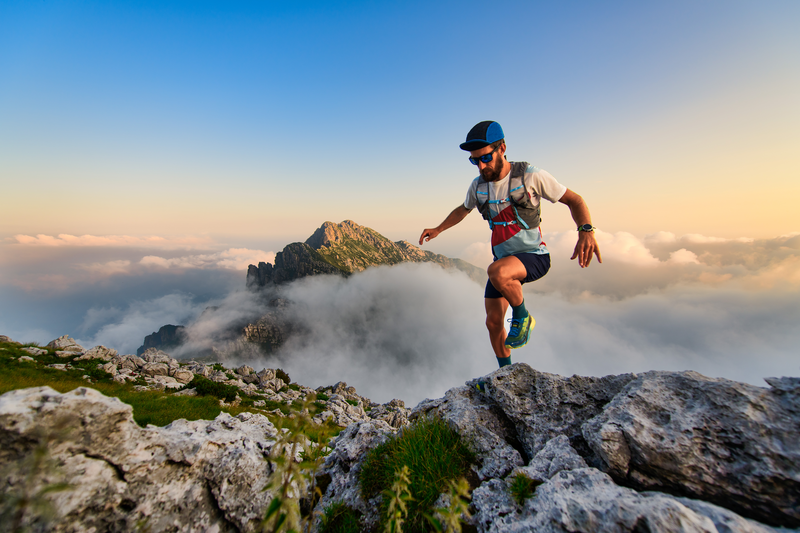
94% of researchers rate our articles as excellent or good
Learn more about the work of our research integrity team to safeguard the quality of each article we publish.
Find out more
ORIGINAL RESEARCH article
Front. Energy Res. , 02 February 2024
Sec. Sustainable Energy Systems
Volume 12 - 2024 | https://doi.org/10.3389/fenrg.2024.1362957
This article is part of the Research Topic Sustainable Road Infrastructure: Technologies and Assessments View all 14 articles
Highways consume a significant amount of electrical energy annually, especially in remote desert regions where the cost of electricity is high. This research explores the utilization of natural resources along desert highways to establish hybrid energy generation systems for service areas. Three service areas along the desert highway in northern Xinjiang, China, serve as case studies. To assess the feasibility of hybrid energy generation systems in these service areas, meteorological data for the three locations were obtained from the NASA platform. The HOMER Pro software was employed for technical, economic, and environmental analyses of the systems. The results indicate the feasibility of Photovoltaic (PV)/Wind/Battery hybrid energy systems in the Huanghuagou, Kelameili, and Wujiaqu service areas. The application of these hybrid energy generation systems across the three service areas could provide 3,349,557 kWh of electrical energy annually for the desert highway. Sensitivity analysis reveals that the Net Present Cost (NPC) and Cost of Energy (COE) values decrease with increasing radiation levels, while NPC shows an increasing trend with growing load demand, and COE exhibits a decreasing trend. Among the three regions, Wujiaqu demonstrates the highest economic viability, with a COE of $0.34/kWh and an NPC of $3,141,641/kWh. Furthermore, Wujiaqu exhibits the lowest environmental impact, with CO2 emissions of 198,387 kg/yr, SO2 emissions of 493 kg/yr, and NOx emissions of 1,711 kg/yr.
The development of highways significantly impacts a nation’s economic growth. Highways consume a substantial amount of electrical energy annually, and typically, the power supply for highways is sourced from nearby cities to ensure reliable electricity. However, for highways located in remote areas, particularly desert highways, the cost of electricity transportation is prohibitively high. Addressing the power supply challenges for desert highways has become an urgent matter. Desert regions, where highways are often situated, possess immense potential for energy asset utilization. Exploring the renewable natural resources such as wind and solar energy within the road corridor of desert highways could offer a strategic solution to alleviate the energy challenges faced by the transportation system. The electrification of infrastructure assets in desert highway areas holds significant promise for mitigating energy-related concerns in the transportation sector.
In recent years, the construction technology of highways has been continuously developing (Jiang et al., 2022; Sun et al., 2023a; Sun et al., 2023b; Wang et al., 2023; Shi et al., 2024), while new energy technologies are also constantly being updated and iterated. In the field of renewable energy utilization on expressways, (Zhang et al., 2022), assessed the photovoltaic road potential for electricity generation in Chinese highways. The research findings indicate that the installed capacity of Chinese highways is 700.85 GW, with the capability to generate 629.06 TWh. (Yuan et al., 2023). Research proposes a technology method for energy collection using temperature differences on highway road surfaces. (Kim et al., 2018). studied the establishment of site selection criteria for photovoltaic (PV) power generation projects on Korean national roads. They conducted a survey of spatial information data for unused roads, determining the feasibility of potential project locations selected using these criteria for future solar road projects. Jiang et al. (2024) proposed a multi indicator evaluation method based on Analytic Hierarchy Process (AHP) and Geographic Information System (GIS) to fully utilize solar energy resources along highways. (Ahmad et al., 2020) investigated the design method of roadside vertical axis wind turbine generators and conducted experimental validation. The results show that the equipment can be installed on one side of the road, providing clean and affordable energy for road lighting. It is evident that individual renewable energy sources such as wind power and photovoltaics have broad prospects for application in the transportation sector. However, the main drawback of utilizing a single renewable energy source is the intermittent nature of its use, significantly reducing the reliability of renewable energy systems. To enhance the reliability of power supply and reduce economic costs, this study considers combining renewable energy with batteries and diesel generators to create a Hybrid Renewable Energy System (HRES). This system aims to supply power to critical loads at important energy nodes on highways. Compared to a single renewable energy system, a hybrid energy system has advantages such as high reliability and low cost (Ribó-Pérez et al., 2021).
In terms of technical and economic analysis, (El-houari et al., 2021), conducted a simulation of technical, economic, and environmental analyses of hybrid renewable energy systems in 24 selected cities in Morocco. They demonstrated the social and economic benefits of promoting the use of hybrid energy systems in remote towns and villages on the African continent (Haghighat Mamaghani et al., 2016). employed Net Present Cost (NPC), initial capital cost, and Levelized Cost of Energy (COE) as economic indicators to analyze the technical and economic feasibility of hybrid energy systems in three small rural communities in Colombia. They proposed different configuration combinations of diesel generators, solar photovoltaic modules, and wind power equipment (Rezaei et al., 2021). and others studied off-grid hybrid renewable energy systems, analyzing the impact of changes in discount rates and inflation rates on system costs (Uwineza et al., 2021). conducted a feasibility analysis of mixed energy resources on Popova Island, studying the influence of discount rates and fuel costs on hybrid energy systems, providing references for decision-makers (Vendoti et al., 2020). and others utilized renewable resources in rural locations to find optimal configuration combinations that meet power loads, constructing a hybrid energy generation model. The research results indicate that a combination of photovoltaic, wind, biogas biomass, diesel generators, and batteries is an economical and reliable solution (Coban et al., 2022). performed a feasibility analysis of a wind and photovoltaic hybrid energy generation system in selected locations in Somalia, comparing off-grid and grid-connected modes.
During the construction process of highways, harmful gas emissions will be generated. Therefore, in the feasibility analysis stage of new energy construction, it is also necessary to analyze environmental emissions (Renken et al., 2018; Büchner et al., 2019; Walther et al., 2019; Wu et al., 2024). In terms of environmental impact, (Vergara-Zambrano et al., 2022), proposed a design method for a solar-biogas hybrid renewable energy system that can help reduce environmental pollutant emissions by 30%–80%. (Razmjoo et al., 2021). studied a hybrid power system composed of photovoltaics, wind, diesel generators, and batteries. The results indicate that the renewable rate of this hybrid system exceeds 72%, resulting in a reduction of over 2000 kg of CO2 emissions per household annually. (El-houari et al., 2020). investigated a mixed energy system incorporating renewable sources such as solar, wind, and biomass, leading to an annual reduction of 26.48 tons of CO2 emissions. (Das et al., 2021). researched a photovoltaic and wind energy hybrid system using different energy storage technologies, demonstrating that the hybrid energy system can reduce carbon dioxide emissions by 134,183.5 kg annually.
The focus of this study is to analyze the technical and economic feasibility of hybrid energy systems in service areas of desert highways, using three service areas along a desert highway in northern Xinjiang, China, as a case study. The study optimizes the best configuration of renewable energy for critical energy nodes in the road domain. In off-grid mode, using Net Present Cost (NPC) and Levelized Energy Cost (COE) as economic indicators, different system configuration parameters are optimized through simulation, analyzing the economics of service areas in different regions. Additionally, sensitivity analysis of hybrid energy systems in different regions is conducted, considering variables such as radiation intensity and the impact of load demand growth on the system.
The hybrid energy simulation software used in this study is HOMER Pro, designed by the National Renewable Energy Laboratory (NREL) in the United States. This simulation tool is primarily utilized for optimizing hybrid power systems, conducting feasibility analyses, and performing technical, economic, and sensitivity analyses. Figure 1 illustrates the feasibility analysis process for the hybrid energy system in service areas along a desert highway.
Initially, the location of the desert service area where the hybrid energy system is to be constructed is selected. The available types of green energy in the region are analyzed, and meteorological data for the area, as well as technical and economic parameters for the selected green energy equipment, are obtained. Subsequently, through simulation and optimization calculations using HOMER Pro software, the best hybrid energy configuration is evaluated based on two crucial economic output parameters: Net Present Cost (NPC) and Levelized Cost of Energy (COE), considering environmental and technical factors. Finally, a sensitivity analysis of the hybrid energy system is conducted for uncertain variables.
The Net Present Cost (NPC) is a crucial economic parameter for assessing hybrid energy systems. The formula for calculating the total Net Present Cost is as follows (Jahangir et al., 2020; Sifakis et al., 2021; Al-Buraiki and Al-Sharafi, 2022; Ma et al., 2022b; Das et al., 2022):
Where TAC is the Total Annualized Cost (the sum of all the annualized costs for each system component), and CRF is the Capital Recovery Factor. The calculation formula is as follows (Ramesh and Saini, 2020; Ma et al., 2022a; Demirci et al., 2022):
Where n is the project’s lifecycle in years, and q is the annual interest rate.
The Levelized Cost of Energy (COE) is the average cost of electricity produced by the hybrid energy system per kilowatt-hour. The calculation of COE involves dividing the annualized cost of electricity generation (total annualized cost minus the cost for servicing thermal loads) by the total electrical load served. The COE calculation formula is as follows (Toopshekan et al., 2020; Al-Najjar et al., 2022; Ang et al., 2022; Khan et al., 2022):
Where TAC is the annualized cost of electricity generation; EAC, EDC, and Edef represent the total amounts of alternating current (AC), direct current (DC), and deferred load (kWh/year), respectively.
NPC and COE are used as evaluation indicators for hybrid energy systems. The smaller the NPC and COE, the better the economic efficiency of the hybrid energy system. The lower the investment cost of establishing hybrid energy, the greater the benefits obtained.
The selected desert highway in this study is located in the Gurbantünggüt Desert region of Xinjiang, China, with the highway overall running in a north-south direction. As illustrated in Figure 2A, the chosen service areas along the highway, from north to south, are Huanghuagou, Kelameili, and Wujiaqu. The geographical and climatic data for the selected region are presented in Table 1.
The solar radiation data used in this study are sourced from the NASA database (July 1983- June 2005). Figure 2B illustrates the monthly average solar radiation and clarity index data for the selected highway service areas. The solar radiation in the regions of Huanghuagou, Kelameili, and Wujiaqu ranges between 1.37 kWh/m2/d and 6.42 kWh/m2/d. The annual average solar radiation values for Huanghuagou, Kelameili, and Wujiaqu are 3.94, 4.09, and 4.08 kWh/m2/d. From the graph, it can be seen that solar radiation has larger data in May and June, while the radiation data in January and December is smaller. This is because in summer, the Sun is closer to the region, resulting in a significant increase in radiation.
The available annual average wind speeds within the study location are depicted in Figure 2C. The maximum wind speeds for Huanghuagou, Kelameili, and Wujiaqu occur in April at 5.19 m/s, while the minimum speeds are observed in January at 3.05 m/s. The annual average wind speeds for Huanghuagou, Kelameili, and Wujiaqu are 4.23, 4.51, and 3.87 m/s, respectively. Kelameili experiences the highest annual average wind speeds from January to April and July to December, while Huanghuagou peaks from June to July. Wujiaqu consistently maintains lower average wind speeds than the other regions. Altitude information affects the magnitude of wind speed, and it is also an important parameter in hybrid energy simulation analysis. Therefore, when obtaining data, it is also necessary to collect altitude information of the area where it is located.
Environmental temperature data significantly impact the actual output power of PV modules. The monthly average environmental temperature data for the three service areas are illustrated in Figure 2D. The highest temperature in Huanghuagou is 25.57°C in July, with a minimum of −14.13°C in January. Kelameili experiences a maximum temperature of 27.81°C in July, with a minimum of −10.07°C in January. Wujiaqu reaches a maximum temperature of 26.46°C in July, with a minimum of −8.19°C in January.
The desert highway service area hybrid energy system is comprised of PV modules, wind turbines, diesel engines, and batteries. Photovoltaic components and batteries are connected to the DC bus, while diesel generators and wind turbines are connected to the AC bus. The electrical load for highway use is connected to the AC bus, and bidirectional converters facilitate the connection between the DC and AC buses, as shown in Figure 3A.
Based on the design data for the desert highway, the annual energy demand for Huanghuagou, Kelameili, and Wujiaqu in 2022 was obtained, and the power consumption was assessed. Based on the collected load data, combined with the time of energy consumption in different service areas and the power of energy consuming equipment, the corresponding energy consumption loads for different areas can be calculated. According to the collected load data, the daily average power consumption for Huanghuagou, Kelameili, and Wujiaqu service areas is 10.5, 10.5, and 5.79 MWh, respectively. The main reason for the different load data in different regions is that there is inconsistency in the number of electrical equipment used in different areas. Compared to Huanghuagou and Kela Meili, Wujiaqu has the smallest electrical equipment. We obtained these data through design documents. The service area is a critical energy node for the highway, encompassing a mix of small industrial buildings for refueling, charging, monitoring, communication, business (including supermarkets and public restrooms), and residential purposes. Among various load types, monitoring and lighting are essential loads for the daily operation of the service area. Therefore, this study considers monitoring and lighting as important loads, intending to harness natural resources within the road domain to provide green energy for these loads, constructing a hybrid energy system. The daily average power consumption for monitoring and lighting in Huanghuagou, Kelameili, and Wujiaqu service areas is 1920, 1920, and 2,880 kWh, respectively, as shown in Table 2.
In the HOMER Pro software, input relevant data for the Huanghuagou, Kelameili, and Wujiaqu highway service areas to conduct an analysis of the hybrid energy system’s capacity, environmental impact, and economic considerations. The hybrid energy system diagrams for the three service areas are depicted in Figures 3B–D, respectively.
Photovoltaic Modules: The final power generated by solar photovoltaics is influenced by various factors such as solar irradiance, characteristics of the photovoltaic cells (e.g., conversion efficiency, placement of photovoltaic panels, and derating factors), and the temperature of the photovoltaic cells. The equation used to calculate the output of a photovoltaic array is as follows (Oko. et al., 2012):
Where RT is the solar radiation incident on the site’s photovoltaic array (kW/m2); RT, STC is the solar irradiance at Standard Test Conditions (STC) (1 kW/m2); dPV is the derating factor for PV (%); YPV is the rated capacity of the photovoltaic array; αPV is the power temperature coefficient, 0.44 (%/°C), for the selected photovoltaic modules; TC is the temperature of the photovoltaic cells in °C; TC, STC is the temperature of the photovoltaic cells at STC (25°C).
Wind Components: Wind turbines can convert kinetic energy into electrical energy. The electricity generated by a wind power system depends on factors such as air density, wind direction, site height, nearby topography, site temperature, and other elements. An arc is used to simulate the wind turbine. The power curve is a graph illustrating the relationship between energy output and wind speed at a specific hub height. The power output of a wind turbine under standard temperature and pressure conditions is expressed as (Sharma et al., 2022):
Where pa is the air density at standard pressure and temperature (1.225 kg/m³), A is the cross-sectional area of the wind flow (m2), Uhub is the wind speed at the hub height of the wind turbine (m/s), Uan is the wind speed at the anemometer height (m/s), Zhub is the hub height of the wind turbine (m), Zan is the anemometer height (m), vp is the power-law exponent, q is the actual air density (kg/m³). This equation represents the output power equation of the wind turbine at standard pressure and temperature. The actual performance of the wind turbine is expressed as:
Battery Components: As the hybrid energy system constructed in this study operates as an off-grid model, it is necessary to store excess electricity when the system’s capacity exceeds demand or when the energy load requirements decrease. Additionally, when the power generated by the hybrid energy system does not meet the demand for load electricity, energy storage batteries are essential. The battery selected for this study is a lithium iron phosphate battery, which uses lithium iron phosphate (LiFePO4) as the positive electrode material and carbon as the negative electrode material in a lithium-ion battery. Lithium iron phosphate batteries have advantages such as high operating voltage, large energy density, long cycle life, good safety performance, low self-discharge rate, and no memory effect (Ramli et al., 2018). The battery capacity is calculated based on the load electricity demand and the number of autonomous days, as follows:
Where nd represents the autonomous days. EL: Daily load electricity demand. Vb: Battery efficiency (90%). Vinv: Inverter efficiency (95%). Dd: Battery discharge depth (80%).
Diesel Generator: In this study, a diesel generator is utilized as a backup for renewable electricity output to meet power demands, with a minimum capacity of 25%. The fuel cost is set at 0.88$/L, and the estimated diesel maintenance oil cost is 1.34$/L/HG. The fuel consumption rate F (t) is defined by the formula (Askari and Ameri, 2012):
In the equation: F(t): Fuel consumption rate of the diesel generator. Fo: Intercept coefficient. F1:Slope of the fuel curve. Prate gen: Rated output power of the diesel generator. Pgen(t): Active output power of the diesel generator.
Converters: Converters are integrated into the microgrid system, where the DC component is used for AC loads and vice versa. Converters can be inverters (converting DC to AC), rectifiers (converting AC to DC), or a combination of both. In this study, the SG100k3 inverter is employed. Both the inverter and rectifier have an efficiency of 95%. It is assumed that the inverter can operate simultaneously with the AC generator, and the rated capacity of the rectifier relative to the inverter is 100% (He et al., 2018).
The primary input parameters for the components of the hybrid energy system are detailed in Table 3.
This study analyzes the electricity generation potential of independent hybrid energy systems at three different locations—Huanghuagou, Kelameili, and Wujiaqu—along the desert highway based on cost-benefit and environmental indicators. Evaluation is conducted based on parameters such as COE, NPC, photovoltaic capacity, wind turbine electricity generation, diesel generator electricity generation, battery capacity, bidirectional converter capacity, excess energy production, and renewable energy penetration rate. The findings for the three distinct locations along the desert highway are summarized as follows.
(1) Huanghuagou
Optimization results for various system configurations at Huanghuagou service area are presented in Table 4. According to the optimization outcomes, the hybrid energy system composed of PV/Wind/Diesel/Battery has the lowest COE and NPC, amounting to 0.32$/kWh and 4430135$, respectively. This system requires 331 kW of photovoltaic modules, 30 wind turbines, 440 kW diesel generators, 607 kWh of battery storage, and a 161 kW bidirectional converter. The cost summary of different components in the PV/Wind/Diesel/Battery hybrid energy system at Huanghuagou service area is depicted in Figure 4A. The wind turbine component incurs the highest investment cost, followed by the battery and photovoltaic components. Additionally, fuel costs contribute significantly to the overall expenses.
The monthly average electricity generation profile for the PV/Wind/Diesel/Battery hybrid energy system at Huanghuagou service area is illustrated in Figure 4B. During the summer, photovoltaic generation dominates the energy supply, while in winter, due to reduced sunlight hours, diesel generators become the primary energy source.
(2) Kelameili
Optimization results for various system configurations at Kelameili service area are presented in Table 5. According to the optimization outcomes, the hybrid energy system composed of PV/Wind/Diesel/Battery has the lowest COE and NPC, amounting to 0.34$/kWh and 3738790$, respectively. This system requires 427 kW of photovoltaic modules, 9 wind turbines, 440 kW diesel generators, 1,069 kWh of battery storage, and a 153 kW bidirectional converter. The cost summary of different components in the PV/Wind/Diesel/Battery hybrid energy system at Kelameili service area is depicted in Figure 5A. The battery component incurs the highest investment cost, followed by photovoltaic modules and wind turbine components. Additionally, fuel costs contribute significantly to the overall expenses.
The monthly average electricity generation profile for the PV/Wind/Diesel/Battery hybrid energy system at Kelameili service area is illustrated in Figure 5B. As depicted, photovoltaic generation is the main energy source during the summer, while in winter, due to reduced sunlight hours, diesel generators contribute the primary energy supply.
(3) Wujiaqu
Optimization results for various system configurations at Wujiaqu service area are presented in Table 6. According to the optimization outcomes, the hybrid energy system composed of PV/Wind/Diesel/Battery has the lowest COE and NPC, amounting to 0.34$/kWh and 3141641$, respectively. This system requires 422 kW of photovoltaic modules, 14 wind turbines, 440 kW diesel generators, 1,090 kWh of battery storage, and a 139 kW bidirectional converter. The cost summary of different components in the PV/Wind/Diesel/Battery hybrid energy system at Wujiaqu service area is depicted in Figure 6A. The battery component incurs the highest investment cost, followed by photovoltaic modules and wind turbine components. Additionally, fuel costs contribute significantly to the overall expenses.
The monthly average electricity generation profile for the PV/Wind/Diesel/Battery hybrid energy system at Wujiaqu service area is shown in Figure 6B. As depicted, photovoltaic generation is the main energy source during the summer, while in winter, due to reduced sunlight hours, diesel generators contribute the primary energy supply.
Through the above analysis, it can be found that the three case areas have different COE and NPC after optimized configuration simulation. The main reason is that different areas have different solar and wind energy resources, and there are differences in energy demand among different areas.
The emissions of pollutants in hybrid energy systems primarily depend on the fuel consumption of diesel generators. Table 7 presents a comparison of different types of pollutants emitted annually at Huanghuagou, Kelameili, and Wujiaqu highway service areas.
The comparison of CO2 emissions for optimal hybrid energy systems and diesel-only systems at Huanghuagou, Kelameili, and Wujiaqu locations is illustrated in Figure 7A. In comparison to other regions, Huanghuagou exhibits higher CO2 emissions, generating approximately 896,305 kg of carbon dioxide annually with the diesel-only power system, whereas the optimal hybrid energy system produces 425,862 kg of carbon dioxide. Conversely, Wujiaqu demonstrates lower CO2 emissions, with the diesel-only system producing around 792,513 kg of carbon dioxide annually, and the optimal hybrid energy system generating 198,387 kg of carbon dioxide. The renewable energy penetration rates and electricity generation from different hybrid energy systems in various regions are depicted in Figure 7B. Wujiaqu service area has the highest renewable energy penetration rate at 65%, while Huanghuagou service area has a lower rate at 47%. Examining the electricity generation, Huanghuagou has a higher annual output, while Wujiaqu has a lower annual output. Permeability can reflect the proportion of green energy in hybrid energy. The higher the penetration rate, the more environmentally friendly the system is and the less impact it has on the environment. Therefore, in the process of hybrid energy configuration, it is necessary to pursue a higher penetration rate.
The sensitivity analysis reveals the impact of specific variables on the optimal system type, NPC, COE, and emissions. This study investigates the influence of load growth and solar radiation intensity as variables. Considering a 10% growth in load demand, the load variables for Huanghuagou, Kelameili, and Wujiaqu are presented in Table 8. Based on the 2.2 load data and considering a 10% increase rate, calculate the data for four load increases.
Taking into account a 10% increase in solar radiation variables, the radiation variables for Huanghuagou, Kelameili, and Wujiaqu are outlined in Table 9. Based on the 2.2 radiation data and considering a 10% increase rate, calculate the data for four radiation increases.
Figure 8 illustrates the sensitivity analysis results of the optimal hybrid energy systems to changes in load demand. The NPC values for all locations increase with the growth in load demand, while the COE values decrease with the increasing load demand.
Figure 9 depicts the sensitivity analysis results of the increase in solar radiation on NPC and COE. The NPC and COE values for all locations decrease with the rise in radiation intensity.
This In this paper, a technical, economic, and environmental analysis of the hybrid energy generation systems in three service areas of a desert highway was conducted. The main conclusions are as follows:
i. Proposed the process and method of constructing a hybrid energy system for the Desert Expressway service area using HOMER Pro software. Using NPC and COE as control indicators, combined with environmental impact assessment, configure a hybrid energy scheme.
ii. The technical and economic analysis of the Huanghuagou, Kelameili, and Wujiaqu service areas of the Desert Expressway indicates that the PV/Wind/Diesel/Battery combination is the lowest-cost configuration for the hybrid energy system, and this combination is feasible in all three regions.
iii. Looking at the annual average electricity generation of the hybrid energy systems, the Huanghuagou system has a higher annual average electricity generation, producing 1,323,860 kWh. In contrast, the Wujiaqu system has a lower annual average electricity generation, producing 959,139 kWh. According to the analysis results, it can be seen that among the three service areas, the average annual CO2 emissions in Huanghuagou are relatively high at 434502 kg/yr, SO2 emissions are 1,080 kg/yr, and NOx emissions are 3,748 kg/yr. The average annual CO2 emissions in Wujiaqu are 198387 kg/yr, SO2 emissions are 493 kg/yr, and NOx emissions are 1711 kg/yr.
iv. Examining environmental pollutant emissions, the primary pollutant in the hybrid energy systems is carbon dioxide (CO2). Therefore, reducing CO2 emissions is a key focus of current air pollution control efforts. The analysis results indicate that among the three service areas, Huanghuagou has the highest annual average CO2 emissions at 434,502 kg/yr, while Wujiaqu has the lowest at 198,387 kg/yr.
v. Sensitivity analysis results indicate that the Net Present Cost (NPC) of all three service areas’ hybrid energy systems increases with an increase in load demand, while the Cost of Energy (COE) decreases with an increase in load demand. Both NPC and COE values for the hybrid energy systems in all three service areas decrease with an increase in radiation values
This study, through the analysis of the technical, economic, and environmental impacts of the hybrid energy systems in three service areas of the Desert Expressway, demonstrates the power generation potential of independent hybrid energy systems for service areas along the desert highway. The optimal hybrid energy configurations for each service area are established, providing an economically and operationally efficient solution for the hybrid energy systems. This enhances the energy independence of highways in desert regions and promotes the sustainable development of highway energy. The feasibility analysis presented in this study can serve as an application guide for the design and implementation of hybrid energy generation at critical energy nodes such as service areas along desert highways.
The original contributions presented in the study are included in the article/Supplementary material, further inquiries can be directed to the corresponding author.
GW: Formal Analysis, Funding acquisition, Resources, Writing–review and editing. YZ: Methodology, Project administration, Writing–original draft, Writing–review and editing. WT: Writing–review and editing. ZL: Writing–review and editing. TW: Investigation, Writing–original draft. SZ: Data curation, Writing–review and editing. XZ: Investigation, Supervision, Writing–original draft.
The author(s) declare financial support was received for the research, authorship, and/or publication of this article. This study was sponsored by the National Key Research and Development Program of China (Grant No. 2021YFB1600200)、Guangxi Transportation Science and Technology Demonstration Project “Guilin-Zhongshan Highway Green Energy Self-consistent Supply and Efficient Utilization Key Technology Integration Application Research and Demonstration”: 2023-0002 and Scientific Research Projects (21–53K, 21-36X) of Shaanxi Provincial Department of Transportation. The authors are grateful for the financial supports.
Authors GW, WT, and ZL were employed by Guangxi Communications Investment Group Corporation Ltd. Author XZ was employed by Shaanxi Transportation Planning and Design Institute Co, Ltd.
The remaining authors declare that the research was conducted in the absence of any commercial or financial relationships that could be construed as a potential conflict of interest.
The handling editor DY declared a past collaboration with authors YZ, TW, SZ at the time of the review.
All claims expressed in this article are solely those of the authors and do not necessarily represent those of their affiliated organizations, or those of the publisher, the editors and the reviewers. Any product that may be evaluated in this article, or claim that may be made by its manufacturer, is not guaranteed or endorsed by the publisher.
Ahmad, A., Loya, A., Ali, M., Iqbal, A., Baig, F. M., and Afzal, A. M. (2020). Roadside vertical Axis wind turbine (VAWT): an effective evolutionary design for Australian highway commuters with minimum dynamic stall. Engineering 12 (09), 601–616. doi:10.4236/eng.2020.129042
Akhtari, M. R., and Baneshi, M. (2019). Techno-economic assessment and optimization of a hybrid renewable co-supply of electricity, heat and hydrogen system to enhance performance by recovering excess electricity for a large energy consumer. Energy Convers. Manag. 188, 131–141. doi:10.1016/j.enconman.2019.03.067
Al-Buraiki, A. S., and Al-Sharafi, A. (2022). Hydrogen production via using excess electric energy of an off-grid hybrid solar/wind system based on a novel performance indicator. Energy Convers. Manag. 254, 115270. doi:10.1016/j.enconman.2022.115270
Al-Najjar, H., El-Khozondar, H. J., Pfeifer, C., and Al Afif, R. (2022). Hybrid grid-tie electrification analysis of bio-shared renewable energy systems for domestic application. Sustain. Cities Soc. 77, 103538. doi:10.1016/j.scs.2021.103538
Ang, Y. Q., Polly, A., Kulkarni, A., Chambi, G. B., Hernandez, M., and Haji, M. N. (2022). Multi-objective optimization of hybrid renewable energy systems with urban building energy modeling for a prototypical coastal community. Renew. Energy 201, 72–84. doi:10.1016/j.renene.2022.09.126
Askari, I. B., and Ameri, M. (2012). Techno-economic feasibility analysis of stand-alone renewable energy systems (PV/bat, Wind/bat and Hybrid PV/wind/bat) in Kerman, Iran. Energy Sour. Part B: econ. Plan. Policy 7, 45–60. doi:10.1080/15567240903330384
Büchner, J., Wistuba, M. P., Remmler, T., and Wang, D. (2019). On low temperature binder testing using DSR 4 mm geometry. Mater. Struct. 52 (6), 113. doi:10.1617/s11527-019-1412-3
Coban, H. H., Rehman, A., and Mohamed, A. (2022). Technical and economical investigation of a centralized and decentralized hybrid renewable energy system in cadaado, Somalia. Processes 10 (4), 667. doi:10.3390/pr10040667
Das, B. K., Hasan, M., and Das, P. (2021). Impact of storage technologies, temporal resolution, and PV tracking on stand-alone hybrid renewable energy for an Australian remote area application. Renew. Energy 173, 362–380. doi:10.1016/j.renene.2021.03.131
Das, P., Das, B. K., Rahman, M., and Hassan, R. (2022). Evaluating the prospect of utilizing excess energy and creating employments from a hybrid energy system meeting electricity and freshwater demands using multi-objective evolutionary algorithms. Energy 238, 121860. doi:10.1016/j.energy.2021.121860
Demirci, A., Akar, O., and Ozturk, Z. (2022). Technical-environmental-economic evaluation of biomass-based hybrid power system with energy storage for rural electrification. Renew. Energy 195, 1202–1217. doi:10.1016/j.renene.2022.06.097
El-houari, H., Allouhi, A., Rehman, S., Buker, M. S., Kousksou, T., Jamil, A., et al. (2020). Feasibility evaluation of a hybrid renewable power generation system for sustainable electricity supply in a Moroccan remote site. J. Clean. Prod. 277, 123534. doi:10.1016/j.jclepro.2020.123534
El-houari, H., Allouhi, A., Salameh, T., Kousksou, T., Jamil, A., and El Amrani, B. (2021). Energy, Economic, Environment (3E) analysis of WT-PV-Battery autonomous hybrid power plants in climatically varying regions. Sustain. Energy Technol. Assessments 43, 100961. doi:10.1016/j.seta.2020.100961
Haghighat Mamaghani, A., Avella Escandon, S. A., Najafi, B., Shirazi, A., and Rinaldi, F. (2016). Techno-economic feasibility of photovoltaic, wind, diesel and hybrid electrification systems for off-grid rural electrification in Colombia. Renew. Energy 97, 293–305. doi:10.1016/j.renene.2016.05.086
He, L., Zhang, S., Chen, Y., Ren, L., and Li, J. (2018). Techno-economic potential of a renewable energy-based microgrid system for a sustainable large-scale residential community in Beijing, China. Renew. Sustain. Energy Rev. 93, 631–641. doi:10.1016/j.rser.2018.05.053
Jahangir, M. H., Shahsavari, A., and Vaziri Rad, M. A. (2020). Feasibility study of a zero emission PV/Wind turbine/Wave energy converter hybrid system for stand-alone power supply: a case study. J. Clean. Prod. 262, 121250. doi:10.1016/j.jclepro.2020.121250
Jiang, W., Yuan, D. D., Shan, J. H., Ye, W. L., Lu, H. H., and Sha, A. M. (2022). Experimental study of the performance of porous ultra-thin asphalt overlay. Int. J. PAVEMENT Eng. 23 (6), 2049–2061. doi:10.1080/10298436.2020.1837826
Jiang, W., Zhang, S., Wang, T., Zhang, Y., Sha, A., Xiao, J., et al. (2024). Evaluation method for the availability of solar energy resources in road areas before route corridor planning. Appl. Energy 356, 122260. doi:10.1016/j.apenergy.2023.122260
Khan, F. A., Pal, N., Saeed, S. H., and Yadav, A. (2022). Techno-economic and feasibility assessment of standalone solar Photovoltaic/Wind hybrid energy system for various storage techniques and different rural locations in India. Energy Convers. Manag. 270, 116217. doi:10.1016/j.enconman.2022.116217
Kim, S., Lee, Y., and Moon, H.-R. (2018). Siting criteria and feasibility analysis for PV power generation projects using road facilities. Renew. Sustain. Energy Rev. 81, 3061–3069. doi:10.1016/j.rser.2017.08.067
Li, J., Liu, P., and Li, Z. (2020). Optimal design and techno-economic analysis of a solar-wind-biomass off-grid hybrid power system for remote rural electrification: a case study of west China. Energy 208, 118387. doi:10.1016/j.energy.2020.118387
Li, J., Liu, P., and Li, Z. (2022). Optimal design of a hybrid renewable energy system with grid connection and comparison of techno-economic performances with an off-grid system: a case study of West China. Comput. Chem. Eng. 159, 107657. doi:10.1016/j.compchemeng.2022.107657
Ma, Q., Huang, X., Wang, F., Xu, C., Babaei, R., and Ahmadian, H. (2022a). Optimal sizing and feasibility analysis of grid-isolated renewable hybrid microgrids: effects of energy management controllers. Energy 240, 122503. doi:10.1016/j.energy.2021.122503
Ma, X., Zhang, T., Xie, Y., and Tan, Q. (2022b). A simulation-assessment-optimization approach towards energy self-sufficiency and carbon reduction in regional-scale sewage systems. Resour. Conservation Recycl. 187, 106595. doi:10.1016/j.resconrec.2022.106595
Oko, C., Eo, D., Omunakwe, N.F., and Anthony Nnamdi, Ezemerihe (2012). Design and economic analysis of a photovoltaic system-a case study. Int. J. Renew. Energy Dev. 1 (3), 65–73. doi:10.14710/ijred.1.3.65-73
Ramesh, M., and Saini, R. P. (2020). Dispatch strategies based performance analysis of a hybrid renewable energy system for a remote rural area in India. J. Clean. Prod. 259, 120697. doi:10.1016/j.jclepro.2020.120697
Ramli, M. A. M., Bouchekara, H. R. E. H., and Alghamdi, A. S. (2018). Optimal sizing of PV/wind/diesel hybrid microgrid system using multi-objective self-adaptive differential evolution algorithm. Renew. Energy 121, 400–411. doi:10.1016/j.renene.2018.01.058
Razmjoo, A., Gakenia Kaigutha, L., Vaziri Rad, M. A., Marzband, M., Davarpanah, A., and Denai, M. (2021). A Technical analysis investigating energy sustainability utilizing reliable renewable energy sources to reduce CO2 emissions in a high potential area. Renew. Energy 164, 46–57. doi:10.1016/j.renene.2020.09.042
Renken, P., Büchler, S., Falchetto, A. C., Wang, D., and Wistuba, M. P. (2018). Warm mix asphalt-a German case study. Asph. Paving Technol. 87, 685–716. doi:10.12783/aapt2018/33821
Rezaei, M., Dampage, U., Das, B. K., Nasif, O., Borowski, P. F., and Mohamed, M. A. (2021). Investigating the impact of economic uncertainty on optimal sizing of grid-independent hybrid renewable energy systems. Processes 9 (8), 1468. doi:10.3390/pr9081468
Ribó-Pérez, D., Herraiz-Cañete, Á., Alfonso-Solar, D., Vargas-Salgado, C., and Gómez-Navarro, T. (2021). Modelling biomass gasifiers in hybrid renewable energy microgrids; a complete procedure for enabling gasifiers simulation in HOMER. Renew. Energy 174, 501–512. doi:10.1016/j.renene.2021.04.083
Sharma, S., Sood, Y. R., Sharma, N. K., Bajaj, M., Zawbaa, H. M., Turky, R. A., et al. (2022). Modeling and sensitivity analysis of grid-connected hybrid green microgrid system. Ain Shams Eng. J. 13 (4), 101679. doi:10.1016/j.asej.2021.101679
Shi, K., Ma, F., Fu, Z., Song, R., Yuan, D., and Ogbon, A. W. (2024). Enhancing aged SBS-modified bitumen performance with unaged bitumen additives. Constr. Build. Mater. 412, 134768. doi:10.1016/j.conbuildmat.2023.134768
Sifakis, N., Konidakis, S., and Tsoutsos, T. (2021). Hybrid renewable energy system optimum design and smart dispatch for nearly Zero Energy Ports. J. Clean. Prod. 310, 127397. doi:10.1016/j.jclepro.2021.127397
Sun, J., Huang, W., Lu, G. Y., Luo, S., and Li, Y. H. (2023a). Investigation of the performance and micro-evolution mechanism of low-content thermosetting epoxy asphalt binder towards sustainable highway and bridge decks paving. J. Clean. Prod. 384, 135588. doi:10.1016/j.jclepro.2022.135588
Sun, J., Luo, S., Wang, Y. Z., Dong, Q. F., and Zhang, Z. H. (2023b). Pre-treatment of steel slag and its applicability in asphalt mixtures for sustainable pavements. Chem. Eng. J. 476, 146802. doi:10.1016/j.cej.2023.146802
Toopshekan, A., Yousefi, H., and Astaraei, F. R. (2020). Technical, economic, and performance analysis of a hybrid energy system using a novel dispatch strategy. Energy 213, 118850. doi:10.1016/j.energy.2020.118850
Uwineza, L., Kim, H.-G., and Kim, C. K. (2021). Feasibility study of integrating the renewable energy system in Popova Island using the Monte Carlo model and HOMER. Energy Strategy Rev. 33, 100607. doi:10.1016/j.esr.2020.100607
Vendoti, S., Muralidhar, M., and Kiranmayi, R. (2020). Techno-economic analysis of off-grid solar/wind/biogas/biomass/fuel cell/battery system for electrification in a cluster of villages by HOMER software. Environ. Dev. Sustain. 23 (1), 351–372. doi:10.1007/s10668-019-00583-2
Vergara-Zambrano, J., Kracht, W., and Díaz-Alvarado, F. A. (2022). Integration of renewable energy into the copper mining industry: a multi-objective approach. J. Clean. Prod. 372, 133419. doi:10.1016/j.jclepro.2022.133419
Walther, A., Bchler, S., Falchetto, A. C., Wang, D., Riccardi, C., and Wistuba, M. P. (2019). Experimental investigation on asphalt mixtures prepared with reclaimed asphalt pavement and rejuvenators based on the BTSV method. ROAD Mater. PAVEMENT Des. 20 (7), 1695–1708. doi:10.1080/14680629.2019.1594053
Wang, H. P., Airey, G. D., Leng, Z., and Lu, G. Y. (2023). Optimisation of the preparation procedure of crumb rubber modified bitumen with wax-based additives. ROAD Mater. PAVEMENT Des., 1–12. doi:10.1080/14680629.2023.2191724
Wu, W., Cavalli, M. C., Jiang, W., and Kringos, N. (2024). Differing perspectives on the use of high-content SBS polymer-modified bitumen. Constr. Build. Mater. 411, 134433. doi:10.1016/j.conbuildmat.2023.134433
Yuan, D. D., Jiang, W., Sha, A. M., Xiao, J. J., Wu, W. J., and Wang, T. (2023). Technology method and functional characteristics of road thermoelectric generator system based on Seebeck effect. Appl. ENERGY 331, 120459. doi:10.1016/j.apenergy.2022.120459
Keywords: road engineering, service area, hybrid energy, feasibility, economic, technical, environmental
Citation: Wang G, Zhang Y, Tang W, Liao Z, Wang T, Zhang S and Zhao X (2024) Feasibility analysis of hybrid energy generation systems for desert highway service areas: a case study in northern Xinjiang, China. Front. Energy Res. 12:1362957. doi: 10.3389/fenrg.2024.1362957
Received: 29 December 2023; Accepted: 19 January 2024;
Published: 02 February 2024.
Edited by:
Dongdong Yuan, Chang’an University, ChinaReviewed by:
Jinhuan Shan, University of Leeds, United KingdomCopyright © 2024 Wang, Zhang, Tang, Liao, Wang, Zhang and Zhao. This is an open-access article distributed under the terms of the Creative Commons Attribution License (CC BY). The use, distribution or reproduction in other forums is permitted, provided the original author(s) and the copyright owner(s) are credited and that the original publication in this journal is cited, in accordance with accepted academic practice. No use, distribution or reproduction is permitted which does not comply with these terms.
*Correspondence: Yufei Zhang, enl1ZmVpQGNoZC5lZHUuY24=
Disclaimer: All claims expressed in this article are solely those of the authors and do not necessarily represent those of their affiliated organizations, or those of the publisher, the editors and the reviewers. Any product that may be evaluated in this article or claim that may be made by its manufacturer is not guaranteed or endorsed by the publisher.
Research integrity at Frontiers
Learn more about the work of our research integrity team to safeguard the quality of each article we publish.