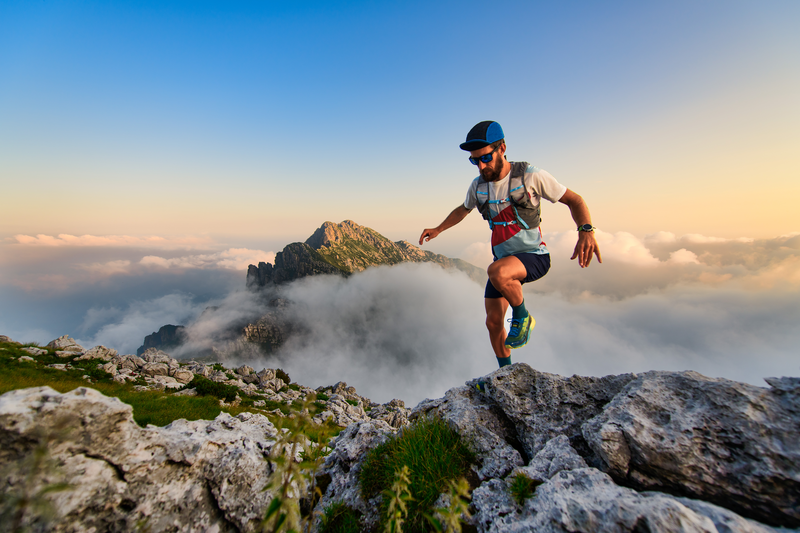
95% of researchers rate our articles as excellent or good
Learn more about the work of our research integrity team to safeguard the quality of each article we publish.
Find out more
ORIGINAL RESEARCH article
Front. Energy Res. , 19 March 2024
Sec. Sustainable Energy Systems
Volume 12 - 2024 | https://doi.org/10.3389/fenrg.2024.1338657
The linkage, coordination, and complementary cooperation of energy supply can improve the efficiency of transportation and utilization. At present, the level of new energy consumption needs to be improved, the coordination of the source network load storage link is insufficient, and the insufficient complementarity of various types of power sources in the power system. This article fully explores the differences and complementarities of various types of wind-solar-hydro-thermal-storage power sources, a hierarchical environmental and economic dispatch model for the power system has been established. Among them, the upper level model takes the flexible consumption of new energy as the optimization goal, the middle level model uses a combination of hydropower station and energy storage to minimizes the fluctuation variance and peak-to-valley difference of the net load curve, the lower level model aims to achieve optimal environmental and economic benefits of the power system, comprehensively considers the coal consumption cost, startup and shutdown cost, energy storage operation cost, and pollutant emissions of thermal power units, determines the startup and shutdown mode and output power of thermal power units. Finally, an improved IEEE 6-machine 30-node system is used as an example for simulation analysis, the results show that after applying the proposed hierarchical environmental and economic dispatch strategy of the power system, the fluctuation variance and the peak-to-valley difference of the net load curve have been reduced by 46.3% and 31.5%, respectively, and the environmental and economic benefits of the system is improved by 5.1% compared with the traditional economic dispatch strategy. It can meet the requirements of energy system cleaning and decarbonization while improving the operation economy, which verifies the effectiveness of the proposed environmental economic dispatch model.
With the proposal of the national “30·60″dual carbon targets (Guo et al., 2022; Zhou et al., 2022), the continuous promoting of the industrial and energy structure adjustment, the advocacy of green, environmentally friendly, and low-carbon lifestyles, as well as the acceleration construction of the new type power system featuring new energy (Wang et al., 2019; Hu et al., 2021; Wang et al., 2022), have pointed out the direction for the development of the energy and power industry. However, since the new energy possesses the characteristics of randomness, fluctuation, and intermittency (Teleke et al., 2010; Da Cunha et al., 2021; Yang et al., 2022), its large-scale and high proportion of access has posed great threat on the operational economy and power supply reliability of the power system (Yin et al., 2019). At present, the urgent need on the improvement of the new energy consumption rate, the source-grid-load-storage link coordination, and the complementarity of various types of power sources is becoming increasingly prominent. It is of great significance to fully explore and optimize the flexible adjustment ability of multiple types of resources, and promote the transformation of the power balance mode from the traditional “source changes according to load, load changes according to power grid” to the “source-grid-load-storage multiple coordinated scheduling system”, thus promoting the mutual assistance of various types of energy sources (Gong and Wang, 2021), improving the consumption rate of new energy and the economic operation of the power system.
At present, scholars from home and abroad have conducted in-depth and extensive research on the joint optimization scheduling strategy of power system involving clean energy sources such as wind, solar and hydro. Literature (Wang et al., 2013) considers the nature of wind, hydro and thermal power, and establishes a multi-objective optimization model for the coordinated scheduling of wind-hydro-thermal power systems with the goal of improving the consumption rate of new energy, reducing system operating costs, and improving the stability of thermal generators, but does not take into account the effect of the network cross-section power limit on the consumption of renewable energy. Literature (Wang et al., 2023) in order to address the issues of randomness and volatility caused by the high integration of renewable energy into the grid, focuses on the wind-solar-hydro energy storage joint optimal scheduling strategy of a new power system using an improved particle swarm optimization algorithm to enhance renewable energy utilization. Literature (Gejirifu et al., 2022; Gu et al., 2022) take the lowest comprehensive operation cost and the largest amount of new energy consumption as the objective function, and establish a joint scheduling model to realize the multi-energy complementary operation of the wind-photovoltaic-thermal-energy storage system. Literature (Liantao et al., 2021) takes the maximum economic benefits of hybrid energy system as the objective function, builds a day-ahead scheduling optimization strategy for power systems with pumped storage, wind power, photovoltaic and thermal-electricity units, aims at reducing pollution control costs and improving the output capacity and stability of thermal power units. Literature (Espinosa-Juárez et al., 2020) considers wind power, photovoltaic power output forecasting, and load demand forecasting, and proposes a multi-objective environmental and economic dispatch strategy for power systems with new energy and storage systems, effectively reducing the power generation cost and pollutant emissions of traditional thermal power units. Literature (Jiang et al., 2022) considers factors such as system rotation reserve and network loss, and transforms the multi-objective dynamic environmental economic dispatch problem of wind-solar connected power systems into a single objective optimization problem by introducing normalization coefficients, reducing power generation costs and pollution emissions. The above studies have realized the joint optimal scheduling of the power system by selecting several power sources to form a hybrid power system, but the types of power sources are small, usually containing only three or four types of power sources, and there are few hybrid systems containing five types of power sources or more, which fail to comprehensively cover all the resources of the hybrid power system.
In addition, the joint optimal scheduling of new power systems containing renewable energy sources at home and abroad usually focuses on economic scheduling. Literature (Sheng and Zhang, 2016) establishes a multi-objective optimization model for the coordinated scheduling of wind-hydro-thermal power systems with the goal of improving the consumption rate of new energy, reducing system operating costs, and improving the stability of thermal power units. Literature (Li et al., 2020) proposes a scheduling strategy for integrating wind-solar-storage system to smooth the load curve and suppress load fluctuations by introducing a multi-objective function. Literature (Peng et al., 2016) in order to deal with the uncertainty of large-scale intermittent power supply, a flexible robust optimization with adjustable uncertainty budget dispatch model is built for hybrid power system to achieve coordination between reliability and economy. Literature (Efecik and Wang, 2023) constructs the objective function based on the minimum dispatching cost of the generators within the grid, and proposes an economic dispatch model for an energy storage system integrated into a modern power grid to improve the grid stability while reducing costs. Literature (Lu et al., 2020) proposes dynamic economic dispatch strategy with optimal transmission switching for wind integrated power systems to improve wind power consumption and reduce system operating costs. Literature (Bai et al., 2011) studies the economic benefits brought by the energy conversion of wind, PV and small hydro power, and the environmental benefits of energy saving and emission reduction brought by the development of clean energy. However, the traditional joint dispatch of power systems usually focuses on economic efficiency as the optimization objective, with less consideration of the impact of pollutants such as SO2 and NOx emissions on the natural ecological environment (Dong et al., 2009; Geng et al., 2015; Manojkumar and Singh, 2018), and is unable to meet the requirements of energy system cleanliness and low-carbon goal.
In response to the above problems, based on the analysis of the complementary characteristics of wind-solar-hydro-thermal-storage multi-source economy, this article establishes a hierarchical environmental economic dispatch model for the power system with the goal of achieving the highest consumption rate of new energy, minimizing net load fluctuations, system operating costs, and pollutant emissions (Van Hong et al., 2021). Simulation analysis is conducted on an improved IEEE 6-machine 30-node system. The results show that the scheduling strategy can effectively improve the level of flexible consumption of new energy, meanwhile minimizing the fluctuation and peak valley difference of the net load curve, maximizing environmental and economic benefits.
Figure 1 shows the structure of a wind-solar-hydro-thermal-storage multi-source complementary power system, which is composed of conventional units (thermal power units, hydropower units, etc.), new energy units (photovoltaic power plants, wind farms, etc.), energy storage systems, and loads. The system operates collaboratively and various types of power sources are complementary.
Wind power is currently the most economical and mature new energy generation technology. The prediction of power generation is relatively mature, with a small probability of significant fluctuations in the minute level time scale, while time scales above the hour level have significant volatility. The output of wind power is usually related to the wind speed, and the fluctuation in each wind farm usually has prominent time shift characteristics due to different installation locations, joint scheduling of various wind farms can effectively smooth the output power and improve the level of wind power consumption.
The variation of wind power output and photovoltaic power output has a natural complementary characteristic in time scale. Wind farms typically have lower output during the day and higher output at night, which is highly complementary to solar power that operate normally during the day. In clear weather, the output of the solar power increases, while the wind power is relatively small. Vice versa, during cloudy and rainy weather, the solar power output decreases, while the wind increases. Joint scheduling of wind farms and solar power stations can effectively improve the reliability of power supply.
China has rich reserves of hydraulic resources, but due to the significant monsoon climate, the distribution of runoff time in most rivers is uneven, showing a characteristic of more in summer and autumn, and less in winter and spring. The overall seasonal characteristics of wind power output in China are high in winter and spring, and low in summer and autumn, the output of wind power and hydropower also have natural complementary characteristics in terms of time. At the same time, hydropower units start and stop quickly and have fast regulation speed. If the hydropower station and wind farm are combined for operation, it can not only smooth the integrated output of wind and solar power to a certain extent, but also improve the peak shaving capacity of hydropower.
As a stable power source, thermal power units are the main source of China’s electricity supply, with the advantages of being unaffected by natural conditions and high controllability of output. They can suppress the randomness and fluctuation of new energy through start-stop peak shaving and output peak shaving, and play an inertial supporting role in power scheduling considering the complementary characteristics of new energy. Thermal power undertakes the tasks of base load, frequency regulation, peak shaving, and backup.
The energy storage system has fast response speed, large peak shaving amplitude, and strong power throughput ability due to its power transferring ability. It fully utilizes the fast charging and discharging characteristics to achieve “peak shaving and valley filling”, reducing the load peak valley difference, avoiding frequent startup and shutdown of thermal power units or long-term operation in deep peak shaving state, improving the consumption rate of new energy and the safety and economy of power system.
In summary, although significant difference exists in the operating characteristics of various types of power sources, they have strong complementarity. By coordinating and interacting with the source, grid, load and storage, and complementing various types of sources, reasonable arrangements for the joint optimization of the power system operation will bring about significant environmental and economic benefits.
Based on the above analysis of the wind-solar-hydro-thermal-storage multi-source complementary characteristics, considering power balance constraints, reserve capacity constraints, and related constraints on the operation of various energy sources, a hierarchical environmental economic dispatching model of the power system is established with the goal of achieving the highest consumption rate of new energy, minimizing net load fluctuation, system operating costs, and pollutant emissions. Based on the differences and complementarities in multi-type power sources, the principles and objectives of the hierarchical environmental economic dispatching strategy are: wind-solar power integrated generation, priority scheduling, full consumption, and improving the level of new energy consumption. To cope with the randomness, intermittency and anti-peak shaving characteristics of wind and solar integrated output, and to avoid frequent start and stop or long-term operation of the thermal power unit in deep peak shaving state, the net load curve is smoothed by adjusting the output of the hydropower station and the charging and discharging status of the energy storage devices, so as to minimize the fluctuation of the net load curve. Assuming that the operating costs of wind farms, solar power stations, and hydropower stations are zero, taking into account the coal consumption cost, startup and shutdown cost, energy storage system operating cost, and pollutant emissions of thermal power units, the startup and shutdown mode and output size of thermal power units are determined to maximize the environmental and economic benefits. The hierarchical environmental and economic dispatching strategy for power systems is shown in Figure 2.
In the upper level model, wind-solar power integrated generation, priority scheduling, full consumption, and improving the level of new energy consumption.
As shown in Eq. 1,
In the middle level model, in order to cope with the randomness, intermittency and anti-peak shaving characteristics of wind and solar integrated output, the net load curve is smoothed by adjusting the output of the hydropower station and the charging and discharging status of the energy storage devices, and the fluctuating variance
Where, T is the dispatching period,
In the lower level model, the system operation cost
Where, NG is the number of thermal units;
Since the inherent conflict between minimizing system operating costs and pollutant emissions in Eq. 3, these two objective functions cannot be minimized simultaneously. Generally speaking, multiple objective functions can be transformed into a single objective function problem by assigning different weights to each function using the weighted coefficient method (Liang et al., 2019).
As shown in Eq. 4,
As shown in Eq. 5,
As shown in Eq. 6,
As shown in Eq. 7,
As shown in Eq. 8,
As shown in Eq. 10,
As shown in Eq. 11,
As shown in Eq. 12,
Adjustable hydropower plants usually undertake the comprehensive utilization tasks of power generation, flood control, breeding, water supply and so on, in order to ensure the overall social and ecological benefits, it is necessary to arrange the amount of water used for power generation according to the requirements of the reservoir scheduling department. Therefore, in this paper, the water use for power generation allocated by the reservoir dispatch department is used in the constraints as the upper and lower limits of the daily flow integral (Wang et al., 2013). The hydropower plant can ensure the lowest amount of water abandoned in the range of daily flow integral while satisfying the maximum technical output constraint, as shown in Eq. 13.
Where, A is hydro-electric conversion constant, usually taken as 9.81;
As shown in Eq. 14,
The energy storage system should satisfy the charging and discharging power constraints along with the upper and lower limit constraints on the state of charge (SOC) of the energy storage system, the output depends on the SOC and charging and discharging behaviors of the previous moment, and the value of the SOC of the energy storage system at the end of the scheduling cycle should be the same as the initial moment, as shown in Eq. 15.
Where,
To verify the effectiveness of the hierarchical environmental and economic dispatching model proposed in this article, an improved IEEE 6-machine-30-nodes system is used for simulation analysis, including six thermal units, two wind farms, one solar power station, one hydropower plant, and two sets of lead-acid batteries.
The scheduling period in this article is set to 24 h, with each hour as a scheduling period. The wind farm is a large-scale centralized one composed of multiple wind turbines. The installed capacity of wind farm one is 500 MW, wind farm two is 270 MW, and solar power station is 150 MW. The predicted output of a typical daily wind farm and solar power station can be found in reference (Xiong et al., 2013), and the predicted load values can be found in reference (Ting et al., 2006).
The cost and emission coefficient of thermal units are shown in Table 1 (Luo et al., 2014; Yin et al., 2019). The adjustment range of the output of a hydro-electric unit within a certain period can directly reach the maximum output from the shutdown state, without considering the constraints of climbing speed and minimum startup/shutdown time. The assembled capacity of the hydro-electric motor is 200 MW, relevant parameters can be found in reference (Wang et al., 2013). As a relatively mature electrochemical energy storage technology, lead-acid batteries have the advantages of low cost and reliable safety. However, the operating and maintenance costs are relatively high. At the same time, it is necessary to avoid a decrease in the usable capacity of lead-acid batteries caused by deep discharge. The operating and maintenance cost of lead-acid batteries is 7 $/MW, relevant parameters can be found in reference (Liantao et al., 2021).
The hierarchical environmental and economic dispatching of power systems based on the complementary combination of multiple sources belongs to a high latitude, multi-objective, and nonlinear optimization problem. Therefore, the MATLAB platform and YALMIP + GRUOBI kit are used to solve and determine the strategy with the highest environmental and economic benefits.
According to the hierarchical environmental and economic dispatching model and relevant basic data and parameters, in the upper model, the time shift characteristics of wind power output and the complementary characteristics of wind farm and solar power station output are fully utilized. The wind power and solar power station complement each other to achieve integrated output, priority scheduling, full consumption, and improve the flexible consumption rate of new energy. The load values and predicted output of wind and solar power at each period are shown in Figure 3.
Due to the fluctuation and anti-peak shaving characteristics of the integrated output of wind-solar power, the fluctuation and peak valley difference of the net load curve will be large, which will cause frequent startup and shutdown of thermal units or its long-term operation in deep peak shaving state. In middle model, the net load curve is smoothed by flexibly adjusting the output of the hydropower and the charging and discharging status of the lead battery. The optimized scheduling curves for the four scenarios are shown in Figures 4–6.
Scenario 1: Hydropower plants and lead-acid batteries are not involved in net load balancing.
Scenario 2: Hydropower plants participate in net load balancing only.
Scenario 3: Lead acid batteries participate in net load balancing only.
Scenario 4: Hydropower and lead-acid batteries jointly participate in net load balancing.
Comparative analysis of different scenarios through Table 2 and Figure 7, scenario one hydropower station and lead battery are not involved in the net load smoothing, the load fluctuation variance is 73286, load peak-to-valley difference is 875 MW. Although it can improve the level of new energy flexible consumption and save the hydropower station and lead battery operating costs at the same time, due to the integration of wind and solar output of random volatility and anti-peak characteristics of significant, large load fluctuation variance and peak-to-valley difference will cause thermal power units to frequently start and stop or long time running in the depth of the peaking state, which is not conducive to the economic operation of the power system.
Scenario two hydropower station participates in net load smoothing, it can reduce the load fluctuation variance and load peak-to-valley difference to a certain extent, in which the load fluctuation variance is 35611 and the load peak-to-valley difference is 675 MW. Compared with Scenario 1, the load fluctuation variance is reduced by 37675, and the peak-to-valley difference of the load is reduced by 200 MW. Although the hydropower units have the characteristics of both rapid start-stop and fast regulation speeds, which can effectively deal with the anti-peak characteristics of wind and solar integrated output and thus reduce the fluctuation variance of load, the capacity of hydropower station to “fill in the valley” is insufficient, and the load peak-to-valley difference is still large after smoothing.
Scenario 3 lead battery participates in net load smoothing, load fluctuation variance and load peak-to-valley difference can be effectively reduced by flexibly adjusting the charging and discharging state of lead battery, in which the load fluctuation variance is 49373, and the load peak-valley difference is 635 MW. Compared with Scenario 2, due to the significant role of lead battery in “peak shaving and valley filling”, the peak regulation amplitude is large, the power throughput capacity is strong, and the load peak-to-valley difference is reduced by 5.9%. But due to the capacity of lead battery, its ability to reduce the load fluctuation variance is limited, the load fluctuation variance is increased by 38.6%, and the load fluctuation variance is still large after smoothing.
In summary, when hydropower station and lead battery are involved in net load smoothing respectively, although both can smooth the integrated wind and solar power output to a certain extent, they are unable to significantly reduce the load fluctuation variance and load peak-to-valley difference due to the large differences in their operating characteristics.
Scenario 4 hydropower station and lead battery jointly participate in net load smoothing, the complementary advantages of the hydropower station and lead battery can be fully exploited, and the ability to reduce the load fluctuation variance and load peak-to-valley difference is significant, in which the load fluctuation variance is 19125 and the load peak-to-valley difference is 435 MW, which is reduced by 46.3% and 31.5% compared with Scenario 2 and Scenario 3, respectively. Therefore, by complementing various types of energy sources, the net load curve is smoothed by adjusting the output of the hydropower station and the charging and discharging status of the lead battery, thus minimizing the volatility of the net load curve.
As shown in Figure 6, the net load curve after smoothing is generally stable, with periods 1-8 being the valley and 16–21 being the peak. This can effectively avoid frequent startup and shutdown of thermal units or long-term operation in deep peak shaving state, so as to maintain the output of each unit as stable as possible and improve the operating efficiency. In the lower model, considering the coal consumption cost, startup and shutdown cost, energy storage system operation cost, and pollutant emissions of thermal units, the startup and shutdown mode and output size of thermal power units are determined with the objective functions of the lowest system operation cost, pollutant emissions, and the highest environmental and economic benefits. The simulation results of different scheduling strategies are shown in Table 3.
By comparing and analyzing the simulation results of different scheduling strategies in Table 3, it is clear that economic and environmental dispatching strategies can both minimize the system operating cost and pollutant emissions (Bedrinana et al., 2007; Chansareewittaya, 2018), with a minimum operating cost of 174040 $ and a minimum pollutant emission of 8454.3 kg respectively. According to the characteristics of the operating mode of thermal units, minimizing system operating cost and pollutant emissions are fundamentally conflicting, namely, minimizing one evaluation indicator is inevitably at the expense of sacrificing the other. Although economic dispatching can minimize system operating cost, pollutant emissions will significantly increase, compared with environmental dispatching pollutant emissions increased by 6083.7 kg, failing to meet the requirements of system cleanliness and low-carbon. Similarly, although environmental dispatching can minimize pollutant emissions, the system operating costs will increase by 17250 $ compared to the economic dispatching, which does not meet the requirements of the power system operating economics. Therefore, these two objective functions of the system cannot be minimized simultaneously. In the environmental and economic dispatching strategy, different weights (compromise factor
From Figures 8, 9, it can be seen that thermal units G1, G4, and G5 are always in operation during the dispatching period, thermal unit G2 is in operation during the 9–24 period, and thermal units G3 and G6, as cold standby units, stop operating during the dispatching period. In environmental and economic dispatching, the overall output of each thermal unit is stable, which can effectively avoid frequent startup and shutdown of units or long-term operation in deep peak shaving state, thereby improving the operational efficiency. The environmental and economic dispatching strategy comprehensively considers the system operating cost and pollutant emissions, reduces pollutant emissions with smaller system operating cost, improving the cleanliness and low-carbon of the system. The system operating cost is 179430 $, the pollutant emissions is 9876.4 kg, and the environmental economic benefits is 153460, which is 5.1% and 3.6% higher compared to the traditional economic dispatching and environmental dispatching strategies, respectively. The system achieves the highest environmental and economic benefits.
Based on the complementary characteristics of wind, solar, hydro, thermal, and storage energy sources, a hierarchical environmental and economic dispatching model for power systems was established with the goals of achieving the highest consumption rate of new energy, minimizing the net load fluctuations, system operating cost, and pollutant emissions. An improved IEEE 6-machine 30-node system was used as an example for numerical analysis, and the results are shown below:
1) Fully tapping into the flexible regulation ability of multi-source complementarity, wind and solar power complementarity, priority scheduling, and full consumption can effectively improve the consumption rate of new energy;
2) The joint participation of hydropower and lead-acid batteries in net load balancing can effectively cope with the randomness, intermittency and anti-peak shaving characteristics of wind-solar integrated output, avoiding frequent startup and shutdown or long-term operation of the thermal unit in deep peak shaving state, the fluctuation variance of the net load curve after smoothing is 19125, and the peak-to-valley difference is 435 MW, which is 46.3% and 31.5% lower compared to the participation of hydropower station and lead battery in net load smoothing, respectively;
3) Through the weighted coefficient method to achieve the unity of the two objective functions of system operating cost and pollutant emissions, the startup and shutdown mode and the output of thermal units can be determined. This can not only meet the requirements of clean and low-carbon energy systems, but also improve operational economy. The system operating cost is 179430 $, and the pollutant emissions is 9876.4 kg, which represents a 5.1% increase in environmental and economic benefits compared to the traditional economic dispatch strategy.
Although this paper considers system power balance constraints, system reserve capacity constraints, and constraints related to the operation of various types of energy sources such as wind, solar, hydro, thermal, and storage, it does not take into account network losses and network constraints, and the system is considered to be capable of accepting a certain percentage of wind and solar fully into the grid. In the subsequent work, it is necessary to consider the ultra-short-term prediction errors of loads and output power of wind farms and photovoltaic power plants, and to study the rolling intra-day optimal scheduling strategy for short time scales of source-grid-load-storage.
The original contributions presented in the study are included in the article/Supplementary material, further inquiries can be directed to the corresponding author.
XL: Conceptualization, Methodology, Writing–original draft, Writing–review and editing. KW: Data curation, Writing–review and editing. MX: Data curation, Investigation, Validation, Writing–review and editing. MF: Writing–review and editing. SM: Supervision, Validation, Writing–review and editing.
The author(s) declare financial support was received for the research, authorship, and/or publication of this article. The authors appreciate the financial support provided by the Science and Technology Project of China Electric Equipment Group Co., Ltd. “Research on Key Technologies and Product Development of the New Generation Dispatching System for Collaborative Control of Source Network Load Storage in High Ratio New Energy Power Systems” (CEE-2022-B-01-03-001-XJ).
Authors XL, KW, and MF were employed by Xuchang Xuji Software Technology Co., Ltd.
The remaining authors declare that the research was conducted in the absence of any commercial or financial relationships that could be construed as a potential conflict of interest.
The authors declare that this study received funding from the Science and Technology Project of China Electric Equipment Group Co., Ltd. The funder had the following involvement in the study: study design, data collection and analysis, decision to publish, preparation of the manuscript.
All claims expressed in this article are solely those of the authors and do not necessarily represent those of their affiliated organizations, or those of the publisher, the editors and the reviewers. Any product that may be evaluated in this article, or claim that may be made by its manufacturer, is not guaranteed or endorsed by the publisher.
Bai, X., Yuan, Y., and Fu, Z. (2011). Economical and environmental benefits of grid-connected small hydropower-wind power-photovoltaic power. Power Syst. Clean Energy 27 (6), 75–80.
Bedrinana, M. F., Bosco, J. A., Murari, C. A. F., and Castro, C. A. (2007). “Decisions in power system operation based on fuzzy economic and environmental dispatch,” in 2007 IEEE Lausanne Power Tech, Lausanne, Switzerland, 1296–1301. doi:10.1109/PCT.2007.4538503
Chansareewittaya, S. (2018). “Hybrid differential evolutionary/tabu search for economic dispatch and environmental dispatch,” in 2018 15th International Conference on Electrical Engineering/Electronics, Computer, Telecommunications and Information Technology (ECTI-CON), Chiang Rai, Thailand, 9–12. doi:10.1109/ECTICon.2018.8619978
Da Cunha, P. H. C., Saavedra, O. R., and Da Silva, G. S. (2021). “Economic dispatch of fast reserves including storage systems to compensate wind ramps,” in 2021 IEEE PES Innovative Smart Grid Technologies Conference - Latin America (ISGT Latin America), Lima, Peru, 1–5. doi:10.1109/ISGTLatinAmerica52371.2021.9543048
Dong, J., Ma, B., and Cheng, Q. (2009). “Environmental and economical coherence of energy-saving dispatch based on environmental cost internalization,” in 2009 International Conference on Energy and Environment Technology, Guilin, China, 129–133. doi:10.1109/ICEET.2009.38
Efecik, K., and Wang, X. (2023). “Economic dispatch of energy storage systems for smart power grid,” in 2023 IEEE Transportation Electrification Conference and Expo (ITEC), Detroit, MI, 1–4. doi:10.1109/ITEC55900.2023.10186941
Espinosa-Juárez, E., Solano-Gallegos, J. L., and Ornelas-Tellez, F. (2020). “Environmental-economic dispatch with renewable sources forecasting and energy storage,” in 2020 International Confer-ence on Computational Science and Computational Intelligence (CSCI), Las Vegas, NV, 1372–1377. doi:10.1109/CSCI51800.2020.00255
Gejirifu, D., Fuqiang, L., Xueqin, T., Jing, Z., Yujie, G., Wenxuan, L., et al. (2022). “Dispatching strategy of joint wind, photovoltaic, thermal and energy storage considering utilization ratio of new energy,” in 2022 4th International Conference on Smart Power and Internet Energy Systems (SPIES), Beijing, China, 1655–1660. doi:10.1109/SPIES55999.2022.10082154
Geng, Z., Chen, Q., Chen, X., Xia, Q., Li, J., Wang, Y., et al. (2015). “Environmental economic dispatch towards multiple emissions control coordination considering a variety of clean generation technologies,” in 2015 IEEE Power and Energy Society General Meeting, Denver, CO, 1–5. doi:10.1109/PESGM.2015.7286311
Gong, C., and Wang, Y. (2021). “Research on scheduling of wind solar energy storage microgrid considering new energy consumption,” in 2021 3rd International Academic Exchange Conference on Science and Technology Innovation (IAECST), Guangzhou, China, 1630–1633. doi:10.1109/IAECST54258.2021.9695529
Gu, J., Li, X., Niu, Y., and Wang, X. (2022). “Research on day-ahead optimal scheduling of wind-photovoltaic-thermal-energy storage combined power generation system based on opportunity-constrained programming,” in 2022 IEEE/IAS Industrial and Commercial Power System Asia (I&CPS Asia), Shanghai, China, 607–612. doi:10.1109/ICPSAsia55496.2022.9949648
Guo, X., Chen, B., Chi, J., Miao, Q., Zhang, Y., and Tao, Y. (2022). “Economic optimal dispatch of microgrid with P2G and multi-type energy storage to promote renewable energy consumption,” in 2022 5th International Conference on Power and Energy Applications (ICPEA), Guangzhou, China, 515–519. doi:10.1109/ICPEA56363.2022.10052448
Hu, H., Wen, L., and Zheng, K. (2021). “Low carbon economic dispatch of multienergy combined system considering carbon trading,” in 2021 11th International Conference on Power and Energy Systems (ICPES), Shanghai, China, 838–843. doi:10.1109/ICPES53652.2021.9683862
Jiang, C., Xiong, G., Chen, J., Yang, J., and Liang, J. (2022). Hybrid optimization method for dynamic environmental economic dispatch of wind-solar power system. Electr. Power Sci. Eng. 38 (09), 8–15. doi:10.3969/j.ISSN.1672-0792.2022.09.002
Liang, C., Yu, K., and Xie, J. (2019). “A consensus-based distributed environmental-economic multi-objective dispatch strategy for combined heat and power generation systems,” in 2019 IEEE 3rd Conference on Energy Internet and Energy System Integration (EI2), Changsha, China, 1631–1636. doi:10.1109/EI247390.2019.9062072
Liantao, J., Pu, W., Deshun, W., Bo, Y., Jun, Z., Ze, L., et al. (2021). “Research on economic optimal dispatch of pumped storage-new energy-thermal power hybrid system,” in 2021 IEEE Sustainable Power and Energy Conference (iSPEC), Nanjing, China, 939–943. doi:10.1109/iSPEC53008.2021.9735452
Li, T., Li, Z., Yang, J., Cui, D., Wang, Z., Ma, K., et al. (2020). Coordination and optimal scheduling of multi-energy complementary system considering peak regulation initiative. Power Syst. Tech-nology 44 (10), 3622–3630. doi:10.13335/j.1000-3673.pst.2020.0626
Lu, M., Zhou, M., and Li, Y. (2020). “Dynamic economic dispatch of power systems with optimal transmission switching,” in 2020 IEEE 1st China International Youth Conference on Electrical Engineering (CIYCEE), Wuhan, China, 1–6. doi:10.1109/CIYCEE49808.2020.9332555
Luo, C., Yang, J., Sun, Y., Lin, F., and Cui, M. (2014). Dynamic economic dispatch of wind integrated power system considering optimal scheduling of reserve capacity. Proc. CSEE 34 (34), 6109–6118. doi:10.13334/j.0258-8013.pcsee.2014.34.012
Manojkumar, T., and Singh, N. A. (2018). “Solution of environmental/ economic(EED) power dispatch problem using particle swarm optimization technique,” in 2018 International Conference on Control, Power, Communication and Computing Technologies (ICCPCCT), Kannur, India, 347–351. doi:10.1109/ICCPCCT.2018.8574256
Peng, C., Xie, P., Pan, L., and Yu, R. (2016). Flexible robust optimization dispatch for hybrid wind/photovoltaic/hydro/thermal power system. IEEE Trans. Smart Grid 7 (2), 751–762. doi:10.1109/TSG.2015.2471102
Sheng, S., and Zhang, L (2016). An economical scheduling strategy in power system based on integration of wind, photovoltaic, water and thermal power. Electr. Meas. Instrum. 53 (22), 66–71.
Teleke, S., Baran, M. E., Bhattacharya, S., and Huang, A. Q. (2010). Optimal control of battery energy storage for wind farm dispatching. IEEE Trans. Energy Convers. 25 (3), 787–794. doi:10.1109/TEC.2010.2041550
Ting, T. O., Rao, M. V. C., and Loo, C. K. (2006). A novel approach for unit commitment problem via an effective hybrid particle swarm optimization. IEEE Trans. Power Syst. 21 (1), 411–418. doi:10.1109/tpwrs.2005.860907
Van Hong, T. P., Ngoc, D. V., and Dang Tuan, K. (2021). “Environmental economic dispatch using stochastic fractal search algorithm,” in 2021 International Symposium on Electrical and Electronics Engineering (ISEE), Ho Chi Minh, Vietnam, 214–219. doi:10.1109/ISEE51682.2021.9418796
Wang, C., Zhao, H., Wang, Y., Wu, Z., Shen, Z., Liang, Y., et al. (2022). “Coordinated economic dispatch of wind-photovoltaic-thermal-storage system considering the environmental cost,” in 2022 4th International Conference on Power and Energy Technology (ICPET), Beijing, China, 677–682. doi:10.1109/ICPET55165.2022.9918519
Wang, J., Dong, R., Zhang, X., Xu, Z., Liu, H., Qiu, J., et al. (2019). “Microgrid optimal dispatch model considering energy storage system,” in 2019 IEEE 3rd Conference on Energy Internet and Energy System Integration (EI2), Changsha, China, 1665–1669. doi:10.1109/EI247390.2019.9061943
Wang, Z., Wu, X., Fang, Z., Xia, L., and Li, L. (2023). “Research the optimization dispatch method of new power systems with wind-solar-hydro storage,” in 2023 3rd International Conference on Energy, Power and Electrical Engineering (EPEE), Wuhan, China, 1242–1246. doi:10.1109/EPEE59859.2023.10351998
Wang, K., Luo, X., Wu, L., and Liu, X. (2013). Optimal dispatch of wind-hydro-thermal power system with priority given to clean energy. Proc. CSEE 33 (13), 27–35. doi:10.13334/j.0258-8013.pcsee.2013.13.010
Xiong, H., Xiang, T., Chen, H., Lin, F., and Su, J. (2013). Research of fuzzy chance constrained unit commitment containing large-scale intermittent power. Proc. CSEE 33 (13), 36–44. doi:10.13334/j.0258-8013.pcsee.2013.13.003
Yang, D., He, C., Wang, T., and Zhang, M. (2022). “Optimal economic dispatch of microgrid with battery energy storage considering uncertainty of renewable generation,” in 2022 4th International Conference on Power and Energy Technology (ICPET), Beijing, China, 854–859. doi:10.1109/ICPET55165.2022.9918463
Yin, Q., Wang, Z., Sun, T., Li, W., Chong, F., et al. (2019). “Environmental and economic dispatch with photovoltaic and wind power,” in 2019 IEEE Sustainable Power and Energy Conference (iSPEC), Beijing, China, 1108–1112. doi:10.1109/iSPEC48194.2019.8975242
Zhou, G., Wang, Z., Wang, S., Jiang, W., and Cai, J. (2022). “Economic dispatch modeling of multi-energy flow coupled network considering hydrogen energy storage,” in 2022 IEEE 2nd International Conference on Mobile Networks and Wireless Communications (ICMNWC), Tumkur, Karnataka, India, 1–6. doi:10.1109/ICMNWC56175.2022.10032024
Keywords: source network load storage, wind-solar-hydro-thermal-storage, complementary combination, environmental and economic dispatch, multi-objective optimization
Citation: Li X, Wang K, Xu M, Fu M and Miao S (2024) Environmental and economic dispatching strategy for power system with the complementary combination of wind-solar-hydro-thermal-storage multiple sources. Front. Energy Res. 12:1338657. doi: 10.3389/fenrg.2024.1338657
Received: 15 November 2023; Accepted: 28 February 2024;
Published: 19 March 2024.
Edited by:
Jun Ma, University of Bristol, United KingdomReviewed by:
Kenneth E. Okedu, Melbourne Institute of Technology, AustraliaCopyright © 2024 Li, Wang, Xu, Fu and Miao. This is an open-access article distributed under the terms of the Creative Commons Attribution License (CC BY). The use, distribution or reproduction in other forums is permitted, provided the original author(s) and the copyright owner(s) are credited and that the original publication in this journal is cited, in accordance with accepted academic practice. No use, distribution or reproduction is permitted which does not comply with these terms.
*Correspondence: Shihong Miao, c2htaWFvQGh1c3QuZWR1LmNu
Disclaimer: All claims expressed in this article are solely those of the authors and do not necessarily represent those of their affiliated organizations, or those of the publisher, the editors and the reviewers. Any product that may be evaluated in this article or claim that may be made by its manufacturer is not guaranteed or endorsed by the publisher.
Research integrity at Frontiers
Learn more about the work of our research integrity team to safeguard the quality of each article we publish.