- Department of Electrical Engineering, Mehran University of Engineering and Technology, Jamshoro, Pakistan
Pakistan is facing the worst level of energy and economic crisis of its history. The underlying reason of the economic crisis is basically due to the energy crisis. Various approaches have been adopted to tackle the energy crises which the country is facing for over 2 decades. Demand-side management (DSM) is the most potent and cost-effective option to redress the energy crisis, which, unfortunately, has been the most neglected strategy in Pakistan. DSM has the potential to save up to 10.0%–15.0% of primary energy to ensure the country’s future energy security. Laws and codes do exist, but ironically, the most vital element of DSM’s policy framework and implementation mechanism is missing. Hence, the main objective of this research is to develop a DSM model for Pakistan and analyze the reference and DSM scenarios. Low-emission analysis platform (LEAP) is used to develop Pakistan’s DSM model for the study period 2021–2050. The three alternative DSM scenarios developed include energy efficiency (EEF), energy conservation (EC), and load management (LOM), and they are all analyzed. The model results estimate the electricity demand forecast of 1009.8 TWh under the reference scenario in 2050, whereas DSM tends to result in a 26.38% decreased electricity demand compared to the reference scenario. The alternative DSM scenarios also outperform the reference scenario. In 2050, the EC scenario reduces consumption by 178.0 TWh and GHG emissions by 19.20 million metric tons, the EEF scenario reduces consumption by 110.30 TWh and GHG emissions by 10.04 million metric tons, and the LOM scenario suggests reduced consumption by 101.0 TWh and GHG emissions by 6.20 million metric tons. This study concluded that the DSM must be institutionalized in Pakistan by building a robust regulatory framework and execution mechanism at the government and utility levels.
1 Introduction
Pakistan’s power sector planning has been predominantly based on power generation capacity addition strategies. These strategies, however, could not deliver but have rather added to the suffering of electricity consumers (Abdullah et al., 2020). Apart from the lack of financial resources, instability of political situation, and governance issues, the energy planning efforts in Pakistan lacks to recognize demand-side management (DSM) as a key ingredient of policy instrument (Aghahosseini et al., 2023). Contemporary literature suggests that only partial and piecemeal DSM steps were considered in the past but not as a policy endeavor. Resultantly, the country has been in the grip of energy crises for over 2 decades, and currently, the crises are the worst in its history. As such, the country’s depleting energy system necessities the development of a sound DSM planning, policy, and implementation framework to overcome the ongoing energy crises (Abbas et al., 2023).
Despite sufficient capacity addition over the years, the per capita electricity consumption of Pakistan is one of the lowest in the world. On the other hand, the power losses are also critically high in the electricity transmission and distribution system. Again, there is no cogent effort to control such waste of energy.
Among various other improvement methods, Pakistan essentially needs to consider DSM as a key policy endeavor as it has significant potential to conserve electricity to the extent of 17.0%, which can be further enhanced between 15.0% and 20.0% (Raza et al., 2024). This would help to conserve an estimated amount of energy to the tune of 11 MTOE (million tons of oil equivalent). This could greatly help to cut the net oil import by 51.0%. It would cause a significant reduction in import costs for the nation and reduce the energy costs, as for each dollar of GDP, Pakistan is consuming 15.0% more energy than India and 25.0% more energy than Philippines (Ahmad et al., 2020).
Energy-efficiency and conservation measures aim to reduce energy demand by using energy-efficient appliances/tools/equipment or by changing the behavior patterns of consumers (Abeykoon et al., 2021). These measures altogether help to ensure energy security and help in containing the climate change (Huang and Zhai, 2021). Energy systems worldwide are shifting toward sustainability, driven by energy security, equity, and environmental sustainability goals (Hulio et al., 2022). Historically, Pakistan has been subjected to energy demand suppression due to limited supplies and a lack of adequate infrastructure development to provide energy to the industrial sector. Resultantly, the country had suffered from a large demand–supply energy gap in the power sector, which at times stood at 5,000.0 MW (Raza et al., 2023a). This demand–supply gap has been the result of poor policy endeavor and even the lack of implantation of various energy and power policies announced from time to time (Baloch et al., 2021).
Currently, the country is experiencing a situation where the energy demand exceeds the supply. The issue is not because of a capacity shortage but rather due to insufficient fuels, operational issues in public-sector thermal power plants, and limitations of the transmission and distribution systems (Mirjat et al., 2017). Regarding the DSM, there is generally a lack of awareness among the public about the importance of conserving electricity and taking advantages of such savings.
Pakistan’s indigenous fossil fuel resources are limited and not sufficient to meet the demand, whereas the import costs are too high for the national exchequer. On the other hand, harnessing hydro and other renewable energy resources effectively requires significant financial resources, which the country does not have (Masoomi et al., 2020). Thus, prioritizing the development of alternative energy sources and implementing a sustainable demand-side management policy through ongoing research and development should be the focus of power planning. There are various benefits of DSM in contrast to alternative energy-efficient techniques, and some of these are listed in the following text (Khan et al. (2023); Li and Pye (2018); Williams et al. (2023); Hosseinnia et al. (2018); Raza et al. (2023b)):
⁃ DSM is often more cost-effective than building new power plants or upgrading infrastructure.
⁃ DSM allows for real-time adjustments to energy demand, making it easier to balance supply and demand.
⁃ DSM helps to reduce the peak demand which can prevent brownouts and blackouts.
⁃ Increased grid resilience: by managing energy demand, DSM enhances grid stability and reliability.
⁃ DSM promotes energy-efficient practices and technologies.
⁃ DSM encourages active customer participation in energy management.
⁃ By reducing energy consumption, DSM helps to decrease greenhouse gas emissions.
⁃ DSM can optimize energy use when combined with renewable energy sources.
⁃ DSM provides valuable data for energy planning and optimization.
⁃ DSM can be applied to various industries and sectors, making it a versatile solution.
On the technology side, the DSM measure needs to be prioritized; some of the most feasible measures include (Kiptoo et al. (2020); Meyabadi and Deihimi (2017); Kanakadhurga and Prabaharan (2022); Raza et al. (2023c)) the following:
⁃ Installation of LEDs to replace conventional lamps and CFLs, starting from the government sector buildings and streetlights.
⁃ Power factor improvement by installation of capacitors, particularly on industrial and agricultural connections.
⁃ Application of pre-paid, smart/AMI meters.
⁃ Promoting the use of inverter-type air conditioners.
⁃ Replacing inefficient substandard agriculture motors and pumps by standard efficient equipment.
⁃ Strict compliance of the implementation of the Energy Efficiency and Conservation Act and Building Energy Code of Pakistan.
⁃ Strong monitoring of prohibition on the sale of poor/inferior quality material life cables, wires, accessories, switches, sockets, chokes, lamps, and fans.
Given the above advantages and approaches of DSM, it is extremely feasible for Pakistan to consider a full-scale DSM study across various consumer groups and devise an effective policy narrative. The country has a significant energy-saving potential which can be attained by implementing meaningful energy conservation and management programs. Energy saving is cheaper than energy generation and is the most affordable solution to energy shortfall. Various energy consuming sectors have huge potential to conserve energy in Pakistan, as illustrated in Figure 1 (Bughio et al., 2021).
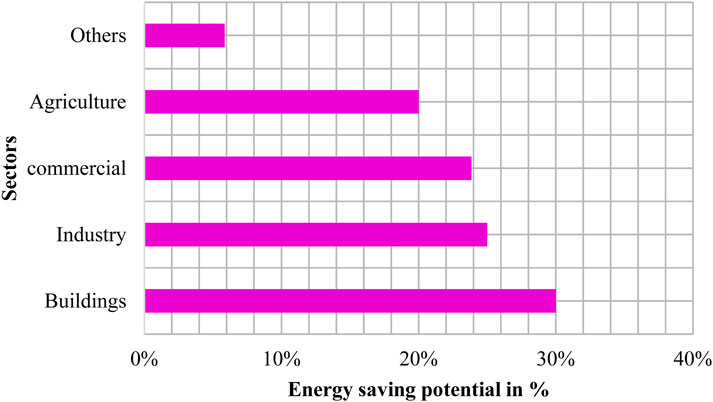
Figure 1. Energy saving potential of Pakistan (Bughio et al., 2021)
Considering the huge energy-saving potential in the power sector of Pakistan, this study develops a DSM energy model for the sector. Following modeling the baseline reference scenario and a broad DSM scenario, three alternative DSM scenarios, viz., energy efficiency (EEF), energy conservation (EC), and load management (LOM), are developed and analyzed. The low emission analysis platform (LEAP) modeling framework has been employed in this study to undertake the DSM scenario analysis for the study period 2021–2050. The results of this study are significant and endorse undertaking the DSM endeavor in Pakistan. Various initiatives pertaining DSM undertaken across the globe and Pakistan are briefly reviewed in Section 2 of this study. A detailed description of the research methodology of this study is provided in Section 3, whereas Section 4 contains the results and analysis of the scenario modeling exercise of this study. The recommendations in the shape of DSM strategies devised based on this study’s finding are provided in Section 4. Finally, Section 5 summarizes the conclusion drawn from this study.
2 Worldwide DSM experience: a review
Global recognition and favorable outcomes of DSM strategies and measures have pushed various nations to consider as well implement DSM initiatives. A summary of the DSM experience as reported in the contemporary literature follows as under:
2.1 Demand-side management in India
There are various studies reported in the literature which highlight the DSM endeavor accomplished in India. In a study by Patnam and Pindoriya (2021), a detailed review of state-level DSM initiatives in India is reported. These states include Andhra Pradesh, Delhi, Gujarat, Haryana, Karnataka, Maharashtra, Tamil Nadu, and West Bengal. These states experienced energy-related peak demand deficits. This deficit ranged from 138.0 million KWh in Delhi to 17,000.0 million KWh in Andhra Pradesh. The peak deficit varied from approximately 1,000.0 MW to 3,000.0 MW in Maharashtra. All of the states were showing energy requirement growth from 5.7% to 7.5% (Patnam and Pindoriya, 2021). DSM initiatives, therefore, were considered and undertaken as stop-gap arrangements to cope with the increasing demand and avert the capacity addition. Another study by Harsh and Das (2021), also pertaining India, established that large-scale DSM activities can help to reduce power purchase costs during peak hours by decreasing the demand. It is recommended in this study that one way to redirect energy to those who pay higher tariffs is to reduce the consumption of those who pay a subsided cost of energy. Lowering consumption can help lower purchase costs and provide energy to high-revenue commercial and industrial consumers. These and other similar studies concluded that electricity distribution companies (DISCOMs) act as intermediaries between consumers and regulators, making them a key player in the success of state DSM programs. DISCOMs and consumers stand to benefit from DSM programs. Therefore, DISCOMs should lead in conducting load research studies and integrating DSM into their power purchase plans.
2.2 Utility demand-side management programs in the USA
The USA is pioneer in the utility-level DSM initiative. According to various studies (Golmohamadi (2022); Niamir et al. (2020); Creutzig et al. (2022)), DSM programs in USA utilities are divided into various categories, as listed below:
a) Undertaking information and awareness.
b) Energy audits and technical information with reference for improvement in energy usage.
c) For availing efficient technologies offering financial assistance in the form of direct payments or loans.
d) Installation of energy-efficient technologies either directly or for free.
e) To guarantee energy performance, forming third-party contracts between the customer and the utilities.
f) Financial assistance or bill reduction for customers who adopt technologies that shift and control loads, such as thermal storage.
g) Introducing innovative tariffs using time-of-day and real-time prices that can optimize DSM programs.
The programs from “a” to “e” focus on energy efficiency, whereas programs “f” to “g” aimed for peak load reduction through load shifting or building in off-peak periods. In response to concerns about reliance on foreign oil imports and the potential environmental effects of nuclear power, DSM programs were initiated in 1970s. The state regulators emphasize extending these programs toward integrated resource planning and offering incentives as cost-effective solutions. Considering the feasible outcome of such programs, from mid-1980s, US utility companies have allocated approximately 1.0% of their revenues for DSM initiatives and implementation.
2.3 Demand-side management in Canada
Following the 1973 oil embargo, Canada emerged as a prominent player in developing and executing DSM programs (Arihan, 2021). The energy consumers responded positively to this initiative, resulting in a 2.5% decrease in the annual energy consumption (Schramm, 2024). Therefore, demand-side management programs have become a common practice for most utilities in North America (Raskin et al., 2022). Policy decisions on building codes, automotive fuel efficiency, and industrial processes are also included as key DSM considerations in Canada. These programs include incentives, subsidies, communication and education, standard legislation, and pricing (MacKinnon et al., 2020). The DSM programs have incorporated several features, including the energy labeling, residential audit program, appliance inspection, motor performance analysis programs, heating-ventilation air conditioning system evaluation program, performance contracting, and new efficient building program. These programs have significantly reduced energy intensity in residential, commercial, industrial, and road transportation sectors, and decreased the amount of energy used per dollar of gross domestic product. Based on the success and friable outcome of these programs, various consumers have the option to obtain custom programs that are designed to meet their individual needs (Haley et al., 2020).
2.4 Demand-side management in France
In France, electricity sales are governed by strict policies based on DSM strategies, accurate pricing, and offering customers a range of optional tariffs (Kaluthanthrige and Rajapakse, 2021). Customers are categorized based on their specific needs and preferences, and a combination of static and dynamic tariffs is used to accommodate this diversity. The goal is to ensure that each customer can select a tariff that best suits their circumstances. Electricite De France has been implementing tariff incentives, physical controls, and marketing programs for over 50 years, with the two-part and optional tariffs being in place since 1965. Based on such measures, the daily load factor in France’s power consumers has increased to 90.0%, up from 85.0% in the past (Karunathilake et al., 2020).
2.5 Demand-side management in the UK
Alongside the rest of the developed world, the UK also greatly emphasizes and implements DSM. Various energy utilities offered residential customers attractive time of use (TOU) tariffs and efficient built storage heating appliances to eliminate a significant afternoon dip in the load curve (Forouli et al., 2021). Following 1960s, a double tariff, called ‘the white meter,’ which is similar to the German dual tariff, was also introduced in the UK (Johnson, 2020). DSM activities involve actions taken on the customer’s electric meter either directly or indirectly stimulated by the utility. These actions include load management, strategic conservation, electrification, strategic growth, or deliberately increased market share. In a study by Rehman et al. (2020), DSM has been highlighted as an important approach to attain utilities’ financial soundness. It also emphasized regulatory support as an essential component in determining the success of the DSM policy.
Since mid-1990s, the UK has implemented various DMS policies while considering regulatory, financial, and voluntary approaches. Among other DSM measures, in the UK, the on-site backup generation and storage have historically been used for smoothening the load curve to reduce peaks and thus reduce the requirement of capacity addition (Christensen et al., 2020). The country has been, as such, recognized as a global leader in DSM initiatives over several decades and was also ranked first among the 12 leading economies in 2012 (Cruz et al., 2024).
2.6 DSM measures in Pakistan
Pakistan has belatedly considered DSM efforts, and yet, their effective implantation and outcome must bear some fruit. Following are the key institutional and regulatory endeavors the Pakistan government has undertaken to implement energy efficiency and electricity conservation in the country.
2.6.1 The National Energy Efficiency and Conservation Authority (NEECA)
The National Energy Conservation Center (ENERCON) was established in 1985 under the USAID project and became an autonomous authority in 1986. In 2016, the ENERCON was transformed into the National Energy Efficiency and Conservation Authority (NEECA). It serves as a focal point for energy conservation and energy-efficiency activities in all sectors of the economy, such as industry, agriculture, transport, building, and the domestic sector (Sheikh et al., 2024).
2.6.2 NEECA Act, 2016
The NEECA Act (2016) mandates the creation of authorities to improve energy-efficiency and conservation mechanisms. The authorities created under this bill are the NEECA and provincial energy-efficiency and conservation organization. The NEECA Act (2016) provides the governance framework to facilitate national efforts and reinvigorate wide-scale adoption of sound energy-efficient practices. The act declares the NEECA as the apex agency to coordinate and catalyze efforts to promote conservation in all sectors of the economy. The NEECA has been entrusted with various regulatory responsibilities under the act (Jamil et al. (2022); Raza et al. (2022a)).
2.6.3 NEECA Policy, 2023
This policy identifies interventions to ensure deep-rooted institutionalization, operationalization, and implementation of energy efficiency and conservation in the country. The policy also informs, on the basis of techno-economic analysis, enforcement mechanisms required for the adoption and compliance of energy-efficiency and conservation regulatory measures along with precise guidelines for coordination with the provincial governments (Chughtai et al. (2023); Raza et al. (2022b)).
The NEECA Policy (2023) has some salient regulatory features, including ensuring compliance with minimum energy performance standards and labeling regimes for electric (fans, air conditioners, refrigerators, motors, and LEDs) and gas appliances (geysers, space heaters, and cook stoves) (Asif et al. (2023); Sarang et al. (2024)).
2.6.4 The Building Code of Pakistan (BCP)
The BCP is an essential document that provides guidelines for building design, construction, and safety. It was first published by the Ministry of Housing and Works of the Government of Pakistan in 1986 as a reference for engineers. Although it was not initially enforced as a mandatory requirement, it has been regularly updated to incorporate new and improved studies, techniques, and provisions approved by legislative bodies and stakeholders. In 2013, it was included in the Building Code of Pakistan to make this document more helpful for practitioners to implement energy-efficiency and conservation policies throughout the country (Shah et al., 2021). BCP implementation in letter and spirit would deliver the DSM cause.
2.6.5 Net metering regulations
The National Electric Power Regulatory Authority (NEPRA) has passed the “Distributed Generation and Net Metering Regulations 2015,” thus opening opportunities for net metering. Pakistan is one of the 98.0 countries around the world that have policies for net metering. Net metering is an arrangement where customers who have renewable energy resources installed, such as solar PV, can transfer any extra electricity they generate back to the grid and receive credits on their electric bill from the utility company. Net metering is a policy that can help address the country’s energy deficit by allowing individuals and businesses to generate their own electricity from renewable sources such as solar and wind power, and sell any excess back to the grid (Zahid et al. (2020); Raza et al. (2023d)). These regulations, which encourage DSM, require to be implemented in letter and spirt to accomplish the overall goal of sustainable energy supplies.
2.6.6 Pakistan intended nationally determined contribution
To comply with the decisions of the Paris Agreement (2015), Pakistan has submitted their intended nationally determined contribution to achieve stabilization of GHG in the atmosphere. DSM, including energy efficiency and conservation, is one of the most significant mitigation options to contain emission and attain goals apart from other essential measures (Amjath-Babu et al. (2019); Raza et al. (2022c)). As such, in case of required financial assistance extended under this arrangement, DSM measures could greatly help to reduce the demand and contain the climate change.
It is evident from the above brief literature review that DSM practices are greatly appreciated and implemented across the globe. These practices not only help to reduce the demand but also help to reduce the energy cost and contain the climate change. In case of developing countries such as Pakistan, the DSM is acknowledged, but various constraints do not allow smooth implementation of various DSM policies. This study, as such, is an attempt in this direction to develop DSM scenarios for Pakistan’s electricity sector and analyze their benefits.
3 Research methodology
This analytical study focuses on the electricity supply and consumption system in Pakistan and assesses the DSM potential as well as its benefits for the economy. Low-emissions analysis platform (LEAP) has been used in the study to develop Pakistan’s DSM model for the period 2021–2050, which includes a reference scenario, a DSM scenario, and three alternative DSM scenarios. The alternative scenarios include energy efficiency (EEF), energy conservation (EC), and load management (LOM) scenarios. The model is also used for the forecasting of electricity demand and generation potential considering the energy-saving potential. LEAP facilitates tracking energy resource extraction, production, and consumption in all sectors of the economy. This analysis leads to prioritizing energy efficiency, energy conservation, and load management to institutionalize DSM practices in Pakistan by developing a robust policy framework and implementation mechanism regarding DSM.
3.1 LEAP model
LEAP is a widely used energy-environment modeling tool to develop and assess energy scenarios. The modeling approach of LEAP is based on an accounting framework which tracks energy production and consumption in a particular region under price, available technology, and available resources. LEAP follows a hierarchical tree structure of four different levels like sector and its subsector, a device and end-use (Raza et al., 2022d). The energy required by any sector is equivalent to the product of the activity level and intensity of energy usage. This scenario-based modeling tool also explores how emissions may change in the future under alternative policy settings (e.g., baselines and low emission development scenarios) (Rehan et al., 2023a). It has various modules such as key assumptions, demand, transformation, and resources. In the key assumption module, data such as total population, households, GDP, and similar data have been used (Rehan et al., 2023b). In the demand module, broad sectors such as domestic sector, industrial sector, commercial sector, agriculture sector, and others are created using the sectorial electricity consumption.
In this study model, 2021 is taken as the base year and 2050 is the targeted/end year. Historical data related to electricity generation power plants, such as installed capacity in MW, historical production in thousand GWh, maximum availability, and lifetime, are inputs in the transformation module (Hussain et al., 2022). These data have been used in various expressions of the model to derive various further input data requirements of the mode.
Following the development of Pakistan’s electricity sector model, two forecast scenarios were initially calibrated and tested in this study. The first scenario has been developed according to the government’s existing power plans and power policies, and another scenario as DSM, which considered broad DSM strategies. The fundamental LEAP methodology of this study is illustrated in Figure 2.
Subsequently, three alternative DSM scenarios, namely, energy efficiency (EEF), energy conservation (EC), and load management (LOM) scenarios, were developed to meet the forecasted energy. Key input data used for this modeling exercise are described in the following section.
3.2 Input data for LEAP modeling
At a growth rate of 2.41%, Pakistan’s population is taken as 207.70 million in the base year, whereas at a growth rate of 5.80%, the GDP of 314.58 billion USD is considered. The industrial GDP of 52.31 billion USD at the growth rate of 5.80, the agriculture GDP of 53.56 billion USD at 3.81 growth rates, and commercial GDP of 132.95 billion USD at the growth rate of 6.43% have also been considered in this model. The number of households has been taken as 32.2 million, and the average number of household members has been considered as 6.40 people per house.
The transmission and distribution losses in Pakistan’s power sector stood at 17.13% (Mirjat et al. (2018); Rehman et al. (2017)), which has also been taken as the input in this study model. According to the NEECA, the apex body for planning and implementation of energy efficiency and conservation in Pakistan, the category-wise percentage impact for reduction electricity consumption based on estimated DSM potential is as follows: buildings: 30.0%, industry: 25.0%, commercial: 24.0%, agriculture: 20.0%, and others: 6.0% (Bughio et al., 2021). The electricity consumers in Pakistan are categorized as domestic, commercial, industrial, agricultural, and other services. Historical sector-wise consumption for the period 2014–15 to 2020–21 has been provided in Table 1 (Raza et al. (2022e); Raza et al. (2022f); Bhaagat et al. (2023)).
All projections in the LEAP forecast, for all scenarios, have been initiated and based on the data mentioned in this section.
3.3 Scenario development
In this study, LEAP modeling frameworks have been used to initially construct two broad scenarios, that is, the reference and DSM scenarios, to forecast the energy demand/generation and undertake their analysis based on the data sources provided in the earlier section. Subsequently, three alternative DSM scenarios, viz., energy efficiency (EEF), energy conservation (EC), and load management (LOM), were developed and analyzed. A brief description of each of these scenarios is as follows:
3.3.1 Reference scenario
In the reference scenario, future energy demand and generation has been forecasted based on the ongoing policies of the government of Pakistan.
3.3.2 DSM scenarios
In this study, for a general comparison, following the reference scenario, a broad DSM scenario has been constructed to analyze the benefits of DSM for Pakistan. This followed the development and analysis of three DSM alternative scenarios, viz., energy efficiency (EEF), energy conservation (EC), and load management (LOM). A brief description of each of these alternative scenarios is given as follows:
3.3.2.1 Energy-efficiency (EEF) scenario
Energy efficiency implies smarter technologies to deliver the same outcomes. Energy-efficiency systems, such as replacing an old refrigerator with a more energy-efficient model, enable consumers to use less energy while receiving the same quality of end services. As such, in this scenario, consumers are assumed to be using energy-efficient appliances and conserve energy.
3.3.2.2 Energy conservation (EC) scenario
Energy conservation practices have always been a vital part of managing demand, and it plays a significant role in reducing energy consumption. Smart behavior is needed for energy conservation so as to meet the needs economically. Energy conservation refers to any activity or practice that reduces the use of energy. As an example of energy conservation, the consumers may be encouraged to dry clothes outside in the sunshine instead of consuming energy. In this scenario, as such, energy conservation programs are assumed to be adopted by the end users to avoid excessive consumption, and in return, the adopted programs help in reducing their energy bills; for example, energy can be conserved.
3.3.2.3 Load management (LOM) scenario
In order to modify, control, and adjust the pattern of load/consumption of different consumers in an electric utility, certain interventions, such as valley filling and peak clipping, are introduced to achieve the DSM objective and are termed as load management. The load management enables the utilities to maintain the supply and meet demand economically. It is utilized on all loads and varies on a daily, monthly, or seasonal basis. As the load on the system is variable and not constant, the utilities need to monitor the average and maximum load to avoid abnormal situations and critical power shortfall. These assumptions are considered in this DSM scenario and named as the load management (LOM) scenario.
4 Results and analysis
The results of this study model provide demand and generation projections under the reference and DSM scenarios. Subsequently, considering the assumptions under three alternative DSM scenarios, demand projections under each EEF, EC, and LOM scenario have been undertaken and analyzed. The findings of the model also include the comparative analysis of environmental impact under each scenario.
4.1 Demand projections for reference and DSM scenarios
The forecast of electricity demand for two scenarios developed, viz., the reference and DSM, is shown in Figure 3. The LEAP’s forecasted demand for both scenarios is the same as 115.30 TWh in base year 2021. However, it differs as the DSM measures are considered over the modeling period. The electricity demand under the reference scenario would have subsequently increased from 115.30 TWh in 2021 and become 1,009.80 TWh in 2050. In the meantime, the electricity demand under the DSM scenario would have become 743.50 TWh in 2050, which was 115.3 TWh in the base year.
It is evident that DSM measures have caused a reduced demand, which remains 26.38% less than that in the reference scenario by the end of the modeling period. This significantly reduced demand would require reduced capacity addition and would help in containing the climate change.
4.2 Generation projections under reference and DSM scenarios
The forecast of electricity generation for two scenarios developed, viz., reference and DSM, is shown in Figure 4. The LEAP’s forecasted generation for both scenarios is the same, which is 140.5 TWh in base year 2021. The electricity production to meet the demand under the reference scenario increased significantly from 140.50 TWh in base year 2021 and reached 907.40 TWh during end year 2050, whereas for the DSM scenario, it would have reached 800.20 TWh during 2050.
DSM tends to result in 11.81% decrease in electricity generation compared to the reference scenario in 2050. The model result reveals that the DSM scenario is better than the reference scenario and helpful to contain the demand.
It is evident from the demand and generation forecast analysis of the reference and DSM scenario that the later scenario is greatly advantageous both in containing the demand and requiring lower generation. A decrease of 26.38% in demand is also supported by an 11.8% reduced generation forecast under the DSM scenario. As such, considering economic and environmental advantages altogether, it is greatly feasible to consider DSM endeavors as a key component of electricity planning in the long run.
In addition to the above favorable results of the DSM scenario, this study further takes an insight into DSM approaches and develops three alternative DSM scenarios, viz., energy efficiency (EEF), energy conservation (EC), and load management (LOM), and projects demand under each scenario to analyze the impact of each DSM strategy. Each of alternative DSM scenarios demand forecast is also compared with the reference scenario as follows.
4.3 Demand projections under energy-efficiency scenario
The forecast of electricity demand for the reference and DSM alternative scenario EEF is shown in Figure 5. The electricity demand for both scenarios is the same as 115.30 TWh in base year 2021. However, it differs as the EEF measures/replacement is considered over the modeling period. The electricity demand under the reference scenario is projected to be 1,009.80 TWh and that of the EEF scenario is projected to be 899.50 TWh during end year 2050. It is established from this analysis that the annual average growth rate of the reference scenario is 8.0%, whereas the annual demand growth rate in the EEF scenario is reduced to 7.50%.
The energy-efficiency measure under the EEF scenario results in a 10.92% decrease in electricity demand compared to that in the reference scenario in 2050.
4.4 Demand projections under energy conservation (EC) scenario
The electricity demand forecast for the reference and energy conservation (EC) scenarios is shown in Figure 6. In base year 2021, the forecasted demand for both scenarios is the same as 115.30 TWh. However, it differs in the subsequent years as the EC measures are considered over the modeling period. The annual average growth rate of electricity demand in the reference scenario is 8.00%, which is 7.20% in the EC scenario. The projected electricity demand under the reference scenario in end year 2050 reaches 1,009.80 TWh, whereas under the EC scenario, it remains 831.90 TWh. The result analysis under this scenario reveals that the projected electricity demand under the EC scenario is 17.61% less than that in the reference scenario by the end of modeling period in 2050.
4.5 Demand projections under load management (LOM) scenario
The forecast of electricity demand under the reference scenario and LOM scenario for the modeling period 2021 to 2050 is shown in Figure 7. The forecasted demand for both scenarios is the same, which is 115.30 TWh in base year 2021. However, it differs as the LOM measures are considered over the modeling period. It is found that the annual average growth rate of the reference scenario is 8.00%. However, the annual demand growth rate in the LOM scenario is reduced to 7.40%. The projected electricity demand in end year 2050 under the reference scenario is 1,009.80 TWh, and under the LOM scenario, it is forecasted to be 908.90 TWh.
These results reveal that LOM tends to result in a 9.99% decrease in electricity demand compared to the reference scenario in 2050.
4.6 Comparison of electricity demand under different scenarios
Energy efficiency is a key strategy for reducing energy demand and achieving significant emissions reductions across sectors. Improving energy efficiency in buildings, industry, transportation, and other sectors directly lowers energy consumption and demand. Efficient lighting, appliances, HVAC systems, industrial processes, and vehicles significantly reduce electricity and fuel use. Efficiency is the single most important measure to avoid energy demand growth in the net zero emissions by 2050. As such, considering these measure alternative DSM scenarios of this study appear more promising, as illustrated in Figure 8.
It is evident from Figure 8 that in all alternative DSM scenarios, projected electricity demand substantially reduces over the modeling period. It is established from this modeling effort that the EC scenario is the most feasible DSM scenario to reduce the demand and attain the associated benefits, followed by the EEF and LOM scenarios.
4.7 Environmental impact of the model
Energy is currently a major contributor to Pakistan’s emissions profile, with approximately 46% of total emissions, and its share is expected to increase significantly in the future. This study modeling exercise also reflects the significant impact on GHG emission under different scenarios as per the results provided in Table 2.
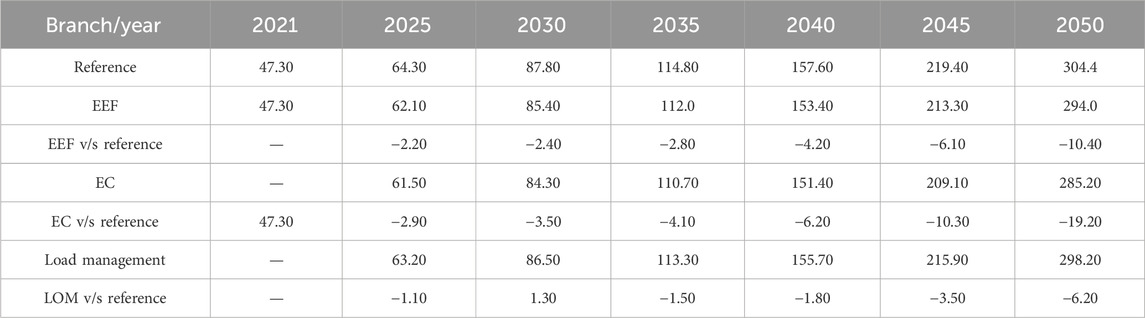
Table 2. Impact of GHG emission reduction under different scenarios (million metric tons CO2 equivalent).
The comparative analyses of carbon emissions under all scenarios are shown in Figure 9. Energy-efficient technologies and practices are a highly effective way to cut greenhouse gas emissions, lower energy demand, save money, and enable the transition to renewable energy. Efficiency delivers emission reductions across sectors, from buildings and transportation to industry and IT.
It is evident that all alternative DSM scenarios are highly feasible from the environmental perspective, and the implementation of these can greatly help in containing the climate change. In summary, the benefits of DSM span environmental, economic, and social domains, making it a win–win–win situation for individuals, businesses, and society.
5 Recommended DSM strategies and plans
The energy modeling results of this study establish categorically that DSM scenarios are most feasible energy pathway Pakistan should undertake as they are advantageous from economic, environment, and social perspectives. Their benefits for individual, business, industrial consumers require a fast-track DSM policy and plan development as well as their effective implementation. This study’s results show that specific DSM measures for domestic and industrial consumers have the saving potential of approximately 30.0% and 25.0%, respectively, as provided in Tables 3, 4.
Based on results analysis and significant findings of this study, it is recommended that Pakistan undertake the following essential crucial DSM steps and strategies:
⁃ Develop a DSM policy framework and implementation mechanism to institutionalize DSM practices in Pakistan.
⁃ Develop public awareness to voluntarily participate in DSM programs and purchase energy-efficient equipment and develop a culture of energy audit in residential, commercial, and industrial consumers.
⁃ Plan sector-wise activities as per financial resources.
⁃ Financial incentive programs for targeted groups such as industries and commercial entities.
⁃ Penalize wasteful use of energy and encourage efficiency by devising a tariff structure.
⁃ Curb sale of inefficient, sub-standard appliances and materials.
⁃ Frame incentive programs for manufacturers and equipment sellers to encourage them to produce and supply energy-efficient appliances, equipment, or services to the end users.
It is anticipated that implementation of these DSM steps and strategies would take Pakistan out of the ongoing energy crises and greatly help to contain the climate change.
6 Conclusion
This study, considering the huge energy saving potential in the power sector of Pakistan, developed a DSM energy model for the sector. Following modeling the baseline reference scenario, and a broad DSM scenario, three alternative DSM scenarios, viz., energy efficiency (EEF), energy conservation (EC), and load management (LOM), were developed and analyzed. The low emission analysis platform (LEAP) modeling framework has been employed in this study to undertake the DSM scenario analysis for the study period 2021–2050. This study’s results reveal that DSM scenarios are superior to the reference scenario. The DSM scenarios provide alternate pathways to overcome the ongoing electricity crisis. DSM scenario results suggest a 26.38% decrease in electricity demand compared to the reference scenario in 2050. It also results in an 11.81% decrease in electricity generation compared to the reference scenario in 2050. The alternative DSM scenarios also outperform the reference scenario. In 2050, the EC scenario will reduce consumption by 178.0 TWh and GHG emissions by 19.20 million metric tons, the EEF scenario will reduce consumption by 110.30 TWh and GHG emissions by 10.04 million metric tons, and the LOM scenario suggests reduced consumption by 101.0 TWh and GHG emissions by 6.20 million metric tons. All three alternative DSM scenarios are technically and economically feasible, with a track record of success on a global scale.
However, DSM must be institutionalized by building a robust regulatory framework and execution mechanism at the government and utility levels. In Pakistan, demand-side management is non-existent in practice; however, there is substantial saving potential of 20% across all sectors. If this is achieved, it would correspond to a substantial reduction in the country’s oil imports. Continued supply–demand deficit, mounting circular debt, escalating losses, non-availability of fuels, lack of incentives, and weak T&D infrastructure and constraints are some indications of the dismal situation resulting in load shedding and frequent interruptions. Lack of efficiency and lack of technology are the major handicaps to overcome the energy crisis.
This study concludes that at minimum, the DSM measures provided in Table 5 require systematic implantation to attain the much-desired results of DSM for the power sectors of Pakistan.
It is, therefore, concluded that without a complete shift toward energy conservation and efficiency, all efforts to mitigate energy crises may be futile. It is thus recommended that to draw a firm line of action, there is a need to develop a “DSM policy framework and implementation mechanism” to regularly monitor the measures and achieve tangible results based on the DSM model, which would result in energy savings through various DSM interventions on the existing system and future projections.
Data availability statement
The original contributions presented in the study are included in the article/supplementary materials; further inquiries can be directed to the corresponding author.
Author contributions
AC: writing–original draft. MU: writing–review and editing. NH: writing–review and editing. FS: writing–review and editing. SA: writing–review and editing.
Funding
The author(s) declare that no financial support was received for the research, authorship, and/or publication of this article.
Acknowledgments
The authors would like to thank Muhammad Amir Raza for suggesting various improvements in the manuscript and Mehran University of Engineering and Technology, Jamshoro, Pakistan, for facilitating this research work.
Conflict of interest
The authors declare that the research was conducted in the absence of any commercial or financial relationships that could be construed as a potential conflict of interest.
Publisher’s note
All claims expressed in this article are solely those of the authors and do not necessarily represent those of their affiliated organizations, or those of the publisher, the editors, and the reviewers. Any product that may be evaluated in this article, or claim that may be made by its manufacturer, is not guaranteed or endorsed by the publisher.
References
Abbas, T., Ashraf, M. M., and Malik, T. N. (2023). Least cost generation expansion planning considering renewable energy resources using sine cosine algorithm. Arabian J. Sci. Eng. 48, 6185–6203. doi:10.1007/s13369-022-07303-5
Abdullah, F. B., Iqbal, R., Hyder, S. I., and Jawaid, M. (2020). Energy security indicators for Pakistan: an integrated approach. Renew. Sustain. Energy Rev. 133, 110122. doi:10.1016/j.rser.2020.110122
Abeykoon, C., McMillan, A., and Nguyen, B. K. (2021). Energy efficiency in extrusion-related polymer processing: a review of state of the art and potential efficiency improvements. Renew. Sustain. Energy Rev. 147, 111219. doi:10.1016/j.rser.2021.111219
Aghahosseini, A., Solomon, A., Breyer, C., Pregger, T., Simon, S., Strachan, P., et al. (2023). Energy system transition pathways to meet the global electricity demand for ambitious climate targets and cost competitiveness. Appl. energy 331, 120401. doi:10.1016/j.apenergy.2022.120401
Ahmad, T., Zhang, H., and Yan, B. (2020). A review on renewable energy and electricity requirement forecasting models for smart grid and buildings. Sustain. Cities Soc. 55, 102052. doi:10.1016/j.scs.2020.102052
Amjath-Babu, T. S., Aggarwal, P. K., and Vermeulen, S. (2019). Climate action for food security in South Asia? Analyzing the role of agriculture in nationally determined contributions to the Paris agreement. Clim. Policy 19, 283–298. doi:10.1080/14693062.2018.1501329
Arihan, C. (2021). Oil crisis (oil price revolution) of 1973 and the United States’ response to the crisis: the international energy agency. J. Manag. Policy Pract. 22. doi:10.33423/jmpp.v22i1.4176
Asif, M. H., Zhongfu, T., Irfan, M., Ahmad, B., and Ali, M. (2023). Assessing eco-label knowledge and sustainable consumption behavior in energy sector of Pakistan: an environmental sustainability paradigm. Environ. Sci. Pollut. Res. 30, 41319–41332. doi:10.1007/s11356-023-25262-8
Baloch, Z. A., Tan, Q., Kamran, H. W., Nawaz, M. A., Albashar, G., and Hameed, J. (2021). A multi-perspective assessment approach of renewable energy production: policy perspective analysis. Environ. Dev. Sustain. 24, 2164–2192. doi:10.1007/s10668-021-01524-8
Bhaagat, M. A., Mirjat, N. H., Khatri, S. A., Mahar, A., and Raza, M. A. (2023). Demand side management for the energy-efficient future of Pakistan using a low emission analysis Platform. Eng. Proc. 46, 28. doi:10.3390/engproc2023046028
Bughio, M., Khan, M. S., Mahar, W. A., and Schuetze, T. (2021). Impact of passive energy efficiency measures on cooling energy demand in an architectural campus building in Karachi, Pakistan. Sustainability 13, 7251. doi:10.3390/su13137251
Christensen, M. H., Li, R., and Pinson, P. (2020). Demand side management of heat in smart homes: living-lab experiments. Energy 195, 116993. doi:10.1016/j.energy.2020.116993
Chughtai, A., Uqaili, M. A., Mirjat, N. H., and Shaikh, F. U. (2023). Diagnosis of energy crisis of Pakistan and assessment of DSM as viable solution. Eng. Proc. 46, 35. doi:10.3390/engproc2023046035
Creutzig, F., Niamir, L., Bai, X., Callaghan, M., Cullen, J., Díaz-José, J., et al. (2022). Demand-side solutions to climate change mitigation consistent with high levels of well-being. Nat. Clim. Change 12, 36–46. doi:10.1038/s41558-021-01219-y
Cruz, C., Tostado-Véliz, M., Palomar, E., and Bravo, I. (2024). Pattern-driven behaviour for demand-side management: an analysis of appliance use. Energy Build. 308, 113988. doi:10.1016/j.enbuild.2024.113988
Forouli, A., Bakirtzis, E. A., Papazoglou, G., Oureilidis, K., Gkountis, V., Candido, L., et al. (2021). Assessment of demand side flexibility in european electricity markets: a country level review. Energies 14, 2324. doi:10.3390/en14082324
Golmohamadi, H. (2022). Demand-side management in industrial sector: a review of heavy industries. Renew. Sustain. Energy Rev. 156, 111963. doi:10.1016/j.rser.2021.111963
Haley, B., Gaede, J., Winfield, M., and Love, P. (2020). From utility demand side management to low-carbon transitions: opportunities and challenges for energy efficiency governance in a new era. Energy Res. and Soc. Sci. 59, 101312. doi:10.1016/j.erss.2019.101312
Harsh, P., and Das, D. (2021). Energy management in microgrid using incentive-based demand response and reconfigured network considering uncertainties in renewable energy sources. Sustain. Energy Technol. Assessments 46, 101225. doi:10.1016/j.seta.2021.101225
Hosseinnia, H., Nazarpour, D., and Talavat, V. (2018). Benefit maximization of demand side management operator (DSMO) and private investor in a distribution network. Sustain. cities Soc. 40, 625–637. doi:10.1016/j.scs.2018.04.022
Huang, M.-T., and Zhai, P.-M. (2021). Achieving Paris Agreement temperature goals requires carbon neutrality by middle century with far-reaching transitions in the whole society. Adv. Clim. Change Res. 12, 281–286. doi:10.1016/j.accre.2021.03.004
Hulio, Z. H., Jiang, W., and Chandio, G. S. (2022). Power policies, challenges, and recommendations of renewable resource assessment in Pakistan. Energy Explor. and Exploitation 40, 947–976. doi:10.1177/01445987211064678
Hussain, A., Raza, M. A., Aman, M., Shahid, M., and Ara, D. (2022). Energy demand projection and economy nexus of Pakistan. Quaid-e-Awam Univ. Res. J. Eng. Sci. Technol. 20, 138–144. doi:10.3934/energy.2023013
Jamil, M. H., Ullah, K., Saleem, N., Abbas, F., and Khalid, H. A. (2022). Did the restructuring of the electricity generation sector increase social welfare in Pakistan? Renew. Sustain. Energy Rev. 157, 112017. doi:10.1016/j.rser.2021.112017
Johnson, C. (2020). Is demand side response a woman's work? Domestic labour and electricity shifting in low income homes in the United Kingdom. Energy Res. and Soc. Sci. 68, 101558. doi:10.1016/j.erss.2020.101558
Kaluthanthrige, R., and Rajapakse, A. D. (2021). Demand response integrated day-ahead energy management strategy for remote off-grid hybrid renewable energy systems. Int. J. Electr. power and energy Syst. 129, 106731. doi:10.1016/j.ijepes.2020.106731
Kanakadhurga, D., and Prabaharan, N. (2022). Demand side management in microgrid: a critical review of key issues and recent trends. Renew. Sustain. Energy Rev. 156, 111915. doi:10.1016/j.rser.2021.111915
Karunathilake, H., Nahiduzzaman, K. M., Prabatha, T., Hewage, K., Sadiq, R., Alam, S., et al. (2020). The nexus of climate change and increasing demand for energy: a policy deliberation from the Canadian context. Dyn. Energy, Environ. Econ. A Sustain. Perspective, 263–294. doi:10.1007/978-3-030-43578-3_13
Khan, M., Raza, M. A., Jumani, T. A., Mirsaeidi, S., Ali, A., Abbas, G., et al. (2023). Modeling of intelligent controllers for solar photovoltaic system under varying irradiation conditions. Front. Energy Res. 11, 1288486. doi:10.3389/fenrg.2023.1288486
Kiptoo, M. K., Adewuyi, O. B., Lotfy, M. E., Ibrahimi, A. M., and Senjyu, T. (2020). Harnessing demand-side management benefit towards achieving a 100% renewable energy microgrid. Energy Rep. 6, 680–685. doi:10.1016/j.egyr.2019.11.137
Li, P.-H., and Pye, S. (2018). Assessing the benefits of demand-side flexibility in residential and transport sectors from an integrated energy systems perspective. Appl. energy 228, 965–979. doi:10.1016/j.apenergy.2018.06.153
MacKinnon, K. R., Grace, D., Ng, S. L., Sicchia, S. R., and Ross, L. E. (2020). “I don’t think they thought I was ready”: how pre-transition assessments create care inequities for trans people with complex mental health in Canada. Int. J. Ment. Health 49, 56–80. doi:10.1080/00207411.2019.1711328
Masoomi, M., Panahi, M., and Samadi, R. (2020). Scenarios evaluation on the greenhouse gases emission reduction potential in Iran’s thermal power plants based on the LEAP model. Environ. Monit. Assess. 192, 235. doi:10.1007/s10661-020-8196-3
Meyabadi, A. F., and Deihimi, M. H. (2017). A review of demand-side management: reconsidering theoretical framework. Renew. Sustain. Energy Rev. 80, 367–379. doi:10.1016/j.rser.2017.05.207
Mirjat, N. H., Uqaili, M. A., Harijan, K., Valasai, G. D., Shaikh, F., and Waris, M. (2017). A review of energy and power planning and policies of Pakistan. Renew. Sustain. Energy Rev. 79, 110–127. doi:10.1016/j.rser.2017.05.040
Mirjat, N. H., Uqaili, M. A., Harijan, K., Walasai, G. D., Mondal, M. A. H., and Sahin, H. (2018). Long-term electricity demand forecast and supply side scenarios for Pakistan (2015–2050): a LEAP model application for policy analysis. Energy 165, 512–526. doi:10.1016/j.energy.2018.10.012
Niamir, L., Ivanova, O., Filatova, T., Voinov, A., and Bressers, H. (2020). Demand-side solutions for climate mitigation: bottom-up drivers of household energy behavior change in The Netherlands and Spain. Energy Res. and Soc. Sci. 62, 101356. doi:10.1016/j.erss.2019.101356
Patnam, B. S. K., and Pindoriya, N. M. (2021). Demand response in consumer-Centric electricity market: mathematical models and optimization problems. Electr. Power Syst. Res. 193, 106923. doi:10.1016/j.epsr.2020.106923
Raskin, J. D., Maynard, D., and Gayle, M. C. (2022). Psychologist attitudes toward DSM-5 and its alternatives. Prof. Psychol. Res. Pract. 53, 553–563. doi:10.1037/pro0000480
Raza, M. A., Aman, M., Abbas, G., Soomro, S. A., Yousef, A., Touti, E., et al. (2024). Managing the low carbon transition pathways through solid waste electricity. Sci. Rep. 14, 5490. doi:10.1038/s41598-024-56167-2
Raza, M. A., Aman, M., Abro, A. G., Shahid, M., Ara, D., Waseer, T. A., et al. (2023a). Modelling and development of sustainable energy systems. AIMS Energy 11, 256–270. doi:10.3934/energy.2023014
Raza, M. A., Aman, M., Abro, A. G., Shahid, M., Ara, D., Waseer, T. A., et al. (2023b). The role of techno-economic factors for net zero carbon emissions in Pakistan. AIMS Energy 11, 239–255. doi:10.3934/energy.2023013
Raza, M. A., Aman, M., Abro, A. G., Shahid, M., Ara, D., Waseer, T. A., et al. (2023c). A simulation model of climate policy analysis for sustainable environment in Pakistan. Environ. Prog. and Sustain. Energy 42, e14144. doi:10.1002/ep.14144
Raza, M. A., Aman, M. M., Abro, A. G., Tunio, M. A., Khatri, K. L., and Shahid, M. (2022a). Challenges and potentials of implementing a smart grid for Pakistan’s electric network. Energy Strategy Rev. 43, 100941. doi:10.1016/j.esr.2022.100941
Raza, M. A., Aman, M. M., Rajpar, A. H., Bashir, M. B. A., and Jumani, T. A. (2022b). Towards achieving 100% renewable energy supply for sustainable climate change in Pakistan. Sustainability 14, 16547. doi:10.3390/su142416547
Raza, M. A., Aman, M. M., Tunio, N. A., Soomro, S. A., Shahid, M., Ara, D., et al. (2023d). Energy transition through bioelectricity in Pakistan: implications for limiting global mean temperature below 1.5°C. Environ. Prog. and Sustain. Energy 42, e14189. doi:10.1002/ep.14189
Raza, M. A., Khatri, K. L., Haque, M. I. U., Shahid, M., Rafique, K., and Waseer, T. A. (2022f). Holistic and scientific approach to the development of sustainable energy policy framework for energy security in Pakistan. Energy Rep. 8, 4282–4302. doi:10.1016/j.egyr.2022.03.044
Raza, M. A., Khatri, K. L., and Hussain, A. (2022c). Transition from fossilized to defossilized energy system in Pakistan. Renew. Energy 190, 19–29. doi:10.1016/j.renene.2022.03.059
Raza, M. A., Khatri, K. L., Israr, A., Haque, M. I. U., Ahmed, M., Rafique, K., et al. (2022d). Energy demand and production forecasting in Pakistan. Energy Strategy Rev. 39, 100788. doi:10.1016/j.esr.2021.100788
Raza, M. A., Khatri, K. L., Memon, M. A., Rafique, K., Haque, M. I. U., and Mirjat, N. H. (2022e). Exploitation of Thar coal field for power generation in Pakistan: a way forward to sustainable energy future. Energy Explor. and Exploitation 40, 1173–1196. doi:10.1177/01445987221082190
Rehan, M., Raza, M. A., Abro, A. G., Aman, M., Ismail, I. M. I., Nizami, A. S., et al. (2023b). A sustainable use of biomass for electrical energy harvesting using distributed generation systems. Energy 278, 128036. doi:10.1016/j.energy.2023.128036
Rehan, M., Raza, M. A., Aman, M., Abro, A. G., Ismail, I. M. I., Munir, S., et al. (2023a). Untapping the potential of bioenergy for achieving sustainable energy future in Pakistan. Energy 275, 127472. doi:10.1016/j.energy.2023.127472
Rehman, S., Habib, H. U. R., Wang, S., Büker, M. S., Alhems, L. M., and Al Garni, H. Z. (2020). Optimal design and model predictive control of standalone HRES: a real case study for residential demand side management. IEEE Access 8, 29767–29814. doi:10.1109/access.2020.2972302
Rehman, S. A. U., Cai, Y., Fazal, R., Das Walasai, G., and Mirjat, N. H. (2017). An integrated modeling approach for forecasting long-term energy demand in Pakistan. Energies 10, 1868. doi:10.3390/en10111868
Sarang, S. A., Raza, M. A., Panhwar, M., Khan, M., Abbas, G., Touti, E., et al. (2024). Maximizing solar power generation through conventional and digital MPPT techniques: a comparative analysis. Sci. Rep. 14, 8944. doi:10.1038/s41598-024-59776-z
Schramm, L. (2024). The neglected integration crisis: France, Germany and lacking European Co-operation during the 1973/1974 oil shock. JCMS J. Common Mark. Stud. 62, 583–602. doi:10.1111/jcms.13518
Shah, B. A., Sadiq, M. M., Memon, S. A., and Rehman, S. K. U. (2021). Assessment of the seismicity of Peshawar region in line with the historical data and modern building codes (ASCE-07 and IBC-2006). J. Earthq. Eng. 25, 1826–1850. doi:10.1080/13632469.2019.1605315
Sheikh, N., Laverge, J., and Delghust, M. (2024). Critical analysis of institutional and regulatory framework for building stock energy efficiency and transition in Pakistan. Environ. Sci. and Sustain. Dev., 32–41. doi:10.21625/essd.v9i1.1066
Williams, B., Bishop, D., Gallardo, P., and Chase, J. G. (2023). Demand side management in industrial, commercial, and residential sectors: a review of constraints and considerations. Energies 16, 5155. doi:10.3390/en16135155
Keywords: demand-side management, energy efficiency, energy conservation, load management, energy policy
Citation: Chughtai A, Uqaili MA, Hussain Mirjat N, Shaikh F and Khatri SA (2024) Demand-side management scenario analysis for the energy-efficient future of Pakistan: Bridging the gap between market interests and national priorities. Front. Energy Res. 12:1391973. doi: 10.3389/fenrg.2024.1391973
Received: 26 February 2024; Accepted: 30 August 2024;
Published: 17 September 2024.
Edited by:
Fei Ding, National Renewable Energy Laboratory (DOE), United StatesReviewed by:
Sajid Qazi, Ghent University, BelgiumGodwin Norense Osarumwense Asemota, University of Rwanda, Rwanda
Copyright © 2024 Chughtai, Uqaili, Hussain Mirjat, Shaikh and Khatri. This is an open-access article distributed under the terms of the Creative Commons Attribution License (CC BY). The use, distribution or reproduction in other forums is permitted, provided the original author(s) and the copyright owner(s) are credited and that the original publication in this journal is cited, in accordance with accepted academic practice. No use, distribution or reproduction is permitted which does not comply with these terms.
*Correspondence: Arshad Chughtai, ZW5nci5hcnNoYWRjaHVnaHRhaUBnbWFpbC5jb20=