- 1College of Electrical and Power Engineering, Taiyuan University of Technology, Taiyuan, China
- 2Power China Huadong Engineering Co., Ltd., Hangzhou, China
- 3Department of Electrical and Computer Engineering, Mohammad Ali Jinnah University, Karachi, Pakistan
- 4Faculty of Engineering, Islamic University of Madinah, Medina, Saudi Arabia
- 5Department of Electrical Engineering, Mehran University of Engineering and Technology, Jamshoro, Pakistan
- 6Faculty of Electrical and Control Engineering, Gdansk University of Technology, Gdansk, Poland
In this paper, a comprehensive review of the impacts and imminent design challenges concerning such EV charging stations that are based on solar photovoltaic infrastructures is presented, which is based on state-of-the-art frameworks for PV-powered charging stations and the latest case studies. The main factors that are targeted in this review are the management of an EV charging system that is a composite of PV and public grid, as well as a charging system business model that can affect consumer behavior by charging at different rates and the best design infrastructure for a PV electric vehicle charging services. The economic, environmental, and social impacts caused by the installation of PV-driven charging infrastructures are also compared. Moreover, the framework for recently emerging vehicle-to-grid and vehicle-to-home services, as well as the integration of these frameworks, is discussed. Finally, survey results for future EV demand and its public acceptance are presented. Appertaining to these issues, the case studies highlight that EV transportation between parking lots and charging stations is necessary to make the best use of the available charging stations. Statistically, in this study, it was inferred that there are no limitations on the amount of EV battery capacity that can be stored, and users of EVs can charge in both slow and fast modes. Furthermore, V2G systems are not suitable for widespread industrial use. Moreover, finding solutions and overcoming numerous obstacles remain unaddressed issues, and the size of the EV industry must reach a certain level to make profitable sales of V2B, V2H, V2G, and other V2X systems. The collected statistics indicate that although respondents generally have a favorable opinion of the mobility applications powered by photovoltaic cells, it seems unlikely that they will use them anytime soon.
1 Introduction
Many people believe that the development of electro-mobility presents a chance to lessen the adverse effects that transportation has on the environment and general public health. By setting the primary energy source to be photovoltaic (PV) systems, solutions based on EV usage can attain a significant decrease in CO2 emissions. The goal of the International Energy Agency is to make clear the potential of PV use in transportation. PV-powered charging stations (PV-CS) can function both with and without less reliance on the electrical grid, and they can be used for both slow and fast charging. PV-CS also boosts the efficient use of locally generated solar electricity and offers extra services via vehicle-to-home (V2H) and vehicle-to-grid (V2G). Recent trends in PV-CS for passenger cars are covered in a report presented in (Sierra Rodriguez et al., 2020a; Soomar et al., 2022).
It is essential to have backup sources, such as stationary storage or a connection to the public grid, because PV electricity generation is highly dependent on the weather. Both independent and grid-connected modes of operation are possible for PV-CS. Reviewing current projects reveals that slow-charging terminals are a common feature of the majority of small-scale photovoltaic infrastructure. On the other hand, using the ultra-fast charging option for EVs necessitates power grid connectivity. In addition to charging the battery storage system, the power grid frequently supplements the solar power that is available during the charging sessions. Both slow and fast charging ports can be held at the PV-CS. If the PV-CS is a microgrid (MG) system that includes chargeable stationary storage supported solely by photovoltaic sources that may or may not be connected to the public grid and makes use of an optimization system, communication system, intelligent power control, and intelligent power interface. Accordingly, MG is being researched as a potential EV charging solution in this context, enabling the utilization of PV energy at the availability and technique of generation, charge regulating, and boosting PV benefits (Soomar et al., 2023; Mouli et al., 2016; Ching, 2011).
Flow control of power in MGs for EV charging is predicated in sequence: stationary storage, PV sources, and connection to the public grid. The typical daily commutation of an electric vehicle in an urban setting is approximately 20–40 km, while a usual mode of driving will consume energy of 3–6 kWh. Multiple situations are simulated for Northern France, which is a fixed-angle estimation of 45.5 kWh/m2 in December, to determine the minimal amount of PV energy required for EV charging. Furthermore, the user presumably provides PV-CS with information at the arrival time, desired state of charge of electrical vehicle (SOCEV) at departure, at arrival, and estimated park time. According to the findings of these studies, in order to increase the benefits of PV systems, specific preliminary requirements and feasibility conditions must be met. In addition, the benefits of PV are highest if the EV is being charged daily as opposed to the charging being employed on a weekly basis and when parking time is scheduled ahead of time to maximize EV charging during the projected parking time. To facilitate communication between EV users and charging stations while also taking into account their preferences, a user interface is necessary. Energy can be supplied by the public grid as needed, and excess photovoltaic production can be fed back into the system (Tirunagari et al., 2022).
From an analytical standpoint, technological, economic, and environmental aspects have all been examined with regard to PV-CSs. The PV benefits realized for PV-CS were quantitatively evaluated through the design of a three-step technique. Four factors have been suggested in a tool to modify the PV-CS investment cost: the type of the PV based panels, the quantity of the PV panels, the available amount of the terminals, in addition to the auxiliary storage capacity. Through this program, one may size PV-CSs as efficiently as possible and then use simulation to determine the operating modes that maximize the utilization of PV energy for charging EVs. The results of evaluations based on economy and environment concerning PV-CS with 10-year stationary storage through four various destinations and conditions indicate peak Net Present Value (NPV) is present in sole grid scenario because the storage system is expensive to install. When compared to a vehicle powered by petrol, scenarios involving photovoltaics and stationary storage demonstrate lower emissions (Dang, 2018; Sierra and Rodriguez, 2020b).
EV batteries for storage opportunistically utilize energy disposal by utilizing V2G and V2H if the new services regarding PV-powered charging stations are taken into account. Current cutting-edge research indicates that as far as widespread industrial usage, V2G systems are not yet suitable. Still, numerous projects are testing V2G apps. For instance, a large-scale trial of a bidirectional EV sharing innovative PV based charging system is being conducted by the Dutch city of Utrecht. When V2G and V2H services are operated, PV-CS can offer an environmental benefit. Developing a management algorithm capable of meeting the charging and discharging demand is also necessary for the successful implementation of V2G and V2H. With EV batteries playing a twofold load to source part in the public grid during peak hours, a searching peak and valley algorithm (SPVA) that can handle the erratic nature of generation of PV, demand of EV, and V2G has been developed. The goal of SPVA is to minimize energy costs from the public grid and achieve the highest shaving and filling of the valley while defining the ideal charging/discharging start times, arrival and departure times, minimum or maximum state of charging, and initial/final states of charge (Arya et al., 2024).
Research has indicated that the success of PV-CS is mainly dependent on the visual appeal, experience of the user, and acceptance of the user for new EV charging methods. A case study conducted in France demonstrates that while certain imperatives must be considered, the PV-CS is socially accepted by a sizable majority. Furthermore, the precise effects of PV possession on EV respondent experiences and probability of adopting (buying, leasing, or using) solar mobility applications were demonstrated by a survey conducted in Netherlands. There was a noteworthy distinction in the number of electric vehicles owned by those with residential photovoltaic systems and those without, suggesting a favorable correlation between home solar energy use and a preference for electric cars. The fundamental problems and the direction for the efficient installation and usage of charging stations powered by PV are the primary concerns for the efficient deployment and utilization of PV-powered charging stations. Improved knowledge is required regarding 1) the benefits and drawbacks of slow vs fast charging from the viewpoints of the relevant parties and 2) the impact of (V2G/V2H) bidirectional charging on the lifespan of EV batteries as well as power electronics. PV-CS has the potential to assist significant drivers and play an essential role in the energy transition. In addition to changing vehicle use and driver behavior, their widespread execution will necessitate size and technical optimization of the system, which includes grid connection and stationary storage. The Ev’s extended parking duration, 45 km of driving distance, and slow charging mode enable PV-CS to optimize PV benefits (Sierra and Reinders, 2021; Stamatellos et al., 2022; Zhang et al., 2023).
Regarding renewable energy policies at the government level, China is one of the top coal-producing countries in the world. The complete generation mix of China is majorly fueled by coal. However, these resources are utilized by other businesses. As a result, China is emphasizing renewable energy sources like wind. The Wind Resource Concession (WRC) is a policy tool that encourages both the public and private sectors to invest in wind turbines in strategic places to produce coal-free energy. Since 2010, China has boosted its wind energy generation by 73 TWh. The wind potential of the country is so vast that it has tripled its annual capacity every year since 2005. Wind density in coastal regions and islands exceeds 500 W/m2 and may create 11 GW, or 4% of the total reserves of the country. The government has agreed to support the plans of the National Development and Reform Commission (NDRC) to increase capacity to 100 GW by 2016. The government is building and subsidizing wind farms to achieve this goal (Barone et al., 2019; Zenhom et al., 2023; Deshmukh and Pearce, 2021).
In 2015, the Government of Pakistan introduced the Power Generation Policy to facilitate the private power sector. This enabled and encouraged the private sector to invest in setting up new power generation projects. It also allowed the private sector to invest in public sector-based power generation projects that are in a state of being developed. Mainly, the Power Generation Policy 2015 intended to prioritize the utilization of indigenous resources while facilitating all financially involved stakeholders and protecting the environment by prompting the least-cost power generation capacity in Pakistan. An Alternative and Renewable Energy Policy was introduced in 2019 to promote and assist the country’s renewable resource development. The policy is intended to provision a supportive environment for renewable energy-based projects and boost the green energy share capacity to 20% by the year 2025 and 30% by the year 2030 consecutively through attracting private capital in the market of green energy (Calabro et al., 2019; Wittkopf et al., 2012).
Given the abovementioned different aspects highlighted in the literature, this paper presents a review of impacts and future design challenges for electric vehicle charging stations based on solar PV infrastructures. Multiple case studies and statistical results have been presented. Environmental and economic aspects of introducing PV-CSs have also been discussed, along with the social acceptance statistics (Ali and Alomar, 2023; Kassem et al., 2023a).
The paper is organized as follows. Section 1 introduces the theme of the paper, whereas Section 2 presents the state-of-the-art PV-CS overview and system infrastructures, followed by a discussion on real-world applications case studies in Section 3. Section 4 presents the international standards and charging protocols. Section 5 presents social, environmental, economic and challenging aspects of PV-CSs for electric vehicles, provides details on the latest vehicle-to-home and vehicle-to-grid services. Finally, future design challenges and horizons for PV-CSs and the paper is concluded.
2 Overview and system components
The PV-CS Generic Structure of the charging station and the integration of the EV in electrical system with energy management, power grid setup in order to take the power when ever needed in terms of solar energy is not available, the typical EV system is now shown in the Figure 1. To charge EVs, installation of PV systems can be done with car parking shades. These systems typically include PV system, electronics for maintaining power balance, energy storage, an energy management system, EV supply equipment, and other hardware such as switches, wiring, and mounting structures for an array of PV (Wu et al., 2023a; Sekhar et al., 2023; Swamynathan et al., 2023).
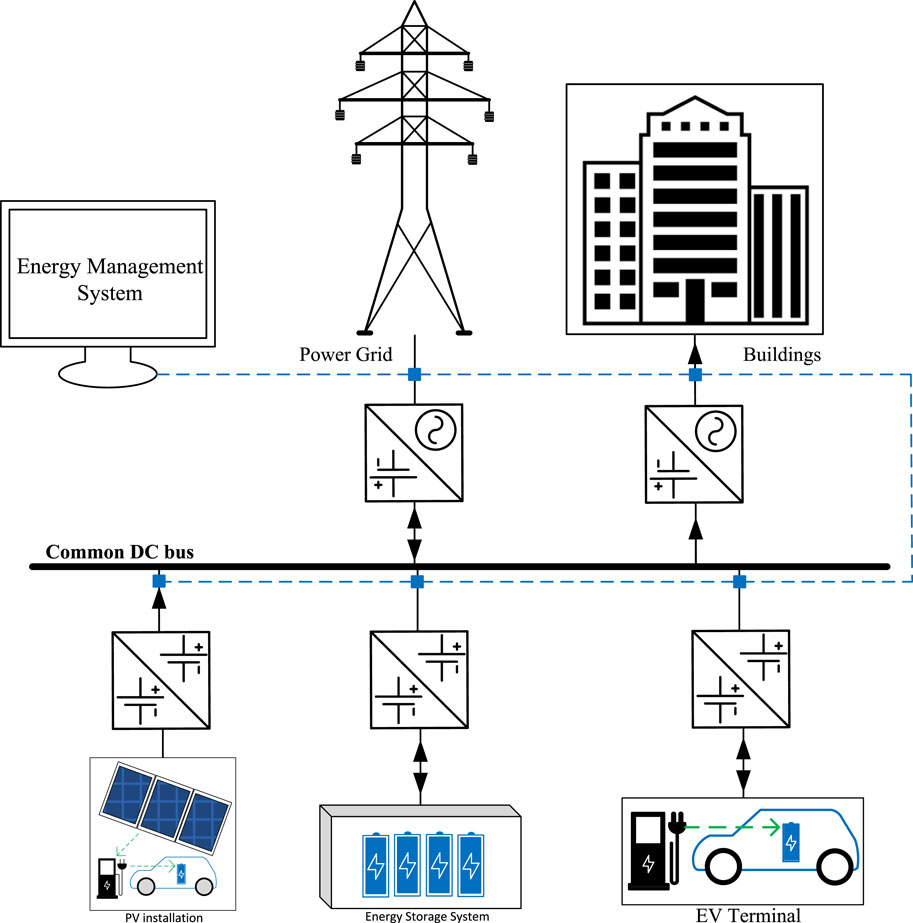
Figure 1. Typical PV driven Electrical vehicle setup (Wu et al., 2023a).
2.1 Photovoltaic systems
PV systems are renewable energy systems that transform sunlight directly into electricity via photovoltaic cell-based solar panels. These systems are made up of solar panels or arrays that absorb sunlight and generate direct current (DC) electricity, inverters that convert the DC to usable alternating current (AC), mounting structures that optimize panel orientation toward the sun, and balancing system components such as wiring and switches. PV systems can be grid-connected to feed excess electricity back into the utility grid, or stand-alone with battery storage for energy storage in off-grid environments. They are silent, have no moving parts, produce no direct emissions during operation, and use the sun’s energy to generate clean, renewable electricity for home, commercial, or utility-scale power generation (Yan et al., 2023; Pratap et al., 2022).
2.2 Energy storage system
Energy storage systems are critical components of photovoltaic-based electric vehicle charging infrastructure because they store excess solar energy for later use and provide backup power when solar irradiance is low or during peak demand. These systems help to counteract the intermittent nature of solar energy, ensuring consistent and uninterrupted charging services (Sarker et al., 2024; Liu et al., 2023a).
2.2.1 Batteries
Batteries are the most prevalent type of energy storage in photovoltaic-powered EV charging stations. They store electrical energy in the form of chemical energy that can be released as needed. Various battery technologies, including lithium-ion, lead-acid, and flow batteries, are used depending on energy density, cycle life, and cost. Proper battery management systems are critical for efficient charging and discharging, as well as extending battery life (He et al., 2019; Han et al., 2014; An, 2020a; Liu et al., 2024a).
2.2.2 Supercapacitors
Supercapacitors, sometimes known as ultracapacitors, are electrochemical energy storage devices capable of quickly storing and releasing electrical energy. They have a higher power density than batteries, which allows for faster charging and discharging times. Supercapacitors are frequently used in conjunction with batteries to handle high power demands during peak charging periods while also extending battery life by decreasing stress caused by repeated charge/discharge cycles (Yilmaz and Krein, 2012; Pathak et al., 2022; Costa et al., 2023; García-Triviño et al., 2016).
2.3 PV based grid integration and smart charging strategies
This section examines large-scale infrastructures designed primarily for PV production distribution on the power grid and self-consumption. Three factors are driving the increasing integration of PV solar energy into EV charging systems: the explosive growth of EV’s, the need to mitigate the environmental effects of greenhouse gases (GHGs), and the ongoing decline in the price of PV panels (Noussan and Jarre, 2021; Zhang et al., 2019). The number of charging stations is predicted to be a 7.3 million global rise by the end of 2019, which is 38% more than the previous year due to the explosive growth of the EV market. The majority of new installations of around 2 million last year, or over 86% of the increase, are attributed to new private slow charging points (Khan et al., 2023). Utilizing photovoltaic power to recharge EV batteries is of great interest because it enhances the environmental benefits of EVs. Energizing EVs with PV power during daylight hours enhances their low greenhouse gas emissions while driving, as PV electricity has minimal emissions throughout its lifespan (Zhao et al., 2023).
The numerous charging solution types that are currently being tested under actual operating conditions are discussed, along with recent installations in various nations. With the first charging unit, i.e., Beam-branded EV ARCTM 2020, Beam Global and San Diego, United States, are collaborating in the provision of a free-of-cost EV charging service to the public. For businesses, government buildings, and municipalities that require EV charging, EV ARCTM provides a transportable and standalone charging infrastructure that is entirely driven by PV energy, as seen in Figure 2 (Wu et al., 2023b). Table 1 provides an overview of the main specifications of the EV ARCTM 2020 (Sivaraman and Sharmeela, 2021).
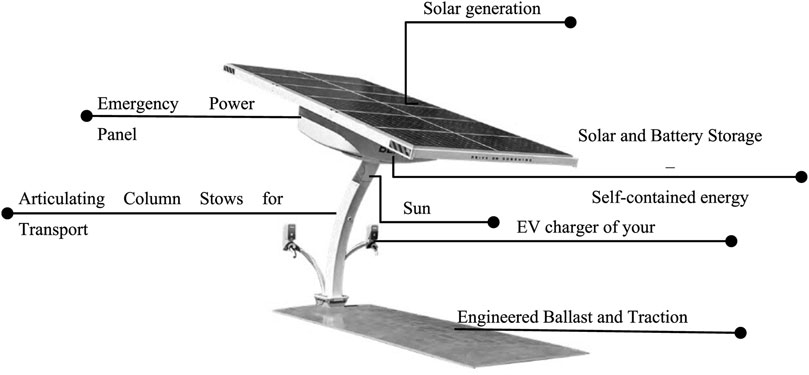
Figure 2. ARC EV charging setup (Wu et al., 2023b).
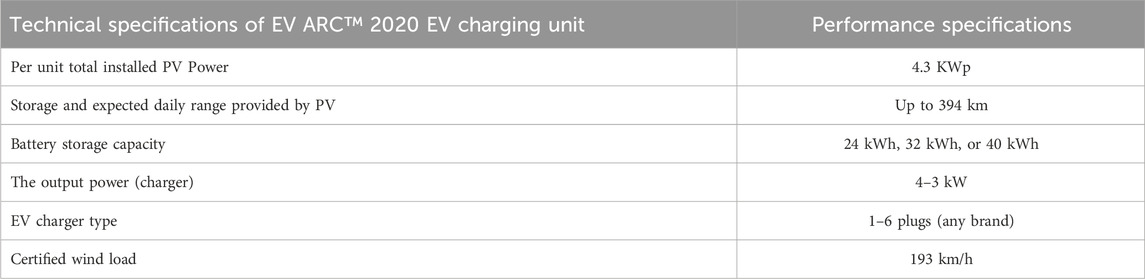
Table 1. Technical specifications of ARC EV charging setup (Wu et al., 2023b).
In Table 2, a few more instances of current PV-CS infrastructure for small or private parking lots with basic specifications are shown. The following Figure 3 shows the well-known approach for the 3-phase power system with an EV charging system.
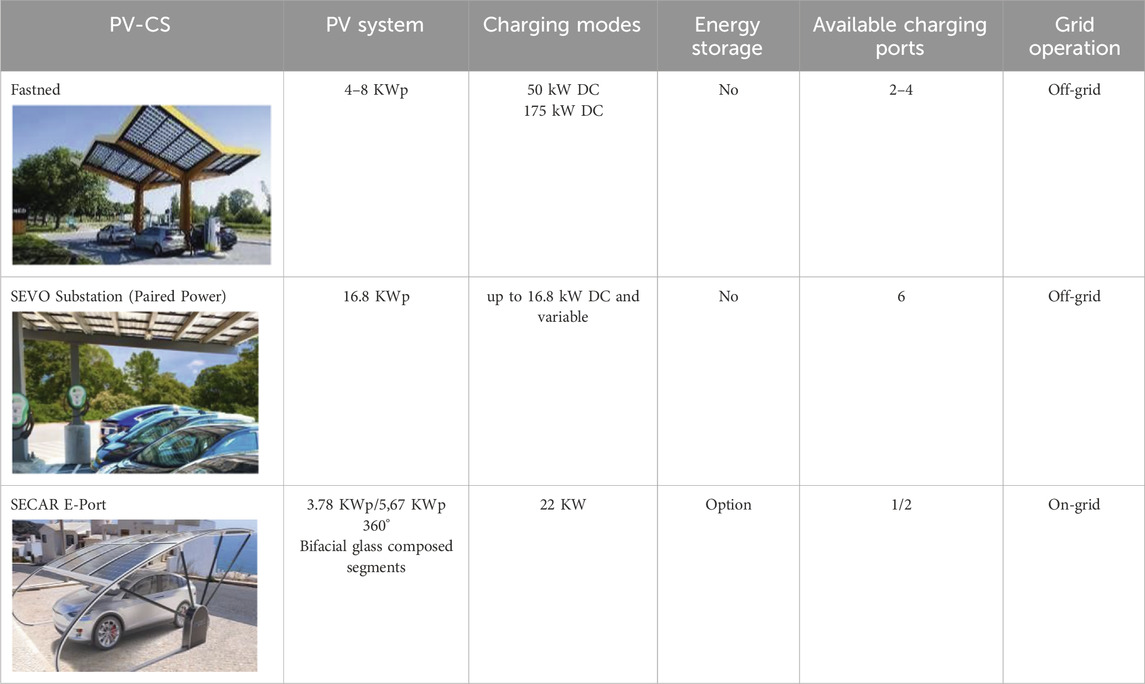
Table 2. Exemplary PV-CS stations (Pilotti et al., 2023; Rehman A. U. et al., 2023; Cheikh-Mohamad et al., 2021; Sechilariu et al., 2021; Jang et al., 2021).
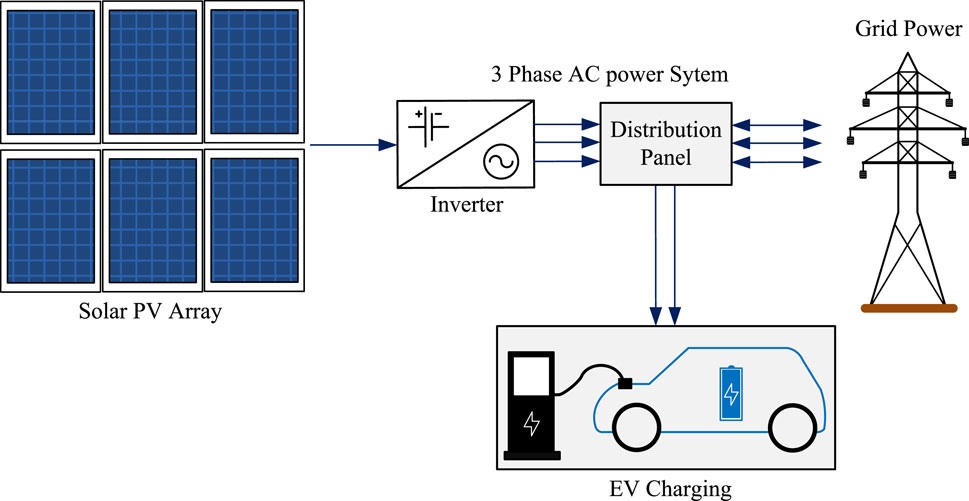
Figure 3. Typical Solar power system with EV charging system (Sivaraman and Sharmeela, 2021).
A car parking shade based on second-life EV batteries is being used in a PV self-consumption pilot project by the French retailer GÉMO. In October 2019, this pilot project got underway in Trignac, Loire-Atlantique. The 306 m2 car parking shade is made up of 185 photovoltaic panels, each of which can generate approximately 47 MWh annually, or 10 households’ worth of electricity. The assigning of a photovoltaic plant based on a public airport at La Tontouta was approved by the New Caledonian government in February 2020. This choice was made in response to a government call for innovative photovoltaic power generation projects. An entire parking lot at Saint Aignan de Grandlieu in Loire-Atlantique will be covered by a 9.8 MWp PV power plant that Urbasolar inaugurated in February 2020 and the transport-focused Charles André Group. With a surface area of 53,000 m2 and more than 32,000 PV panels, the parking shades will generate 10,070 MWh annually (Linru et al., 2020; Martínez-Lao et al., 2017; Mohamed et al., 2022).
Moreover, the ASSYCE Asia-constructed Robinson Chonburi PV rooftop plant, which was finished in 2018, is the third rooftop PV plant designed for a Robinson shopping Centre. With 999.3 kW of installed PV power, it is situated in the province of Chonburi. The majority of the PV manufacturing in this installation is consumed to power the shopping mall, and there is only one EV charging station available for customers. Additionally, the first self-consumption PV system was commissioned in Madagascar by Cap Sud Madagascar in October 2018, a subsidiary of the Cap Sud Group. A PV power plant with 410 kW and 1.1 MWh of battery storage is part of the Kube project. Table 2 offers a concise overview of the various infrastructures discussed previously (Acharige et al., 2022; Fachrizal et al., 2022; Kong et al., 2018; Bayram et al., 2013; Khan et al., 2024).
2.4 EV charging stations and interfaces
PV-powered EV Local energy storage charging station’s system configuration and the flowchart of the charging algorithm of the EV feasibility model are shown in Figure 4 (Niccolai et al., 2021; Saleh et al., 2021).
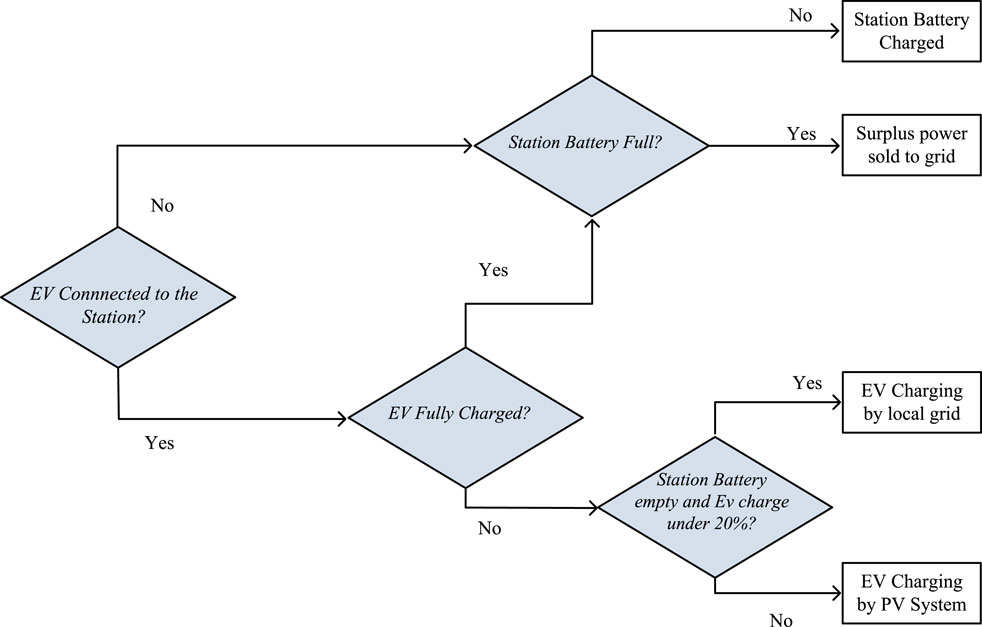
Figure 4. Algorithm for EV charging (Jang et al., 2021; Niccolai et al., 2021).
This section provides a summary of the advancements regarding various services concerning electric vehicles EVs can offer to other systems (Jin et al., 2022; Singh et al., 2022; Savari et al., 2023; Willrett, 2017) such as.
2.4.1 Level 1 charging
This is a basic type of EV charging that uses regular 120-V outlets. It is widely investigated because of its accessibility and convenience of use; yet, it gives the slowest charging speed.
2.4.2 Level 2 charging
This level chargers use 240-V outlets and charge at a significantly faster rate than Level 1 chargers. They are widely used in both domestic and business settings, and they are a focus of research on enhancing charging efficiency and ease.
2.4.3 DC fast charging
This type of charging utilizes DC to give rapid charging capabilities. The research is focused on optimizing the technology and combining it with various vehicle types and charging interfaces such as CHAdeMO, CCS, and Tesla’s proprietary systems.
2.4.4 Vehicle to grid
The technology enables an EV to be tethered to a terminal for recharging Figure 5. In a unidirectional model, electricity conducts from the grid to the EV, and is regarded as an electricity consumer. On the other hand, technologies are referred to as V2G when an EV provides the power grid with a flexible service in accordance with the demands of the electricity network (bidirectional model). V2G refers to feeding electricity from cells of the electric vehicle back into the public grid. In contrast, the car is stationary and parked. To accomplish this, the batteries in an EV fleet function as energy storage devices while the EV communicates with the intelligent power grid. Bidirectional charging stations are necessary for V2G services. The three primary services that have been researched, put into practice, and tested are voltage support, regulating the frequency of the national grid, and mitigating electrical consumption at its peak (Onishi et al., 2020a; Jaman et al., 2022).
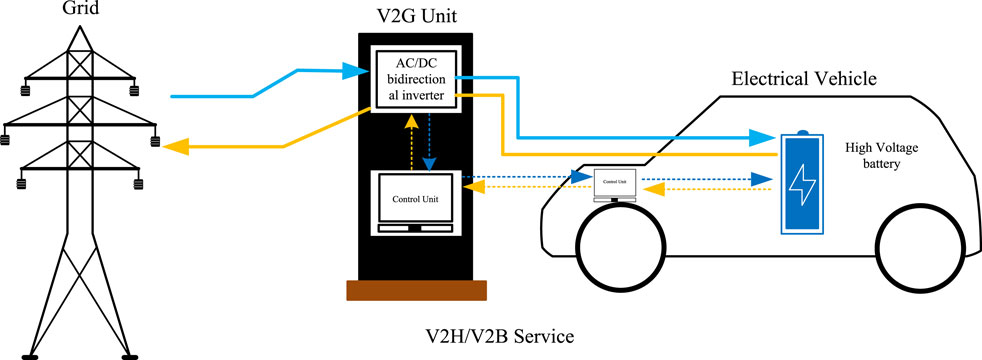
Figure 5. Structure of V2G service (Jaman et al., 2022).
2.4.4.1 Salient features of state-of-the-art V2G charging stations
The limited technological details we have managed to gather pertain to a bidirectional charging station that facilitates the 10-kW power discharge and recharge of EVs Figure 6 and Table 3. This system is advertised by Enel (Arya et al., 2024), the primary electricity provider in Italy, and is said to be able to offer services like supply of reserve power in the event of a power outage, network balancing, and consumption smoothing (Zentani et al., 2024).
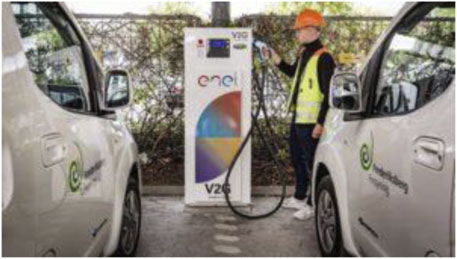
Figure 6. Example of a Fasto V2G charging station (Zentani et al., 2024).
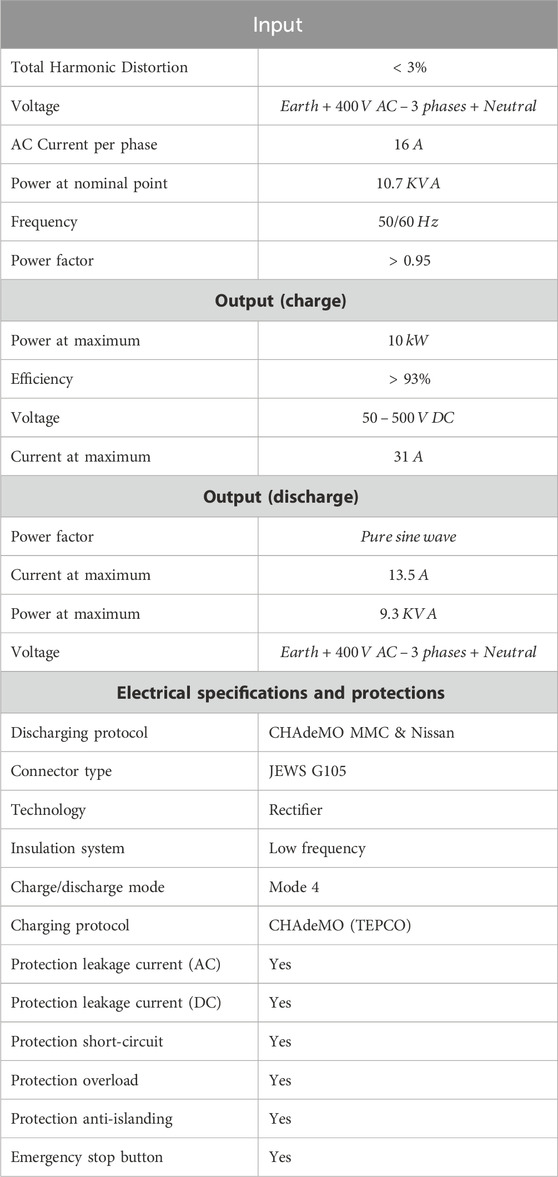
Table 3. Features of V2G charging station (Zentani et al., 2024).
2.4.4.2 Integration of V2G with DC microgrid structure
An MG is crucial to the implementation of the smart grid because it allows for the coordinated control of various loads and power sources, public grid synchronization, and satisfaction of user demands for power quality, power reliability, and safety. Because there are no negative or zero sequence currents, an MG based on a DC common bus is taken into consideration for its superior current control. The DC-MG is the foundation of the proposed design of the EV charging station with V2G. The DC-MG is made up of four components the DC-MG power flow. The public grid (PG), the storage system, and EV for electric vehicles. The arrow indicates the priority of power in real time. PV sources, for instance, support EVs first, charge storage, and then inject energy into the PG (Khan et al., 2018).
The flow of energy can be separated into two scenarios based on the “peak” and “valley” times of the grid in public. Electricity from sources of PV is initially used to charge EVs during the “valley” periods of the national grid. In addition to being the second-best energy source for EV charging, storage is also utilized to absorb excess energy generated by PV sources. The public grid, which can purchase excess energy from PV sources, is the third-best energy source for EV charging. Under typical circumstances, the 400 PV panels (40 in parallel and 10 in series) that make up the DC-MG system have a power of 100 kW. The capacity and voltage for storage are 300 Ah and 300 V, respectively. The public grid is a three-phase, low-voltage network operating at a frequency of 50 Hz. In EVs, lithium-ion batteries are charged using constant current/constant voltage, and they are discharged using constant power. To guarantee the authenticity of the simulation, an assumption was made that every EV has identical battery properties. On 28 May 2019, in Compiegne, France, from 8:00 to 22:00 h, the PV cell temperature and absolute solar irradiance are displayed in Figure 7.
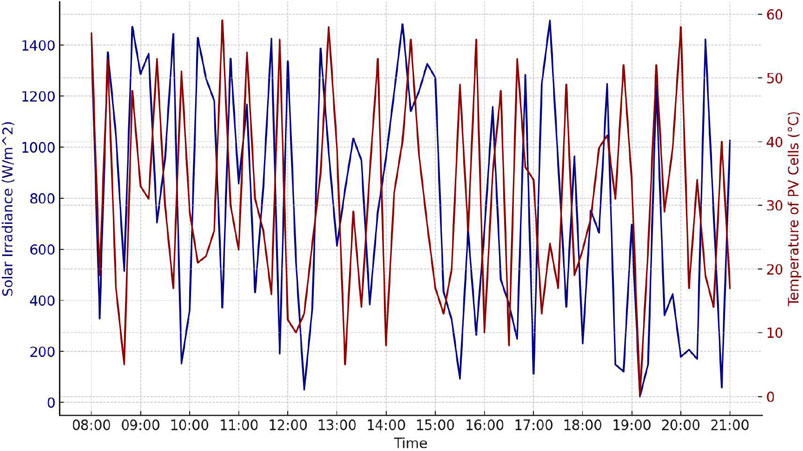
Figure 7. Solar irradiance and PV cell temperature (Rehman A. U. et al., 2023).
2.4.4.3 Drawbacks and technological risks of V2G
Some risks and drawbacks that are involved for V2G services are discussed below (Khan et al., 2018).
2.4.4.3.1 Battery life
Batteries typically have a 1000–1500 cycle life. Depending on how the EV is used, this is roughly equal to 200000–500000 km. The discharge depth, temperature, and other environmental factors, in addition to the charge and discharge currents used, will all affect how long a battery lasts.
2.4.4.3.2 Additional investment and maintenance costs
The desire for improved bidirectional chargers raises the initial investment cost. Furthermore, maintaining these complex systems necessitates constant attention, which contributes to higher operating expenses. The intricacy of V2G technology raises the danger of operational failure, necessitating specialist staff for effective management and repair. The basic, unidirectional EV charging system will be less expensive.
2.4.4.3.3 Changing the batteries
As long as the system was created with this in mind, they can be readily switched out. If not, this kind of adjustment may prove to be difficult and expensive.
2.4.4.3.4 Implementation complexity
Implementing an approach that lowers the cost of owning and recharging cars without the need for user intervention will inevitably involve more complexity.
2.4.4.3.5 Fragmented market
V2G-base service users must be prepared to pay for power from sources that have the flexibility to be connected or disconnected from their network at any time, if EV users maintain some degree of control. The total unpredictability decreases as the managed EV fleet grows. Services that utilize V2G technology will want to be able to call upon power reserves in the order of megawatts, which requires the presence and connectivity of hundreds of thousands of vehicles.
2.4.4.3.6 Battery recycling
According to Battery Directive 2066/66/EC, Manufacturers of batteries and accumulators, as well as other product producers that are composed of accumulators or batteries, are accountable for managing unusable batteries and accumulators that they have supplied to the market.
2.4.5 Vehicle-to-building or vehicle-to-home
The V2H variations are shown in Figure 8. In V2B, a group of electric vehicles power commercial or residential buildings, and in V2H, an EV powers a home for self-consumption. The power grid does not get the electrical energy fed back like in a V2H or V2B system, in contrast to V2G systems, where a building is connected to the grid permanently. Consequently, there is no outgoing electrical meter. However, V2H and V2B allow the building or residence to maintain access to an electricity supply if the grid causes a power outage (Wang et al., 2024; Liu Z. et al., 2023; Habib et al., 2015; Sagaria et al., 2022; Shakeel and Malik, 2019).
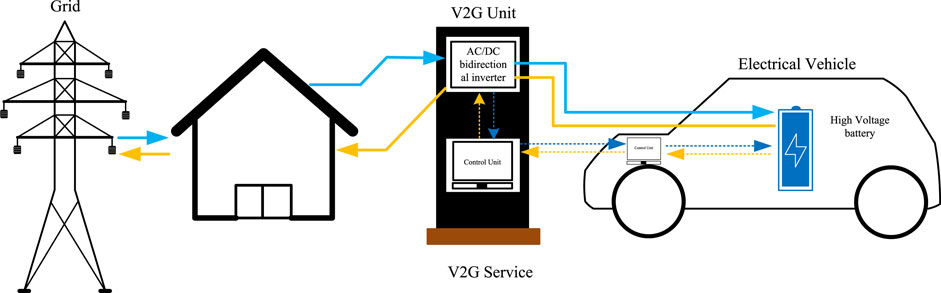
Figure 8. Structure of V2H/V2B service (Jaman et al., 2022).
3 Case study of real-world application
The PV-powered EV charging station at SAP Labs Mougins, France, based on actual data. The study focuses on power management software and parking time management in relation to EV charging at a workplace (Sánchez et al., 2022; Gbadamosi et al., 2022; Kassem et al., 2023b; Kassem et al., 2023c; Kassem et al., 2023d).
3.1 Infrastructure of SAP Labs Mougins, France
The multinational software company SAP SE’s subsidiary SAP Labs France is interested in electro-mobility. It has started several policy schemes to promote as well as quicken the energy transition, especially with regard to electro-mobility. At the end of 2021, PV car parking shade devices were installed, and SAP Labs planned to expand its PV energy production in line with the expansion of its fleet of EVs. PV panels significantly lessen the consumption peaks caused by charging terminals. The production of site and consumption capacities are summarized in Figure 9 (Pazzaglia, 2024).
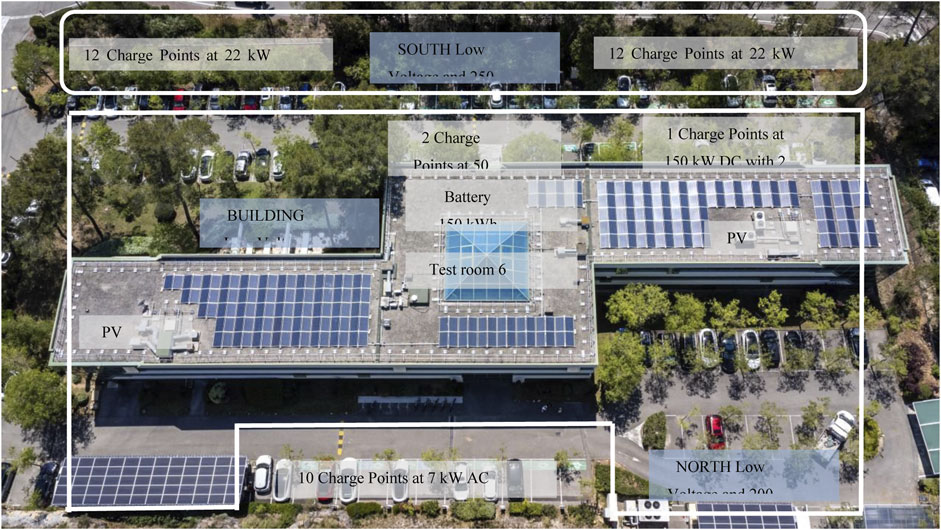
Figure 9. PV-driven setup of SAP France Labs (Kassem et al., 2023d).
An algorithm is used to control the power demand in order to guarantee cost-effective EV charging. In 2020, SAP Labs consumed 634,082 kWh of electricity, compared to 57,000 kWh of PV production. The assets, such as fast charging points that use the most significant amount of electricity are connected to the delivery point building because the PV panels were attached to it. In 2020, the energy consumption of the fast chargers amounted to 49,442 kWh, signifying 86.73% of the PV energy generated on the premises and 10.42% of the overall consumption of power of the building, as shown in Figure 10.
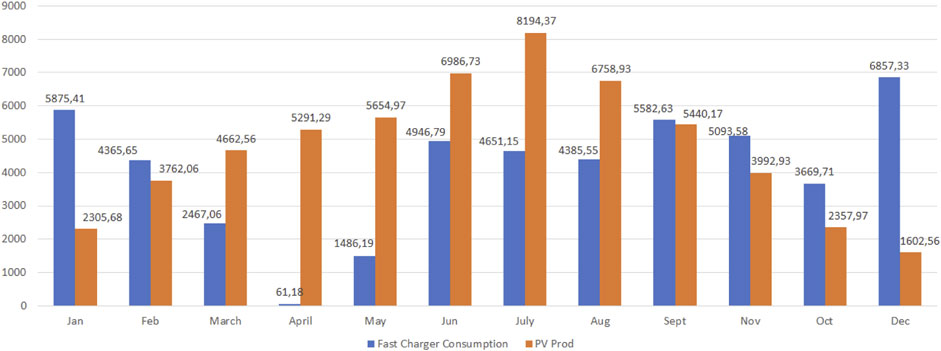
Figure 10. Production and consumption of SAP France Labs fast chargers for 2020 (Kassem et al., 2023d).
The objective of infrastructure is to store the PV panel’s generated energy on weekends to use it during Monday mornings when the energy demand is high, taking into account various optimization factors that will be discussed shortly. 1) Charging peak shaving: A method had to be found to smooth the peak energy requirements for charging, which occur between 4:00 and 5:00 p.m. on Fridays and between 8:00 and 9:00 a.m. on Mondays while still enabling a sufficiently quick charge. 2) Peak and off-peak hours for the public grid. The peak hours and off-peaks set by the electricity provider can also be taken into consideration by the e-Mobility smart grid solution: In order to maximize the amount of grid capacity that is available, charging time can be planned by e-mobility based on the convenience of off-peak hours. 3) Flexibility of the energy provider: The electricity provider in SAP Labs France is actively involved in assessing the flexibility offered by stationary storage to the grid.
3.2 e-Mobility software for PV-CS supervision
A software program called e-Mobility is used to oversee EV charging infrastructure. It makes it possible to distribute and manage the charging infrastructures more effectively as shown in Supplementary Table SA3. Summarized details of PV-driven infrastructures for EV. Figure 11 presents how user 1’s charging power.
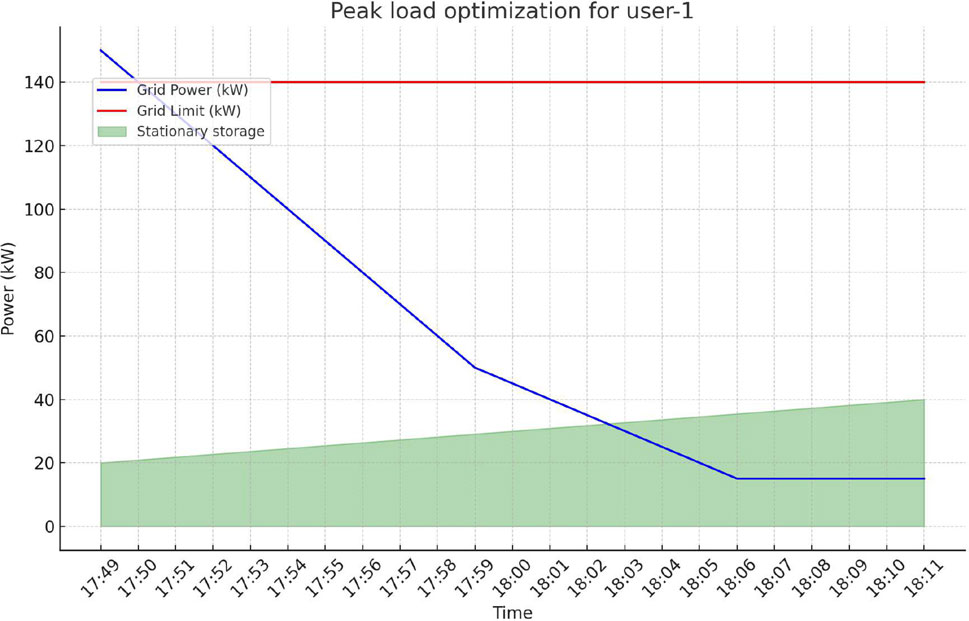
Figure 11. Peak load optimization for user-1 (Wu et al., 2023b).
Figure 12 presents how user 2’s charging power is instantly capped at 75 kW. Essentially, this is to safeguard the electrical grid. A more significant infrastructure investment in electricity would be required in the absence of this protection.
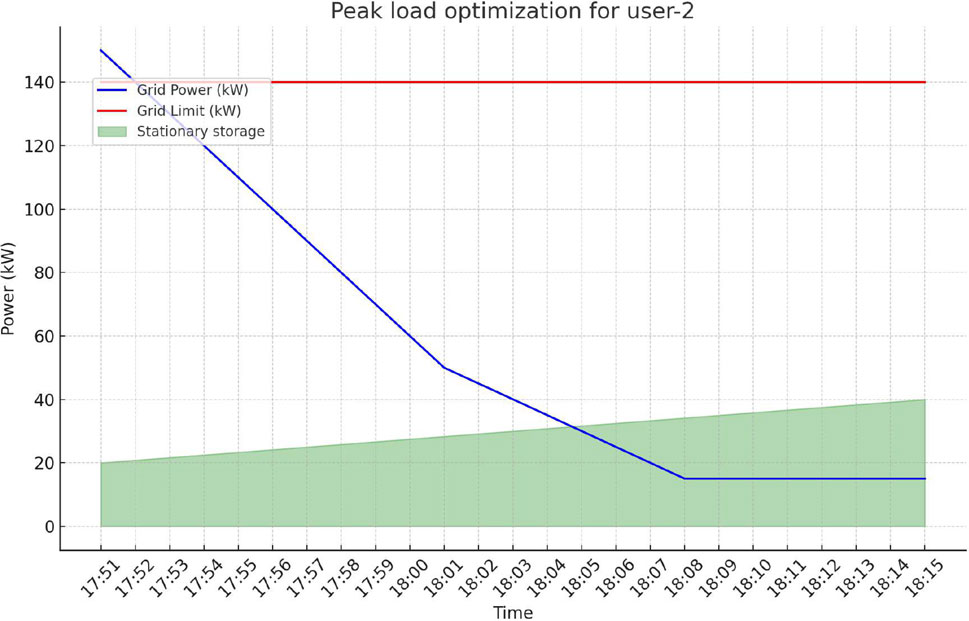
Figure 12. Peak load optimization for user-2 (Wu et al., 2023b).
The findings of an economic analysis conducted over 10 years at each location for 5 KWh storage-based PV charging systems are displayed in Figure 13. Grid-only charging has the highest net present value (NPV) across all locations, which is probably because the storage system has high investment costs. Systems lacking storage (shown by icons) exhibit a higher net present value in the 50% and 75% PV scenarios. For example, in a 50% PV + 50% Grid scenario, the present value of a Chicago charging station with a 5 kWh BESS is −1233 USD. If the PV-based energy share is kept the same and storage systems are removed, the value rises to 3 549 USD. Moreover, environmental impacts have been picturized in Figure 14.
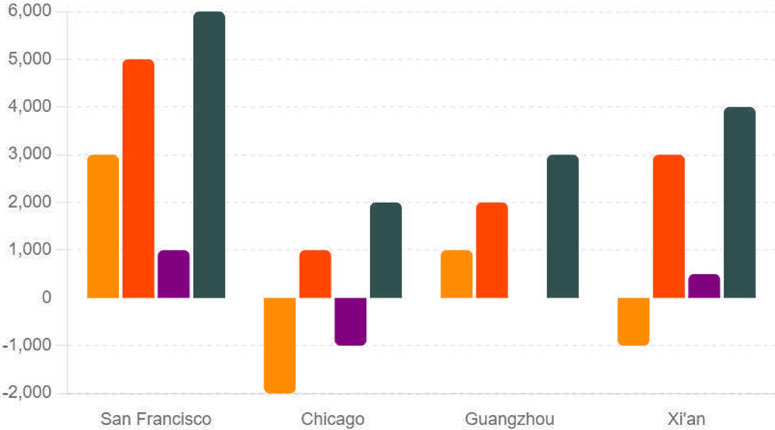
Figure 13. Economic impact statistics after 10 years for NPV (Acharige et al., 2022; Fachrizal et al., 2022; Mohamed et al., 2022).
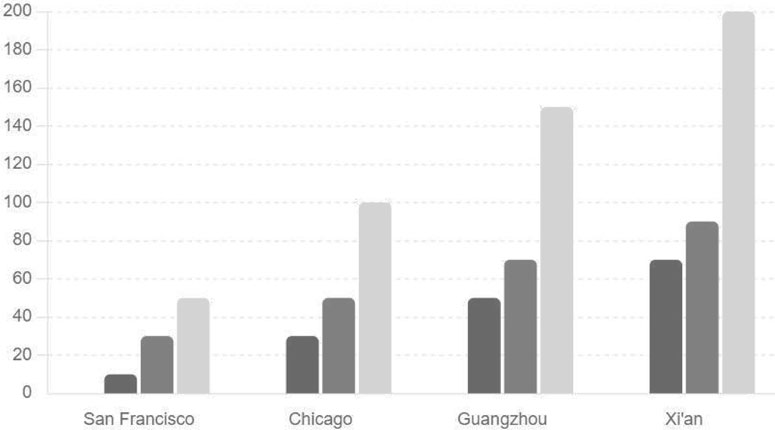
Figure 14. Environmental Impact Statistics for PV-CSs (CO2-equivalent emissions per kilometer at each of the four locations under analysis) (Fachrizal et al., 2022).
3.3 Innovative and revolutionary designs for applications for EV charging infrastructure
The design of a product is a multidisciplinary character; thus, solar applications for EV charging are studied in aspects related to technology, user interface, regulations, and aesthetics. At the University of Twente (UT), a design project was conducted, and eleven conceptual designs were created in 2019. These designs included solutions for vehicle-integrated PV (VIPV) products, vehicle-powered EV charging, and other applications. The concepts center on different forms of transportation other than private automobiles, such as electric bicycles, utility vehicles, and public transportation. In certain instances, the designs incorporate alternative charging technologies like induction charging and battery swapping. Two conceptual designs are showcased as case studies out of these. The Innovation Flower shown in Figure 15 depicts the five elements of a successful PV-CS.
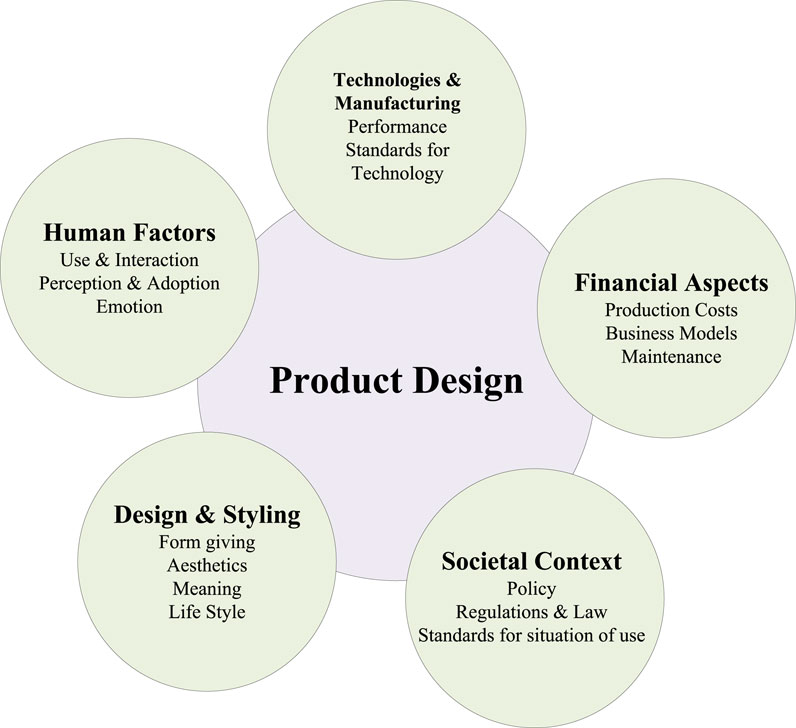
Figure 15. Elements of a successful PV-CS design (Cheikh-Mohamad et al., 2021; Sechilariu et al., 2021).
The PV materials and manufacturing processes utilized to produce PV cells and modules are covered under the “Technologies and Manufacturing” section. An essential part of this process is the electric and electronic machinery used to store, distribute, monitor, and convert solar energy. “Financial Aspects” covers the cost of purchasing solar panels and associated photovoltaic products as well as the economic worth of energy producers. The “Social Context” is crucial to the adoption and realization of PV systems in society. Societal aspects usually include laws, policies, rules, and standards. Nonetheless, the perception of the public with respect to sustainability and their readiness to adopt PV technologies are also significant factors. The use of PV systems is covered by the Human Factors aspect; this is particularly significant for product-integrated PV and systems in the built environment, where user appreciation will be determined by features such as usability, performance, and visibility (Sierra and Reinders, 2020). The final component, “Design & Styling,” addresses how PV technologies seem. Similarly, in the case of building-integrated PV systems, an appealing and modern look can have a significant impact on suitable PV-integrated products as well as help increase user acceptance of the systems in their environment of use. Because of the positive and understanding attitude of the user, well-designed objects typically face increased functionality and less resistance (Khalid M. et al., 2021).
In order to investigate the mobility-related evolution of PV-based applications in the near future, a conceptual design study was conducted in 2019 with students from the UT in the Netherlands studying mechanical and industrial design engineering. Under the auspices of the research project “PV in Mobility” as well as a component of the UT Master’s course “Sources of Innovation,” the design study was carried out. In order to increase the likelihood that future users would adopt solar PV-powered EV charging solutions, a conceptual product-service combination was designed by students between September and November 2019. To improve their chances of being adopted, the student teams intended to develop a concept-based design of a combination of products and services for EV charging solutions based on power. Out of eleven, eight concepts were chosen for this conceptual design, and the corresponding designs are displayed in Figure 16 (DAmbrosio et al., 2021).
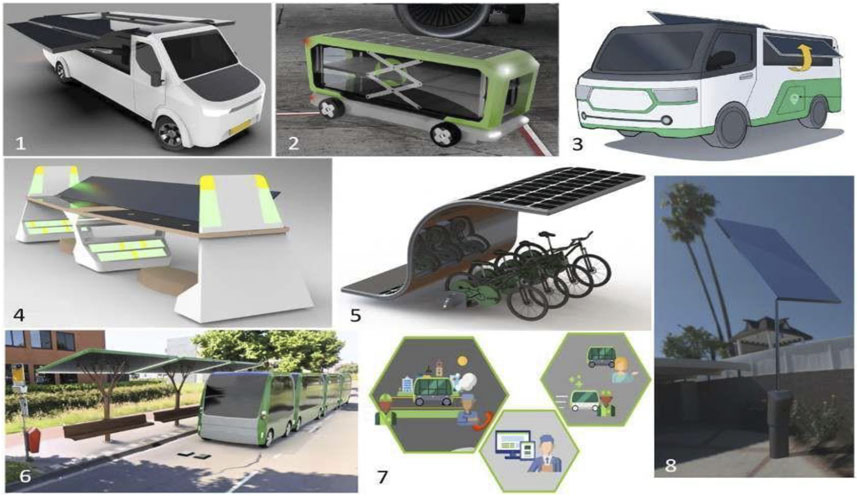
Figure 16. PV-CS design concept project (Sources of Innovation) (Pilotti et al., 2023).
The case study that follows includes an overview of two cases, namely, Solar Train Stop and Solar Bus Terminal.
3.3.1 Solar bus terminal
This conceptual design includes an electric city bus terminal where battery packs are charged by a photovoltaic system atop the building, allowing the buses to be recharged while parked at the terminal as can be seen in Figure 17.
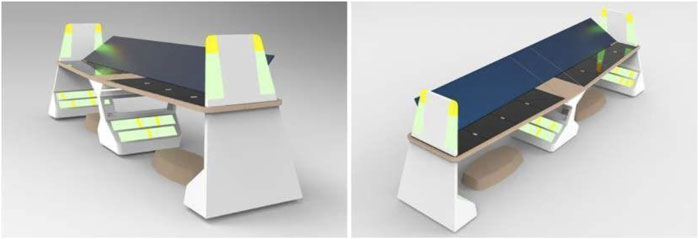
Figure 17. Concept of Solar Bus design (Pilotti et al., 2023).
LED lights can be seen throughout the bus terminal in multiple panels close to the passenger waiting area and the two pillars beside the PV system. The number of battery packs that the PV system has charged at any given time is indicated by the ratio between the two colors that the lights can display: yellow or green. A primary constraint of the suggested idea pertains to the difficulties presented by the battery replacement procedure, which necessitates drivers to step outside the bus for a brief duration. When there are high traffic volumes or bad weather, this could be an issue. Passengers might also find it inconvenient as it could mean having to wait longer. Furthermore, the brief period city buses spend stationary on their routes is probably another factor in the decision to use a change in batteries as a method for this design charging. However, a high charging speed is needed to supply enough charge during this short time, and the terminal of the PV system is unable to provide it directly. Replacing the battery packs with a centralized battery storage system which can supply the power required for high-speed charging could be one way to address this problem. One more drawback of this design is that the PV system may self-shade because of the pillars at its sides. By creating pillars that are less intrusive or eliminating them, this can be fixed. It is also possible to integrate more PV cells into other structural elements, but where they are placed will ultimately depend on the orientation and precise location of each terminal. This solar bus terminal concept can also be modified for other similar uses, like charging electric bicycles or passenger EVs in the form of a structure that offers seating areas to people in parks or squares, as illustrated in Figure 18.
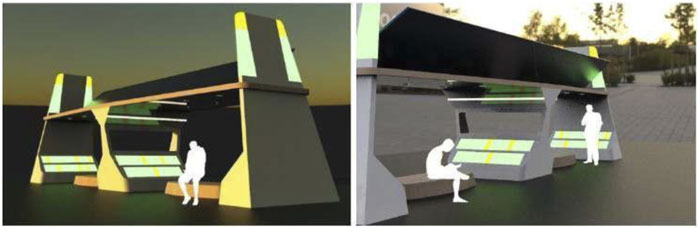
Figure 18. Concept of Solar Bus passenger terminal design (Bayram et al., 2013).
3.3.2 Solar train terminal
Here, a street bench and a small photovoltaic system are combined to construct a stop for city trains that offer users cover and a local renewable energy source. When combined with an electric train, where the vehicle and the stop have integrated photovoltaic cells, Figure 19 illustrates how this design would function. The concept of modular design allows for flexibility in bench count based on needed PV capacity and available space.
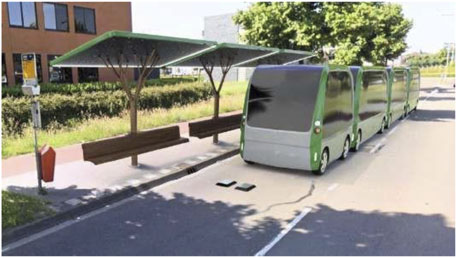
Figure 19. Concept of Solar Train Stop design (Bayram et al., 2013).
When the passengers are being picked up and dropped off, a set of charging pads on the street is used to provide a small amount of charge via induction charging in the vehicle. The idea behind the solar train stop is to make the process of charging a car as inconspicuous as possible for both the driver and the user while simultaneously offering a comfortable environment for passengers who are waiting. The blending of the urban surroundings is done by giving users a more pleasant and environmentally friendly experience; the visual design of the station mimics the shape of a tree. The station must have a distinctive look in order to be identified as a component of a sustainable transportation network. While passengers board or disembark, only for a few seconds during this operation the solar train will only be in front of the stop. The concept creator suggested adding PV systems to neighboring buildings to boost their overall PV capacity. Still, this idea could also be expanded to create a local microgrid where the partial electric needs of the buildings are met by the surplus production from the solar train stop.
Using a simulation model that the authors have previously developed and published, the approximate share of the developed mobility concepts energy demand of design project that the integrated PV system would cover was calculated intended to assess powering concepts of PV principles empirically. In conclusion, this study resulted in the development of eleven design solutions, the majority of which addressed charging infrastructure or VIPV systems. Additionally, designs were made for a service-only option and a product-service combo targeted at one of the participating companies of the project. The concepts that were developed centered on a variety of vehicle types, including shared, private, and public ownership electric bicycles, buses, and passenger cars.
Overall, the study developed diverse design solutions focusing on charging infrastructure and VIPV systems, targeting different vehicle types and ownership models. This project aimed to increase the adoption of solar PV-powered EV charging solutions, contributing to sustainable and efficient transportation systems.
4 Electric vehicles international standards and charging protocols
In pursuit of competent service provisions, the EV charging stations must adhere to international standards and protocols. Various societies and technical bodies such as the Society of Automotive Engineers (SAE), Institute of Electrical and Electronics Engineer (IEEE), and the Infrastructure Working Council (IWC) focus on developing regulations for consumer interfaces and utilities standards (Das et al., 2020b; Das Himadry et al., 2020; Shah et al., 2023; Das et al., 2020c; Habib et al., 2018). A basic overview of sections of charging infrastructure is given in Figure 20. The charging infrastructure has various aspects to be reviewed in order to become competent and feasible and have been discussed below.
4.1 Safety standards, protocols, and best practices for charging infrastructure
The wider acceptance of EVs has various challenges to face and overcome, such as battery life cycles, charger-related issues, increased costs and a lack of charging infrastructure. Another aspect is harmonics generation that affects conduction efficiency. As can be seen in Table 4, power level summary is shown. Level 1 chargers are for domestic use, whereas Level 2 chargers are suitable for both public and domestic use. Level 3 chargers are designed for three-phase solutions, and are designed for commercial as well as public use (Wang et al., 2021; Rajendran et al., 2021).
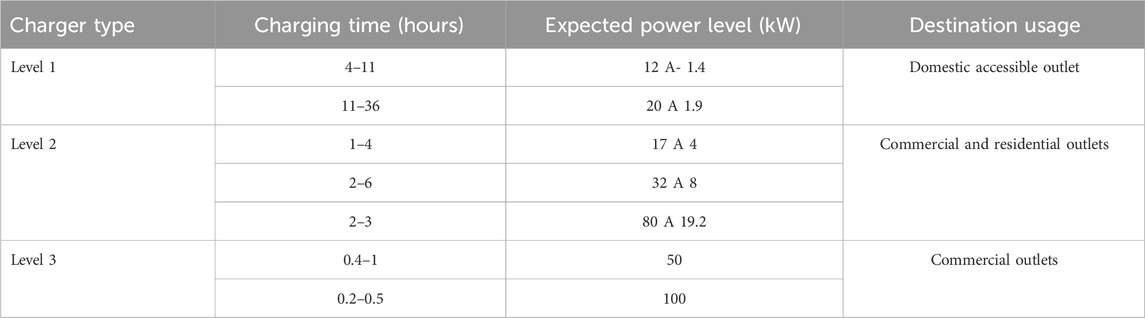
Table 4. Charger Power Level descriptions (Wang et al., 2021).
EV battery chargers both on-board and off-board can be categorized into unidirectional and bidirectional power flow. Unidirectional power flow chargers involve simplified hardware. This benefits in resolving connectivity issues and decreased battery deteriorations. The bidirectional charging system has stabilized power conversion, charging from the grid and reverse battery energy flow to the grid. The charging protocols and rules are essential for an effective EV infrastructure operation. Various international organizations are defining energy management rules for EV infrastructures (Alrubaie et al., 2023). The charging codes and the details can be seen in Table 5.
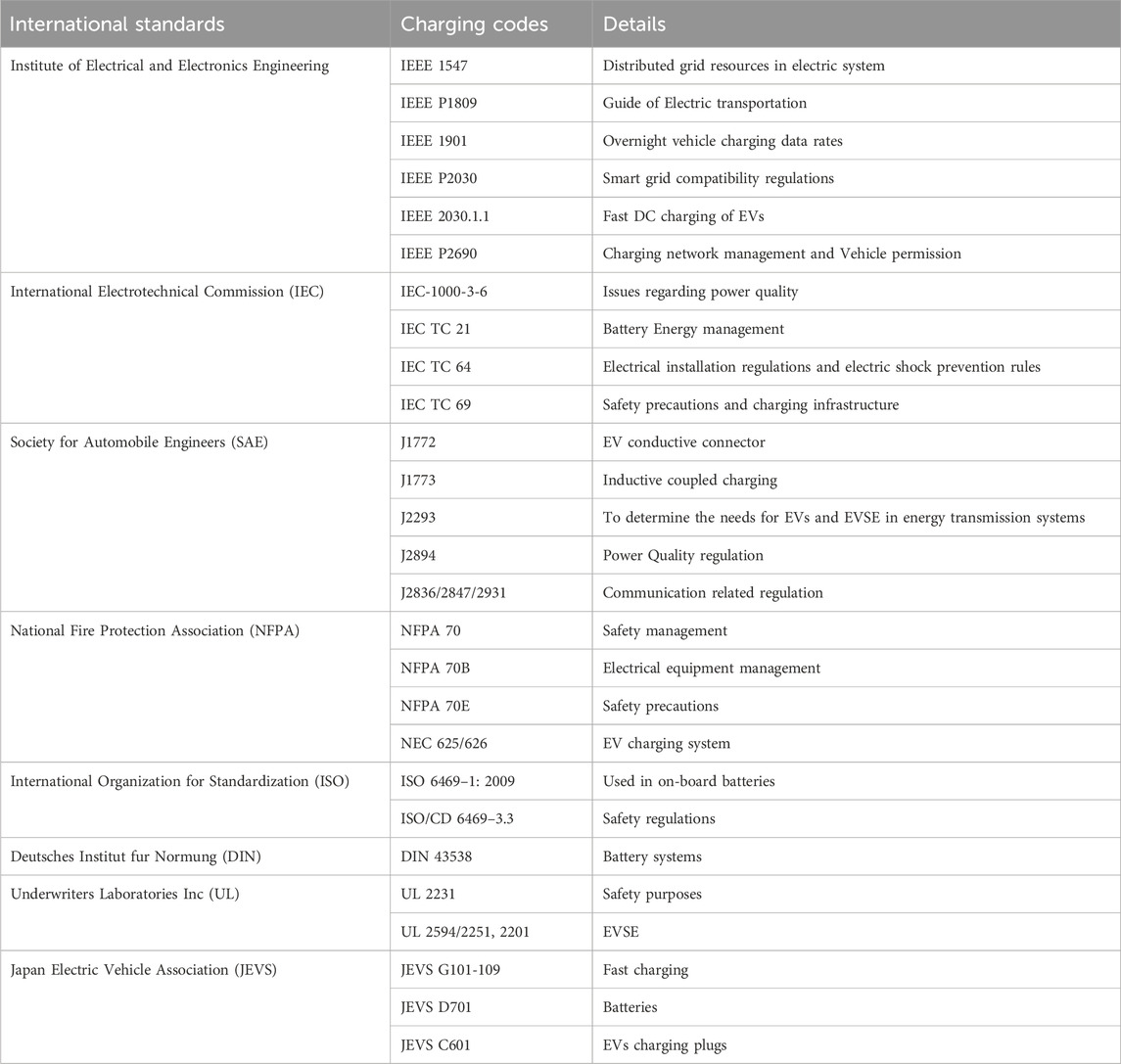
Table 5. International standards and charging codes (Alrubaie et al., 2023).
4.2 Protocol for communication between charging units and electrical vehicles
In a public charging unit, in order to successfully manage a significant EV number, effective communication within the network is crucial to ensure grid stability and efficiently managed energy flow. The built-in capabilities of EVs to monitor metering, energy management and bi-directional power flow will determine their acceptability in the forthcoming years (Khalid M. R. et al., 2021).
4.3 Communication between V2G
The V2G perception is defined by technological feasibility, and PEV user behavior and expectations. Bi-directional communication protocol enables customer allocations, manages EV tasks, and even reroutes. A plug-in hybrid EV (PHEV) possessing V2G technology has capability to regulate energy between self-usage and power grid as per need, though an intelligent bidirectional flow (Pilotti et al., 2023). This bidirectional frow requires security protocols, and the V2G is limited due to security concerns of communication. Intelligent control integration will allow direct coordination of charging/discharging. The smart grid system offers many benefits, if mindfully constructed. As a utility’s aspect, the grid’s power quality increases as it is integrated with smart electronic technologies, as well as achieve easier grid monitoring. The capability of smart grid to manage renewable resources is a vital sustainable achievement, and is impactful to affect the renewable market in a supportive manner. The transformation of grid into intelligent communicative system smart metering and charge/discharge events can be coordinated. Enhance grid operators return has been done to evaluate real time power for charging and discharging. New opportunities are foreseen in urban administration as sensors are induced in communications to enhance urban dispatch methods. Effective power management in grid can also be possible if aided by the traffic data, GPS indications end energy consumptions though smart meters. The commination established by the transmission system operator (TSO), and the distribution system operator (DSO) should be established via a smart metering system. This will result in effective implementation of V2G, PHEV integration with renewable sources and turning PHEV in a controlled load (Dharmakeerthi et al., 2011).
4.4 A charge strategy based on tokens (T-Charge)
It is probable for an EV to be plugged in but not receive charge for a while. A token-based pricing model according to reserved tokens that were put out for network. Tactive, that concerns token holding time and a scheduler, indicates maximum duration for a socket to be active. After the token reserved time period, the socket turns inactive, the token is sent for subsequent inactive socket for an unserved EV to be connected (Fathabadi, 2020).
4.5 Communication between utility and EV
For EVs to be more acceptable, appropriate communication and charging controls are crucial (Sivaraman and Sharmeela, 2021; Wu et al., 2023b).
The Advanced Metering Infrastructure (AMI) framework uses various form of communications such as wireless and power line communication (PLC) and broadband over power lines (BPL) to incorporate a bidirectional communication link between the smart meters, computer network infrastructure, various sensors, utility network, and the EV management system (EVMS).
ZigBee is a viable option to support communications and require less bandwidth. The notable problems to be addressed would be limited memory, delays, and interfaces from other devices. Fast charging stations for EVs and PHEVs have studied and employed a cosine firing scheme-based voltage regulator and electronic tap changer to rectify fluctuations in input supply and contribute to sustainable development and energy availability (Habib et al., 2017).
The EV networks must be secure against cyberattacks such as malicious software that alters prices or scams the system. Additionally, it is important to offer protected EV services to the visiting networks. The cost of the home charging case appears to grow due to the SMs and communication infrastructure, favoring the PV-based workplace charging station (Almasri et al., 2024).
4.6 Communication between EV and charging station
The energy management infrastructures are composite of various elements such as outlets, charging station safeguard and charging cables are part of EV-CS, designed to deliver power to PV, and hence affects the communication with the EV and the charging modes. Communication with the Power Source. It monitors the charging station and makes transactions with it. A data network will be necessary for communication; any of the available technologies, such as GPRS/3G, Wi-Fi, etc., may be employed. Connect with the User. It notifies and reports the user of the charging status through their mobile device, smartphone, or the Internet. Having conversations with the electricity distributor provides information to the energy distributor on the state of the power supply. Interacting with the Help Desk. The help desk receives information about the charging system’s condition from it. By delivering information on customers who are utilizing the network of another charging management system (CMS) with which they are not enrolled, it maintains the accessibility of the whole network of charging stations, even if they belong to different CMS (Chandra et al., 2024).
The charging station and EV communication is executed via ISO/IEC 15118 protocol stack. This communication is assisted by predefined parameters and messages. These parameters include tariff tables, charge power, voltage and current, and the desired initiation or end of the charging procedure. If the communication is not up to the required standard, the charging may not begin or may be put to halt. This protocol system is used to evaluate communication and exhibit compatibility (Singh et al., 2022). Without the grid to EV communication, local parameters such as EV departure time and voltage magnitude can be employed to regulate EV charging process. The EV user can communicate on board with the EV charger to convey the departure time. Based upon the required time and charging energy, charging power rating of the EV can be reduced. The off-board charging option also enables features such as over-protection, fault current and circuit braking (Nawaz et al., 2024).
4.7 Communication with chargers
Communication with Chargers are also known as Electric vehicle supply equipment (EVSE) for the preservation of state of health of batteries, EVSE must charge at an optimum rate, which is possible after establishing communication with the Battery management system. The communication at the central management system at the power utility company and EVSE allows maximum c-rate regulation based on grid supply rates, and affect rate metering. This is important for grid readiness to supply huge current upon demand, and affects charger reservations by customers. The public off-board chargers utilize open charge point protocol (OCPP) communication protocol, communicable over media through internet (Ghasemi-Marzbali, 2022).
4.8 Communication with EV and EVSE
The communication between EV charging station and EV should follow IEC61851-24B. The foundation for application layer is GB/T 27930 protocol. According to GB/T 27930, Vehicle Identification Number (VIN) parameters is necessary. The communication interface between EVs and EVSE is the basic preference of the ISO/IEC joint initiative on the Vehicle to Grid Communication Interface (V2G CI) (Yu et al., 2024).
5 Future photovoltaic charging station design challenges
The essential step before installing a PV setup for charging EVs is to research the social acceptability of infrastructure and its impact on society, as well as the new services it will bring with it. The PV-based EV charging infrastructure has impacted society and how it is being accepted, as well as a new service that will be offered in conjunction with it that will benefit users outside of urban areas and early adopters (John et al., 2024). The integration of solar energy systems with EV charging infrastructure holds significant promise for addressing carbon emissions, enhancing energy security, and fostering sustainable transportation. However, this integration faces several key challenges that need to be addressed for its successful implementation. Designing and installing solar PV systems for EV charging stations is complex and requires optimization for maximum efficiency. Selecting suitable sites for solar panels involves considering sun exposure and physical space, which presents a significant challenge. Maximizing the efficiency of solar panels to generate enough energy for EV charging is essential. Integrating solar energy with the existing power grid requires managing the variability of solar generation and EV charging patterns, necessitating advanced energy management strategies (Cevik and Ninomiya, 2023).
The high initial cost of setting up solar-powered EV charging infrastructure can be prohibitive. Securing financing for these projects is challenging due to the substantial upfront costs and extended payback periods. Developing sustainable and profitable revenue models is crucial for the long-term viability of this infrastructure. Despite decreasing costs of solar PV technology, significant economic barriers still hinder widespread adoption. Establishing interconnection standards for solar-powered EV charging systems is essential for grid integration. Navigating utility regulations affecting deployment and operation can be complex. Streamlining the permitting process is crucial to avoid delays and reduce deployment complexity. Developing clear and supportive regulatory frameworks is necessary to facilitate the growth of solar-powered EV charging infrastructure. In this regard, potential aspects of future challenges are listed below (Pareek et al., 2020; Chandra Mouli et al., 2016).
5.1 Integration and acceptance of photovoltaic systems in protected landscapes
PV systems have the potential to redefine architectural and urban design approaches, owing to their innovative application in module design and integration in both new and retrofitted buildings, urban areas, energy communities, and landscapes. The benefits for this technology are notably associated with energy savings, climate change mitigation, reduction in electricity prices, and economic growth. Advancements in panel aesthetics foster a seamless blend of visual appeal, technological progress, and efficient energy production. These systems offer several advantages, including innovative architectural design and multi-functionality (Kassem et al., 2023e; Onishi et al., 2020b).
Despite these advantages, the application of PV systems remains controversial in buildings, cities, and landscapes. These concepts are related also to social preference that refers to the collective inclination or bias of a community towards the PV systems, based on perceived benefits, cultural inclinations, or societal priorities. The shift from buildings to landscapes PV integration improve the degree of design complexity, involving urban planning, architecture design, energy planning, heritage conservation, and natural protection. Thus, the scope of this sector was to investigate the openness to PV technology and willingness to their adoption in landscapes protected for their heritage and natural values. The first question refers to the acceptance of PV integrated in protected landscapes. PV systems in protected landscapes are high 90%, composed by complete (46.5%), and partial (43.5%) acceptance. As previously for integrated PV, partial acceptance refers to the need of a tailored PV design. Only few respondents consider this technology not suitable (7.0%) or have some doubts for its application (3.0%). The results are in line with the previous analysis about PV systems integrated in historic buildings, with small differences on the ranges of total and partial acceptance (Kotarela et al., 2024).
5.2 Challenges of energy management systems in PV charging station
EV owners recharge the batteries from the residential connection, which presents a considerable system loss in the power sector and leads to a decreased profitability index. Additionally, many EV chargers present power quality problems connected to the distribution grid, such as voltage fluctuations, harmonics, and power loss owing to their non-linear behavior. These power quality problems within the distribution network are attributed to uncoordinated and inefficient EV charging schemes. These can be solved by redesigning charging patterns, improving converter topologies, integrating renewable resources, and utilizing energy management approaches. Utilization of the available renewable resources is considered the best approach from techno-economic and environmental perspectives. It also reduces the pressure on the utility grid, improving power quality. Energy management schemes enable maximum utilization of renewable energy with the lowest possible charging cost. The optimization of the proposed hybrid renewable energy-based EVCS can be achieved using other algorithms, such as particle swarm optimization (PSO), the genetic algorithm (GA), and the GA-PSO algorithm, for better results. A new scheme can be designed as a bidirectional energy transfer facility similar to a smart grid for the EVCS, known as the V2G technology. During blackout and peak hour periods, the EVs can transfer energy to the utility grid through this scheme. It contributes significantly to the sustainable development of power system networks and the transportation sector, and the proposed strategy presents various techno-economic and environmental benefits (Liu et al., 2024b; Tseng and Hsieh, 2023).
The integration of PV systems into EV charging infrastructure presents future challenges and opportunities. Addressing power quality issues and system losses requires innovative solutions like hybrid renewable energy systems, advanced energy management schemes, and optimized algorithms. As PV technology advances, integrating it with EV charging will become essential to enhance efficiency, reduce grid pressure, and promote sustainable energy use. The adoption of V2G technology further underscores the potential for PV systems to play a crucial role in the evolving energy landscape, highlighting the need for continued research and development in this area (Liu et al., 2023c).
5.3 Optimal site selection for electric vehicle solar charging stations
EV takes significantly longer to charge compared to refueling conventional fuel-based vehicles. As a result, many more charging stations will be needed to provide equally convenient services for EV users as gas stations currently do for internal combustion engine vehicle users. To support the transition to electric transportation, many governments have introduced measures such as tax reductions, combustion engine vehicle replacement schemes, and subsidies for EV purchases. Additionally, the cost of new EVs has decreased over the past decade (Rehman S. et al., 2023; Liu et al., 2023d; An, 2020b).
The selected locations for electric vehicle charging stations by presenting a novel approach using a Geographic Information System (GIS) for the site selection of EV solar charging stations. Previous research has developed GIS and Multi-Criteria Decision Making (MCDM) based methods for selecting optimal locations for solar plants or traditional grid energy-based EV charging stations separately. However, the integration of renewable energy and the optimal site selection for solar-supplied EV charging stations have received very little attention (Sagaria et al., 2022).
Before installing a PV setup for charging EVs, it is crucial to research the social acceptability of the infrastructure and its societal impacts. Examining how PV-based EV charging infrastructure is received and understanding the new services it will provide are essential steps. The integration of solar energy systems with EV charging infrastructure holds significant promise for reducing carbon emissions, enhancing energy security, and fostering sustainable transportation. However, several key challenges must be addressed for successful implementation. These include optimizing design and installation for maximum efficiency, selecting suitable sites considering sun exposure and physical space, and managing the variability of solar generation and EV charging patterns with advanced energy management strategies. High initial costs and securing financing are significant economic barriers, as substantial upfront investments and extended payback periods can be prohibitive. Establishing interconnection standards and navigating complex utility regulations are also crucial for seamless grid integration. Advanced energy management strategies and algorithms, such as PSO and GA, are necessary for optimal performance. The adoption of V2G technology presents additional opportunities for energy transfer and grid support during blackouts and peak periods. Addressing these challenges and capitalizing on the potential benefits of PV systems in EV charging infrastructure require continued research and development (Cheikh-Mohamad et al., 2023).
The charging power levels, charging types, charger topologies and deployment, communication protocols, standards and infrastructure are reviewed. Battery performance of EV is affected by charging infrastructure and charger characteristics. Charging power is categorized for three levels, Level 1, Level 2 and Level 3. The unidirectional flow is on-board, is easier to tackle for hardware problems, slows battery deterioration, and has constrained power due to size, weight and price. The bidirectional flow is off-board and allows grid to battery energy flow. The EV charging infrastructures were compared based on various factors such as locations, power volume, equipment and location. Initiatives to improve power management, standardizations and infrastructure-based issue resolution are crucial for EVs acceptance (Lee et al., 2020).
5.4 PV-CS social impacts
An essential first step before installing a PV setup for charging EVs is to research the social acceptability of infrastructure and its impact on society, as well as the new services it will bring with it. The PV-based EV charging infrastructure has impacted society and how it is being accepted, as well as a new service that will be offered in conjunction with it that will benefit users outside of urban areas and early adopters. Four conceptual designs are presented as case studies; all solar based: a solar luggage vehicle, a train stop, a bus terminal, and a mobile charger (Lucchi et al., 2023).
5.5 PV-driven EV charging stations Impacts on economy and environment
Positively impacting both the economy and the environment, EV charging stations powered by PV provide several advantages. From an economic perspective, these stations lessen reliance on the power grid, which in turn lowers running costs and gives consumers and companies long-term savings. They help achieve energy independence while mitigating the effects of rising fuel costs through the use of solar electricity. Charging stations powered by photovoltaic panels lessen the environmental impact of electric vehicles by reducing pollution and greenhouse gas emissions. Electric vehicle charging stations and PV systems work together to improve grid stability and efficiency through better charge scheduling. Additionally, these stations are grid-independent, which aids transportation system sustainability and provides resilience during power outages. The simulation of the viability of onsite storage for electrical vehicles of PV charging stations in China and the United States and the findings are discussed. The energy balance serves as the basis for the calculation of environmental and economic indicators. Based on the PV array investment costs and the BESS of the station, the profit from selling electricity to the grid, and the purchases of grid electricity, the economic analysis computes an annual cash flow. The mitigation cost of GHG stations is also determined by combining the environmental and financial feasibility assessments. Figure 21 displays the kWh grid supply and hourly EV charge for each of the defined situations for systems in San Francisco. Another advantage of utilizing solar power scenarios of high share for EVs is the correlation between a lower PV share and more battery charging cycles, especially those comprising deep discharging, which is predicted to shorten the lifespan of EV batteries (Rehman A. U. et al., 2023; Kotarela et al., 2024; Rauf et al., 2023).
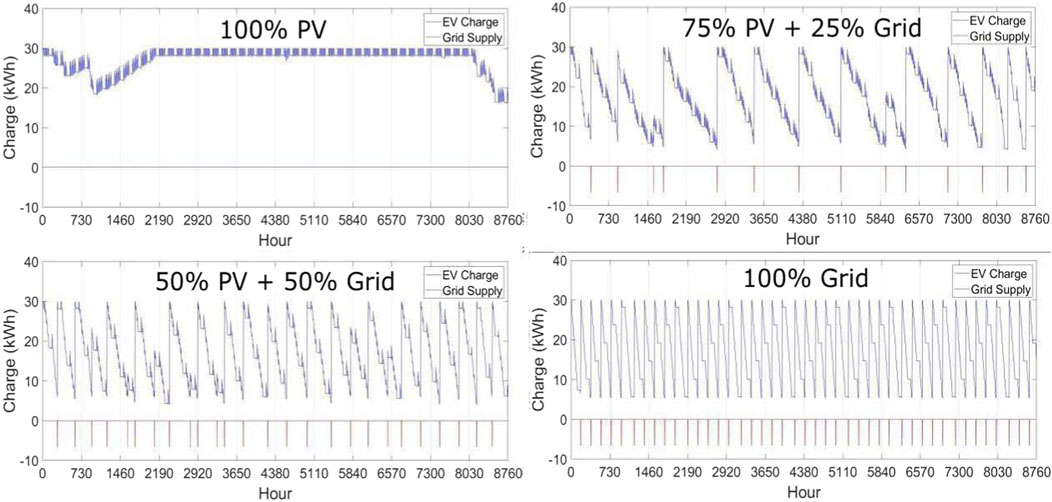
Figure 21. Hourly EV charging of San Francisco and supply grid (in kWh) for 1 year (Martínez-Lao et al., 2017; Linru et al., 2020; Mohamed et al., 2022).
6 Conclusion
The integration of PV systems with EV charging infrastructure presents a promising solution for sustainable transportation and energy management. This comprehensive review has explored the various components, technologies, and strategies involved in developing PV-CS. The importance of energy storage systems, such as batteries and supercapacitors, in conjunction with PV systems to ensure reliable and efficient charging operations. Additionally, the integration of PV-CS with the grid and the implementation of smart charging strategies have been discussed, enabling bidirectional power flow and optimized energy management.
The review has also covered the different levels of EV charging interfaces, including Level 1, Level 2, and DC fast charging, as well as the concept of V2G technology. While V2G offers numerous benefits, such as grid support and energy arbitrage, it also faces challenges related to battery degradation, additional costs, and implementation complexity. Real-world case studies, such as the SAP Labs Mougins in France and innovative designs for solar bus and train terminals, have demonstrated the practical applications and potential of PV-CS infrastructures. Furthermore, the review has highlighted the importance of international standards, protocols, and communication frameworks for ensuring the interoperability, safety, and efficient operation of PV-CS systems.
Several future challenges were addressed, including the integration and acceptance of PV systems in protected landscapes, the optimization of energy management systems in PV-CS, and the optimal site selection for these charging stations. Additionally, the social impacts, economic considerations, and environmental implications of PV-driven EV charging stations have been discussed. This comprehensive review serves as a valuable resource for researchers, policymakers, and industry stakeholders, providing insights into the current state of PV-based EV charging infrastructures and highlighting the opportunities and challenges that lie ahead. Addressing these challenges through continued research, innovation, and collaboration will be crucial in realizing the full potential of PV-CS systems and accelerating the transition toward sustainable transportation and energy systems.
Author contributions
HR: Conceptualization, Data curation, Investigation, Methodology, Resources, Software, Visualization, Writing–original draft. LH: Resources, Writing–review and editing. LG: Formal Analysis, Investigation, Resources, Visualization, Writing–review and editing. AA: Conceptualization, Data curation, Formal Analysis, Investigation, Methodology, Resources, Software, Visualization, Writing–review and editing. AA: Formal Analysis, Project administration, Supervision, Validation, Writing–review and editing. RH: Conceptualization, Data curation, Formal Analysis, Investigation, Methodology, Software, Supervision, Validation, Visualization, Writing–review and editing. AS: Conceptualization, Formal Analysis, Investigation, Methodology, Resources, Software, Supervision, Validation, Visualization, Writing–original draft, Writing–review and editing. ShS: Data curation, Formal Analysis, Investigation, Methodology, Project administration, Software, Validation, Writing–original draft. SyS: Funding acquisition, Project administration, Resources, Supervision, Validation, Visualization, Writing–original draft, Writing–review and editing. PM: Supervision, Writing–review and editing.
Funding
The author(s) declare that no financial support was received for the research, authorship, and/or publication of this article.
Conflict of interest
Authors LG, LH, and SS were employed by the Power China Huadong Engineering Co., Ltd.
The remaining authors declare that the research was conducted in the absence of any commercial or financial relationships that could be construed as a potential conflict of interest.
Publisher’s note
All claims expressed in this article are solely those of the authors and do not necessarily represent those of their affiliated organizations, or those of the publisher, the editors and the reviewers. Any product that may be evaluated in this article, or claim that may be made by its manufacturer, is not guaranteed or endorsed by the publisher.
References
Acharige, S. S. G., Haque, M. E., Arif, M. T., and Hosseinzadeh, N. (2022). Review of electric vehicle charging technologies, configurations, and architectures. arXiv preprint arXiv:2209.15242.
Ali, O. M., and Alomar, O. R. (2023). Technical and economic feasibility analysis of a PV grid-connected system installed on a university campus in Iraq. Environ. Sci. Pollut. Res. 30 (6), 15145–15157. doi:10.1007/s11356-022-23199-y
Almasri, R. A., Alharbi, T., Alshitawi, M. S., Alrumayh, O., and Ajib, S. (2024). Related work and motivation for electric vehicle solar/wind charging stations: a review. World Electr. Veh. J. 15 (5), 215. doi:10.3390/wevj15050215
Alrubaie, A. J., Salem, M., Yahya, K., Mohamed, M., and Kamarol, M. (2023). A comprehensive review of electric vehicle charging stations with solar photovoltaic system considering market, technical requirements, network implications, and future challenges. Sustainability 15 (10), 8122. doi:10.3390/su15108122
An, K. (2020a). Battery electric bus infrastructure planning under demand uncertainty. Transp. Res. Part C Emerg. Technol. 111, 572–587. doi:10.1016/j.trc.2020.01.009
An, K. (2020b). Battery electric bus infrastructure planning under demand uncertainty. Transp. Res. Part C Emerg. Technol. 111, 572–587. doi:10.1016/J.TRC.2020.01.009
Arya, R., Soni, M., Soomar, A. M., and Keshta, I. (2024). “Battery-operated electric vehicles and plug-in hybrid electric vehicles,” in Artificial intelligence-empowered modern electric vehicles in smart grid systems (Elsevier), 207–228.
Barone, G., Buonomano, A., Calise, F., Forzano, C., and Palombo, A. (2019). Building to vehicle to building concept toward a novel zero energy paradigm: modelling and case studies. Renew. Sustain. Energy Rev. 101, 625–648. doi:10.1016/j.rser.2018.11.003
Bayram, I. S., Michailidis, G., Devetsikiotis, M., and Granelli, F. (2013). Electric power allocation in a network of fast charging stations. IEEE J. Sel. Areas Commun. 31 (7), 1235–1246. doi:10.1109/jsac.2013.130707
Calabro, A., Cohen, B., Daga, A., Miller, J., and McMahon, F. (2019). “Performance of 200-kW inductive charging system for range extension of electric transit buses,” in Itec 2019 - 2019 IEEE transportation electrification conference and expo. doi:10.1109/ITEC.2019.8790490
Cevik, S., and Ninomiya, K. (2023). Chasing the sun and catching the wind: energy transition and electricity prices in Europe. J. Econ. Finance 47 (4), 912–935. doi:10.1007/s12197-023-09626-x
Chandra, I., Singh, N. K., and Samuel, P. (2024). A comprehensive review on coordinated charging of electric vehicles in distribution networks. J. Energy Storage 89, 111659. doi:10.1016/j.est.2024.111659
Chandra Mouli, G. R., Bauer, P., and Zeman, M. (2016). System design for a solar powered electric vehicle charging station for workplaces. Appl. Energy 168, 434–443. doi:10.1016/J.APENERGY.2016.01.110
Cheikh-Mohamad, S., Celik, B., Sechilariu, M., and Locment, F. (2023). PV-powered charging station with energy cost optimization via V2G services. Appl. Sci. 13 (9), 5627. doi:10.3390/app13095627
Cheikh-Mohamad, S., Sechilariu, M., Locment, F., and Krim, Y. (2021). Pv-powered electric vehicle charging stations: preliminary requirements and feasibility conditions. Appl. Sci. 11 (4), 1770. doi:10.3390/app11041770
Ching, T. W. (2011). Design of electric vehicle charging station in Macau. J. Asian Electr. Veh. 9 (1), 1453–1458. doi:10.4130/JAEV.9.1453
Costa, V. B. F., Capaz, R. S., and Bonatto, B. D. (2023). Small steps towards energy poverty mitigation: life cycle assessment and economic feasibility analysis of a photovoltaic and battery system in a Brazilian indigenous community. Renew. Sustain. Energy Rev. 180, 113266. doi:10.1016/j.rser.2023.113266
DAmbrosio, V., Losasso, M., and Tersigni, E. (2021). Towards the energy transition of the building stock with BIPV: innovations, gaps and potential steps for a widespread use of multifunctional PV components in the building envelope. Sustainability 13 (22), 12609. doi:10.3390/su132212609
Dang, Q. (2018). “Electric vehicle (EV) charging management and relieve impacts in grids,” in 2018 9th IEEE international symposium on power electronics for distributed generation systems (Charlotte, NC, Unites States: PEDG 2018). doi:10.1109/PEDG.2018.8447802
Das, H., Rahman, M., Li, S., and Tan, C. W. (2020a). Electric vehicles standards, charging infrastructure, and impact on grid integration: a technological review. Renew. Sustain. Energy Rev. 120, 109618. doi:10.1016/j.rser.2019.109618
Das, H. S., Rahman, M. M., Li, S., and Tan, C. W. (2020b). Electric vehicles standards, charging infrastructure, and impact on grid integration: a technological review. Renew. Sustain. Energy Rev. 120, 109618. doi:10.1016/J.RSER.2019.109618
Das, H. S., Rahman, M. M., Li, S., and Tan, C. W. (2020c). Electric vehicles standards, charging infrastructure, and impact on grid integration: a technological review. Renew. Sustain. Energy Rev. 120, 109618. doi:10.1016/j.rser.2019.109618
Deshmukh, S. S., and Pearce, J. M. (2021). Electric vehicle charging potential from retail parking lot solar photovoltaic awnings. Renew. Energy 169, 608–617. doi:10.1016/j.renene.2021.01.068
Dharmakeerthi, C. H., Mithulananthan, N., and Saha, T. K. (2011). “Overview of the impacts of plug-in electric vehicles on the power grid,” in 2011 IEEE PES innovative smart grid technologies, IEEE, 1–8.
Fachrizal, R., Shepero, M., Åberg, M., and Munkhammar, J. (2022). Optimal PV-EV sizing at solar powered workplace charging stations with smart charging schemes considering self-consumption and self-sufficiency balance. Appl. Energy 307, 118139. doi:10.1016/j.apenergy.2021.118139
Fathabadi, H. (2020). Novel stand-alone, completely autonomous and renewable energy based charging station for charging plug-in hybrid electric vehicles (PHEVs). Appl. Energy 260, 114194. doi:10.1016/j.apenergy.2019.114194
García-Triviño, P., Torreglosa, J. P., Fernández-Ramírez, L. M., and Jurado, F. (2016). Control and operation of power sources in a medium-voltage direct-current microgrid for an electric vehicle fast charging station with a photovoltaic and a battery energy storage system. Energy 115, 38–48. doi:10.1016/j.energy.2016.08.099
Gbadamosi, S. L., Ogunje, F. S., Wara, S. T., and Nwulu, N. I. (2022). Techno-economic evaluation of a hybrid energy system for an educational institution: a case study. Energies (Basel) 15 (15), 5606. doi:10.3390/en15155606
Ghasemi-Marzbali, A. (2022). Fast-charging station for electric vehicles, challenges and issues: a comprehensive review. J. Energy Storage 49, 104136. doi:10.1016/j.est.2022.104136
Habib, S., Kamran, M., and Rashid, U. (2015). Impact analysis of vehicle-to-grid technology and charging strategies of electric vehicles on distribution networks–a review. J. Power Sources 277, 205–214. doi:10.1016/j.jpowsour.2014.12.020
Habib, S., Khan, M. M., Abbas, F., Sang, L., Shahid, M. U., and Tang, H. (2018). A comprehensive study of implemented international standards, technical challenges, impacts and prospects for electric vehicles. IEEE Access 6, 13866–13890. doi:10.1109/access.2018.2812303
Habib, S., Khan, M. M., Hashmi, K., Ali, M., and Tang, H. (2017). “A comparative study of electric vehicles concerning charging infrastructure and power levels,” in 2017 international conference on frontiers of information technology (FIT) (IEEE), 327–332.
Han, S., Han, S., and Aki, H. (2014). A practical battery wear model for electric vehicle charging applications. Appl. Energy 113, 1100–1108. doi:10.1016/j.apenergy.2013.08.062
He, Y., Song, Z., and Liu, Z. (2019). Fast-charging station deployment for battery electric bus systems considering electricity demand charges. Sustain Cities Soc. 48, 101530. doi:10.1016/j.scs.2019.101530
Jaman, S., Verbrugge, B., Garcia, O. H., Abdel-Monem, M., Oliver, B., Geury, T., et al. (2022). Development and validation of V2G technology for electric vehicle chargers using combo CCS type 2 connector standards. Energies (Basel) 15 (19), 7364. doi:10.3390/en15197364
Jang, Y., Sun, Z., Ji, S., Lee, C., Jeong, D., Choung, S., et al. (2021). Grid-connected inverter for a PV-powered electric vehicle charging station to enhance the stability of a microgrid. Sustainability 13 (24), 14022. doi:10.3390/su132414022
Jin, H., Lee, S., Nengroo, S. H., and Har, D. (2022). Development of charging/discharging scheduling algorithm for economical and energy-efficient operation of multi-EV charging station. Appl. Sci. 12 (9), 4786. doi:10.3390/app12094786
John, L., Patro, R., Padmakala, S., and Madhumala, R. B. (2024). “E-mobility as a service and its economic impact: regulations, policies, governance, and best practices in E-mobility ecosystems,” in E-mobility in electrical energy systems for sustainability (IGI Global), 42–64. doi:10.4018/979-8-3693-2611-4.ch003
Kassem, Y., Gokcekus, H., Ab Albakoush, I. A., and Abdullah, K. S. B. (2023b). Solar-powered solutions for the water and energy shortage problem: the case study of nahr el bared, Lebanon. Eng. Technol. Appl. Sci. Res. 13 (3), 10861–10869. doi:10.48084/etasr.5858
Kassem, Y., Gokcekus, H., and Agila, F. A. R. (2023c). Techno-economic feasibility assessment for the promotion of grid-connected rooftop PV systems in Botswana: a case study. Eng. Technol. Appl. Sci. Res. 13 (2), 10328–10337. doi:10.48084/etasr.5668
Kassem, Y., Gokcekus, H., and Aljatlawe, A. (2023a). Utilization of solar energy for electric vehicle charging and the energy consumption of residential buildings in northern Cyprus: a case study. Eng. Technol. Appl. Sci. Res. 13 (5), 11598–11607. doi:10.48084/etasr.6142
Kassem, Y., Gokcekus, H., and Aljatlawe, A. (2023e). Utilization of solar energy for electric vehicle charging and the energy consumption of residential buildings in northern Cyprus: a case study. Eng. Technol. Appl. Sci. Res. 13 (5), 11598–11607. doi:10.48084/ETASR.6142
Kassem, Y., Gokcekus, H., Hamad, O. A. M., and Fayid, F. M. B. (2023d). Economic viability of a 6.5 kW off-grid solar PV with various sun-tracking systems in Northern Cyprus: a case study. Eng. Technol. Appl. Sci. Res. 13 (2), 10608–10621. doi:10.48084/etasr.5744
Khalid, M., Ahmad, F., and Panigrahi, B. K. (2021a). Design, simulation and analysis of a fast charging station for electric vehicles. Energy Storage 3 (6), e263. doi:10.1002/est2.263
Khalid, M. R., Khan, I. A., Hameed, S., Asghar, M. S. J., and Ro, J.-S. (2021b). A comprehensive review on structural topologies, power levels, energy storage systems, and standards for electric vehicle charging stations and their impacts on grid. IEEE Access 9, 128069–128094. doi:10.1109/access.2021.3112189
Khan, S., Ahmad, A., Ahmad, F., Shafaati Shemami, M., Saad Alam, M., and Khateeb, S. (2018). A comprehensive review on solar powered electric vehicle charging system. Smart Sci. 6 (1), 54–79. doi:10.1080/23080477.2017.1419054
Khan, S., Sudhakar, K., and bin Yusof, M. H. (2023). Building integrated photovoltaics powered electric vehicle charging with energy storage for residential building: design, simulation, and assessment. J. Energy Storage 63, 107050. doi:10.1016/j.est.2023.107050
Khan, S., Sudhakar, K., Hazwan Yusof, M., and Sundaram, S. (2024). Review of building integrated photovoltaics system for electric vehicle charging. Chem. Rec. 24, e202300308. doi:10.1002/tcr.202300308
Kong, F., Liu, X., and Lee, I. (2018). “Joint rate control and demand balancing for electric vehicle charging,” in 2018 IEEE/ACM third international conference on internet-of-things design and implementation (IoTDI) (IEEE), 213–224.
Kotarela, F., Rigogiannis, N., Glavinou, E., Mpailis, F., Kyritsis, A., and Papanikolaou, N. (2024). Techno-economic and environmental assessment of a photovoltaic-based fast-charging station for public utility vehicles. Energies (Basel) 17 (3), 632. doi:10.3390/en17030632
Lee, Z. J., Pang, J. Z. F., and Low, S. H. (2020). Pricing EV charging service with demand charge. Electr. Power Syst. Res. 189, 106694. doi:10.1016/j.epsr.2020.106694
Linru, J., yuanxing, Z., taoyong, L., xiaohong, D., and jing, Z. (2020). “Analysis on charging safety and optimization of electric vehicles,” in 2020 IEEE 6th international conference on computer and communications (ICCC) (IEEE), 2382–2385.
Liu, X., Liu, X., Zhang, X., Zhou, Y., Chen, J., and Ma, X. (2023d). Optimal location planning of electric bus charging stations with integrated photovoltaic and energy storage system. Computer-Aided Civ. Infrastructure Eng. 38 (11), 1424–1446. doi:10.1111/MICE.12935
Liu, X., Liu, X. C., Xie, C., and Ma, X. (2023a). Impacts of photovoltaic and energy storage system adoption on public transport: a simulation-based optimization approach. Renew. Sustain. Energy Rev. 181, 113319. doi:10.1016/j.rser.2023.113319
Liu, X., Liu, X. C., Xie, C., and Ma, X. (2023c). Impacts of photovoltaic and energy storage system adoption on public transport: a simulation-based optimization approach. Renew. Sustain. Energy Rev. 181, 113319. doi:10.1016/J.RSER.2023.113319
Liu, X., Shang, W. L., Correia, G. H. de A., Liu, Z., and Ma, X. (2024b). A sustainable battery scheduling and echelon utilization framework for electric bus network with photovoltaic charging infrastructure. Sustain Cities Soc. 101 (Feb), 105108. doi:10.1016/j.scs.2023.105108
Liu, X., Shang, W.-L., de Almeida Correia, G. H., Liu, Z., and Ma, X. (2024a). A sustainable battery scheduling and echelon utilization framework for electric bus network with photovoltaic charging infrastructure. Sustain Cities Soc. 101, 105108. doi:10.1016/j.scs.2023.105108
Liu, Z., Wang, Y., and Feng, J. (2023b). Vehicle-type strategies for manufacturer’s car sharing. Kybernetes 52 (10), 4686–4711. doi:10.1108/k-11-2021-1095
Lucchi, E., Adami, J., and Stawinoga, A. E. (2023). Social acceptance of photovoltaic systems in heritage buildings and landscapes: exploring barriers, benefits, drivers, and challenges for technical stakeholders in northern Italy. Sustain. Energy Technol. Assessments 60, 103544. doi:10.1016/j.seta.2023.103544
Martínez-Lao, J., Montoya, F. G., Montoya, M. G., and Manzano-Agugliaro, F. (2017). Electric vehicles in Spain: an overview of charging systems. Renew. Sustain. Energy Rev. 77, 970–983. doi:10.1016/j.rser.2016.11.239
Mohamed, A. A. S., Shaier, A. A., Metwally, H., and Selem, S. I. (2022). An overview of dynamic inductive charging for electric vehicles. Energies (Basel) 15 (15), 5613. doi:10.3390/en15155613
Mouli, G. R. C., Bauer, P., and Zeman, M. (2016). System design for a solar powered electric vehicle charging station for workplaces. Appl. Energy 168, 434–443. doi:10.1016/j.apenergy.2016.01.110
Nawaz, M. U., Qureshi, M. S., and Umar, S. (2024). Integration of solar energy systems with electric vehicle charging infrastructure: challenges and opportunity. Rev. Espanola Doc. Cient. 18 (02), 1–18.
Niccolai, A., Bettini, L., and Zich, R. (2021). Optimization of electric vehicles charging station deployment by means of evolutionary algorithms. Int. J. Intelligent Syst. 36 (9), 5359–5383. doi:10.1002/int.22515
Noussan, M., and Jarre, M. (2021). Assessing commuting energy and emissions savings through remote working and carpooling: lessons from an Italian region. Energies (Basel) 14 (21), 7177. doi:10.3390/en14217177
Onishi, V. C., Antunes, C. H., and Fernandes Trovão, J. P. (2020b). Optimal energy and reserve market management in renewable microgrid-PEVs parking lot systems: V2G, demand response and sustainability costs. Energies (Basel) 13 (8), 1884. doi:10.3390/EN13081884
Onishi, V. C., Antunes, C. H., and Trovão, J. P. F. (2020a). Optimal energy and reserve market management in renewable microgrid-PEVs parking lot systems: V2G, demand response and sustainability costs. Energies (Basel) 13 (8), 1884. doi:10.3390/en13081884
Pareek, S., Sujil, A., Ratra, S., and Kumar, R. (2020). “Electric vehicle charging station challenges and opportunities: a future perspective,” in Proceedings - 2020 international conference on emerging trends in communication, control and computing, ICONC3 2020. doi:10.1109/ICONC345789.2020.9117473
Pathak, P. K., Yadav, A. K., Padmanaban, S., and Alvi, P. A. (2022). Design of robust multi-rating battery charger for charging station of electric vehicles via solar PV system. Electr. Power Components Syst. 50 (14–15), 751–761. doi:10.1080/15325008.2022.2139870
Pazzaglia, J.-C. (2024). ‘Plan vert’ by SAP Labs France: growing a sustainable lifestyle. Available at: https://www.linkedin.com/pulse/plan-vert-sap-labs-france-growing-sustainable-pazzaglia-kn45f/.
Pilotti, L., Moretti, L., Martelli, E., and Manzolini, G. (2023). Optimal E-fleet charging station design with V2G capability. Sustain. Energy, Grids Netw. 36, 101220. doi:10.1016/j.segan.2023.101220
Pratap, A., Palwalia, D. K., Tepan, M., Jain, N., Kumari, A., and Mina, A. (2022). “Machine learning-based DC microgrid control for electric vehicle charging stations,” in International conference on flexible electronics for electric vehicles (Springer), 395–404.
Rajendran, G., Vaithilingam, C. A., Misron, N., Naidu, K., and Ahmed, M. R. (2021). A comprehensive review on system architecture and international standards for electric vehicle charging stations. J. Energy Storage 42, 103099. doi:10.1016/j.est.2021.103099
Rauf, A., Nureen, N., Irfan, M., and Ali, M. (2023). The current developments and future prospects of solar photovoltaic industry in an emerging economy of India. Environ. Sci. Pollut. Res. 30 (16), 46270–46281. doi:10.1007/s11356-023-25471-1
Rehman, A. U., Ullah, Z., Shafiq, A., Hasanien, H. M., Luo, P., and Badshah, F. (2023a). Load management, energy economics, and environmental protection nexus considering PV-based EV charging stations. Energy 281, 128332. doi:10.1016/j.energy.2023.128332
Rehman, S., Mohammed, A. B., Alhems, L., and Alsulaiman, F. (2023b). Comparative study of regular and smart grids with PV for Electrification of an academic campus with EV charging. Environ. Sci. Pollut. Res. 30 (31), 77593–77604. doi:10.1007/s11356-023-27859-5
Sagaria, S., Duarte, G., Neves, D., and Baptista, P. (2022). Photovoltaic integrated electric vehicles: assessment of synergies between solar energy, vehicle types and usage patterns. J. Clean. Prod. 348, 131402. doi:10.1016/j.jclepro.2022.131402
Saleh, B., Yousef, A. M., Ebeed, M., Abo-Elyousr, F. K., Elnozahy, A., Mohamed, M., et al. (2021). Design of PID controller with grid connected hybrid renewable energy system using optimization algorithms. J. Electr. Eng. Technol. 16 (6), 3219–3233. doi:10.1007/s42835-021-00804-7
Sánchez, J. T., Del Río, J. A., and Sánchez, A. (2022). Economic feasibility analysis for an electric public transportation system: two cases of study in medium sized cities in Mexico. PLoS One 17 (8), e0272363. doi:10.1371/journal.pone.0272363
Sarker, M. T., Haram, M. H. S. M., Shern, S. J., Ramasamy, G., and Al Farid, F. (2024). Second-life electric vehicle batteries for home photovoltaic systems: transforming energy storage and sustainability. Energies (Basel) 17 (10), 2345. doi:10.3390/en17102345
Savari, G. F., Sathik, M. J., Raman, L. A., El-Shahat, A., Hasanien, H. M., Almakhles, D., et al. (2023). Assessment of charging technologies, infrastructure and charging station recommendation schemes of electric vehicles: a review. Ain Shams Eng. J. 14 (4), 101938. doi:10.1016/j.asej.2022.101938
Sechilariu, M., et al. (2021). PV-powered electric vehicle charging stations: preliminary requirements and feasibility conditions.
Sekhar, K. S. R., Chaudhari, M. A., and Khadkikar, V. (2023). Enhanced hybrid converter topology for PV-grid-EV integration. IEEE Trans. Energy Convers. 38, 2634–2646. doi:10.1109/tec.2023.3287890
Shah, T. H., Shabbir, A., Waqas, A., Janjua, A. K., Shahzad, N., Pervaiz, H., et al. (2023). Techno-economic appraisal of electric vehicle charging stations integrated with on-grid photovoltaics on existing fuel stations: a multicity study framework. Renew. Energy 209, 133–144. doi:10.1016/j.renene.2023.03.128
Shakeel, F. M., and Malik, O. P. (2019). “Vehicle-to-grid technology in a micro-grid using DC fast charging architecture,” in 2019 IEEE Canadian conference of electrical and computer engineering (CCECE) (IEEE), 1–4.
Sierra, A., and Reinders, A. (2020). “A design-driven exploration of photovoltaic applications in electric mobility systems,” in 2020 47th IEEE photovoltaic specialists conference (PVSC) (IEEE), 245–249.
Sierra, A., and Reinders, A. (2021). Designing innovative solutions for solar-powered electric mobility applications. Prog. Photovoltaics Res. Appl. 29 (7), 802–818. doi:10.1002/PIP.3385
Sierra Rodriguez, A., de Santana, T., MacGill, I., Ekins-Daukes, N. J., and Reinders, A. (2020a). A feasibility study of solar PV-powered electric cars using an interdisciplinary modeling approach for the electricity balance, CO2 emissions, and economic aspects: the cases of The Netherlands, Norway, Brazil, and Australia. Prog. Photovoltaics Res. Appl. 28 (6), 517–532. doi:10.1002/PIP.3202
Sierra, A., and Rodriguez, A. (2020b). Electric vehicle charging station challenges and opportunities: a future perspective. IEEE. doi:10.1002/pip.3385
Singh, S., Vaidya, B., and Mouftah, H. T. (2022). Smart EV charging strategies based on charging behavior. Front. Energy Res. 10, 773440. doi:10.3389/fenrg.2022.773440
Sivaraman, P., and Sharmeela, C. (2021). “Power quality problems associated with electric vehicle charging infrastructure,” in Power quality in modern power systems (Elsevier), 151–161.
Soomar, A. M., Guanghua, L., Shaikh, S., Shah, S. H. H., and Musznicki, P. (2023). Scrutiny of power grids by penetrating PV energy in wind farms: a case study of the wind corridor of Jhampir, Pakistan. Front. Energy Res. 11 (Jun). doi:10.3389/fenrg.2023.1164892
Soomar, A. M., Hakeem, A., Messaoudi, M., Musznicki, P., Iqbal, A., and Czapp, S. (2022). Solar photovoltaic energy optimization and challenges. Front. Energy Res. 10. doi:10.3389/fenrg.2022.879985
Stamatellos, G., Zogou, O., and Stamatelos, A. (2022). Interaction of a house’s rooftop PV system with an electric vehicle’s battery storage and air source heat pump. Solar 2 (2), 186–214. doi:10.3390/SOLAR2020011
Swamynathan, K., Mahalingam, N., Paramasivam, A., and Vijayalakshmi, S. (2023). PV based OFF grid charging station for E-vehicles using PWM and phase shift controlled interleaved three port converter. SN Appl. Sci. 5 (12), 331. doi:10.1007/s42452-023-05571-w
Tirunagari, S., Gu, M., and Meegahapola, L. (2022). Reaping the benefits of smart electric vehicle charging and vehicle-to-grid technologies: regulatory, policy and technical aspects. IEEE Access 10, 114657–114672. doi:10.1109/ACCESS.2022.3217525
Tseng, W. C., and Hsieh, I. Y. L. (2023). Impacts of electric fleet charging patterns under different solar power penetration levels: hourly grid variations and operating emissions. Transp. Res. D. Transp. Environ. 122 (Sep), 103848. doi:10.1016/j.trd.2023.103848
Wang, J., Wang, Z., Yang, B., Liu, F., Wei, W., and Guan, X. (2024). V2G for frequency regulation service: a stackelberg game approach considering endogenous uncertainties. IEEE Trans. Transp. Electrification, 1. doi:10.1109/tte.2024.3392496
Wang, L., Qin, Z., Slangen, T., Bauer, P., and Van Wijk, T. (2021). Grid impact of electric vehicle fast charging stations: trends, standards, issues and mitigation measures-an overview. IEEE Open J. Power Electron. 2, 56–74. doi:10.1109/ojpel.2021.3054601
Willrett, U. (2017). “Interoperability between charging infrastructure and electric vehicle,” in Netzintegration der Elektromobilität 2017: Mobilitätswandel konsequent entwickeln-2. Internationale ATZ-Fachtagung (Springer), 113–125.
Wittkopf, S., Valliappan, S., Liu, L., Ang, K. S., and Cheng, S. C. J. (2012). Analytical performance monitoring of a 142.5kW p grid-connected rooftop BIPV system in Singapore. Renew. Energy 47, 9–20. doi:10.1016/j.renene.2012.03.034
Wu, J., Zhang, M., Xu, T., Gu, D., Xie, D., Zhang, T., et al. (2023a). A review of key technologies in relation to large-scale clusters of electric vehicles supporting a new power system. Renew. Sustain. Energy Rev. 182, 113351. doi:10.1016/j.rser.2023.113351
Wu, J., Zhang, M., Xu, T., Gu, D., Xie, D., Zhang, T., et al. (2023b). A review of key technologies in relation to large-scale clusters of electric vehicles supporting a new power system. Renew. Sustain. Energy Rev. 182 (Aug), 113351. doi:10.1016/j.rser.2023.113351
Yan, D., Huang, S., and Chen, Y. (2023). Real-time feedback based online aggregate EV power flexibility characterization. IEEE Trans. Sustain Energy 15, 658–673. doi:10.1109/tste.2023.3324705
Yilmaz, M., and Krein, P. T. (2012). Review of battery charger topologies, charging power levels, and infrastructure for plug-in electric and hybrid vehicles. IEEE Trans. Power Electron 28 (5), 2151–2169. doi:10.1109/tpel.2012.2212917
Yu, G., Ye, X., Xia, X., and Chen, Y. (2024). Digital twin enabled transition towards the smart electric vehicle charging infrastructure: a review. Sustain Cities Soc. 108, 105479. doi:10.1016/j.scs.2024.105479
Zenhom, I. A., Shaaban, M. F., and Omran, W. A. (2023). Grid interactive charging of EVs in PV-powered parking lots considering uncertainties. IEEE Access 11, 111292–111301. doi:10.1109/ACCESS.2023.3322201
Zentani, A., Almaktoof, A., and Kahn, M. T. (2024). A comprehensive review of developments in electric vehicles fast charging technology. Appl. Sci. 14 (11), 4728. doi:10.3390/app14114728
Zhang, B., Zhang, Y., Li, J., Song, Y., and Wang, Z. (2023). Does the energy efficiency of buildings bring price premiums? Evidence from urban micro-level energy data. Renew. Sustain. Energy Rev. 181, 113315. doi:10.1016/j.rser.2023.113315
Zhang, X., Zou, Y., Fan, J., and Guo, H. (2019). Usage pattern analysis of Beijing private electric vehicles based on real-world data. Energy 167, 1074–1085. doi:10.1016/j.energy.2018.11.005
Keywords: electric vehicles, photovoltaic energy generation, charging stations, renewable energy resources, grid integration photovoltaic charging station control system solar PV charging station
Citation: Rashid H, Hua LM, Guanghua L, Hasan R, AlKaseem A, Ali A, Shah SHH, Shaikh S, Soomar AM and Musznicki P (2024) A comprehensive review on economic, environmental impacts and future challenges for photovoltaic-based electric vehicle charging infrastructures. Front. Energy Res. 12:1411440. doi: 10.3389/fenrg.2024.1411440
Received: 03 April 2024; Accepted: 27 June 2024;
Published: 06 August 2024.
Edited by:
Sudhakar Kumarasamy, Universiti Malaysia Pahang, MalaysiaCopyright © 2024 Rashid, Hua, Guanghua, Hasan, AlKaseem, Ali, Shah, Shaikh, Soomar and Musznicki. This is an open-access article distributed under the terms of the Creative Commons Attribution License (CC BY). The use, distribution or reproduction in other forums is permitted, provided the original author(s) and the copyright owner(s) are credited and that the original publication in this journal is cited, in accordance with accepted academic practice. No use, distribution or reproduction is permitted which does not comply with these terms.
*Correspondence: Rija Hasan, cmlqYS5oYXNhbkBqaW5uYWguZWR1, cmlqYWhhc2FuMjVAZ21haWwuY29t