7 times the funding level of WAP — LIHEAP has not been able to reach all energy insecure households, nor has it made significant headway on providing lasting relief from the pervasive problem of energy insecurity and poverty (Murray and Mills, 2014; Bednar and Reames, 2020).
Federal failures to recognize energy poverty as a distinct and salient policy problem may be significantly accountable for WAP and LIHEAP’s shortfalls. Further exacerbating these shortfalls is the fact that for most of their existence, the programs’ success has been measured in terms of policy outputs (such as federal resource outlays and number of households assisted) instead of policy outcomes like absolute and percentage reductions in the number and share of households suffering from energy poverty (Bednar and Reames, 2020). The ultimate indicator of WAP and LIHEAP’s massive shortfalls is the fact that 5 decades after the introduction of these federal programs to improve low-income energy affordability, one in three US households (37 million households in total) continue to experience energy poverty (Bednar and Reames, 2020).
A number of smaller federal programs complement WAP and LIHEAP in supporting energy efficiency and affordability for low-income households, but none of them are individually or collectively positioned to meet the energy efficiency needs of the millions of energy-inefficient, energy cost burdened, low-income US households (Amann et al., 2021).
Partly in response to such federal shortfalls, some US states have also acted to address energy affordability. The Massachusetts Department of Energy, for example, collaborates with state utility companies to offer Mass Save HEAT Loans, zero-interest loans for efficiency retrofits. The largest ratepayer-funded program of its kind in the country, this loan program reached 13,433 households in 2019 (Amann et al., 2021, p. 46), but it still leaves out the majority of low-income households, for whom such loans remain out of reach. Similar problems affect the energy affordability policies of another state leader, California. Underscoring the value of the private governance and carbon offset financing proposition presented in this paper, California’s experience has been discussed as illustrative of the need to mobilize private investment towards achieving the state’s goal for decarbonization by 2045 (Mast et al., 2020).
In order to ease the high energy burdens for low-income households, the state of Tennessee, where our methodology-development pilot takes place, provided a $5.5 million state supplement to WAP and (LIHEAP) funds in 2015 (American Council for an Energy Efficient Economy, 2017), while the state’s generation utility, Tennessee Valley Authority (TVA), spent another $19 million on low-income energy efficiency upgrades in 2016 (American Council for an Energy Efficient Economy, 2017). Despite these public policy efforts, low-income households in Tennessee continue to face high ECBs that average 9.0% year after federal and state assistance (Office of Community Services, 2023), well above the 6% threshold above which households are considered “energy poor” (Brown et al., 2020).
2.3 Carbon offsets as a tool for expanding energy efficiency upgrades in low-income housing
The term carbon offset refers to a reduction (or removal) of GHG emissions undertaken to compensate for emissions occurring elsewhere. Carbon offsetting activities (like forest restoration or renewable energy development) produce “offset credits” or “carbon credits” — the quantified and tradeable units of GHG reductions that governments, companies, or other entities can purchase or exchange with each other. Each credit typically refers to one metric ton of carbon, or a carbon-equivalent amount of other GHG reductions (Broekhoff et al., 2019).
By enabling companies and governments to invest in carbon reducing activities that compensate for their own carbon emissions, offsets are a form of carbon accounting. Carbon offsetting activities and carbon offset purchases often stem from corporate and institutional commitments to “carbon neutrality” — commitments attained when the GHG emissions generated by an organization’s activities are no greater than the emissions reduced or removed through carbon offsets financed or purchased by the organization. Offset purchases for advancing voluntary decarbonization commitments take place in the Voluntary Carbon Market (VCM) (Battocletti et al., 2023).
As opposed to compliance markets, which are created by government climate regulations such as cap-and-trade schemes, VCM comprises all carbon trading undertaken by companies and organizations for reasons other than regulatory compliance with government carbon mandates. VCM trading is currently driven by major corporate actors, like the 482 large companies that have made public commitments to carbon neutrality or climate action, and which collectively account for $16 trillion in annual revenue (Kreibich and Hermwille, 2021). The quality of carbon offsets and offset credits sold on the VCM is regulated by NGO-run carbon registries, such as Verra, the Gold Standard, and the American Carbon Registry (Broekhoff et al., 2019).
The carbon offset credits purchased on the VCM by corporate and institutional buyers are generated through offsetting projects that vary in type, size, scope, format, quality, and legitimacy (Miltenberger et al., 2021). Project types include carbon forestry (both reforestation and forest restoration efforts), renewable energy development, as well as GHG-reducing economic and human development interventions like the distribution of clean burning cookstoves and water filters to replace open-hearth cooking and boiling of drinking water respectively (Anderson and Zerriffi, 2012; Gold Standard, 2017; Simon et al., 2012). Many existing offset projects are located in the Global South, but intended to compensate for the emissions of companies and institutions located in the Global North. This physical and jurisdictional distribution comes with a potential for positive spillovers (economic and social) in some of the world’s more marginalized communities (Favretto et al., 2020; Kremen and Merenlender, 2018; Simon et al., 2012; Wittman and Caron, 2009). But it also comes with challenges for quality control (Blake, 2023; Lovell, 2010) and a risk of negative social and environmental spillovers that are all but invisible to the corporate and institutional buyers of offset credits (Leach and Scoones, 2015; Lyons and Westoby, 2014).
Carbon offset quality can be indexed by a number of characteristics, including additionality, permanence, “double-counting,” and potential for co-benefits (Broekhoff et al., 2019). Additionality is a particularly important attribute, intended to guarantee that carbon reductions stemming from a particular offset project could not have occurred—would not have occurred — without the offset buyer’s investment in that project (Haya et al., 2020; Mason and Plantinga, 2013). Additionality, in other words, is considered a key attribute of credible offsets, because it helps ensure that offset buyers are not paying to achieve carbon reductions that would happen regardless of the buyer’s investment. Since it is an attribute that hinges on proving a counterfactual, additionality is particularly challenging to prove (Oxford University, 2020). Permanence refers to the longevity of avoided emissions, ensuring that carbon reduction or sequestration is not reversed by future actions or events (for example, a carbon plantation forest burning in a forest fire) (Schneider et al., 2019). The requirement for no-double-counting simply stipulates that credible offset credits are transacted only once (rather than having the same GHG reductions sold to — and claimed towards — the carbon accounting of more than one company or organization) (López-Vallejo, 2022).
The quality, legitimacy, and climate benefits of specific carbon offset projects have often come into question, with concerns proliferating along with the growth in VCM transactions (Forest Trends, 2023; Gifford, 2020). A recent investigation, for example, found the vast majority of rainforest offsets certified by leading VCM registry Verra to be “phantom credits,” i.e., credits not grounded in actual and additional carbon reductions (Greenfield, 2023). These offsets are nonetheless still purchased by some of the world’s biggest companies and counted towards these companies’ progress on decarbonization.
Even offset projects that generate actual carbon reductions can come with unintended and negative economic and social consequences for the often-marginalized communities hosting such projects (Dunne and Quiroz, 2023). Carbon forestry projects operated by western companies and located in Global South countries like India and Uganda, for example, have disrupted land access and the livelihoods of farmers and subsistence users of public land repurposed to carbon forestry (Aggarwal and Brockington, 2020; Lyons et al., 2014).
Despite such notable shortfalls, however, carbon offsetting and the VCM still hold significant potential for helping mitigate climate change, sometimes in ways that generate positive economic, health, and social spillovers for marginalized groups and communities the world over (Miltenberger et al., 2021). Intentionally designed carbon offset projects, for example, have the potential to generate tangible economic, health and environmental co-benefits at the same time as they generate GHG reductions (Bumpus, 2011). The positive spillovers of legitimate offset projects located in the Global North, in particular, have been little explored, yet such projects present a key opportunity for corporate entities to invest in the social and physical health of their proximal communities while offsetting their own emissions and advancing their carbon neutrality aspirations (Forthcoming by several of the authors).
2.3.1 US precedents on carbon offsets with social co-benefits
Clearloop, a private company, and the Maine Department of Housing, a state agency, offer two recent illustrations on mobilizing carbon offsetting and the VCM to finance renewable energy and low-income energy efficiency in the US. Based in the U.S. Southeast, a region that ranks as the sixth-largest global carbon emitter and lacks adequate renewable energy mandates (Vandenbergh and Gilligan, 2017), Clearloop generates carbon offsets by building utility-scale solar in dirty parts of the US electric grid (i.e., grid regions with high GHG emission factors) (Zapata et al., 2020). It finances such solar development through up-front sale of the carbon offsets generated during the lifetime operation of each solar farm. Building solar in dirty parts of the US grid, in turn, maximizes the carbon emissions avoided by interconnecting new solar (Kvale, 2021). In addition to GHG reductions, Clearloop’s carbon offsetting work can generate health co-benefits for host communities, by displacing fossil fueled generation capacity and thus reducing the mercury, NOx, and particulate emissions that commonly accompany GHGs on their way out of power plant smokestacks (Tzankova, 2021).
The Maine State Housing Authority (MaineHousing), an agency overseeing WAP implementation for the state of Maine, has piloted the idea of financing residential energy efficiency upgrades with the sale of carbon offsets generated through such upgrades. Launched in response to low-income weatherization needs exceeding the coverage and resources of public programs, MaineHousing’s program took 5 years to develop, and funded the weatherization of 7,000 low-income units through the sale of efficiency-generated carbon offsets to automaker Chevrolet (Maine State Housing Authority, 2012). Chevrolet invested $750,000 in the MaineHousing’s pilot (Quimby, 2011; Kokai, 2011) — an up-front spend that is significant and impactful, but also beyond the financial reach of many companies with carbon reduction aspirations and commitments. MaineHousing distributed Chevrolet’s offset purchase towards funding a small number of efficiency upgrades in a number of Maine communities.
Existing initiatives for US-based carbon offsetting with social co-benefits, in other words, leave room for improvement on both the offsetting and co-benefit fronts — improvements enabled by our approach to implementing offset-financed efficiency retrofits for low-income housing.
3 Methods
To advance the practice of offset-financed efficiency retrofits in affordable rental housing, we develop a new methodology for estimating retrofit-generated energy savings and CO2 reductions. This methodology is readily applicable in a context of resource and data constraints typical for low-income rental housing. It relies on existing data from a range of easily accessible data sources, and it is based on a series of calculations designed to convert existing data into reliable estimates on energy savings and carbon reductions from rental housing energy efficiency upgrades.
3.1 Data sources
Household energy savings are generated through two categories of retrofits — structural improvements to the building envelope and appliance upgrades. We present accessible and time-efficient approaches for estimating energy and carbon savings through both, covering window and insulation upgrades on the building side, and refrigerator and heat pump upgrades on the appliance side.
We start with physical inspection and measurements of a rental unit’s windows, attic insulation, refrigerator, and HVAC system. Essential data to be collected though this inspection, which should take 3 h for a 2-bedroom stand-alone rental unit, includes the following:
• Brand and model of a unit’s windows + number, size, and type of windows
• Attic area requiring insulation
• Brand and model of a unit’s refrigerator
• Brand and model of a unit’s heating/cooling system
The 2-bedroom unit serving as the physical site for our methodology pilot was chosen as representative of one key type of housing available to low-income families in Nashville and other US cities. In the US over-all, the majority of rental units (40%) are 2-bedroom rental units - the size of the unit in our study, and just under 30% of American renters live in stand-alone detached houses like the one used in this study (Kilroy, 2024).
Our physical measurements were done on a housing unit owned and administered by our community partner - a religious institution that has a housing ministry and rents the several housing units it owns at below-market rates to low-income families who are part of the neighborhood fabric but in danger of getting displaced by growing rents and the loss of rental housing to a development boom replacing older, smaller homes with several larger, high-end homes built on the same lot.
To calculate the energy and carbon savings from each type of efficiency retrofit (windows, insulation, refrigerator and HVAC system, respectively), we also mobilize publicly available data and tools from 5 existing sources:
1. The US EPA ENERGY STAR Product Finder: Provides data on the energy use of existing unit appliances and the most energy-efficient alternatives, listing annual energy use in kWh/yr for different makes and models of refrigerators and HVAC systems, while also offering links to the websites of local appliance retailers (searchable by zip code) who stock top-efficiency-rated appliances. This data is used in calculating energy and carbon savings from appliance and window upgrades, as well as the cost of such upgrades.
2. The US EPA eGRID database: maintained by the Clean Air Markets Division of the United States Environmental Protection Agency (EPA), the Emissions and Generation Resource Integrated Database (eGRID) contains data on environmental characteristics of electric power grids in the United States. Commonly used in CO2 calculations for greenhouse gas inventories and avoided emissions estimates, eGRID gives us data on emissions from household electricity use, based on the grid region where that use occurs (US EPA, 2023). To calculate CO2 emissions from residential electricity use, we rely on two key pieces of information: the electric grid region in which a housing unit is located, and the CO2 emissions rate for that grid region.
3. Historic residential rates tables: utility-provided records of historic residential utility rates are the tool we use to convert household energy use into estimated utility bill payments—and estimate utility savings resulting from efficiency-enabled reductions in energy use. Below, we use historic rate tables provided by NES (Nashville Electric Service, the utility serving the model units used as the basis for developing this methodology)
4. The US DOE Worksheet on Window Energy Savings (see below)
5. The CIMA Insulation Savings Calculator (see below)
As the physical site of our study and methodology pilot, Nashville - a major US city - is representative of the high energy burdens borne by low-income households in the US. Across the United States, low-income households spend between 7.1% and 8.1% of income on home energy bills, in a context where the median US household spends around 3.1%, and spending of 6% or more is considered as high energy burden by the American Council on Energy Efficient Economy (ACEEE), while spending of 8% or more is characterized as severe energy burden (Drehobl et al., 2020).
In the US state of Tennessee, where Nashville is located, households living on incomes less than 200% of the federal poverty line spend 6%–8% of their income on home energy (NES and TVA, 2019). Half of low-income Nashville households face energy burdens greater than 6.4%, while a quarter have energy burdens of 10.9% or more, while the city median energy spending is equal to the national average of 3.1%, and similar to the median energy cost burden for many other Southeastern, Midwestern, Northeastern, Southwestern, and Western cities (most of which have median energy costs between 3% and 4%) (Drehobl and Ross, 2016). An estimated 13.4% of Tennesseans and 14% of Nashvillians live below the federal poverty line (Sycamore Institute, 2024) while an estimated 31.5% of Tennesseans live on incomes less than twice the federal poverty line (HHS, 2023; KFF, 2022). These are single-person households making less than $14,580/year, two-person households making less than $19,720/year, and four-person households making less than $30,000/year, yet paying 6%–10% of their limited annual income on utility bills, in a Nashville context where a majority of low-income households are housing cost burdened (National Low Income Housing Coalition, 2024).
3.2 Calculating carbon reductions from energy efficiency upgrades
3.2.1 Carbon reductions from fridge upgrade
Our methodology entails 5 straightforward steps to estimating the energy savings, carbon reductions, and utility bill savings generated through upgrading a rental unit’s refrigerator (Equations 1–6; Table 1). We start by searching the EPA ENERGY STAR Product Finder for estimates on the annual energy use of the existing refrigerator and its top-efficiency rated upgrade, respectively, both listed in kWh/year. Top-rated upgrade is identified using the ENERGY STAR “Most Efficient” filter. To facilitate calculations of carbon emissions from the annual use of each refrigerator, we then convert the appliance’s annual energy use from kWh/year (the metric provided in ENERGY STAR) into mWh/year:
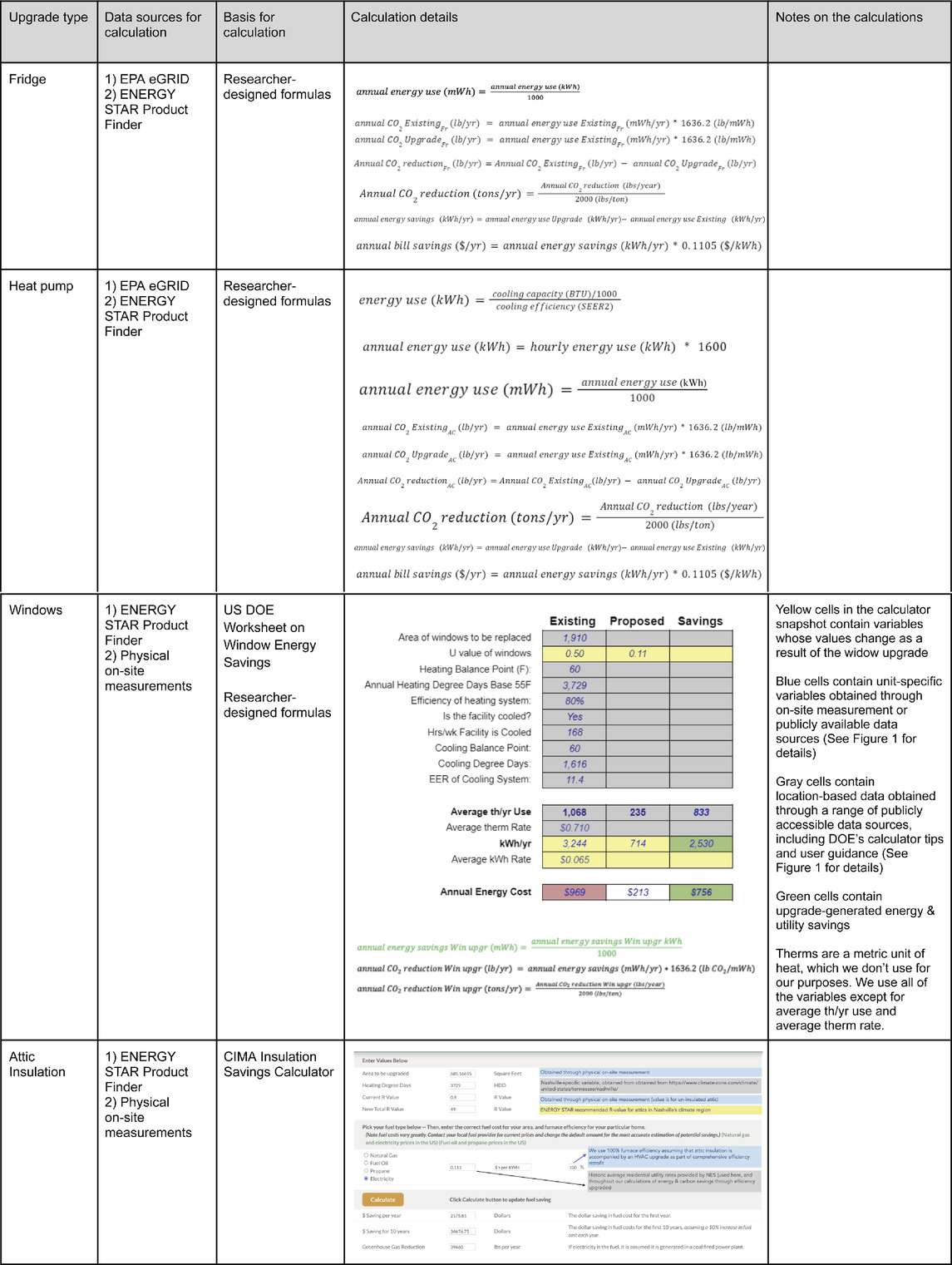
Table 1. Methodology for calculating retrofit-generated energy and carbon savings based on publicly available data and physical measurements in low-income housing units.
Third, we calculate the CO2 emitted in a year of using the existing refrigerator, as well as the CO2 emitted in a year of using an energy efficient upgrade, using Equations 2.1–2.2. We do this by combining our knowledge on the annual amount of electricity used by each refrigerator (in mWh/year) with emissions rate information from eGRID, which gives us the amount of CO2 emitted by the generation of 1 mWh of electricity. The 1,636.2 lb/mWh metric is the regionally specific emissions rate that eGRID lists for the SRTV electric grid subregion, where affordable rentals serving as the empirical basis for this pilot study get their electricity.
Fourth, we determine the carbon reductions expected from a fridge upgrade through simple subtraction, followed by conversion (from lbs/year to tons/year) to make our estimates compatible with standard units for carbon accounting and offset trading:
Finally, annual energy and utility bill savings from a fridge upgrade are calculated by multiplying the amount of energy saved from a fridge upgrade (in kWh/year) by the average monthly energy rate charged by the relevant local utility (in our case, NES). The average monthly energy rate used in our calculations - a rate of $0.1105/kWh - is estimated based on historic monthly rates data provided by NES:
3.2.2 Carbon reductions from HVAC upgrade
Carbon reductions from an upgrade to a rental unit’s HVAC system are calculated through 4 sequential steps, some of them similar to those required for estimating emission reductions from a fridge upgrade. Our calculations are specifically focused on the carbon reductions associated with the electricity-powered cooling function of the system, with the usually natural-gas-powered heating unit remaining outside the scope of our calculations. Our methodology thus offers a conservative estimate (an under-estimate) on the amount of carbon reductions which are practically achievable through energy efficiency upgrades to low-income housing.
First, EPA ENERGY STAR Product Finder is used to determine the cooling capacity (in BTUs) and cooling efficiency (recorded as SEER2 rating) for the existing HVAC (identified by make and model) and a top-rated upgrade (again, identified using the ‘Most Efficient’ ENERGY STAR filter). Second, energy use, in kWh, for each of the two HVACs (existing and upgrade) is determined using Equation 7 (Amskaty, 2024). Annual energy use, in mWh, is then estimated through multiplying the heat pump’s energy consumption (provided in kWh) by 1,600 - the annual number of hours that US households are estimated to use an air-conditioner (Inspire Clean Energy, 2024) (see Equation 8), and dividing the product by 1,000 (Equation 8):
Third, carbon reductions from heat pump upgrade, in tons/year are determined through the same series of calculations used to determine the carbon reduction value of a refrigerator upgrade, but using Equation 4a :
The 1,636.2 lb/mWh metric is the regionally specific emissions rate that eGRID lists for the SRTV electric grid subregion, where affordable rentals serving as the empirical basis for this pilot study get their electricity.
3.2.3 Utility and carbon savings from window upgrades
In contrast to the multistep process for estimating energy and carbon savings from appliance upgrades, CO2 reductions from window upgrades are estimated using a simple Energy Savings Windows Worksheet provided by US DOE (Figure 2). Its calculations on annual energy savings (kWh/yr) and cost savings ($) from residential window upgrades are based on data for the number, dimensions, and overall area of existing windows (obtained through physical on-site measurements), as well as data on the energy performance of top-rated window upgrades (identified through the ENERGY STAR Product Finder, using its ‘Most Efficient’ filter and a filter for Nashville’s region (South-Central Zone)). Compared to existing windows, top-rated ENERGY STAR windows have a lower U-factor—i.e., a lower rate of heat flow transmission, meaning that less of the home’s coolness or heat “escapes” through the window, making the home more energy-efficient (Energy Saver, 2024). The Worksheet calculator produces clear estimates on the annual energy use and utility bill savings generated by window upgrades (Figure 2).
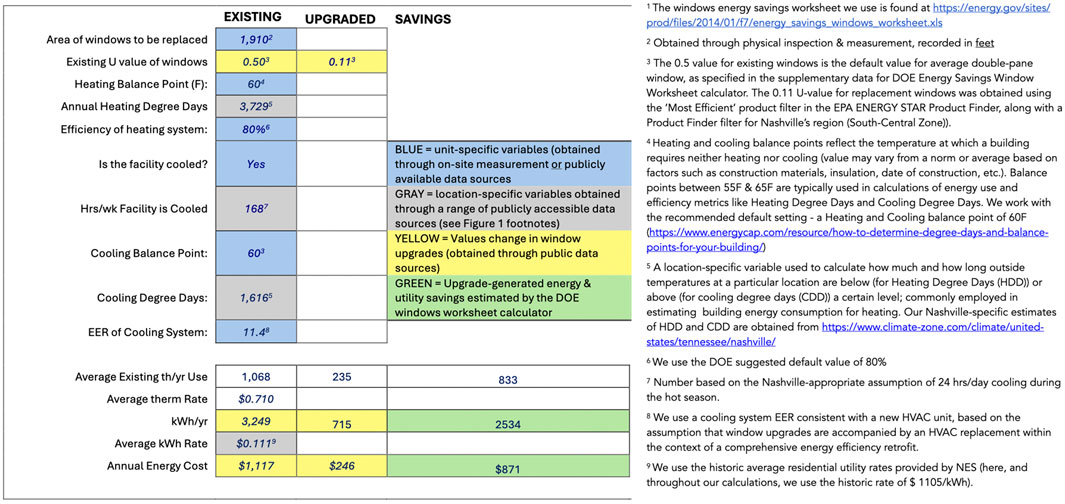
Figure 2. U.S. DOE Windows Worksheet Calculator - using location-specific, unit-specific, and upgrade-specific inputs to estimate annual energy and cost savings from window upgrades.
To calculate carbon reductions stemming from the energy savings delivered by a window upgrade, energy savings are, again, converted to mWh/year, using Equation 9. Carbon reductions are then calculated using adapted versions of Equations 2.1–4:
3.2.4 Utility and carbon savings from upgrading attic insulation
Calculating carbon reductions from improvements to attic insulation is also achievable through an existing and easy to use calculator. Created by the Cellulose Insulation Manufacturers Association (CIMA), the Insulation Savings Calculator (CIMA, 2024) determines annual utility bill savings, while also directly calculating GHG reductions associated with energy savings from upgrades to attic insulation (Figure 3).
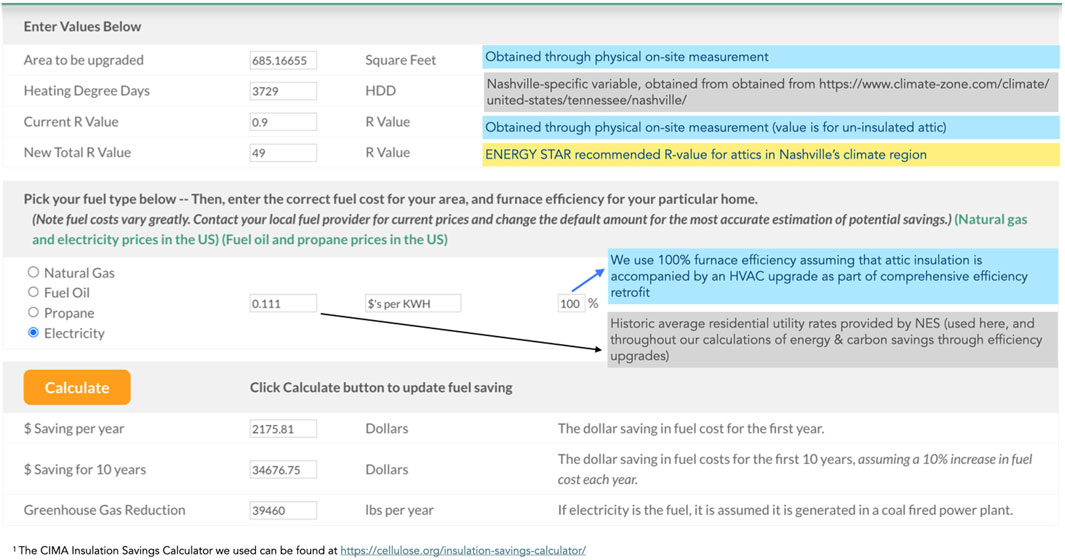
Figure 3. CIMA Insulation Savings Calculator - estimating energy cost and GHG savings from attic insulation upgrades to a model unit.
Using the calculator requires 5 types of data. First, it calls for the square footage of insulated area in need of upgrade — data obtained through physical measurements. It also calls for location-specific data on heating degree days (measures of how warm/cold a location is), necessary for determining the importance of insulation in regulating a home’s temperature, and available through a calculator-provided link to a US EIA fact sheet. Third, the calculator calls for inputting R-values — a measure of insulation’s ability to trap heat — for both existing and upgraded attic insulation. R-value information for the existing insulation comes from physical inspection, while the desired R-value for upgrade insulation is selected after consulting EPA ENERGY STAR recommendations and a range of other professional resources, and balancing cost and energy efficiency when choosing the regionally-appropriate R-values for the upgraded attic insulation (Figure 3). Fourth, the CIMA Insulation Savings calculator calls for data on the unit’s fuel type and fuel cost — in our case, the NES utility rate. Finally, it calls for data on furnace efficiency, which we estimate as 100% based on an assumption that attic insulation is accompanied by a heat pump replacement within the context of a comprehensive energy efficiency retrofit since a new, upgraded heat pump starts out with efficiency of 100%. Based on these inputs, the Insulation Savings Calculator computes energy savings and GHG emissions reduced by increasing attic insulation. All data inputs and outputs for our pilot Nashville-based rental unit can be seen in Figure 3.
3.3 Per-unit costs for retrofit-enabled carbon reduction
For each of the 4 major efficiency retrofits, we estimate the amount of carbon reduction attainable by a dollar invested in that type of retrofit, using Equation 10 below. That is, we calculate the amounts of CO2 reduction that can be achieved by $1 invested in a refrigerator upgrade, HVAC upgrade, window upgrades, and attic insulation, respectively, which allows for easy comparison among different retrofits. Additionally, we calculate the price tag for reducing 1lb of CO2 through a particular type of energy efficiency retrofit (Equation 11). That is, we calculate the cost (excluding labor costs) for 1lb CO2 reductions achieved through window upgrades, so that we can compare it to the cost for 1lb of CO2 reductions attained through attic insulation, refrigerator, and HVAC upgrades, respectively.
These quantifications allow for ready comparison across the different types of energy efficiency upgrades, while enabling easier, more transparent marketing to potential buyers of the carbon offsets generated by retrofit-enabled energy savings and their corresponding GHG reductions.
4 Results
Using our methodology to estimate the energy use and carbon reductions from energy efficiency upgrades to a model stand-alone affordable rental unit, we find significant retrofit-enabled energy savings and correspondingly substantial annual reductions in carbon emissions (Table 2). Further, we find that the aggregate CO2 reductions generated during the 25-year life-span of a comprehensive energy-efficiency retrofit should go all or most of the way towards financing the cost of the retrofit when the efficiency-enabled reductions in CO2 emissions are sold as “social carbon” credits on the voluntary carbon market. If social carbon offsets generated by low-income energy upgrades garner the higher prices more typical for offsets with significant and immediate social, health, and economic co-benefits (Gold Standard, 2016; Ecosystem Marketplace, 2023), revenue generated by the sale of efficiency-based offsets may even exceed the materials costs of energy upgrades, helping cover the labor costs for more labor intensive upgrades to the building envelope (window and attic insulation upgrades).
Below, we present our findings on the implementation costs for each of the 4 types of retrofits (excluding labor costs), along with findings on the utility bill savings and CO2 reductions generated by implementing each type of retrofit to a model unit. We also present the price tag for each lb of CO2 reduction achieved through a particular appliance or building envelope upgrade, and we highlight the amount of CO2 reduction attainable through an $1 investment in each type of efficiency retrofit.
4.1 The costs and benefits of efficiency retrofits, by component
Our model-unit-based calculations indicate that the most significant energy savings, cost savings, and CO2 reductions come from deep-efficiency upgrades, namely, an increase in attic insulation, window and HVAC upgrades. Appliance upgrades produce measurable additions in energy savings, contributing meaningful but much smaller reductions to utility costs and CO2 emissions (Table 2).
Given that over 25% of a home’s heat can escape through its attic, it is perhaps unsurprising that increasing attic insulation emerges as the single most impactful component of an energy efficiency upgrade to a low-income housing unit (Greenlink Energy, 2021). Increasing the attic insulation of the model energy-inefficient rental unit generates the largest reductions in household energy use, producing the largest amount of annual utility bill savings (Table 2). This is also the most cost-effective upgrade, as it yields the highest annual CO2 emissions reductions per dollar spent.
Compared to attic insulation, the other three retrofits (upgrading to a more efficient windows, heat pump, and refrigerator) have roughly equal impact in terms of annual CO2 reductions per $1 spent on each type of retrofit. Window and HVAC replacements produce roughly equal CO2 reductions annually and over the lifespan of each retrofit, while the carbon saving generated through a fridge upgrade are less than 4% of those generated by window or HVAC replacement (Tables 2, 3).
Window replacement costs over 9 times that of increasing attic insulation, yet results in yearly CO2 emissions reductions that are nearly 280 times less than those produced by increasing attic insulation. Though replacing windows is the second most significant retrofit in terms of annual energy cost savings, its carbon-reduction impact is roughly equivalent to that of replacing a unit’s heat pump, ands costs of window and HVAC replacements are quite similar as well. Given that a lot of heat can escape from single-pane windows in winter, and it is difficult to keep a home with single-pane windows properly cooled in the summer, replacing windows ultimately still yields significant energy use and CO2 reductions over their lifespan of the upgrade.
In comparison to increasing attic insulation, upgrading to a more energy efficient heat pump is both more expensive, in absolute terms, and less impactful, in terms of annual CO2 reductions. Still, an HVAC upgrade is a key component of a comprehensive energy efficiency retrofit because upgrading to a more efficient heat pump still produces significant utility and carbon savings over the lifespan of the appliance (Tables 2, 3).
Finally, refrigerator replacement produces the lowest annual savings on utilities, and the lowest annual carbon reductions among the four upgrades. However, it is the least costly and easiest retrofit to implement, which makes it the most readily accessible option. On the other hand, when we look at the price of achieving 1 lb of annual CO2 reduction, the refrigerator upgrade is most expensive of the four studies upgrades, making it the cheapest by total price but the least cost-effective. Analyzing the upgrades in this manner conveys how the cheapest upgrade by price tag is not necessarily the most cost-effective.
4.2 The market value and project finance capacity of carbon offsets generated through energy efficiency upgrades
Assessing the potential to fund energy efficiency upgrades to low-income housing through the up-front sale of carbon offsets generated by such upgrades is a core objective of this research. To meet this objective, we estimate the VCM value of CO2 reductions and their corresponding offset credits.
Calculations on the expected monetary value of offset credits are conducted with a standard assumption for a 25-year lifespan for the energy efficiency retrofits. This means we estimate total emissions reduction per retrofit type–and the total amount of carbon offset credits – by multiplying annual carbon reductions times 25 (Table 3). Since the carbon offsets (and corresponding offset credits) generated through our proposed energy efficiency interventions go beyond just positive climate impact to also generate economic and health co-benefits for members of marginalized groups and populations, our estimates apply pricing ranges typical for high-quality offsets with intended and measurable social co-benefits (Ecosystem Marketplace, 2023). That is, our estimates use some of the higher “social carbon” pricing as, for example, reflected in offsets registered under the Gold Standard carbon registry (Gold Standard, 2016), rather than relying on the low pricing for multiple problematic forestry and land use offsets (CAP, 2022).
5 Discussion
5.1 Value added from this project
By developing a practical and novel way of mobilizing private governance tools — carbon offsetting and the voluntary carbon market — towards advancing the intersecting public goals of improving home energy efficiency and reducing energy cost burdens for economically and socially marginalized groups, we introduce a promising new approach to filling some critical gaps left by public policies on climate and energy equity. Implementing the conceptual and methodological tools developed in this paper can help mitigate climate change while improving energy and health equity in the US and other high-income countries.
From the perspective of societal and public policy goals, our approach and methodology facilitate steering some of the increasing corporate climate spending and investment towards climate mitigation projects that reduce carbon emissions through meeting essential energy and health needs for marginalized groups.
Taking the perspective of corporate and institutional actors pursuing climate mitigation and a range of other related and unrelated ESG activities, purchasing carbon offsets generated through energy efficiency upgrades to low-income housing gives companies an opportunity to have a tangible positive impact on the health and wellbeing of communities where they operate. Simultaneously, it enables them to advance their climate action goals whilst bolstering their image and reputational capital with a wide range of corporate stakeholders (including, but not limited to, host communities).
The approach and methodology we have developed also grants companies the ability to achieve valuable synergies among ESG goals by purchasing a stake in projects that reduce carbon emissions while generating economic and health co-benefits for members of the communities hosting corporate facilities and operations. Beyond the positive social and health spillovers associated with a carbon offset derived through energy efficiency retrofits to low-income housing, we expect that corporate willingness to invest in this type of carbon offset (over alternatives which do not offer such co-benefits) will be enhanced by several additional factors.
First, a growing public and community sentiment that corporate expansion in urban regions brings many harms and few benefits to lower-income, marginalized groups within these regions (Chung, 2020; Guzman, 2021) should make many corporate actors more open to buying carbon offsets from projects that are welcomed by communities hosting their corporate offices and operations, and which directly advance economic wellbeing and human development for the least privileged members of those communities (Lou et al., 2023).
Additionally, carbon offset projects that are located in physical proximity to the corporate offices and operations of offset buyers — such as carbon offsets generated by the low-income energy efficiency retrofits examined in this paper — should be particularly attractive in a context where the legitimacy, effectiveness, and social impacts of many distant carbon forestry projects are increasingly coming into question (Greenfield, 2023; Haya et al., 2023). While forest carbon credits are commonly purchased by corporate and institutional buyers (Macfarlene, 2022), they often come from carbon forestry projects located in the Global South but developed by and serving the climate commitments of companies and countries from the Global North (Shin et al., 2022). Yet many of the forest carbon projects developed for the purposes of carbon offsetting have done a less than stellar job on their main task of climate mitigation, while also generating a range of economic and livelihood risks and problems for host communities (Aggarwal and Brockington, 2020; Greenfield, 2023; Leach and Scoones, 2015).
In this context, putting carbon offset spending to work on offset projects that generate economic and health benefits for marginalized groups in a company’s host community — projects which are desired and welcomed by these communities — should be increasingly desirable for a number of corporate actors, even if the co-benefits of these projects put them at a price tag much higher than the average VCM credit (Gold Standard, 2016; Lou et al., 2023). Indeed, many companies will likely be able to justify the higher price per offset, given the multiple benefits a corporate buyer of efficiency-based offset credits would derive from investing in such credits. These benefits begin with climate mitigation and fulfillment of corporate climate commitments and continue to advancing the social aspect of ESG commitments, thus helping improve reputational capital with key community, civil society, and government stakeholders (Fombrun et al., 2000).
Indeed, we expect that some corporate actors buying carbon offsets generated by energy efficiency upgrades to low-income rentals may be more attracted to the social, economic and health co-benefits generated by these projects than the emission reductions and climate mitigation value of the project. In other words, the need to build or strengthen trust and reputational capital among community stakeholders may be the primary driver for some corporate purchasers of carbon offsets from energy efficiency upgrades to low-income housing.
The biggest challenge likely encountered by carbon offsets based on emission reductions from low-income energy upgrades is a challenge on additionality. In particular, given the numerous government programs targeted at improving energy efficiency and affordability for low-income households in the US (and other developed countries), efficiency-based offsets may be perceived as lacking in additionality, on the assumption that such upgrades would have happened with or without financing from offset purchases by corporate and institutional actors. Indeed, the carbon offsets generated by Clearloop’s innovative approach to solar development in dirty US electric grid regions are categorically excluded from certification under the major carbon registries (like Verra, American Carbon Registry) based on the assumption that renewables will be developed in Global North countries like the US regardless of any investment by corporate offset buyers (Verra, 2021). This assumption is nonetheless defied by on-the-ground realities (Vandenbergh and Gilligan, 2017), and overcome by Clearloop, who has verified its Lifecycle Carbon Standard and Decarbonization Credit Methodology under the Greenhouse Gas Protocol Project Standard, successfully selling permanent, additional, transparent offsets secured against double-counting to a range of companies, whose offset purchases are helping finance the development of new solar throughout the US Southeast (Clearloop, 2021). Further, while such categorical exclusions and other additionality objections can act to obstruct the development of climate- and socially-beneficial offset interventions like that offered by Clearloop, forest carbon credits of questionable climate and social impacts are being readily transacted to justify carbon neutrality claims for massive carbon footprint events such as the 2022 Qatar FIFA World Cup, where air-conditioned open-air stadiums sited in desert heat were claimed carbon neutral based in significant part on the purchase of cheap forest carbon offsets (How to Save a Planet, 2022).
While potentially more challenging to certify than its pioneering MaineHousing predecessor, the efficiency-upgrade based carbon offsets proposed in this paper can expand the reach and impact of the MaineHousing program, which reached only low-income homeowners and required time-and resource-intensive data collection to implement. MaineHousing’s carbon offsets have been certified under Verified Carbon Standard (VCS) and listed in the large, global reach VCS carbon registry run by the nonprofit Verra, but VCS certification is extremely data intensive, taking 3 years of data collecting in the MaineHousing case (Maine State Housing Authority, 2012), and thus limiting the reach and impact of offset-financed energy efficiency retrofits to low-income housing. Though our approach may be more challenging to certify under the existing rules of established carbon registries, it offers tangible and verifiable, emission-reduction based carbon offsets with measurable social and economic co-benefits — and does so at a time when the credibility of these registries, along with the authenticity and impact of their offsets — have been called into question (Gill-Wiehl et al., 2024; Greenfield, 2023).
Our study fills important gaps in MaineHousing’s pioneering carbon offset program by developing an accessible and less data-intensive method to estimate carbon emissions reductions from residential weatherization and energy efficiency upgrades. In order to do so, this method takes advantage of open-source data and tools, cutting down on the time needed to collect, manage, and analyze data. Additionally, this project focuses on individual upgrades within individual units, allowing a wider spectrum of potential offset purchasers to fund upgrades on smaller scales, increasing the audience of companies that can afford to invest in residential upgrades. This enables a significant scaling of MaineHousing’s intervention to low-income rental housing, which houses a larger proportion of marginalized groups than owner-occupied low-income housing. While this method compromises in the rigor of data collection, it increases co-benefits for both low-income residents and investing companies, and it reaches a wider population of potential offset purchasers and marginalized communities.
5.2 What does a scale-up look like in actual practice?
The methodology developed in this project to calculate CO2 reductions from energy efficiency upgrades provides the basis for a potential socially responsible carbon offset. The CO2 emissions calculated from each potential energy efficiency upgrade could be sold as an individual carbon offset. In other words, a company that is interested in financing a locally-based carbon offset project with local co-benefits, but potentially unable to invest the full amount required to complete all 4 components of an energy efficiency upgrade can invest in completing 1 of the 4 types of retrofit, leaving the other retrofits open for financing by other corporate and institutional actors. While purchasing all the offsets generated over the 25-year lifespan of a home energy upgrade — and thus providing upfront financing for completing the energy efficiency upgrade for that unit — may well be within the climate and ESG budget of larger companies, our approach and methods make it possible for much smaller companies to participate in backing this type of offset project. Companies such as small businesses with roots in the community, or smaller and newly established B corporations whose goals and practices are already aligned with the idea of positive spillovers will be able to participate as well.
The fact that each type of efficiency upgrade (fridge, HVAC, windows, insulation) can be financed by different offset buyers makes this approach more likely to scale—as it is not a priori limited by the company and budget size of the corporate and institutional actors seeking to purchase carbon offsets with locally-experienced social, economic, and health co-benefits. Scalability is further advanced by the fact that each efficiency upgrade on a home or rental can be financed by several offset buyers, each of whom purchases a small portion of the offsets generated by that upgrade.
As shown in Table 3, the revenues generated from the sale of “social carbon” offsets through energy efficiency upgrades to low-income rental units can exceed the total materials cost for implementing these efficiency upgrades, particularly if the social carbon offsets generated through low-income efficiency retrofits are priced in accordance with the tangible health, economic, and equity co-benefits they bring to marginalized groups – which would mean pricing on the highest end of the social offset continuum. Such pricing could also allow for offset sales to cover the labor cost for the more labor-intensive upgrades, such as the window and insulation upgrades. While the precise financing calculus remains beyond the scope of this paper, the analysis we present demonstrates significant practical promise and feasibility of carbon offset financing for energy efficiency upgrades to low-income affordable rental units. More broadly, it demonstrates the environmental and social value of mobilizing a private governance solution like voluntary carbon offsetting in the service of long-standing and challenging to accomplish public policy goals for climate mitigation, energy equity, and just environmental and renewable transitions.
6 Conclusion
This study builds on existing ideas and practices to introduce an accessible new methodology for calculating CO2 reductions from energy efficiency upgrades to low-income housing. Calculations enabled by this methodology, in turn, allow for mobilizing a new source of financing for such low-income energy upgrades - emission reductions can be packaged and sold as carbon offsets on the voluntary carbon market, with revenue from the sales footing the up-front cost of upgrading energy inefficient appliances, windows, and insulation. Carbon offsets derived from this type of project are expected to attract corporate buyers looking to meet climate goals and social responsibilities, to build or bolster reputational capital with a range of stakeholders, including host communities, civil society activists, regulators, and the media. The methodology and conceptual approach proposed in this paper enable the mobilizing of a promising market-based tool for alleviating energy poverty and health inequalities in the US and other developed countries. Future research should explore the expansion and scaling of this model, and work to identify other opportunities for interventions that reduce carbon emissions through meeting pressing and long under-addressed needs of marginalized groups and populations in high-income countries.
Data availability statement
The original contributions presented in the study are included in the article/supplementary material, further inquiries can be directed to the corresponding author.
Author contributions
MM-S: Data curation, Formal Analysis, Investigation, Methodology, Validation, Visualization, Writing–original draft, Writing–review and editing. ZT: Conceptualization, Data curation, Formal Analysis, Funding acquisition, Methodology, Project administration, Resources, Supervision, Validation, Visualization, Writing–original draft, Writing–review and editing. CZ: Conceptualization, Funding acquisition, Investigation, Project administration, Resources, Supervision, Writing–review and editing. AL: Investigation, Writing–review and editing. SL: Investigation, Writing–review and editing. YY: Investigation, Writing–review and editing. JM: Conceptualization, Project administration, Resources, Supervision, Writing–review and editing.
Funding
The author(s) declare that financial support was received for the research, authorship, and/or publication of this article. This research was supported by funding through the Vanderbilt Provost’s RAMP (Rapid Advancement Micro-Grant) and Immersion Programs. Funding support for this work was also provided by Rama and Sid Das, who donated research funding to the CHEEL lab, where the research behind this article was based.
Conflict of interest
The authors declare that the research was conducted in the absence of any commercial or financial relationships that could be construed as a potential conflict of interest.
Publisher’s note
All claims expressed in this article are solely those of the authors and do not necessarily represent those of their affiliated organizations, or those of the publisher, the editors and the reviewers. Any product that may be evaluated in this article, or claim that may be made by its manufacturer, is not guaranteed or endorsed by the publisher.
References
AAFA (2020). Asthma disparities in America. Arlington, VA: Asthma and Allergy Foundation of America. Available at: https://aafa.org/wp-content/uploads/2022/08/asthma-disparities-in-america-burden-on-racial-ethnic-minorities.pdf.
Aggarwal, A., and Brockington, D. (2020). Reducing or creating poverty? Analyzing livelihood impacts of forest carbon projects with evidence from India. Land Use Policy 95, 104608. doi:10.1016/j.landusepol.2020.104608
Alizadeh, M. R., Abatzoglou, J. T., Adamowski, J. F., Prestemon, J. P., Chittoori, B., Akbari Asanjan, A., et al. (2022). Increasing heat-stress inequality in a warming climate. Earth’s Future 10 (2), e2021EF002488. doi:10.1029/2021EF002488
Amann, J., Srivastava, R., and Henner, N. (2021). Pathways for deep energy use reductions and decarbonization in homes.
American Council for an Energy Efficient Economy (2017). How energy efficiency can help low-income households in Tennessee. Available at: https://www.aceee.org/sites/default/files/pdf/fact-sheet/ses-tennessee-100917.pdf.
Amskaty (2024). Explore what is AC SEER rating—AMS AC and heating. AMS A/C and Heat. Available at: https://amskaty.com/what-is-ac-seer-rating/(Accessed May 14, 2024).
Anderson, E. K., and Zerriffi, H. (2012). Seeing the trees for the carbon: agroforestry for development and carbon mitigation. Clim. Change 115 (3–4), 741–757. doi:10.1007/s10584-012-0456-y
Antunes, M., Teotónio, C., Quintal, C., and Martins, R. (2023). Energy affordability across and within 26 European countries: insights into the prevalence and depth of problems using microeconomic data. Energy Econ. 127, 107044. doi:10.1016/j.eneco.2023.107044
Battocletti, V., Enriques, L., and Romano, A. (2023). The voluntary carbon market: market failures and policy implications. [ECGI Working Paper Series in Law]. European Corporate Governance Institute. Available at: https://www.ecgi.global/sites/default/files/working_papers/documents/thevoluntarycarbonmarket.pdf.
Bednar, D. J., and Reames, T. G. (2020). Recognition of and response to energy poverty in the United States United States. Nat. Energy, 5(6), 432–439. doi:10.1038/s41560-020-0582-0
Blake, H. (2023). The great cash-for-carbon hustle. New Yorker. Available at: https://www.newyorker.com/magazine/2023/10/23/the-great-cash-for-carbon-hustle.
Bradshaw, J. L., Bou-Zeid, E., and Harris, R. H. (2016). Greenhouse gas mitigation benefits and cost-effectiveness of weatherization treatments for low-income, American, urban housing stocks. Energy Build. 128, 911–920. doi:10.1016/j.enbuild.2016.07.020
Broekhoff, D., Gillenwater, M., Colbert-Sangree, T., and Cage, P. (2019). Securing climate benefit: a guide to using carbon offsets.
Brown, M. A., Soni, A., Lapsa, M. V., Southworth, K., and Cox, M. (2020). High energy burden and low-income energy affordability: conclusions from a literature review. Prog. Energy 2 (4), 042003. doi:10.1088/2516-1083/abb954
Bumpus, A. (2011). “Realizing local development in the carbon commodity chain: political economy, value and connecting carbon commodities at multiple scales,” in Paper presented at the UNRISD conference Green Economy and Sustainable Development: Bringing Back the Social Dimension, Geneva, Switzerland, October 10–11, 2011. United Nations Research Institute for Social Development.
CAP (2022). The CFTC should raise standards and mitigate fraud in the carbon offsets market. Center for American Progress. Available at: https://www.americanprogress.org/article/the-cftc-should-raise-standards-and-mitigate-fraud-in-the-carbon-offsets-market/.
CDC (2021). Asthma-related emergency department visits 2010–2018. Available at: https://www.cdc.gov/asthma/asthma_stats/asthma-ed-visits_2010-2018.html.
Celedón, J. C., Roman, J., Schraufnagel, D. E., Thomas, A., and Samet, J. (2014). Respiratory health equality in the United States. The American thoracic society perspective. Ann. Am. Thorac. Soc. 11 (4), 473–479. doi:10.1513/AnnalsATS.201402-059PS
Chung, C. (2020). A year after amazon rejection, both sides of battle applying lessons learned. the city. Available at: https://www.thecity.nyc/2020/02/13/a-year-after-amazon-rejection-both-sides-of-battle-applying-lessons-learned/.
CIMA (2024). Insulation savings calculator. Cellulose Insulation Manufacturers Association. Available at: https://cellulose.org/insulation-savings-calculator/(Accessed May 21, 2024).
Clearloop (2021). The Clearloop approach to climate action. Clearloop. Available at: https://clearloop.us/2021/02/16/clearloop-approach-to-climate-action/.
Desmond, M., Gershenson, C., and Kiviat, B. (2015). Forced relocation and residential instability among urban renters. Soc. Serv. Rev. 89 (2), 227–262. doi:10.1086/681091
Drehobl, A. (2020). Weatherization cuts bills and creates jobs but serves only a tiny share of low-income homes. ACEEE Blog. Available at: https://www.aceee.org/blog-post/2020/07/weatherization-cuts-bills-and-creates-jobs-serves-only-tiny-share-low-income.
Drehobl, A., and Ross, L. (2016). How energy efficiency can improve low income and underserved communities.
Drehobl, A., Ross, L., and Ayala, R. (2020). How high are energy cost burdens? An assessment of national and metropolitan energy burden across the United States. Washington, DC: american council for an energy-efficient economy.
Dunne, D., and Quiroz, Y. (2023). Mapped: the impacts of carbon-offset projects around the world. Carbon Brief. Available at: https://interactive.carbonbrief.org/carbon-offsets-2023/mapped.html.
Ecosystem Marketplace (2023). Voluntary carbon credit buyers willing to pay more for quality. Carbon Credits. Available at: https://carboncredits.com/voluntary-carbon-credit-buyers-willing-to-pay-more-for-quality/.
Energy Saver (2024). Energy-efficient mortgages. U.S. Department of Energy. Available at: https://www.energy.gov/energysaver/energy-efficient-mortgages (Accessed March 3, 2024).
Evens, A., Garascia, M., and Isaacson, M. (2017). Utilities and health: energy efficiency as a common link. Electr. J. 30 (5), 10–14. doi:10.1016/j.tej.2017.04.009
Favretto, N., Afionis, S., Stringer, L. C., Dougill, A. J., Quinn, C. H., and Ranarijaona, H. L. T. (2020). Delivering climate-development Co-benefits through multi-stakeholder forestry projects in Madagascar: opportunities and challenges. Land 9 (5), 157. Article 5. doi:10.3390/land9050157
Fombrun, C. J., Gardberg, N. A., and Barnett, M. L. (2000). Opportunity platforms and safety nets: Corporate citizenship and reputational risk. Business and society review 105 (1), 85–106.
Forest Trends (2023). Report: Voluntary carbon markets demand in 2023 is concentrating around pricier, high-integrity credits. Forest Trends. Available at: https://www.forest-trends.org/pressroom/report-voluntary-carbon-markets-demand-in-2023-is-concentrating-around-pricier-high-integrity-credits/.
Francisco, P. W., Jacobs, D. E., Targos, L., Dixon, S. L., Breysse, J., Rose, W., et al. (2017). Ventilation, indoor air quality, and health in homes undergoing weatherization. Indoor Air 27 (2), 463–477. doi:10.1111/ina.12325
Gifford, L. (2020). “You can’t value what you can’t measure”: a critical look at forest carbon accounting. Clim. Change 161 (2), 291–306. doi:10.1007/s10584-020-02653-1
Gill-Wiehl, A., Kammen, D. M., and Haya, B. K. (2024). Pervasive over-crediting from cookstove offset methodologies. Nat. Sustain. 7 (2), 191–202. doi:10.1038/s41893-023-01259-6
Gold Standard (2016). Why do prices vary by project type? Gold Standard. Available at: https://www.goldstandard.org/news/why-do-prices-vary-by-project-type.
Gold Standard (2017). Gold standard for the global goals transition annex (to be used by all GS CDM/VER stand alone projects and PoAs, Micro scale stand alone projects and Micro PoAs). Available at: https://www.terraclear.org/wp-content/uploads/2021/05/TC_GS4GG_Transition-Annex_CP2.pdf.
Goldstein, B., Reames, T. G., and Newell, J. P. (2022). Racial inequity in household energy efficiency and carbon emissions in the United States: an emissions paradox. Energy Res. and Soc. Sci. 84, 102365. doi:10.1016/j.erss.2021.102365
Greenfield, P. (2023). Revealed: more than 90% of rainforest carbon offsets by biggest certifier are worthless, analysis shows. The Guardian Available at: https://www.theguardian. com/environment/2023/jan/18/revealed-forest-carbon-offsets-biggest-provider- worthless-verra-aoe.
Greenlink Energy (2021). How much heat is lost through the attic? Greenlink Energy Solutions Available at: https://usgreenlink.com/blog/how-much-heat-is-lost-through-the-attic/.
Guzman, D. T. (2021). Community activists push back on Oracle deal • Tennessee Lookout. Tenn. Lookout. Available at: https://tennesseelookout.com/briefs/community-activists-push-back-on-oracle-deal/.
Haya, B., Cullenward, D., Strong, A. L., Grubert, E., Heilmayr, R., Sivas, D. A., et al. (2020). Managing uncertainty in carbon offsets: insights from California’s standardized approach. Clim. Policy 20 (9), 1112–1126. doi:10.1080/14693062.2020.1781035
Haya, B. K., Evans, S., Brown, L., Bukoski, J., Butsic, V., Cabiyo, B., et al. (2023). Comprehensive review of carbon quantification by improved forest management offset protocols. Front. For. Glob. Change 6. doi:10.3389/ffgc.2023.958879
Hernández, D., and Siegel, E. (2019). Energy insecurity and its ill health effects: a community perspective on the energy-health nexus in New York City. Energy Res. and Soc. Sci. 47, 78–83. doi:10.1016/j.erss.2018.08.011
HHS (2022). Asthma and african Americans. Office of Minority Health. Available at: https://minorityhealth.hhs.gov/asthma-and-african-americans.
HHS (2023). 2023 poverty guidelines: 48 contiguous states (all states except Alaska and Hawaii). Available at: https://aspe.hhs.gov/sites/default/files/documents/1c92a9207f3ed5915ca020d58fe77696/detailed-guidelines-2023.pdf.
Howden-Chapman, P., Matheson, A., Crane, J., Viggers, H., Cunningham, M., Blakely, T., et al. (2007). Effect of insulating existing houses on health inequality: cluster randomised study in the community. BMJ Br. Med. J. 334 (7591), 460. doi:10.1136/bmj.39070.573032.80
Inspire Clean Energy (2024). How much electricity does an air conditioner use? Available at: https://www.inspirecleanenergy.com/blog/sustainable-living/how-much-electricity-does-air-conditioning-use (Accessed May 21, 2024).
KFF (2022). Distribution of total population by federal poverty level. Available at: https://www.kff.org/other/state-indicator/distribution-by-fpl/?currentTimeframe=0&sortModel=%7B%22colId%22:%22Location%22,%22sort%22:%22asc%22%7D.
Khatana, S. A. M., Werner, R. M., and Groeneveld, P. W. (2022). Association of extreme heat with all-cause mortality in the contiguous US, 2008-2017. JAMA Netw. Open 5 (5), e2212957. doi:10.1001/jamanetworkopen.2022.12957
Kilroy, A. (2024). Renting statistics 2024. Forbes Advis. Available at: https://www.forbes.com/advisor/renters-insurance/renting-statistics/.
Kokai, M. (2011). Dubious carbon offsets. John Locke Foundation. Available at: https://www.johnlocke.org/dubious-carbon-offsets/.
Kolokotsa, D., and Santamouris, M. (2015). Review of the indoor environmental quality and energy consumption studies for low income households in Europe. Sci. Total Environ. 536, 316–330. doi:10.1016/j.scitotenv.2015.07.073
Kontokosta, C. E., Reina, V. J., and Bonczak, B. (2020). Energy cost burdens for low-income and minority households: evidence from energy benchmarking and audit data in five U.S. Cities. J. Am. Plan. Assoc. 86 (1), 89–105. doi:10.1080/01944363.2019.1647446
Kreibich, N., and Hermwille, L. (2021). Caught in between: credibility and feasibility of the voluntary carbon market post-2020. Clim. Policy 21 (7), 939–957. doi:10.1080/14693062.2021.1948384
Kremen, C., and Merenlender, A. M. (2018). Landscapes that work for biodiversity and people. Science 362 (6412), eaau6020. doi:10.1126/science.aau6020
Kvale, L. (2021). Solar in the shadows Part 2: clearing the pathway for private sector action to accelerate America’s clean energy transition. Clearloop. Available at: https://clearloop.us/wp-content/uploads/2021/02/Solar-in-the-Shadows-Part-2-_Clearing-the-Pathway-for-Private-Sector-Action-to-Accelerate-Americas-Clean-Energy-Transition.pdf.
M. Leach, and I. Scoones (2015). Carbon conflicts and forest landscapes in Africa (London:Routledge), 232.
López-Vallejo, M. (2022). “Non-additionality, overestimation of supply, and double counting in offset programs: insight for the Mexican carbon market,” in Towards an emissions trading system in Mexico: rationale, design and connections with the global climate agenda. Editor S. Lucatello (Springer International Publishing), 191–221. doi:10.1007/978-3-030-82759-5_10
Lou, J., Hultman, N., Patwardhan, A., and Mintzer, I. (2023). Corporate motivations and co-benefit valuation in private climate finance investments through voluntary carbon markets. Npj Clim. Action 2 (1), 32–14. doi:10.1038/s44168-023-00063-4
Lovell, H. C. (2010). Governing the carbon offset market. Clim. Change 1 (3), 353–362. doi:10.1002/wcc.43
Lyons, K., Richards, C., and Westoby, P. (2014). The darker side of green plantation forestry and carbon violence in Uganda. Oakland, California: The Oakland Institute. Available at: https://www.oaklandinstitute.org/sites/oaklandinstitute.org/files/Report_DarkerSideofGreen_hirez.pdf.
Lyons, K., and Westoby, P. (2014). Carbon colonialism and the new land grab: plantation forestry in Uganda and its livelihood impacts. J. Rural Stud. 36, 13–21. doi:10.1016/j.jrurstud.2014.06.002
Macfarlene, M. (2022). Assessing the state of the voluntary carbon market in 2022. Carbon Direct. Available at: https://www.carbon-direct.com/insights/assessing-the-state-of-the-voluntary-carbon-market-in-2022.
Maine State Housing Authority (2012). Financing residential energy efficiency with carbon offsets. Available at: https://www.mainehousing.org/docs/default-source/carbon/financing-residential-energy-efficiency-with-carbon-offsets.pdf.
Mason, C. F., and Plantinga, A. J. (2013). The additionality problem with offsets: optimal contracts for carbon sequestration in forests. J. Environ. Econ. Manag. 66 (1), 1–14. doi:10.1016/j.jeem.2013.02.003
Mast, B., Hummel, H., and Clinton, J. (2020). Towards an accessible financing solution: a policy roadmap with program implementation considerations for tariffed on-bill programs in California. Building Decarbonization Coalition. Available at: https://buildingdecarb.org/wp-content/uploads/Towards-an-Accessible-Financing-Solution.pdf.
Mateyka, P., and Yoo, J. (2023). Share of income needed to pay rent increased the most for low-income households from 2019 to 2021. US Census Bureau. Available at: https://www.census.gov/library/stories/2023/03/low-income-renters-spent-larger-share-of-income-on-rent.html.
Miltenberger, O., Jospe, C., and Pittman, J. (2021). The good is never perfect: why the current flaws of voluntary carbon markets are services, not barriers to successful climate change action. Front. Clim. 3. doi:10.3389/fclim.2021.686516
Murray, A. G., and Mills, B. F. (2014). The impact of low-income home energy assistance program participation on household energy insecurity. Contemp. Econ. Policy 32 (4), 811–825. doi:10.1111/coep.12050
NASCSP (2018). NASCSP deferral tracking report summer 2018. NASCSP. Available at: https://nascsp.org/wp-content/uploads/2019/12/Report_NASCSP-Deferral-Tracking-2018.pdf.
NASCSP (2022). PY22 WAP annual funding report. Available at: https://nascsp.org/wp-content/uploads/2023/12/PY22-WAP-Annual-Funding-Report-1.pdf.
National Academies of Sciences, Engineering, and Medicine (2023). “The built environment,” in Accelerating decarbonization in the United States: technology, policy, and societal dimensions (The National Academies Press), 277–324. doi:10.17226/25931
National Low Income Housing Coalition (2024). Gap report: Tennessee. Available at: https://nlihc.org/gap/state/tn.
Office of Community Services (2023). Low income home energy assistance program (LIHEAP). Administration for children and families. Department of Health and Human Services. Available at: https://www.acf.hhs.gov/ocs/programs/liheap.
Office of State and Community Energy Programs (2024). Weatherization assistance program. U.S. Department of Energy. Available at: https://www.energy.gov/scep/wap/weatherization-assistance-program (Accessed February 28, 2024).
Pierce, G., Coffee, D., and Steinberg, R. (2023). “LA100 equity strategies,”. [UCLA Report] in Chapter 13 energy affordability and policy solutions analysis. Available at: https://escholarship.org/content/qt8h37k87j/qt8h37k87j.pdf.
Quimby, B. (2011). Chevrolet deal drives Maine low-income weatherization. Press Herald. Available at: https://www.pressherald.com/2011/02/22/chevrolet-deal-drives-maine-low-income-weatherization_2011-02-22/.
Reames, T. G., Daley, D. M., and Pierce, J. C. (2021). Exploring the nexus of energy burden, social capital, and environmental quality in shaping health in US counties. Int. J. Environ. Res. Public Health 18 (2), 620. Article 2. doi:10.3390/ijerph18020620
Schneider, L., Michaelowa, A., Broekhoff, D., Espelage, A., and Siemons, A. (2019). Lessons learned from the first round of applications by carbon-offsetting programs for eligibility under CORSIA. Amsterdam: Climate Focus, Perspectives Climate Group.
Shin, S., Park, M. S., Lee, H., and Baral, H. (2022). The structure and pattern of global partnerships in the REDD+ mechanism. For. Policy Econ. 135, 102640. doi:10.1016/j.forpol.2021.102640
Simon, G. L., Bumpus, A. G., and Mann, P. (2012). Win-win scenarios at the climate–development interface: challenges and opportunities for stove replacement programs through carbon finance. Glob. Environ. Change 22 (1), 275–287. doi:10.1016/j.gloenvcha.2011.08.007
Sycamore Institute (2024). 2022 Census data on income and poverty in Tennessee. Available at: https://www.sycamoreinstitutetn.org/2022-census-income-poverty/.
Tonn, B., Marincic, M., and Rose, E. (2024). A dollar well spent: monetizing the societal benefits of low-income weatherization programs in the United States. Energy Res. and Soc. Sci. 107, 103341. doi:10.1016/j.erss.2023.103341
Tonn, B., Rose, E., Hawkins, B., and Marincic, M. (2021). Health and financial benefits of weatherizing low-income homes in the southeastern United States. Build. Environ. 197, 107847. doi:10.1016/j.buildenv.2021.107847
Tonn, B., Schmoyer, R., and Wagner, S. (2003). Weatherizing the homes of low-income home energy assistance program clients: a programmatic assessment. Energy Policy 31 (8), 735–744. doi:10.1016/S0301-4215(02)00124-6
Tzankova, Z. (2021). Carbon offsetting can be a tool of environmental justice. Fast Company. Available at: https://www.fastcompany.com/90600561/carbon-offsetting-can-be-a-tool-of-environmental-justice.
US Census Bureau (2022). Poverty in the United States: 2022. Census.Gov. Available at: https://www.census.gov/library/publications/2023/demo/p60-280.html.
US EPA (2024). Emissions and Generation Resource Integrated Database (eGRID). Available at: https://www.epa.gov/egrid.
Vandenbergh, M., and Gilligan, J. (2017). Why private “actors” are taking center stage on climate change | GreenBiz. Greenbiz.Com. Available at: https://www.greenbiz.com/article/why-private-actors-are-taking-center-stage-climate-change.
Vandenbergh, M. P. (2018). Keynote: motivating private climate governance: the role of the efficiency gap. Ark. LAW Rev. 71.
Verra (2021). The future of the voluntary carbon market. Verra. Available at: https://verra.org/the-future-of-the-voluntary-carbon-market/.
Wittman, H. K., and Caron, C. (2009). Carbon offsets and inequality: social costs and Co-benefits in Guatemala and Sri Lanka. Soc. and Nat. Resour. 22 (8), 710–726. doi:10.1080/08941920802046858
Zanobetti, A., Ryan, P. H., Coull, B., Brokamp, C., Datta, S., Blossom, J., et al. (2022). Childhood asthma incidence, early and persistent wheeze, and neighborhood socioeconomic factors in the ECHO/CREW consortium. JAMA Pediatr. 176 (8), 759–767. doi:10.1001/jamapediatrics.2022.1446
Zapata, L., Corporation, C., Richardson, H., and James, J. (2020). Solar in the shadows: expanding access to clean energy in forgotten America. Available at: https://clearloop.us/wp-content/uploads/2020/09/White-Paper_-Solar-in-the-Shadows_-Expanding-Access-to-Clean-Energy-in-Forgotten-America-Sept-2020-7.pdf.
Keywords: energy efficiency, energy cost burdens, health disparities, climate mitigation, carbon offsets, co-benefits, social carbon
Citation: Maciel-Seidman M, Tzankova Z, Ziegler CC, Lele A, Lu S, Yan Y and Muchira JM (2024) Mobilizing carbon offsetting to reduce energy cost burdens: a new approach for calculating and monetizing the offset value of energy efficiency upgrades to low-income housing. Front. Energy Res. 12:1437560. doi: 10.3389/fenrg.2024.1437560
Received: 17 June 2024; Accepted: 26 August 2024;
Published: 16 September 2024.
Edited by:
Maria Cristina Piccirilli, University of Florence, ItalyReviewed by:
Tony Gerard Reames, University of Michigan, United StatesJacopo Gaspari, University of Bologna, Italy
Copyright © 2024 Maciel-Seidman, Tzankova, Ziegler, Lele, Lu, Yan and Muchira. This is an open-access article distributed under the terms of the Creative Commons Attribution License (CC BY). The use, distribution or reproduction in other forums is permitted, provided the original author(s) and the copyright owner(s) are credited and that the original publication in this journal is cited, in accordance with accepted academic practice. No use, distribution or reproduction is permitted which does not comply with these terms.
*Correspondence: Zdravka Tzankova, emRyYXZrYS50emFua292YUB2YW5kZXJiaWx0LmVkdQ==