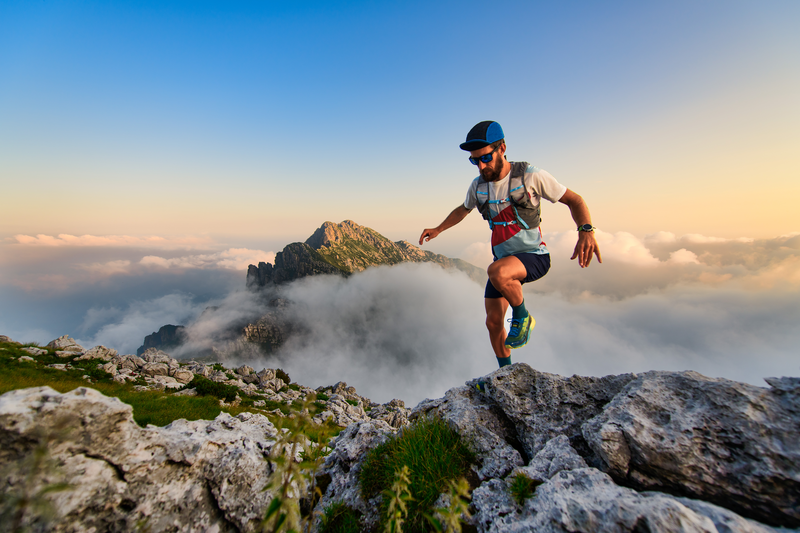
94% of researchers rate our articles as excellent or good
Learn more about the work of our research integrity team to safeguard the quality of each article we publish.
Find out more
OPINION article
Front. Energy Res. , 05 April 2024
Sec. Nano Energy
Volume 12 - 2024 | https://doi.org/10.3389/fenrg.2024.1324866
This article is part of the Research Topic Insights in Nano Energy: 2023 View all 4 articles
Agriculture has always been the cornerstone of the global economy, ensuring the essential livelihoods of people (Pang et al., 2021). Advancements in science and technology have facilitated substantial growth in agriculture (Yu and Wu, 2018; Wang et al., 2020). However, this progress has also caused environmental challenges (Shen et al., 2018). Agricultural groundwater pollution refers to the contamination of groundwater due to the release of detrimental substances stemming from agricultural activities, including nitrogen, phosphorus, veterinary drug residues, and bacteria (Baddam et al., 2016; Zhang et al., 2020). Agricultural wastewater, containing elevated levels of nitrogen and phosphorus, can readily induce water body eutrophication, posing a threat to aquatic life and health. When these substances seep into groundwater, they can damage groundwater quality (Li and Zhang, 1999; Baweja et al., 2020). Additionally, agricultural underground wastewater can easily contaminate drinking water sources and endanger the well-being of residents (Baba and Tayfur, 2011; Jiang et al., 2020). Persistent groundwater pollution undermines the sustainable management of groundwater reservoirs, inflicting irreversible harm on groundwater resources. Consequently, it is imperative to establish a robust system for assessing and monitoring environmental quality. Establishing suitable waste monitoring and treatment systems in remote areas is crucial to ensuring the health of residents and the stability of ecosystems.
Currently, environmental monitoring and pollutant treatment technologies have reached a relatively advanced stage. Various methods for environmental monitoring and waste treatment are continuously being introduced and refined. However, it is worth noting that sewage treatment facilities often come with high construction costs and tend to be concentrated near economically developed areas (Berthouex et al., 1978; Molinos-Senante et al., 2010; Jafarinejad, 2017). Furthermore, these monitoring and treatment technologies rely on a continuous supply of electrical energy. In remote mountainous regions, maintaining a consistent power supply is difficult, which, in turn, hampers the real-time monitoring and treatment of waste and put the health of residents at risk. Therefore, the imperative lies in the development of cost-effective, environmentally friendly, and self-powered wastewater monitoring and treatment systems, which hold significant importance in mitigating groundwater pollution in remote agricultural areas and ensuring the well-being of residents.
In 2012, Wang introduced triboelectric nanogenerator (TENG) technology, which harvest mechanical energy from the environment, and converting it into electrical energy through electrostatic induction and triboelectric electrification principles (Niu and Wang, 2015; Zi et al., 2015; Tinghai et al., 2023). TENG generates charge transfer via friction and the contact separation of dissimilar materials, resulting in electric current generation. TENG possesses advantages such as cost-effectiveness, portability, environmental sustainability, and excellent flexibility (Zhu et al., 2015; Cheng et al., 2019; Dharmasena and Silva, 2019; Wu et al., 2019; Wang, 2020; Zhou et al., 2020; Kim et al., 2021). TENG has demonstrated a diverse array of potential applications encompassing wearable devices (Zou et al., 2020; Wang et al., 2021; Dassanayaka et al., 2022), self-powered sensors (Wang, 2014; Wang S. et al., 2015; Wang Z. L. et al., 2015), smart medicine (Jiang et al., 2021; Parandeh et al., 2021; Chen et al., 2022), environmental monitoring (Chen et al., 2020; Zhao et al., 2020; Chang et al., 2022), and wastewater treatment (Chen et al., 2016; Li et al., 2016; Mo et al., 2022). It is also demonstrated that TENG can be integrated into wearable devices to harvest mechanical energy from the human body, thereby powering small electronic devices. TENG’s environmental sustainability and exceptional flexibility position it as a significant asset in the realm of environmental monitoring. In addition, TENG can detect environmental parameters such as water flow and wind speed, relaying this information through electrical signals. Leveraging TENG’s electrical output enables the degradation of various pollutant types. For instance, electrocatalysis can effectively degrade organic water pollutants, while electrospinning membranes excel at adsorbing particulate matter.
In this paper, we firstly summarized the environmental challenges posed by contemporary agricultural production. Then, we introduced the existing environmental monitoring and sewage treatment technologies and discussed their limitations. Besides, we summarized the working principles and application prospects of TENG and introduced the research about TENG in the realms of environmental monitoring and sewage treatment. Further, we examine the crucial issues and prospects of in the realm of environmental engineering.
TENG primarily relies on the contact separation or friction between two dissimilar materials. In general, materials exhibit varying abilities to gain or lose electrons, which is the basis for TENG’s operation. Electron transfer that driven by differences in electronegativity takes place when two dissimilar materials come into contact or move in relation to each other. After separation of the two materials, an electrical potential difference arises between them. The material is linked to an external circuit via electrodes. To equalize the potential difference, electrons traverse the external circuit, thereby generating an electric current (Luo et al., 2021). Consequently, if the two materials maintain constant contact, separation, or relative motion, the TENG can sustain the generation of current. TENG boasts remarkable energy conversion efficiency, accommodates a broad spectrum of materials, and can be applied in diverse sizes and configurations. Its high sensitivity enables it to detect minor environmental changes (Zhou et al., 2020; Mo et al., 2022; Tinghai et al., 2023). Furthermore, the production process for TENG is exceptionally straightforward, resulting in minimal cost consumption. TENG operates in four distinct modes: contact separation mode, sliding friction mode, single electrode mode, and independent layer mode (Cao et al., 2016).
TENG’s environmental friendliness, cost-effectiveness, and exceptional flexibility confer substantial advantages in environmental monitoring, pollutant treatment, and related domains (Cheng et al., 2019). Firstly, TENG serves as a power source for powering environmental monitoring sensors, enabling their operation in remote regions (Zhang et al., 2019; Liang et al., 2022). Moreover, TENG can construct sensor systems for real-time environmental quality assessment, presenting monitoring results via electrical signals (Chang et al., 2022; Liang et al., 2022). TENG can achieve real-time water quality monitoring by capturing environmental water wave energy. Secondly, the electricity produced by TENG can be employed in electrochemical processes for the degradation of organic wastewater pollutants (Chen et al., 2016). Additionally, it can be integrated with electrospinning membranes to absorb environmental particulate matter. Furthermore, TENG can be seamlessly integrated into wearable devices like masks to provide real-time monitoring of the user’s working environment’s environmental quality (Zou et al., 2020).
The operational principle of TENG demonstrates significant potential for environment quality monitoring. It is important to note that TENG operates based on differing electronegativities between two materials, which can include solids, liquids, and gas. Consequently, solid-liquid TENGs can be engineered to utilize the movement of liquids, inducing friction between materials, and thereby generating electrical signals. Solid-liquid TENG are suitable for monitoring water quality parameters, including water level and ion concentration. In addition, the use of gas-sensitive materials to produce TENGs can enable real-time monitoring of gas concentrations in the environment. The significant advantage of TENG in environmental sustainability enable prolonged monitoring of environmental parameters.
Pollution in aquatic environments poses a severe threat to ecological stability and human health. Due to the rapid expansion of agriculture, the utilization of chemical fertilizers, pesticides, and similar substances has surged, significantly heightening the potential for harm to surface water and groundwater ecosystems. TENG, recognized for its high sensitivity and environmental friendliness, has demonstrated substantial advantages in water quality assessment. Wang et al. developed a direct-current rotating tubular TENG (DC-rTENG) utilizing liquid dielectric contact for sustainable energy harvesting and chemical composition analysis. Figure 1A depicts its structure. They investigated the design, working mechanism, electrical output performance, and potential applications of this new nanogenerator for detecting the chemical composition of liquid dielectrics. Key findings reveal that DC-rTENG can efficiently harvest low-frequency mechanical energy and is capable of analyzing changes in the chemical composition of liquid dielectrics by monitoring its electrical output. Figure 1B illustrates the electrical output of DC-rTENG when detecting different liquids such as hexane, ethanol, and deionized water. This research presents a new method for energy harvesting and chemical composition analysis, and has the potential to significantly affect environmental monitoring, health diagnostics, and additional fields (Wang et al., 2019). Jiang et al. designed a liquid sensor based on liquid-solid basic mode TENG. The specific structure is shown in Figure 1C. Through this sensor, they successfully identified different types of solutions such as HCl aqueous solution, deionized water, and NaOH. The identification signals are shown in Figure 1D (Jiang et al., 2019). Chen et al. introduced a highly flexible capillary TENG (ct-TENG), a microfluidic sensor with the unique ability to perform non-destructive and highly flexible microliter sampling (with a sampling volume of 0.5 µL). The ct-TENG comprises an ultra-fine tubular sandwich structure involving polytetrafluoroethylene capillary tubes, double-helical aluminum foil, and silicone rubber-sealed tubes (Figure 1E). The generator maintains a stable open-circuit voltage of 1.1V, enabling the detection of 0.5 µL of microliquid. The system can differentiate between bottled water, tap water, and deionized water based on the output electrical signal. Furthermore, it is capable of detecting various ion standard solutions (Figure 1F) (Chen et al., 2018).
Figure 1. (A) Structure diagram of DC-rTENG (Wang et al., 2019). (B) Results of DC-rTENG detecting different liquids (Wang et al., 2019). (C) Structural diagram of liquid-solid contact TENG (Jiang et al., 2019). (D) Results of liquid-solid contact TENG detection of different liquids (Jiang et al., 2019). (E) Structure diagram of microfluidic assay (Chen et al., 2018). (F) Standard ion solution test results (Chen et al., 2018). (G) Schematic diagram of TENG-driven electro-Fenton system (Zhu et al., 2021). (H) Result diagram of pollutant degradation by TENG-driven electro-Fenton system (Zhu et al., 2021). (I) Schematic diagram of a self-powered electrocatalytic system (Dong et al., 2022). (J) New material synthesis and system working principle (Wang et al., 2022).
The output of TENG has been shown to drive the normal operation of water quality monitoring sensors. Rui et al. designed a cylindrical TENG with a gate electrode installed inside that can collect water wave energy. The TENG consists of a stator and a rotor. When the TENG is working, there is relative movement between the stator and the rotor, causing friction. They added an arched FEP film to the rotor to improve the output performance of the TENG. The results show that the TENG can successfully drive hydrological monitoring and wireless data transmission systems. This system design is of great significance to the development of water quality monitoring sensors (Rui et al., 2020). Additionally, TENG can also monitor water levels. Li et al. designed a new type of bubble TENG by moving bubbles in a PTFE tube filled with liquid. The steady movement of bubbles in the tube can continuously generate a stable voltage of 17.5V. They studied the effects of motor parameters and bubble parameters on TENG output performance. Integrating this TENG into a water level monitoring system can successfully monitor water level height (Li et al., 2022). Tao et al. used two-dimensional materials with excellent conductivity to fabricate a high-performance TENG and designed a unique multi-layer spring structure. Research shows that the output performance of this TENG is improved by 300% compared with simply using PVDF as a material. They also used the TENG to successfully collect the flow energy of drinking water and realize water level monitoring. This self-powered water level and water quality monitoring system based on TENG is of great significance to the optimization of traditional sensors (Tao et al., 2024). Xuan et al. designed a low-resistance rotating TENG using rabbit hair and FEP brushes and combined it with a memory alloy. Research results show that the TENG can still ensure output stability after 100,000 cycles of operation. They also designed a water temperature monitoring system (Xuan et al., 2023).
In agriculture, the use of pesticides and fertilizers often produces wastewater containing nitrogen, phosphorus, heavy metals, and other toxic substances. In some remote areas, the treatment of such wastewater has become a difficult task because it can easily contaminate drinking water sources and pose a serious threat to human life and health. TENG has shown significant advantages in wastewater monitoring and pollutant treatment due to its remark-able output characteristics and environmental friendliness.
Zhu et al. designed a flexible corrugated TENG to drive an electro-Fenton system for methyl blue degradation. The open-circuit voltage and short-circuit current of this system can reach 610V and 1.93 mA respectively, providing the electric Fenton system with powerful reaction power (Figure 1G). The results showed that the system’s degradation efficiency of methyl blue could reach 98% within 58 min, The degradation results are shown in Figure 1H. This new self-powered electrocatalytic system provides new ideas for the large-scale application of electro-Fenton (Zhu et al., 2021). Dong et al. designed a spherical TENG to increase the generation of free radicals during the electrocatalytic process (Figure 1I). They established a self-powered electrocatalytic system using the DC pulse output of the TENG. The results showed that the ATZ removal rate of this system increased by 8% within 30 min (Dong et al., 2022). Tian et al. designed a spiral spring-type TENG and established a self-powered Fenton system based on it to degrade organic pollutants. The output current of this TENG can reach 700 μA. The results show that the degradation efficiency of malachite green and methylene blue by this system can reach 98% within 80 min (Tian et al., 2021). Wang et al. synthesized a new type of ZnO nanorod array through a hydrothermal method. Using this material to manufacture TENG can greatly increase its output current density (Figure 1J). They used this system to degrade the wastewater pollutant rhodamine, and the results showed that the degradation efficiency was as high as 100%, and it also showed excellent reusability (Wang et al., 2022).
In many remote areas, it is difficult to establish a complete wastewater treatment system and there is not enough electricity supply. TENG can collect and utilize mechanical energy in the environment to realize a self-powered system, which provides a new development direction for wastewater purification in remote areas. Jeon et al. designed a TENG that can collect wind energy and built a self-powered electrocoagulation system based on it. Research results show that this system can effectively remove algae and organic pollutants in water. This system is easy to operate, has a simple structure and is small in scale. It is of extremely important significance for wastewater purification in remote areas (Jeon et al., 2016). In the process of wastewater purification, the most easily collected mechanical energy must be the mechanical energy of water flow. Zhou et al. designed a rotating TENG that can collect water flow energy and achieved efficient removal of hexavalent chromium through the pulse output of the TENG. This also proves the feasibility and effectiveness of self-powered electrochemistry to use harvested energy to clean environmental pollution (Zhou et al., 2019). Furthermore, Li et al. introduced a β-cyclodextrin-based TENG in 2015 (Li et al., 2015), which is capable of harnessing the water wave energy from wastewater and converting it into electricity for the degradation of phenol. The research showed that phenol was ultimately transformed into carbon dioxide and water molecules. Yu et al. proposed a TENG based on graphite microfibers to harvest wind energy and constructed a complete photoelectrocatalytic system (Yu et al., 2015). Similarly, Chen et al. designed a rotating TENG to harvest the mechanical energy from water flow (Chen et al., 2016). This system, connected to an electrode through a rectifier bridge, was used to create a sustainable wastewater treatment device. Experiments with rhodamine solution and copper ion solution demonstrated that the system could completely degrade the rhodamine solution within 15 min, while achieving a 97.3% degradation efficiency of the copper ion solution within 3 h. Moreover, Gao et al. designed a self-contained rotating TENG comprising a stator and a rotor (Gao et al., 2017). By incorporating a rectifier, they established an electrocatalytic system that effectively treated 4-aminoazobenzene. The results revealed that the pollutants were completely mineralized into carbon dioxide during the process.
On the other hand, piezoelectric nanogenerators (PENG) are based on the piezoelectric effect, where certain materials produce charge separation when subjected to external pressure or strain. At the nanoscale, this effect is used to generate electrical energy from pressure or vibration. Piezoelectric nanogenerators are often used for energy harvesting from environmental vibrations or mechanical vibrations. For example, they can be embedded in structures or devices and use surrounding vibrations to generate electrical energy for use in wireless sensors, smart structures, and other fields. PENG has also made great progress in research in the fields of environmental monitoring and pollutant treatment. Mishra et al. designed a piezoelectric catalytic fabric that eliminates the dependence on ultrasonic waves and greatly promotes the application of piezoelectric catalysis in the field of water treatment (Mishra et al., 2019). Tang et al. prepared piezoelectric materials with excellent performance and achieved efficient degradation of TC (Tang et al., 2022). Bagchi et al. prepared NaNbO3 powder through a hydrothermal method, which greatly improved the piezocatalytic efficiency (Bagchi et al., 2020). It is noted that TENG is well-suited for micro-devices and scenarios characterized by frequent frictional motion. On the other hand, PENG excels in vibration environments and demonstrates the capability to deliver relatively high energy density.
Recently, TENG research in environmental engineering has proliferated and advanced rapidly. TENG’s environmental sustainability and cost-effectiveness offer significant advantages in environmental monitoring and pollutant treatment. Nevertheless, certain pivotal issues remain unresolved. In environmental monitoring, the long-term stability of TENG is of paramount importance. Furthermore, extended operation of equipment in challenging aquatic environments, a common requirement for water quality testing, places substantial demands on TENG durability. Additionally, ultra-high-precision parameter monitoring is occasionally required, necessitating enhanced sensitivity and accuracy from TENG. While TENG’s sensitivity offers significant benefits in responding to environmental parameter changes, it is susceptible to environmental interference. Consequently, developing TENG with robust anti-interference capabilities remains a critical challenge for advancing this technology.
Traditional environmental monitoring and waste treatment methods often face challenges in remote areas. TENG’s self-powering capability offers significant advantages in such locations. Implementing a self-powered wastewater monitoring and treatment system addresses the issue of limited power supply in remote regions. Furthermore, TENG is anticipated to find applications in diverse environmental monitoring areas, encompassing not only water quality but also air and soil quality, among other parameters. It can be integrated with compatible intelligent terminals to enable real-time monitoring and transmission of environmental data. Simultaneously, there is an expectation to deploy self-powered wastewater treatment systems utilizing TENG in these regions to harness mechanical energy from the environment for electrochemical pollutant treatment. The discovery of TENG introduces a fresh approach to sewage treatment in remote regions, aligning with the principles of environmental sustainability. TENG exhibits significant potential in wastewater treatment. As technology continues to advance, TENG is anticipated to assume an even more prominent role in wastewater treatment.
WQ: Investigation, Writing–original draft. CZ: Methodology, Writing–review and editing. PL: Conceptualization, Writing–review and editing. WS: Investigation, Writing–review and editing. LG: Conceptualization, Writing–review and editing. GS: Conceptualization, Writing–review and editing. RC: Supervision, Writing–review and editing.
This paper is supported by open funding of Qingdao Key Laboratory of Groundwater Resources Protection and Rehabilitation (DXSKF2022Y02) and Guangdong Province Education Department (Grant No. 2022KTSCX069).
The authors declare that the research was conducted in the absence of any commercial or financial relationships that could be construed as a potential conflict of interest.
All claims expressed in this article are solely those of the authors and do not necessarily represent those of their affiliated organizations, or those of the publisher, the editors and the reviewers. Any product that may be evaluated in this article, or claim that may be made by its manufacturer, is not guaranteed or endorsed by the publisher.
Baba, A., and Tayfur, G. (2011). Groundwater contamination and its effect on health in Turkey. Environ. Monit. Assess. 183 (1), 77–94. doi:10.1007/s10661-011-1907-z
Baddam, R., Reddy, G. B., Raczkowski, C., and Cyrus, J. S. (2016). Activity of soil enzymes in constructed wetlands treated with swine wastewater. Ecol. Eng. 91, 24–30. doi:10.1016/j.ecoleng.2016.01.021
Bagchi, B., Hoque, N. A., Janowicz, N., Das, S., and Tiwari, M. K. (2020). Re-useable self-poled piezoelectric/piezocatalytic films with exceptional energy harvesting and water remediation capability. Nano Energy 78, 105339. doi:10.1016/j.nanoen.2020.105339
Baweja, P., Kumar, S., and Kumar, G. (2020). “Fertilizers and pesticides: their impact on soil health and environment,” in Soil health. Editors B. Giri, and A. Varma (Cham: Springer International Publishing), 265–285.
Berthouex, P. M., Hunter, W. G., and Pallesen, L. (1978). Monitoring sewage treatment plants: some quality control aspects. J. Qual. Technol. 10 (4), 139–149. doi:10.1080/00224065.1978.11980842
Cao, X., Jie, Y., Wang, N., and Wang, Z. L. (2016). Triboelectric nanogenerators driven self-powered electrochemical processes for energy and environmental science. Adv. Energy Mater. 6 (23), 1600665. doi:10.1002/aenm.201600665
Chang, A., Uy, C., Xiao, X., Xiao, X., and Chen, J. (2022). Self-powered environmental monitoring via a triboelectric nanogenerator. Nano Energy 98, 107282. doi:10.1016/j.nanoen.2022.107282
Chen, B. D., Tang, W., He, C., Jiang, T., Xu, L., Zhu, L. P., et al. (2018). Ultrafine capillary-tube triboelectric nanogenerator as active sensor for microliquid biological and chemical sensing. Adv. Mater. Technol. 3 (1), 1700229. doi:10.1002/admt.201700229
Chen, H., Xing, C., Li, Y., Wang, J., and Xu, Y. (2020). Triboelectric nanogenerators for a macro-scale blue energy harvesting and self-powered marine environmental monitoring system. Sustain. Energy and Fuels 4 (3), 1063–1077. doi:10.1039/C9SE01184F
Chen, M., Zhou, Y., Lang, J., Li, L., and Zhang, Y. (2022). Triboelectric nanogenerator and artificial intelligence to promote precision medicine for cancer. Nano Energy 92, 106783. doi:10.1016/j.nanoen.2021.106783
Chen, S., Wang, N., Ma, L., Li, T., Willander, M., Jie, Y., et al. (2016). Triboelectric nanogenerator for sustainable wastewater treatment via a self-powered electrochemical process. Adv. Energy Mater. 6 (8), 1501778. doi:10.1002/aenm.201501778
Cheng, T., Gao, Q., and Wang, Z. L. (2019). The current development and future outlook of triboelectric nanogenerators: a survey of literature. Adv. Mater. Technol. 4 (3), 1800588. doi:10.1002/admt.201800588
Dassanayaka, D. G., Alves, T. M., Wanasekara, N. D., Dharmasena, I. G., and Ventura, J. (2022). Recent progresses in wearable triboelectric nanogenerators. Adv. Funct. Mater. 32 (44), 2205438. doi:10.1002/adfm.202205438
Dharmasena, R. D. I. G., and Silva, S. R. P. (2019). Towards optimized triboelectric nanogenerators. Nano Energy 62, 530–549. doi:10.1016/j.nanoen.2019.05.057
Dong, F., Pang, Z., Lin, Q., Wang, D., Ma, X., Song, S., et al. (2022). Triboelectric nanogenerator enhanced radical generation in a photoelectric catalysis system via pulsed direct-current. Nano Energy 100, 107515. doi:10.1016/j.nanoen.2022.107515
Gao, S., Su, J., Wei, X., Wang, M., Tian, M., Jiang, T., et al. (2017). Self-powered electrochemical oxidation of 4-aminoazobenzene driven by a triboelectric nanogenerator. ACS Nano 11 (1), 770–778. doi:10.1021/acsnano.6b07183
Jafarinejad, S. (2017). Cost estimation and economical evaluation of three configurations of activated sludge process for a wastewater treatment plant (WWTP) using simulation. Appl. Water Sci. 7 (5), 2513–2521. doi:10.1007/s13201-016-0446-8
Jeon, S.-B., Kim, S., Park, S.-J., Seol, M.-L., Kim, D., Chang, Y. K., et al. (2016). Self-powered electro-coagulation system driven by a wind energy harvesting triboelectric nanogenerator for decentralized water treatment. Nano Energy 28, 288–295. doi:10.1016/j.nanoen.2016.08.051
Jiang, M., Lu, Y., Zhu, Z., and Jia, W. (2021). Advances in smart sensing and medical electronics by self-powered sensors based on triboelectric nanogenerators. Micromachines 12 (6), 698. doi:10.3390/mi12060698
Jiang, P., Zhang, L., Guo, H., Chen, C., Wu, C., Zhang, S., et al. (2019). Signal output of triboelectric nanogenerator at oil–water–solid multiphase interfaces and its application for dual-signal chemical sensing. Adv. Mater. 31 (39), 1902793. doi:10.1002/adma.201902793
Jiang, Y., Chen, S., Hu, B., Zhou, Y., Liang, Z., Jia, X., et al. (2020). A comprehensive framework for assessing the impact of potential agricultural pollution on grain security and human health in economically developed areas. Environ. Pollut. 263, 114653. doi:10.1016/j.envpol.2020.114653
Kim, W.-G., Kim, D.-W., Tcho, I.-W., Kim, J.-K., Kim, M.-S., and Choi, Y.-K. (2021). Triboelectric nanogenerator: structure, mechanism, and applications. ACS Nano 15 (1), 258–287. doi:10.1021/acsnano.0c09803
Li, C., Liu, X., Yang, D., and Liu, Z. (2022). Triboelectric nanogenerator based on a moving bubble in liquid for mechanical energy harvesting and water level monitoring. Nano Energy 95, 106998. doi:10.1016/j.nanoen.2022.106998
Li, Y., and Zhang, J. (1999). Agricultural diffuse pollution from fertilisers and pesticides in China. Water Sci. Technol. 39 (3), 25–32. doi:10.2166/wst.1999.0129
Li, Z., Chen, J., Yang, J., Su, Y., Fan, X., Wu, Y., et al. (2015). β-cyclodextrin enhanced triboelectrification for self-powered phenol detection and electrochemical degradation. Energy and Environ. Sci. 8 (3), 887–896. doi:10.1039/C4EE03596H
Li, Z., Chen, J., Zhou, J., Zheng, L., Pradel, K. C., Fan, X., et al. (2016). High-efficiency ramie fiber degumming and self-powered degumming wastewater treatment using triboelectric nanogenerator. Nano Energy 22, 548–557. doi:10.1016/j.nanoen.2016.03.002
Liang, X., Liu, S., Ren, Z., Jiang, T., and Wang, Z. L. (2022). Self-powered intelligent buoy based on triboelectric nanogenerator for water level alarming. Adv. Funct. Mater. 32 (35), 2205313. doi:10.1002/adfm.202205313
Luo, J., Gao, W., and Wang, Z. L. (2021). The triboelectric nanogenerator as an innovative technology toward intelligent sports. Adv. Mater. 33 (17), 2004178. doi:10.1002/adma.202004178
Mishra, R. K., Mishra, P., Verma, K., Mondal, A., Chaudhary, R. G., Abolhasani, M. M., et al. (2019). Electrospinning production of nanofibrous membranes. Environ. Chem. Lett. 17 (2), 767–800. doi:10.1007/s10311-018-00838-w
Mo, J., Liu, Y., Fu, Q., Cai, C., Lu, Y., Wu, W., et al. (2022). Triboelectric nanogenerators for enhanced degradation of antibiotics via external electric field. Nano Energy 93, 106842. doi:10.1016/j.nanoen.2021.106842
Molinos-Senante, M., Hernández-Sancho, F., and Sala-Garrido, R. (2010). Economic feasibility study for wastewater treatment: a cost–benefit analysis. Sci. Total Environ. 408 (20), 4396–4402. doi:10.1016/j.scitotenv.2010.07.014
Niu, S., and Wang, Z. L. (2015). Theoretical systems of triboelectric nanogenerators. Nano Energy 14, 161–192. doi:10.1016/j.nanoen.2014.11.034
Pang, J., Wang, N., Li, X., Li, X., Wang, H., and Chen, X. (2021). Impact of economic development level and agricultural water use on agricultural production scale in China. Int. J. Environ. Res. Public Health 18, 9085. doi:10.3390/ijerph18179085
Parandeh, S., Etemadi, N., Kharaziha, M., Chen, G., Nashalian, A., Xiao, X., et al. (2021). Advances in triboelectric nanogenerators for self-powered regenerative medicine. Adv. Funct. Mater. 31 (47), 2105169. doi:10.1002/adfm.202105169
Rui, P., Zhang, W., Zhong, Y., Wei, X., Guo, Y., Shi, S., et al. (2020). High-performance cylindrical pendulum shaped triboelectric nanogenerators driven by water wave energy for full-automatic and self-powered wireless hydrological monitoring system. Nano Energy 74, 104937. doi:10.1016/j.nanoen.2020.104937
Shen, J., Zhang, F., and Siddique, K. H. M. (2018). Sustainable resource use in enhancing agricultural development in China. Engineering 4, 588–589. doi:10.1016/j.eng.2018.08.007
Tang, Y., Cai, Z., Sun, X., Chong, C., Yan, X., Li, M., et al. (2022). Electrospun nanofiber-based membranes for water treatment. Polymers 14 (10), 2004. doi:10.3390/polym14102004
Tao, Y., Xiang, H., Cao, X., and Wang, N. (2024). Spring design of triboelectric nanogenerator with MXene-modified interface for fluid energy harvesting and water level monitoring. ACS Appl. Mater. Interfaces 16 (3), 3406–3415. doi:10.1021/acsami.3c15558
Tian, M., Zhu, Y., Chen, Y., Liu, X., Yang, Y., and Gao, S. (2021). Template-assisted self-activation of mesoporous carbon with active nitrogen/oxygen configurations for sustainable triboelectric nanogenerator powered electro-Fenton degradation. Nano Energy 83, 105825. doi:10.1016/j.nanoen.2021.105825
Tinghai, C., Jiajia, S., and Zhong Lin, W. (2023). Triboelectric nanogenerators. Nat. Rev. Methods Prim. 3, 39. doi:10.1038/s43586-023-00220-3
Wang, H., Han, M., Song, Y., and Zhang, H. (2021). Design, manufacturing and applications of wearable triboelectric nanogenerators. Nano Energy 81, 105627. doi:10.1016/j.nanoen.2020.105627
Wang, J., Dai, X., Pei, X., Qi, L., Ning, F., Chen, J., et al. (2022). Ferroelectric LaxFe0.1–x codoped ZnO nanorod triboelectric nanogenerators for electrochemical rhodamine B degradation. ACS Appl. Nano Mater. 5 (9), 12756–12764. doi:10.1021/acsanm.2c02648
Wang, J., Wu, Z., Pan, L., Gao, R., Zhang, B., Yang, L., et al. (2019). Direct-current rotary-tubular triboelectric nanogenerators based on liquid-dielectrics contact for sustainable energy harvesting and chemical composition analysis. ACS Nano 13 (2), 2587–2598. doi:10.1021/acsnano.8b09642
Wang, S., Chai, Q., Sun, X., Zhang, Q., and Yu, H. (2020). Construction and application of evaluation system for integrated development of agricultural industry in China. Environ. Dev. Sustain. 23, 7469–7479. doi:10.1007/s10668-020-00926-4
Wang, S., Lin, L., and Wang, Z. L. (2015a). Triboelectric nanogenerators as self-powered active sensors. Nano Energy 11, 436–462. doi:10.1016/j.nanoen.2014.10.034
Wang, Z. L. (2014). Triboelectric nanogenerators as new energy technology and self-powered sensors – principles, problems and perspectives. Faraday Discuss. 176 (0), 447–458. doi:10.1039/C4FD00159A
Wang, Z. L. (2020). Triboelectric nanogenerator (TENG)—sparking an energy and sensor revolution. Adv. Energy Mater. 10 (17), 2000137. doi:10.1002/aenm.202000137
Wang, Z. L., Chen, J., and Lin, L. (2015b). Progress in triboelectric nanogenerators as a new energy technology and self-powered sensors. Energy and Environ. Sci. 8 (8), 2250–2282. doi:10.1039/C5EE01532D
Wu, C., Wang, A. C., Ding, W., Guo, H., and Wang, Z. L. (2019). Triboelectric nanogenerator: a foundation of the energy for the new era. Adv. Energy Mater. 9 (1), 1802906. doi:10.1002/aenm.201802906
Xuan, Z., Wang, Z. L., Wang, N., and Cao, X. (2023). Thermal-driven soft-contact triboelectric nanogenerator for energy harvesting and industrial cooling water monitoring. Small 19 (8), 2206269. doi:10.1002/smll.202206269
Yu, J., and Wu, J. (2018). The sustainability of agricultural development in China: the agriculture–environment nexus. Sustainability 10, 1776. doi:10.3390/su10061776
Yu, X., Han, X., Zhao, Z., Zhang, J., Guo, W., Pan, C., et al. (2015). Hierarchical TiO2 nanowire/graphite fiber photoelectrocatalysis setup powered by a wind-driven nanogenerator: a highly efficient photoelectrocatalytic device entirely based on renewable energy. Nano Energy 11, 19–27. doi:10.1016/j.nanoen.2014.09.024
Zhang, C., Wu, D., and Ren, H. (2020). Bioremediation of oil contaminated soil using agricultural wastes via microbial consortium. Sci. Rep. 10, 9188. doi:10.1038/s41598-020-66169-5
Zhang, X., Yu, M., Ma, Z., Ouyang, H., Zou, Y., Zhang, S. L., et al. (2019). Self-powered distributed water level sensors based on liquid–solid triboelectric nanogenerators for ship draft detecting. Adv. Funct. Mater. 29 (41), 1900327. doi:10.1002/adfm.201900327
Zhao, Z., Zhang, Z., Xu, L., Gao, F., Zhao, B., Kang, Z., et al. (2020). Tumbler-shaped hybrid triboelectric nanogenerators for amphibious self-powered environmental monitoring. Nano Energy 76, 104960. doi:10.1016/j.nanoen.2020.104960
Zhou, L., Liu, D., Li, S., Yin, X., Zhang, C., Li, X., et al. (2019). Effective removing of hexavalent chromium from wasted water by triboelectric nanogenerator driven self-powered electrochemical system – why pulsed DC is better than continuous DC? Nano Energy 64, 103915. doi:10.1016/j.nanoen.2019.103915
Zhou, L., Liu, D., Wang, J., and Wang, Z. L. (2020). Triboelectric nanogenerators: fundamental physics and potential applications. Friction 8 (3), 481–506. doi:10.1007/s40544-020-0390-3
Zhu, G., Peng, B., Chen, J., Jing, Q., and Lin Wang, Z. (2015). Triboelectric nanogenerators as a new energy technology: from fundamentals, devices, to applications. Nano Energy 14, 126–138. doi:10.1016/j.nanoen.2014.11.050
Zhu, Y., Chen, C., Tian, M., Chen, Y., Yang, Y., and Gao, S. (2021). Self-powered electro-Fenton degradation system using oxygen-containing functional groups-rich biomass-derived carbon catalyst driven by 3D printed flexible triboelectric nanogenerator. Nano Energy 83, 105720. doi:10.1016/j.nanoen.2020.105720
Zi, Y., Niu, S., Wang, J., Wen, Z., Tang, W., and Wang, Z. L. (2015). Standards and figure-of-merits for quantifying the performance of triboelectric nanogenerators. Nat. Commun. 6 (1), 8376. doi:10.1038/ncomms9376
Keywords: self-powered system, triboelectric nanogenerator, agricultural activities, environmental monitoring, wastewater treatment, environmental protection
Citation: Qu W, Zhong C, Luan P, Shi W, Geng L, Shi G and Chen R (2024) Self-powered system based on triboelectric nanogenerator in agricultural groundwater pollution monitoring and control. Front. Energy Res. 12:1324866. doi: 10.3389/fenrg.2024.1324866
Received: 20 October 2023; Accepted: 22 March 2024;
Published: 05 April 2024.
Edited by:
Awadesh Kumar Mallik, Nanyang Technological University, SingaporeReviewed by:
Pradip Thakur, Netaji Nagar College for Women, IndiaCopyright © 2024 Qu, Zhong, Luan, Shi, Geng, Shi and Chen. This is an open-access article distributed under the terms of the Creative Commons Attribution License (CC BY). The use, distribution or reproduction in other forums is permitted, provided the original author(s) and the copyright owner(s) are credited and that the original publication in this journal is cited, in accordance with accepted academic practice. No use, distribution or reproduction is permitted which does not comply with these terms.
*Correspondence: Gaofei Shi, Z2ZzaGkxMjNAMTI2LmNvbQ==
Disclaimer: All claims expressed in this article are solely those of the authors and do not necessarily represent those of their affiliated organizations, or those of the publisher, the editors and the reviewers. Any product that may be evaluated in this article or claim that may be made by its manufacturer is not guaranteed or endorsed by the publisher.
Research integrity at Frontiers
Learn more about the work of our research integrity team to safeguard the quality of each article we publish.