- 1State Key Laboratory of Nuclear Power Safety Technology and Equipment, China Nuclear Power Engineering Co., Ltd., Shenzhen, Guangdong, China
- 2China Nuclear Power Engineering Co., Ltd., Shenzhen, Guangdong, China
- 3Xi’an Jiaotong University, Xi’an, Shaanxi, China
Radionuclides emitted by nuclear power plants may have effects on the environment and public health. At present, research on radioactive material effluent in the industry mainly focuses on the treatment of radioactive effluent and the particle size distribution of the primary circuit. There is little research on the particle size of radioactive material during the migration process outside the primary circuit system, as well as the flocculation precipitation and other enrichment phenomena during the collection process of effluent. Therefore, this study relies on the sampling of effluent from an in-service nuclear power plant to measure its radioactivity level by particle size range. At the same time, the mixing process of effluent is simulated in the laboratory to simulate the adsorption behavior of effluent during the migration process. It was found that in the activity concentration of detectable radioactive nuclides in the effluent samples, more than 95% of radioactive nuclides exist in the liquid with particle sizes less than 0.1μm, while particle sizes greater than 0.45 μm account for less than 5%. After the sample was filtered by the demineralizer, the radioactive activity decreased. The flocculation precipitation in the waste liquid of the waste water recovery system has a certain contribution to the enrichment of nuclides. With the extension of time, the enrichment of transition elements such as cobalt and manganese is particularly obvious, so that it is distributed in the liquid again with a large particle size. In addition, large particle size substances such as colloids in seawater have a certain adsorption effect on radionuclides, which will lead to its aggregation effect again.
1 Introduction
The study of the safety of nuclear reactor facilities is of great significance (Qi et al., 2023; Cao et al., 2024). The radioactive nuclides produced by nuclear power plants mainly come from the fission of nuclear fuel and the activation corrosion of Primary circuit structural materials. In terms of effluent, the liquid radioactive nuclides emitted during conventional operation of nuclear power plants include 137Cs, 51Cr, 54Mn, 110mAg, 124Sb, 134Cs, 131I, 90Sr, 103mRh, 58Co, 58Co (Kong et al., 2017). The discharge of liquid effluents from nuclear power plants into the environment under national regulations and standards means that the effluents are safe from a legal perspective (National Nuclear Energy Agency, 2011; Ministry of Environmental and Forestry, 2016; Yuniarto et al., 2016).
However, companies around nuclear power plants hold a questioning attitude towards the safety of effluent, especially those involved in the large-scale use of seawater technology. During the process of seawater reuse in the ocean, radioactivity is trapped and enriched again on the filtering equipment, causing radiation effects on workers. According to theoretical estimation, if the source term of the effluent is measured at a radioactive concentration of 1,000 Bq/L, under extremely conservative assumptions (radioactive nuclides in the effluent are all trapped by the filters of the enterprise’s seawater extraction facilities), the contact dose rate of the filter can reach approximately the level of 10 μSv/h, which corresponds to the dose rate level in the control area (green area) of the nuclear power plant, and is indeed unacceptable for civil enterprises.
It should be pointed out that due to overly conservative assumptions, the possibility of the aforementioned conclusion occurring is unlikely. However, the crux still lies in the lack of understanding of the actual particle size distribution of radioactive substances in the effluent, and the assessment can only conservatively assume that all radioactive substances have been trapped on the filter core. On the other hand, according to feedback from the operation of in-service nuclear power plants, sampling and testing before effluent discharge can indeed detect radioactive hot particles. It should be pointed out that the liquid effluent of nuclear power plants is treated by multiple purification methods such as filtration and desalination of radioactive waste liquid from nuclear power plants. Therefore, it is believed that the effluent is basically non-radioactive. The actual phenomenon of measuring radioactive hot particles indicates that radioactive enrichment may have occurred during the collection process of the effluent, which may have resulted in the regeneration of particles.
Therefore, combining the monitoring methods of effluent (Kang and Cheong, 2022; Wang et al., 2023) and the treatment methods of radioactive nuclides in effluent (Attallah et al., 2019; Bashir et al., 2019; Ahmed et al., 2020; Ma et al., 2020; Tofighy et al., 2020; Gul et al., 2021; Thakur et al., 2022; Nivetha et al., 2023; Oh et al., 2023), mastering the particle size of radioactive substances in effluent, and studying whether the collection process of effluent will produce particulate thermal particles, is the key to more accurate evaluation and reducing the impact of effluent on environmental radiation. The relationship route is shown in Figure 1.
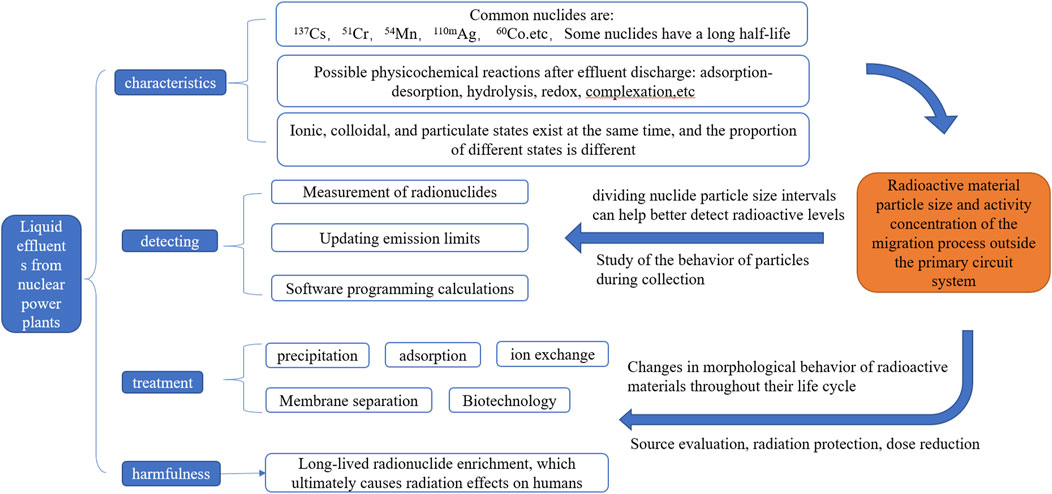
FIGURE 1. Characteristics, hazards, monitoring and treatment of liquid effluents from nuclear power plants, and study on particle size behavior.
This study relied on the sampling of effluent from an in-service nuclear power plant to measure its radioactive levels in particle size intervals. However, it is also necessary to consider the migration process of effluent into the ocean. At present, research on the particle size distribution and adsorption behavior of radioactive nuclides in seawater has focused on seawater measurement. For example, Yuki Kamidaira et al. established an ocean diffusion model for radioactive nuclides, considering the interaction between dissolved radioactive nuclides and suspended particles and multi-scale seabed sediments (Kamidaira et al., 2021). P. G. Appleby et al. believed that radioactive nuclides are soluble in water and adhere to colloidal sized particles (<0.4 μm) The upper part is basically transported with the water phase. The deposition or outflow of particulate matter largely depends on factors that control the transportation of suspended sediment through water bodies, including particle size distribution, residence time, etc. (Appleby et al., 2019). Therefore, this study also utilized laboratory simulations of the mixing process of effluent to simulate the adsorption behavior of effluent during migration.
It should be pointed out that the research on the particle size of radioactive substances in the industry mainly focuses on the primary circuit (Geraldo et al., 2019; Li et al., 2019; Tessaro et al., 2020; Guo et al., 2022), while there is very little research on the particle size of radioactive substances during the migration process outside the primary circuit system. Studying and analyzing the changes in particle size morphology of radioactive materials throughout the entire life cycle of nuclear power plants is a prerequisite for constructing a full life cycle source term evaluation and radiation protection. It helps to further improve the level of source term management in nuclear power plants, reduce collective doses, and select more favorable and suitable environmental conditions to cope with radiation effects. Therefore, research has long-term significance.
2 Material design and manufacture
2.1 The object of study
Effluent from the nuclear Island building of a pressurized water reactor nuclear power plant is collected in the liquid waste storage tank of the Nuclear Island Liquid Waste Discharge System (TER) before discharge. The effluent to be discharged is received in the TER system effluent temporary tank from the Radioactive Effluent Treatment System (TEU), the Boron Recovery System (TEP), and the radioactive effluent Recovery System (SRE). In this study, the effluent from a nuclear power unit during the shutdown overhaul in March 2023 was selected for sampling and measurement. Before sampling, the TER waste liquid temporary storage tank was decontaminated to avoid radioactive mixing. Then each strand of waste liquid discharged from TEU, TEP and SRE systems into TER waste liquid temporary storage tank was sampled, and a total of two groups of waste liquid samples were collected. The sampling information is in Table 1.
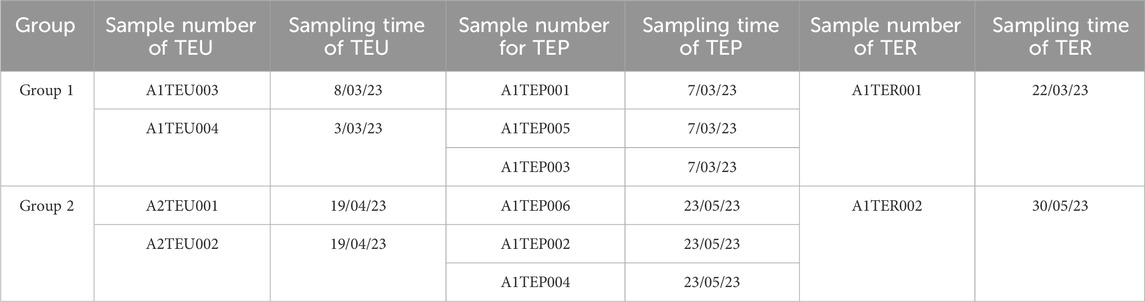
TABLE 1. Sampling information of waste liquid discharged into the TER waste liquid temporary storage tank by TEU, TEP, and SRE systems.
The sample size of each sample is about 5L, and the sample container is taken from a 1L glass bottle. The inner wall of the bottle is soaked with dilute hydrochloric acid with pH = 1 in advance to reduce the adsorption loss of radionuclides. Seven kinds of radionuclides were measured, including 58Co、60Co、134Cs、137Cs、110mAg、54Mn、124Sb, etc. For particle size, five intervals were separated, respectively: d < 0.1 μm, 0.1 μm ≤ d < 0.45 μm, 0.45 μm ≤ d < 1 μm, 1 μm ≤ d < 5 μm and d ≥ 5 μm.
2.2 Equipment material
The nuclide in the sample was analyzed by using the high-purity germanium gamma spectrometer system produced by ORTEC and CANBERRA. There are 3 sets of measuring instruments (the equipment models are GEM40P4-76, GC4019 and BE3830 respectively), and the drying oven produced by Nabertherm (the model is TR240) was used for sample pretreatment. The sample particle size was separated by microporous filter membrane produced by Hangzhou Anuo Filtration Equipment Co., LTD. (Material was polypropylene, diameter was 75 mm, pore size was 0.1, 0.45, 1.0, and 5.0 μm, respectively). All the devices are used within the verification validity period and can be traced to the national standard.
2.3 Sample particle size separation and measurement methods
The colloids or clusters that may exist in different particle size ranges in the sample are separated by microporous filtration membrane combined with the extraction filtration device, as shown in Figure 2. The process is as follows:
(1) The filter membrane is dried in a drying oven at 110°C to constant weight, and the quality of the filter membrane is numbered and recorded;
(2) According to the filter membrane aperture from large to small in the order of separation, the filter membrane with the corresponding aperture is fixed on the G1 sand core funnel with a clamp, the sample is pumped with a constant pressure filtration device, the pressure is set at 0.7 MPa, and the filter membrane is replaced once every 500 mL sample pumped;
(3) The beaker containing the sample is cleaned more than 3 times with distilled water, and the cleaning liquid is also pumped and filtered, and the volume of distilled water used is recorded;
(4) After the filtration is completed, the same group of filter membranes with the same particle size are placed on the surface dish and numbered, dried at 110°C in the oven to constant weight, and four groups of filter membranes with the particle size of 0.1–0.45 μm, 0.45∼1 μm, 1–5 μm and >5 μm are obtained. After weighing the filter membranes, they are pressed into the sample box of φ75 × 10 and sealed. Put into the specified high purity germanium gamma spectrometer for measurement;
(5) The filtrate after pumping and filtering is shaken and measured 200 mL, weighed and put into the sample bottle and put into the specified high purity germanium gamma spectrometer for measurement;
(6) After filtration, nuclide activity concentrations with particle size distribution less than 0.1 μm can be measured in the filtrate. On microporous filter membranes with pore sizes of 0.1, 0.45, 1.0, and 5.0 μm, four sets of nuclide activity concentrations with particle sizes of 0.1–0.45 μm, 0.45–1 μm, 1–5 μm, and >5 μm can be measured, respectively;
(7) After the separation of each sample was completed, the filtration device was cleaned with dilute hydrochloric acid solution with pH = 1, and washed with distilled water before it could be used for the separation of subsequent samples.
2.4 Measurement and treatment of radionuclides adsorbed on the inner wall of the sample container
Although the inner wall of the sample container was treated with anti-adsorption treatment, there were still a small number of nuclides adsorbed on the inner wall of the container. In order to determine the activity of the nuclides adsorbed on the inner wall of the container, the measurement efficiency of the sample container and the corresponding nuclides was established by using LABSOCS software in this experiment, and the results were verified by using Monte-Carlo simulation and different γ spectrometer. The sample container is fully rinsed with distilled water and drained after being placed in the instrument for measurement. The common desorption methods can be roughly divided into physical methods and chemical methods, among which the common physical method is ultrasonic vibration, but the effect is not very good. In chemical methods, acid washing can be used for desorption, and its desorption effect is better than ultrasonic vibration.
After the measurement, 1L of 2 mol/L dilute nitric acid solution was added to each sample container and soaked for about 150 h. The acid solution after soaking was taken for measurement to determine the nuclide activity of the desorbed container wall. After the pH of the acid solution was adjusted to 7∼8 with sodium hydroxide, the particle size was separated and measured. The average desorption efficiency of each nuclide ranged from 80.8% to 90.2%, as shown in Figure 3.
2.5 Experimental simulation
In order to observe the behavior of radioactive particles adsorbed by colloid in seawater during the migration and mixing process of liquid effluent after discharge, the laboratory used SRE waste liquid with low radioactivity to mix with TEP and TEU waste liquid respectively to simulate the mixing process of waste liquid of different systems in TER waste liquid temporary storage tank. Because the SRE waste liquid contains a certain number of surfactants, the colloidal composition inside the solution is more complex than the natural seawater, and this simulation process also simply represents the mixing process of the effluent and seawater.
A total of six groups of simulated samples were prepared in this experiment, including three groups of SRE and A1TEP001 simulated samples and three groups of SRE and A2TEU001 simulated samples, each group was composed of 500 mL SRE waste liquid and 500 mL TEP and TEU waste liquid. Each group of simulated samples was stirred for 1 min in the morning and evening every day. The simulation duration was 5, 10 and 20 days, respectively. After reaching the simulation time, use the above method to separate and measure the particle size of the sample.
3 Result and discussion
3.1 Measurement results of each nuclide in the effluent sample
The measurement results of each nuclide in the initial state of the sample are shown in Table 2. The extracted information is plotted as a column chart, as shown in Figure 4. The activity concentration of radioactive nuclides in TEU and TEP wastewater is reduced to varying degrees after being filtered by a desalination device. The measurement results of all nuclides in TER and SRE wastewater, except for 60Co, are below the detection limit.
You may insert up to five heading levels into your manuscript as can be seen in “Styles” tab of this template. These formatting styles are meant as a guide, as long as the heading levels are clear, Frontiers style will be applied during typesetting.
3.2 Measurement results of activity concentration distribution of various nuclides with different particle sizes in each sample
The activity concentration distribution results of various nuclides with different particle sizes in each sample are shown in Table 3, and plotted as a column chart as shown in Figures 5–8. Except for the SRE system, the measurement results of all other systems with d ≥ 0.45 μm are less than the detection limit. In order to better express the relationship between the main particle size and activity concentration, samples below the detection value in the sample results are omitted from the bar graph.
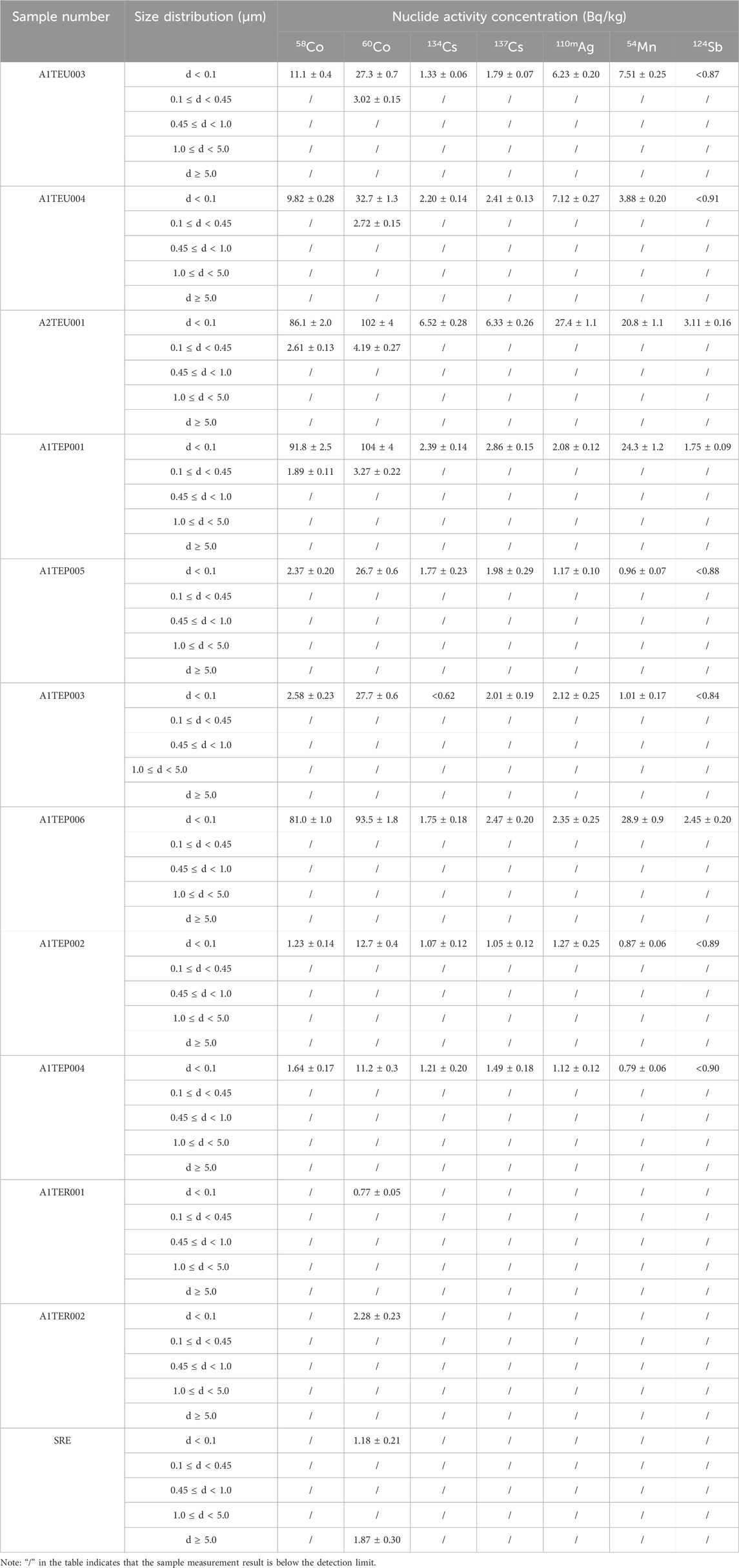
TABLE 3. Activity concentrations of different nuclides with different particle sizes in each system.
According to Table 3, The proportion of nuclide activity concentrations with particle size distribution less than 0.1 μm in sample A1TEU003 is:
The calculation process for other samples is the same as above, and the calculation results are as follows: pA1TEU004 = 0.956, pA2TEU001 = 0.971, pA1TEP001 = 0.978, pA1TEP005 = 1 pA1TEP003 = 1, pA1TEP006 = 1, pA1TEP002 = 1, pA1TEP004 = 1, pA1TER001 = 1, pA1TER002 = 1, pSRE = 0.387. Obviously, for most samples, the proportion of nuclide activity concentrations with particle size distribution less than 0.1 μm is over 95%.
The reason for the distribution of nuclides within the d ≥ 5.0 μm in the SRE system is that the surfactant in the liquid produces a small amount of flocculent precipitation, which adsorbs a small number of nuclides. The flocculent precipitation at the bottom of the SRE system sample is shown in Figure 9.
In addition, all nuclides in other systems are concentrated within the particle size range of d < 0.45 μm, mainly within the particle size range of d < 0.1 μm. It is worth noting that the sum of activity concentrations for each particle size is not the same as the activity concentration in the initial state of the sample, and each nuclide has a certain loss, possibly due to:
(1) There are a small amount of radioactive nuclides present in the interval d > 0.45 μm, but the activity concentration is lower than the judgment limit measured by the instrument;
(2) During the separation and measurement process, a small number of nuclides are adsorbed on the container wall, causing losses.
3.3 Simulate the measurement results of each nuclide in the sample
The activity concentration distribution of various nuclides with different particle sizes in the simulated sample is shown in Table 4, and the column plots are shown in Figure 10 and Figure 11. With the extension of mixing time, the simulated samples of TEU and TEP showed different degrees of radioactive enrichment in the range of d ≥ 5.0 μm, indicating that the flocculent precipitates and colloids in the SRE waste liquid have a certain degree of enrichment effect on Co and Mn. However, the overall activity of radioactive nuclides in the sample shows a decreasing trend, mainly due to a certain amount of wall adsorption during the mixed enrichment process, resulting in a small amount of nuclide loss.
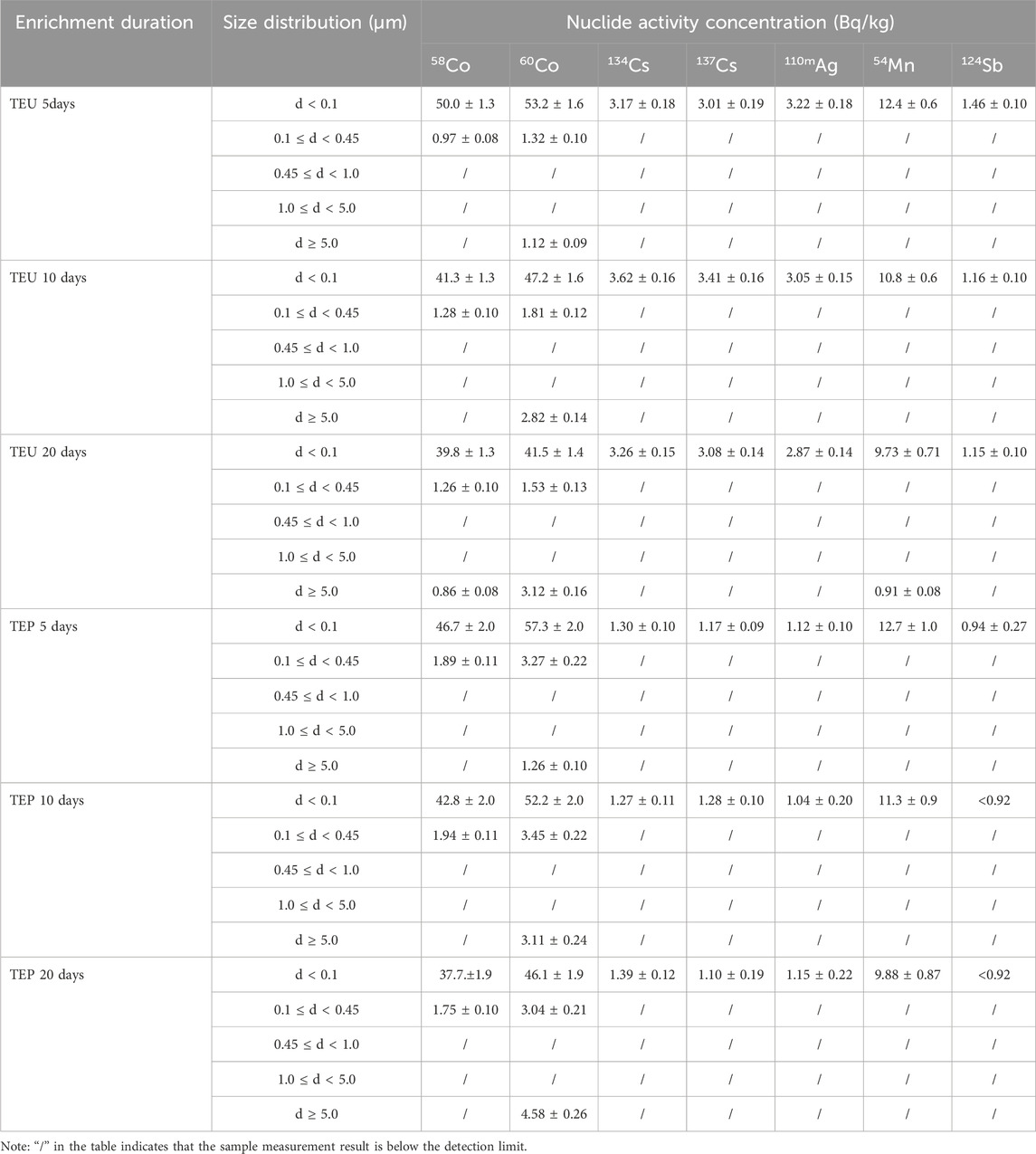
TABLE 4. Activity concentrations of different nuclides with different particle sizes in simulated samples.
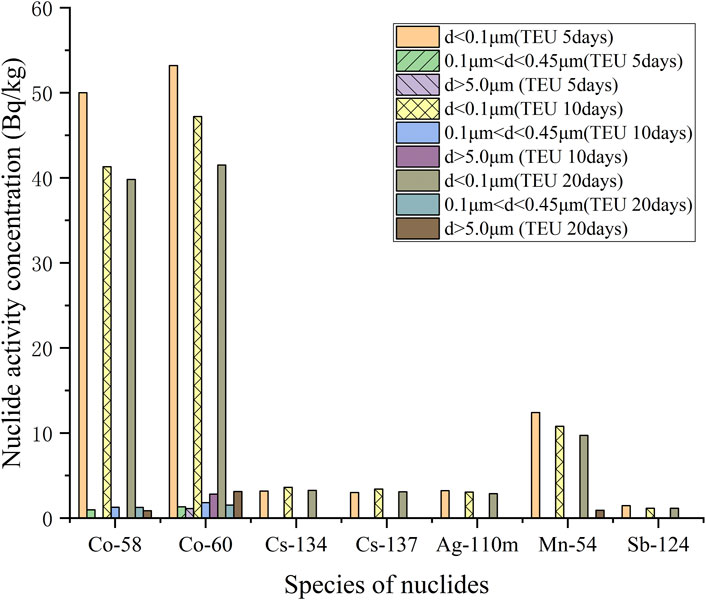
FIGURE 10. Histogram of activity concentration of TEU samples at different enrichment times with different particle sizes of nuclides.
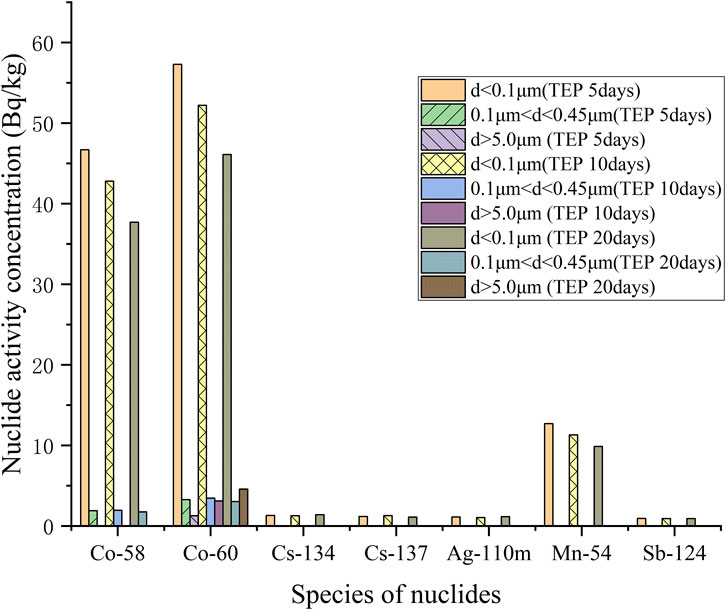
FIGURE 11. Histogram of activity concentration of each nuclide with different particle sizes at different enrichment times of TEP samples.
4 Conclusion
The radioactive nuclides in the effluent samples mostly exist in the liquid with a particle size of d < 0.1 μm, and are almost not distributed in the form of d > 0.45 μm particle size. After the sample is filtered by a desalination device, the radioactive activity is greatly reduced, and the filtering effect of the desalination device on radioactive nuclides cannot be ignored.
The flocculent precipitation in the waste liquid of the SRE system has a certain contribution to the enrichment of nuclides. With the extension of time, its enrichment of transition elements such as cobalt and manganese is particularly significant, causing nuclides to be distributed again in larger particle size forms in the liquid. It can be inferred that after the liquid effluent is discharged into the receiving water body, large particle sized substances such as colloids in the seawater have a certain adsorption effect on radioactive nuclides, which will lead to their aggregation effect again.
Although the liquid effluents from pressurized water reactor nuclear power plants are mainly dispersed in small particle sizes in the TEP and TEU systems, after they merge with SRE liquids containing complex colloids and particles in the TER tank and are stirred by external forces, transition metals such as cobalt and manganese will undergo a small amount of aggregation effect again within a certain period of time. With the extension of time and environmental conditions, after the effluent is discharged into the sea, A small amount of radioactive nuclides will inevitably accumulate in seawater, but the ocean is an extremely complex medium, and nuclides will undergo complex particle size changes as seawater migrates and exchanges with sediment and marine organisms.
Data availability statement
The original contributions presented in the study are included in the article/Supplementary Material, further inquiries can be directed to the corresponding authors.
Author contributions
ZJ: Conceptualization, Methodology, Writing–review and editing. JX: Formal Analysis, Software, Validation, Writing–original draft. YP: Supervision, Validation, Writing–original draft. JH: Supervision, Validation, Writing–review and editing. YC: Data curation, Formal Analysis, Writing–original draft. SY: Conceptualization, Methodology, Writing–original draft. YY: Conceptualization, Methodology, Writing–review and editing.
Funding
The author(s) declare financial support was received for the research, authorship, and/or publication of this article. This research is supported by the National Natural Science Foundation Projects under Grant (11975182).
Conflict of interest
Authors ZJ, JX, YP, YC, and SY were employed by China Nuclear Power Engineering Corporation.
The remaining authors declare that the research was conducted in the absence of any commercial or financial relationships that could be construed as a potential conflict of interest.
Publisher’s note
All claims expressed in this article are solely those of the authors and do not necessarily represent those of their affiliated organizations, or those of the publisher, the editors and the reviewers. Any product that may be evaluated in this article, or claim that may be made by its manufacturer, is not guaranteed or endorsed by the publisher.
References
Ahmed, D. S., Mohammed, M. R., and Mustafa, K. A. M. (2020). Synthesis of multi-walled carbon nanotubes decorated with ZnO/Ag nanoparticles by co-precipitation method. Nanosci. Nanotechnology-Asia 10 (2), 127–133. doi:10.2174/2210681208666181005145644
Appleby, P. G., Semertzidou, P., Piliposian, G. T., Chiverrell, R. C., Schillereff, D. N., and Warburton, J. (2019). The transport and mass balance of fallout radionuclides in Brotherswater, Cumbria (UK). J. Paleolimnol. 62 (4), 389–407. doi:10.1007/s10933-019-00095-z
Attallah, M. F., Hassan, H. S., and Youssef, M. A. (2019). Synthesis and sorption potential study of Al2O3ZrO2CeO2 composite material for removal of some radionuclides from radioactive waste effluent. Appl. Radiat. Isotopes 147, 40–47. doi:10.1016/j.apradiso.2019.01.015
Bashir, A., Malik, L. A., Ahad, S., Manzoor, T., Bhat, M. A., Dar, G. N., et al. (2019). Removal of heavy metal ions from aqueous system by ion-exchange and biosorption methods. Environ. Chem. Lett. 17, 729–754. doi:10.1007/s10311-018-00828-y
Cao, A., Hua, Y., Sun, Z., Meng, Z., Guo, Z., and Zhang, N. (2024). Study on reduction of pressure oscillation in low-velocity steam jet condensation. Ann. Nucl. Energy 197 (2024), 110271. doi:10.1016/j.anucene.2023.110271
Geraldo, B., de Araujo, L. G., Taddei, M. H. T., Ferreira, M. T., Máduar, M. F., Vicente, R., et al. (2019). Radiochemical characterization of spent filter cartridges from the primary circuit of a research reactor. J. Radioanalytical Nucl. Chem. 322, 1941–1951. doi:10.1007/s10967-019-06864-4
Gul, A., Hruza, J., and Yalcinkaya, F. (2021). Fouling and chemical cleaning of microfiltration membranes: a mini-review. Polymers 13 (6), 846. doi:10.3390/polym13060846
Guo, J., Wang, Y., Zhou, Z., Xie, F., Tong, J., Wang, K., et al. (2022). “Summary of methods for studying the chemical states of nuclides in nuclear Energy systems,” in International Conference on Nuclear Engineering, August 8–12, 2022. V015T16A032.
Kamidaira, Y., Uchiyama, Y., Kawamura, H., Kobayashi, T., and Otosaka, S. (2021). A modeling study on the oceanic dispersion and sedimentation of radionuclides off the coast of Fukushima. J. Environ. Radioact. 238, 106724. doi:10.1016/j.jenvrad.2021.106724
Kang, J. S., and Cheong, J. H. (2022). New methodologies to derive dischargelimits considering operational flexibility of radioactive effluents from Korean nuclear power plants based on historical discharge data. Nucl. Eng. Technol. 54 (3), 1003–1015. doi:10.1016/j.net.2021.09.007
Kong, T. Y., Kim, S., Lee, Y., Son, J. K., and Maeng, S. J. (2017). Radioactive effluents released from Korean nuclear power plants and the resulting radiation doses to members of the public. Nucl. Eng. Technol. 49 (8), 1772–1777. doi:10.1016/j.net.2017.07.021
Li, C., Li, W., Sun, L., Xing, H., and Fang, C. (2019). Chemical forms of important fission products in primary circuit of HTR-PM under conditions of normal operation and overpressure and water ingress accidents: a study with a chemical thermodynamics approach. Sci. Technol. Nucl. Installations 2019, 1–12. doi:10.1155/2019/4251280
Ma, F., Gui, Y., Liu, P., Xue, Y., and Song, W. (2020). Functional fibrous materials-based adsorbents for uranium adsorption and environmental remediation. Chem. Eng. J. 390, 124597. doi:10.1016/j.cej.2020.124597
Ministry of Environmental and Forestry (2016). Decree of changes in environmental permits for operational activities of the serpong nuclear area and irradiators and other facilities. Jakarta, Indonesia.
National Nuclear Energy Agency (2011). Emergency and preparedness plan. ‖ safety analysis report of G.A. Siwabessy multipurpose reactor, rev 10.1 p 20, tangerang selatan, Indonesia.
Nivetha, N., Srivarshine, B., Sowmya, B., Rajendiran, M., Saravanan, P., Rajeshkannan, R., et al. (2023). A comprehensive review on bio-stimulation and bio-enhancement towards remediation of heavy metals degeneration. Chemosphere 312 (2023), 137099. doi:10.1016/j.chemosphere.2022.137099
Oh, M., Lee, K., Jeon, M. K., Foster, R. I., and Lee, C. H. (2023). Chemical precipitation–based treatment of acidic wastewater generated by chemical decontamination of radioactive concrete. J. Environ. Chem. Eng. 11, 110306. doi:10.1016/j.jece.2023.110306
Qi, X., Yu, F., Meng, Z., Sun, Z., Zhang, N., and Guo, Z. (2023). Preliminary design of the suppressive containment system based on HPR1000. Nucl. Eng. Des. 415 (2023), 112743. doi:10.1016/j.nucengdes.2023.112743
Tessaro, A. P. G., de Araujo, L. G., and Vicente, R. (2020). Inventorying the radionuclides in spent cartridge filters from the primary circuit of a nuclear research reactor by the dose-to-activity method. Radiat. Phys. Chem. 171, 108710. doi:10.1016/j.radphyschem.2020.108710
Thakur, D. A., Sonar, N. L., Shukla, R., Valsala, T. P., Sathe, D. B., Bhatt, R. B., et al. (2022). Evaluation of cerium–zirconium mixed oxides for separation of 125Sb from radioactive liquid waste. J. Radioanalytical andNuclear Chem. 331 (7), 2903–2909. doi:10.1007/s10967-022-08369-z
Tofighy, M. A., and Mohammadi, T. (2020). “Carbon nanotubes-polymer nanocomposite membranes for pervaporation,” in Polymer nanocomposite membranes for pervaporation. Editor S. Thomas (Elsevier), 105–133.
Wang, X. W., Wang, R. D., Liang, M. C., He, S. J., Zhang, C., Li, K., et al. (2023). High-temperature catalytic oxidation preparation and liquid scintillation counting determination of the carbon-14 in liquid effluent samples from nuclear power plants. Chin. J. Anal. Chem. 51 (4), 100218. doi:10.1016/j.cjac.2022.100218
Keywords: pressurized water reactor, radioactive effluent, γ measurement, particle size, adsorption effect
Citation: Jiang Z, Xiong J, Pan Y, Hu J, Chen Y, Yin S and Yan Y (2024) Study on the actual particle size, activity concentration, and migration process adsorption behavior of radioactive substances in liquid effluents from nuclear power plants. Front. Energy Res. 12:1352706. doi: 10.3389/fenrg.2024.1352706
Received: 08 December 2023; Accepted: 02 January 2024;
Published: 19 January 2024.
Edited by:
Shichang Liu, North China Electric Power University, ChinaReviewed by:
Zhaoming Meng, Harbin Engineering University, ChinaYuanyuan Xue, Northwest Institute of Nuclear Technology, China
Copyright © 2024 Jiang, Xiong, Pan, Hu, Chen, Yin and Yan. This is an open-access article distributed under the terms of the Creative Commons Attribution License (CC BY). The use, distribution or reproduction in other forums is permitted, provided the original author(s) and the copyright owner(s) are credited and that the original publication in this journal is cited, in accordance with accepted academic practice. No use, distribution or reproduction is permitted which does not comply with these terms.
*Correspondence: Shuhua Yin, yinshuhua@cgnpc.com.cn; Yihong Yan, yihong@stu.xjtu.edu.cn