- 1Bohai Oilfield Research Institute, Tianjin Branch, CNOOC China Limited, Tianjin, China
- 2School of Earth Resources, China University of Geosciences, Wuhan, China
The Bozhong 19–6 Condensate gas field is the first giant gas field discovered in the Bohai Bay Basin in recent years. The gas field reservoir has diverse reservoir space and strong heterogeneity.In this paper, we systematically summarize tectonic fractures development patterns and explore the main factors controlling fractures development and clarify the influence of fractures on reservoir quality through core and microscopic thin section observations, physical property data, imaging logging data. The results show that the major types of tectonic fractures in study area are shear fractures, followed by tensile fractures. Study area mainly developed high-angle fractures and completely filled fractures, fractures are commonly filled with carbonate and clay cement. Four groups of tectonic fractures were found in the study area, which corresponds to the four fracture formation period. Tectonic movement controls the fractures development in study area, the multi-stage tectonic movement had caused a complex fractures network system. The faults control the development of associated fractures formation in the fractures zone, different faults control the fractures formation and orientations in the vicinity. Rock type and minrals content is the basis of forming different fractures, such as the high felsic content is the basis of developed fractures in metamorphic rocks, and rock layer thickness, reservoir porosity and permeability are important factors of the fractures development heterogeneity longitudinally.
1 Introduction
In recent years, a large condensate gas field has been found in the deep Archean metamorphic buried hill in the Bozhong 19–6 structural belt, southwestern Bozhong sag, which is the largest gas field discovered so far in the Bohai sea basin. (Wang et al., 2019; Ye et al., 2021; Wang et al., 2022). The permeability of such reservoirs is significantly improved by the tectonic fractures and their associated secondary pores (Lyu et al., 2019), it provides a effective pathways for oil and gas migration and reservoir space for oil and gas accumulation (Cui et al., 2013). In addition, tectonic fractures connect “geological sweet spots” and “engineering sweet spots” together (Wang et al., 2017; Gong et al., 2021), therefore, the study of tectonic fractures in metamorphic buried hill reservoirs should run through the whole exploration and development process.
In recent years, many scholars have studied the controlling factors of fracture development in metamorphic rock buried hill reservoirs. It is believed that tectonic movement, lithology, weathering intensity and thickness of layers are the main controlling factors of fractures development within the metamorphic rock buried hill reservoirs (Bazalgette et al., 2010; Eig and Bergh, 2011; Zeng et al., 2013; Wang et al., 2016; Yin et al., 2019; Li et al., 2020; Huang et al., 2022; Liu et al., 2023a; Liu et al., 2023b). The tectonic movement affects the structure fractures development indirectly by affecting the tectonic stress field distribution, which is the most important external factor controlling the development of reservoir fractures (Ezati et al., 2018; Maerten et al., 2018). Affected by the difference of fault spatial activity, the local stress distribution near the fault is obviously different, which affects the development mode of fracture near the fault (Aydin, 2000). In fault-related folds, faults and turning ends are the main controlling factors of fracture development. The fractures at the turning end of the fold are often more developed than those at the wing of the fold. The density of tectonic fractures decreases exponentially with the distance increasing from the fault (Ding et al., 2013; Lavenu et al., 2013). Under the same tectonic stress, rocks with smaller particles and rich in brittle minerals such as felsic are more likely to break and develop fractures (Ye et al., 2021). Weathering and eluviation can cause irregular fractures of different scales near the rocks surface, high rupture degree and well-development fractures were developed (Salah and Alsharhan, 1998; Liu et al., 2016; Yin et al., 2016). The stress concentration degree of fracture tip in different rock stratum thickness is different. Generally, the stress of thinner rock stratum is more concentrated at the fracture tip, and the development degree of the fracture is higher. However, when the thickness of the rock stratum reaches a certain critical value, the rock stratum thickness does not affect the fracture density, and the fracture density tends to be constant (Lianbo and Xiang-Yang, 2009; Fan et al., 2021).
Many scholars have studied the tectonic fractures of metamorphic buried hill reservoirs in Bozhong 19–6 gas field, Bohai Bay Basin (Yu and Koyi, 2016; Ye, Chen, et al., 2020; Wang et al., 2021; Zeng et al., 2022). However, the main research is the important role of fractures in the process of oil and gas migration and accumulation, there is still a lack of analysis and research on the comprehensive characterization of reservoir tectonic fractures and the influencing factors of reservoir fracture development in the area. Therefore, the observation and statistics of imaging logging, core and thin section tectonic fractures are carried out to quantitatively characterize the tectonic fractures of metamorphic buried hill reservoirs in Bozhong 19–6 gas field, combining with the whole rock mineral analysis, core porosity and permeability analysis, and clarify the control factors of structural fracture development in the study area. These results provide a guidance for the prediction of tectonic fractures in the study area, and provide reliable basic data for the further exploration and development of the Bozhong 19–6 gas field.
2 Geological setting
Bozhong 19–6 condensate gas field is located in the southwest of Bozhong sag, surrounded by Bozhong sag, Shanan sag and Huanghekou sag (Zhao et al., 2015a; Xu et al., 2019) (Figure 1A). From the top to the bottom, the stratum is Pingyuan Formation, Minghuazhen Formation, Guantao Formation, Dongying Formation, Shahejie Formation, Kongdian Formation, local Mesozoic stratum and Archean metamorphic rock basement (Xu et al., 2019; Feng et al., 2020) (Figure 1B). Mesozoic rock reservoirs are mainly developed in the Minghuazhen Formation, Guantao Formation and Kongdian Formation, the lithology is mainly glutenite and tuff (Li et al., 2012).
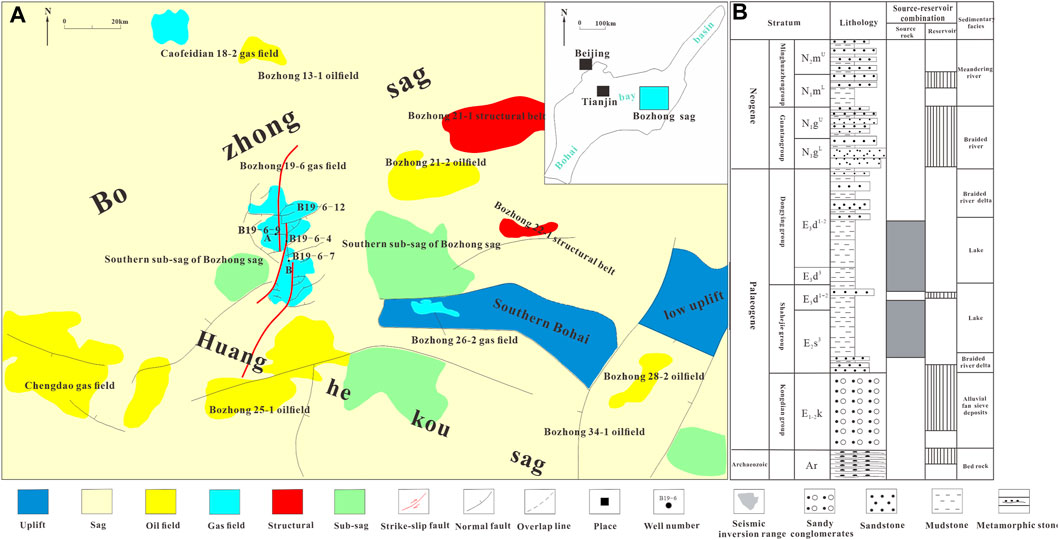
FIGURE 1. (A) Regional location of the BZ19-6 condensate gas field; (B) Comprehensive stratigraphic column of the BZ19–6 condensate gas field.
Archean is the main gas-bearing interval of Bozhong 19–6 condensate gas field, and it is buried more than 3,800 m (Wang et al., 2021). Its lithology is metamorphic granite, mainly including monzonitic gneiss, plagioclase gneiss, locally developed cataclastic mixed granite (Liu et al., 2023b). The rock components are mainly albite, quartz and potassium feldspar, followed by chlorite, calcite, illite, muscovite and dolomite (Ye et al., 2022). The core physical property test shows that the average porosity of Archean metamorphic rock buried hill reservoir is 5.22%, and the average permeability is 0.37 mD (Pei et al., 2022). The reservoir space is mainly intergranular pore and dissolution pore (Cao et al., 2015; Wang et al., 2018).
The Bozhong 19–6 condensate gas field has experienced multi-stage tectonic activities since Indosinian (Liu et al., 2022; Yi et al., 2022). During the Indosinian period, the South China plate subducted and collided with the North China plate, forming a regional compressive stress field from south to north. A series of south to north thrust faults were formed, and the fractures were mainly distributed in the near EW direction (Zhou et al., 2012). During the Yanshanian period, the study area was affected by the NW compressive stress, due to the NW subduction of the ancient Pacific Ocean. At the same time, the Tanlu Fault was left-laterally squeezed, forming a large number of NE strike-slip faults. The large-scale faults of the Indosinian period were reversed during the formation of the buried hill, and the original continuous Indosinian uplift zone was cut into a series of fragments of different sizes. At the same time, the internal part of the buried hill block was broken (Chen, 1998; Huang et al., 2003). The early formation of fractures in the study area was subjected to the north-northwest tensile stress of the Himalayanian period, while it was subjected to the superimposed modification of the right-lateral tensile action of the Tanlu Fault. The early NNW and N-E faults were reactive, and the overall fracture subsided. Finally the tectonic appearance of the buried hill was shaped (Zhao et al., 2015a).
3 Data and methods
The data and samples of this study are derived from the metamorphic buried hill reservoir of Bozhong 19–6 condensate gas field. Nine wells (BZ19-6-A, BZ19-6-B, BZ19-6-C, BZ19-6-D, BZ19-6-E, BZ19-6-F, BZ19-6-G, BZ19-6-H and BZ19-6-I) with a total of 73.6 m cores and 288 wall cores were observed. The lithology and fracture types have been identified, and the development degree and filling degree of fractures also have been identified. A total of 359 casting sheets were collected to observe the development degree and filling degree of microfractures and dissolution along microfractures, A total of 112 samples of reservoir properties test results were collected, including porosity and permeability, and 25 samples of whole rock mineral analysis results, and the mineral content composition of different lithology was obtained.
Logging data, including conventional and imaging logging data from 11 wells, were obtained to interpret the fracture characteristics, including orientation, dip angle, scale, and fracture linear density (Liu et al., 2021). We define the total fractures number divided by the total length or the total fractures number per unit core length as fracture linear density (Ortega et al., 2006). The above data and samples were provided by CNOOC China Limited, Tianjin Branch.
4 Results
4.1 Fracture development characteristics
The tectonic fractures of metamorphic buried hill reservoir in Bozhong 19–6 condensate gas field are mainly shear fractures. High angle fractures (
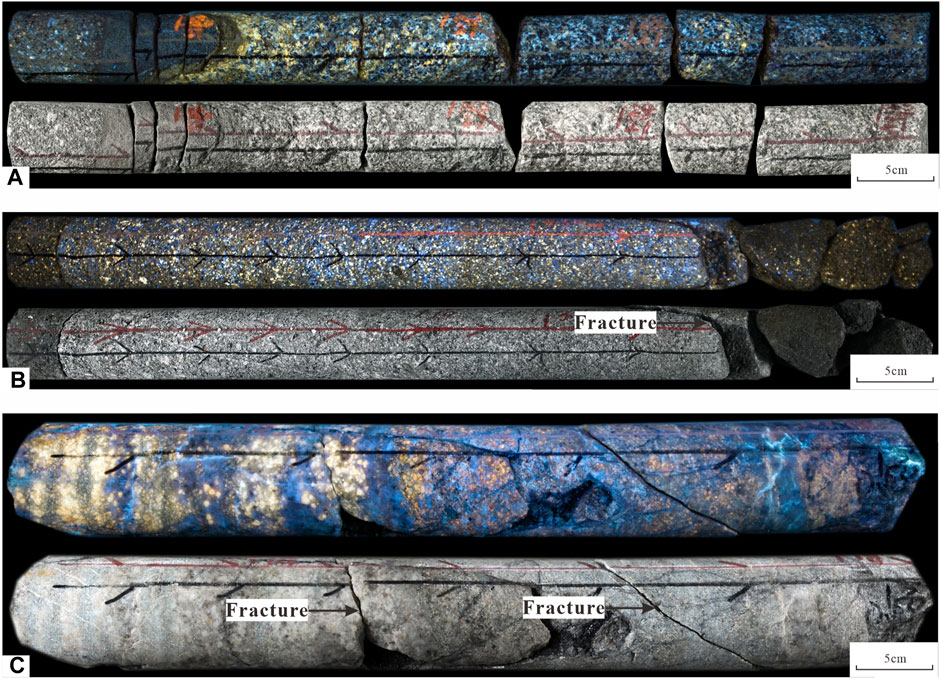
FIGURE 2. (A) Well BZ19-6-C, 4538.05–4538.90 m, exhibits numerous shear and tensile fractures with significant variations in orientations. The distribution forms a mesh-like intersection pattern, with many fractures being partly or completely filled. (B) Well BZ19-6-I, 4429.00–4429.90 m, displays a high-angle shear fracture and a weathered and dissolved fractured zone in the lower part. (C) Well BZ19-6-C, 4678.06–4678.59 m, shows multiple shear and tensile fractures. The filling minerals within the fractures have undergone dissolution.
The microfractures of metamorphic rock buried hill reservoir in Bozhong 19–6 condensate gas field are developed. It can be observed that the early fractures are cut by the later fractures, and the later fractures development is restricted by the early fractures (Figure 3A). Shear fractures are mainly developed, and the fracture surface is relatively straight. The fracture aperture is between 2 and 7 mm and the fracture length is between 1 and 10 mm. The fracture filling degree is high, and the fractures are mainly filled with clay and carbonate minerals (Figure 3B). Fractures mostly cut through quartz, feldspar and other mineral particles, and are accompanied by dissolution, pores enlarged by dissolution are developed along microfractures (Figure 3C). In addition, the network fracture system intersected by fractures can also be observed (Figure 3D), it is significant for improving the storage and permeability properties of metamorphic rock reservoirs.
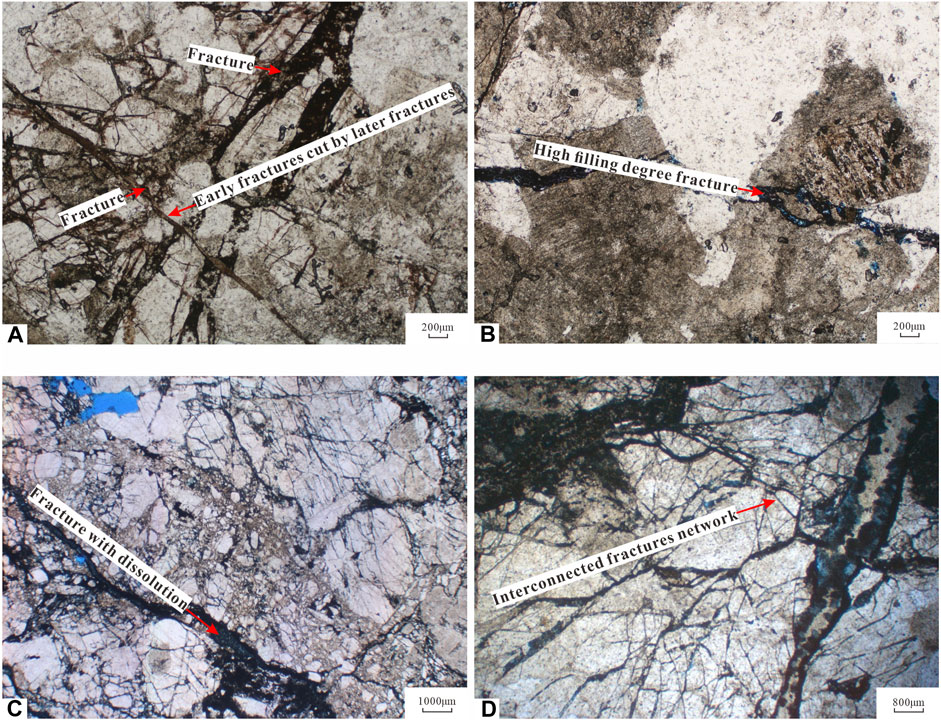
FIGURE 3. (A) Well BZ19-6-C, 4251.1 m, monzonal gneiss, single polarized light, exhibits two phases of fractures, with distinct early fractures being intersected by later fractures; (B) Well BZ19-6-K, 4343.5 m, plagioc gneiss, single polarized light, shows fractures filled with mud, siderite, and ankerite; (C) Well BZ19-6-H, 4349.0 m, broken porphyry, single polarized light, features dissolved pores at the edges of fractures; (D) Well BZ19-6-J, 4068.0 m, monzonal gneiss, single polarized light, displays an intersecting network of fractures forming a mesh-like fracture system.
Interpreting the fracture characteristics from 11 imaging logging, including orientation, dip angle, scale, and fracture density, there are three kinds of combination patterns of tectonic fractures in the study area. It mainly includes parallel patterns fractures (Figure 4A), which refers to two or more fractures with similar orientations; network fractures (Figure 4B), multiple fractures are intertwined, and the orientations are irregular; conjugation fractures (Figure 4C). Two fractures have similar dip angles but opposite trends. High angle fractures (
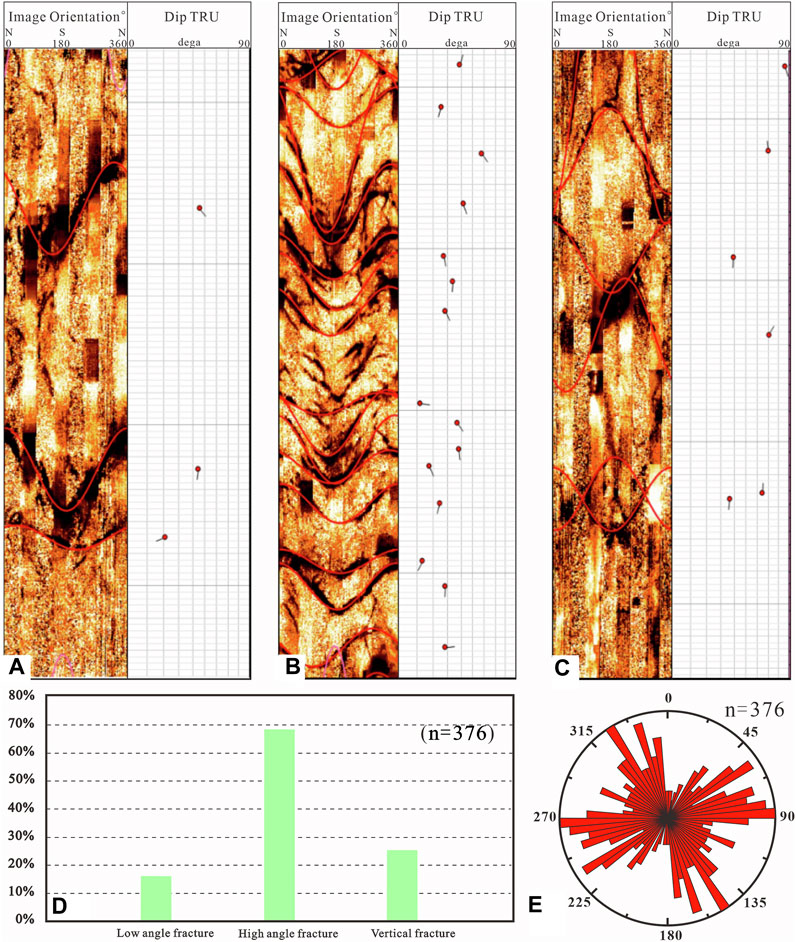
FIGURE 4. (A) Well BZ19-6-D, 4615.8–4617.9 m, exhibits grouped fissures; (B) Well BZ19-6-D, 4602.1–4604.5 m, features a network of fissures; (C) Well BZ19-6-D, 4607.2–4609.6 m, shows widened fissures due to dissolution along fractures; (D) Histogram of the tectonic fractures dip angle spread of metamorphic reservoir; (E) Rose diagram depicting the structural fracture orientations in the metamorphic reservoir.
The fracture filling degree of metamorphic buried hill reservoir in Bozhong 19–6 condensate gas field is high, it is mainly completely filled fractures, accounting for 89.13%, followed by partly filled fractures, accounting for 10.51%, open fractures only accounts for 0.36% (Figure 5A). The fractures are mainly filled with caly, followed by carbonate fillings, quartz fillings and asphaltene account for a small proportion of fractures (Figure 5B). The filling degree of low angle fractures is higher, and the filling degree of high angle fractures and vertical fractures is lower (Figure 5C). The fractures filling degree of glutenite is high, and the fractures effectiveness is low. A certain number of partly filled fractures are developed in granitic gneiss, and the fractures effectiveness is high (Figure 5D).
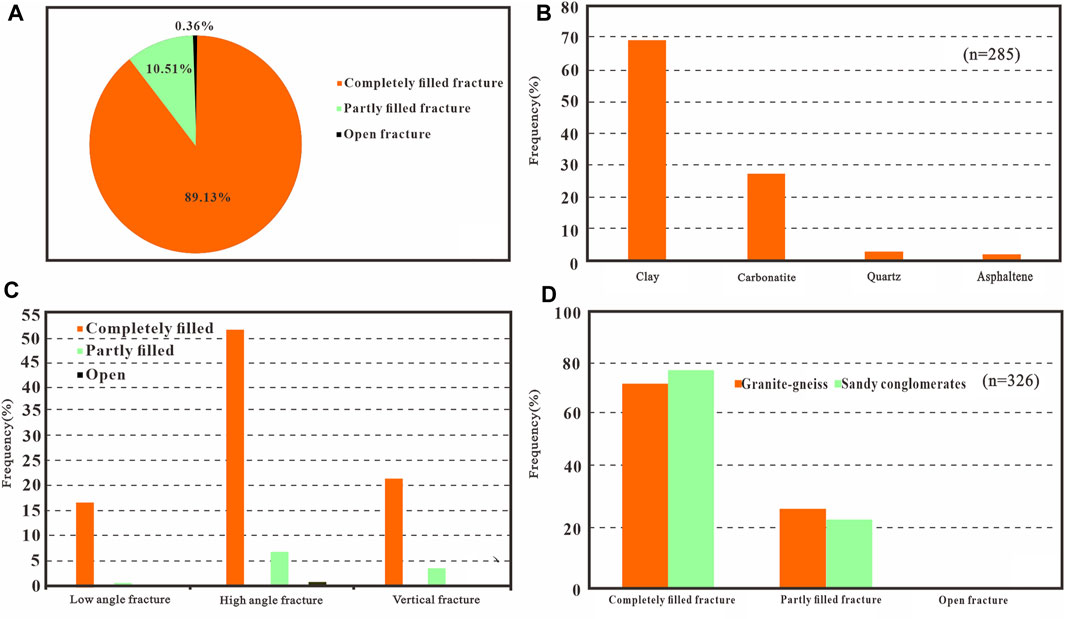
FIGURE 5. (A) Types of structural fracture filling; (B) Types of materials filling tectonic fractures; (C) Filling degree of tectonic fractures at different angles; (D) The Filling degree in tectonic fractures for different lithologies.
5 Discussion
5.1 Controlling factors of fractures in metamorphic hills
5.1.1 Lithology
The difference of rock mineral composition and rock fabric of different lithologies determines the difference of structural fracture development, which is the internal factor determining the difference of fracture development (Ding et al., 2012; Ju and Sun, 2016). There are various types of metamorphic buried hill reservoir rocks in Bozhong 19–6 condensate gas field, and the main reservior rocks are metamorphic rocks and intrusive dikes. The tectonic fractures in the study area are mainly developed in plagioclase gneiss and monzonitic gneiss, which density is high, followed by fractures density in the granite, and the fracture density in diorite porphyrite and diabase is low (Figure 6A). The content of brittle minerals in plagioclase gneiss, monzonitic gneiss and granite is more than 80%, and diorite porphyry and diabase is less than 80% (Figure 6B). The rock of lower plastic minerasl content has a better brittleness. Under the action of tectonic stress, it is easy to development fractures, and the degree of fractures development is higher (Wang et al., 2023). It is common for plagioclase gneiss, monzonitic gneiss and granite to undergo migmatization (Lander and Laubach, 2015). Recrystallization makes the rock grains become larger from small, as the mineral grains increase, the particle size becomes longer (Maréchal et al., 2004). Under the same tectonic stress, the moment exerted on the same kind of mineral increases with larger grain size. Larger mineral grains are more likely to exceed the stress-bearing strength and generate fractures (Li et al., 2017).The observation results of core and thin section show that the rock with relatively high content of brittl minerals has a high degree of composition and structural heterogeneity. The rock contains minerals of various scales, components and shapes, and the transmission speed of each grain to force and its own deformation are different, which leads to the uneven distribution of stress field in the rock. Under the action of stress, it is more likely to development fractures due to the influence of rock composition and structural heterogeneity (Li et al., 2022). Therefore, rocks with a high degree of heterogeneity are more likely to development fractures.
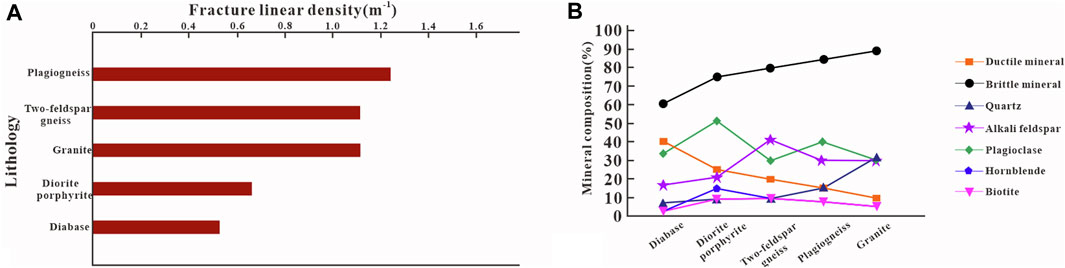
FIGURE 6. (A) Connection about density and lithology of structural fracture; (B) Distribution of mineral content in diverse lithologies.
5.1.2 Rock layers thickness
Under the action of tectonic stress, the fractures development degree of thinner rock thickness is higher (Nelson and Mold, 2000). The difference of rock layers thickness is an important factor causing the vertical heterogeneity of fractures development in the study area. The linear density of tectonic fractures decreases gradually with the increase of rock layers thickness (Figure 7A). The linear density of tectonic fractures decreases significantly when the thickness of the rock layer increases from 2 m to 15 m.When the thickness of the rock layer is greater than 15 m, the degree of fracture development is low, and the thickness of the rock layer has little effect on the linear density of the fractures.
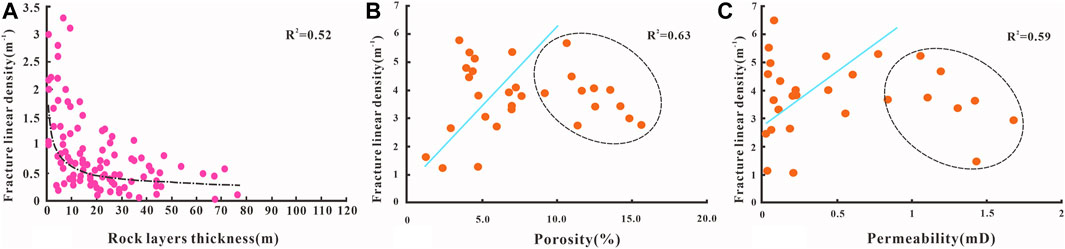
FIGURE 7. (A) Intersection diagram of structural fracture density and rock layer thickness; (B) intersection diagram of structural fracture density and reservoir porosity; (C) intersection diagram of structural fracture density and reservoir permeability.
5.1.3 Reservoir porosity and permeability
If the porosity and permeability of the reservoir are high, it shows that the rock is relatively loose, when the fracture extends and expands, it is easy to produce stress release in the matrix pores, and it is difficult to form large scale fractures. If the porosity and permeability of the reservoir are low, it shows that the rock is dense, its compressive strength and critical fracture pressure become larger, and the degree of tectonic fractures development will be lower when subjected to tectonic stress. Only when the porosity and permeability are in the suitable range, the rock compressive strength, critical fracture value and fracture extension ability can achieve the optimal combination. The relationship between fracture linear density and reservoir porosity and permeability has a two-stage relationship. When the reservoir porosity is less than 10%, the porosity is positively correlated with the development degree of tectonic fractures. When the matrix porosity is more than 10%, the porosity is negatively correlated with the development degree of tectonic fractures (Figure 7B). When the reservoir permeability is less than 1.1 mD, the permeability is positively correlated with the degree of structural fracture development. When the matrix permeability is greater than 1.1 mD, the permeability is negatively correlated with the development degree of tectonic fractures (Figure 7C).
5.1.4 Tectonic background
5.1.4.1 Tectonic movement
The thrust extrusion in the Indosinian period is the key factor for the reservoir fractures development in the study area. The metamorphic rock basement is subjected to strong compression and thrusting, forming a large number of large scale faults in the N-W oriented. Especially, the core of the extrusion is the stress concentration development area, and a large number of tectonic fractures are formed inside the buried hill (Cheng et al., 2018) (Figure 8A). At the same time, the N-E oriented thrust extrusion caused the study area to uplift sharply, forming an anticline uplift structure. The Archean metamorphic rock basement exposed the surface to weathering and leaching, and atmospheric water leached along the fault to the interior of the buried hill, forming a thick weathering crust. Due to the early formation time, these fractures experienced strong diagenesis in the later deep burial process, and most of the fractures were filled (Tong et al., 2012). During the Yanshanian period, it was mainly affected by the N-W trending low angle subduction of the Paleo Pacific Ocean. A large number of N-E oriented trending strike-slip faults were formed in the Bozhong sag, which retransformed the early fractures and further expanded the scale of fracture development (Huang et al., 2003) (Figure 8B). The influence of the Himalayan tectonic movement on the reservoir in the study area is mainly reflected in the reactivation of early fractures and the generation of new fractures. Most of the fractures formed in the Indosinian and Yanshanian periods are filled with argillaceous, iron and carbonate minerals (Wang et al., 2022). A large number of high angle fractures are formed inside or on the edge of the early filled fractures, and a small number of new fractures are generated. The Himalayan period is the main period for the formation of effective fractures in the reservoir of the study area. Although the ability of the Himalayan period to development new fractures is not as good as that of the Indosinian and Yanshanian periods, the Himalayan tectonic movement can re-activate the early fractures (Wang et al., 2018)(Figures 8C,D).
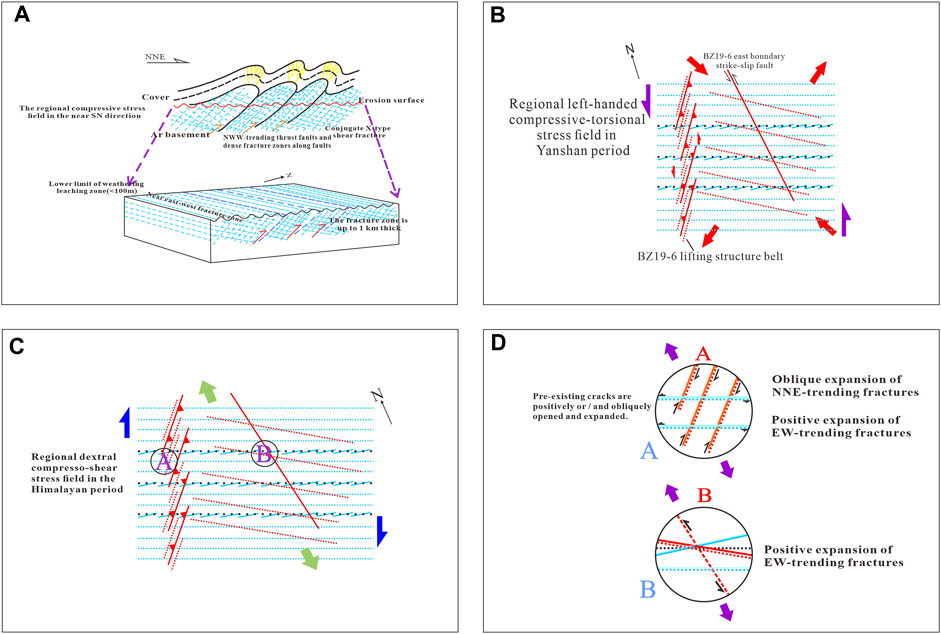
FIGURE 8. Structural fracture development patterns in different tectonic periods within BZ19-6 condensate gas field.
In general, The formation of buried hill reservoir fractures in the study area has experienced the initial formation of buried hill caused by strong extrusion in Indosinian period, the superimposed transformation and destruction of compression-torsion strike-slip in early Yanshanian period, the reformation of fault depression into mountains in middle Yanshanian period, and the multi-stage structural transformation and superimposed transformation process of reactivation of tension-torsion extension fractures in Himalayan period. The strong compressional tectonism in Indosinian period laid the reservoir foundation of buried hill, the sinistral strike slip in Yanshanian period further expanded the scale of fractures, and the dextral strike slip in Himalayan period reactivated the early fractures and formed a super large scale fractures system.
5.1.4.2 Control of faults on fractures
Studies have shown that although there are differences in the characteristics and formation mechanism of tectonic fractures in the fault zone under different mechanical properties, it is a common law that the farther the distance from the fault zone is, the less developed the tectonic fractures are (Lavenu et al., 2013). According to the rose diagram of fracture trends in 11 wells (Figure 9), it can be seen that the fractures orientation is parallel or nearly parallel to the orientation of adjacent faults, which indicates that faults play an important role in the development of tectonic fractures. In addition, near the fault or at the inflection point and intersection of the fault zone, the stress is concentrated, and the tectonic fractures are developed. BZ19-6-I, BZ19-6-J, and BZ19-6-K are located at the intersection of multiple different sequence faults, and the fracture density is high. In addition, the cataclastic zone in these three wells is extremely developed and has a certain direction, which is the result of the combined action of shear stress and tensile stress.
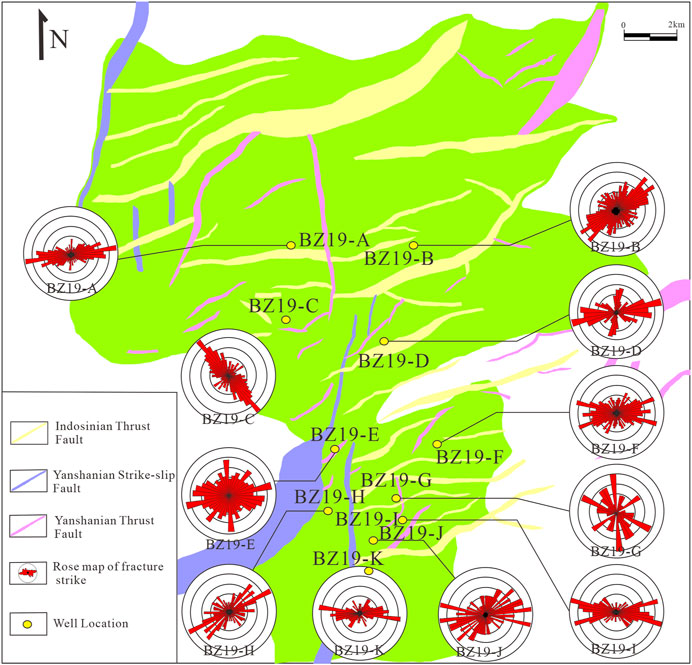
FIGURE 9. Major larger fault distribution map, modified from (Dai et al., 2021), the fracture orientations are obtained from the data of 11 wells.
5.2 Contributions of fractures to reservoir quality
Previous studies have shown that weathering crust, as well as pore-fractures type reservoirs are the major types of the Paleozoic reservoirs in BZ19-6 condensate gas field (Luo et al., 2005). The study shows that the reservoir space of metamorphic buried hill reservoir in Bozhong 19–6 condensate gas field mainly includes intergranular pores, intragranular dissolution pores, fractures of cataclastic grains in sandy conglomerate and metamorphic buried hill, and dissolution expansion pores developed along microfractures (Figure 10). The study area has experienced multi-tectonic movement, and a large number of fractures are developed under the tectonic stress. The fractures are cut and connected with each other, which is an important reservoir space in the study area. In addition, these fractures effectively communicate intergranular pores and intragranular dissolved pores, which play a role in the formation of effective reservoirs. Based on the analysis of 58 samples of reservoir physical property test results collected, it is considered that there are obvious differences in permeability between samples from the same lithology, and the difference in permeability depends on whether the sample has microscopic effective fractures. The measured permeability of core samples without effective fractures is concentrated in 0.01–0.1 mD, while the measured permeability of core samples with effective fractures is 1–10 mD. Therefore, it is considered that the development of fractures increases the reservoir permeability by 1-2 orders of magnitude (Figure 11). This difference in permeability reflects that fractures are the key to improving the seepage capacity of deep reservoirs.
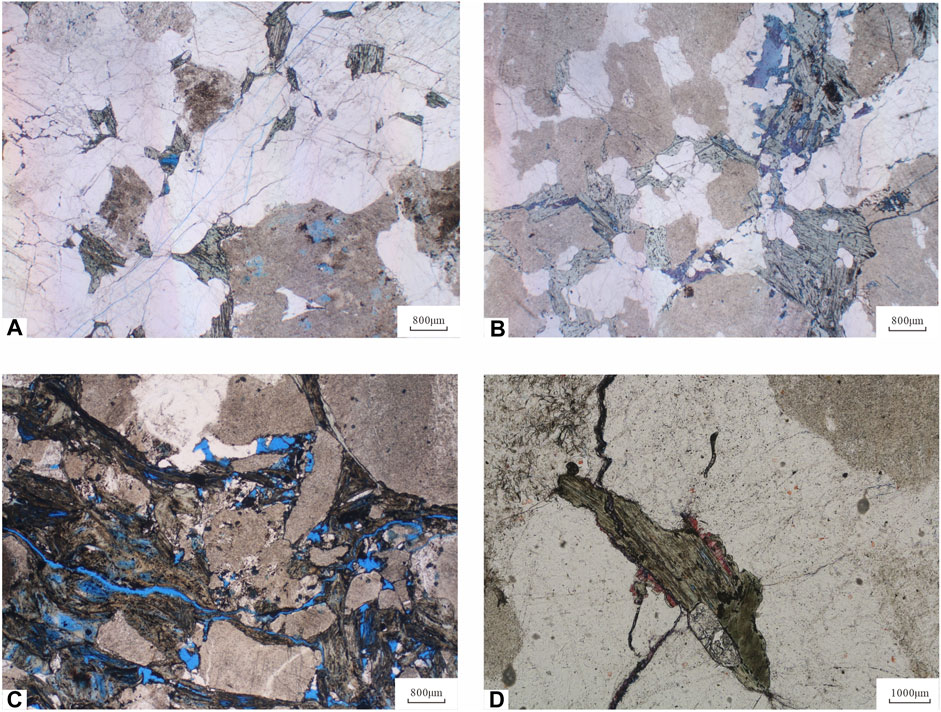
FIGURE 10. (A) Well BZ19-6-E, 4564.0 m, granular rock, single polarized light, dissolution intergranular pores and dissolution intragranular pores; (B) Well BZ19-6-E, 4630.0 m, plagioc gneiss, single polarized light, intragranular dissolution pores; (C) Well BZ19-6-E, 4515.0 m, plagioc gneiss, single polarized light, intergranular pores, intracrystalline dissolution pores, and unfilled fractures; (D) Well BZ19-6-C, 5190.0 m, plagioc gneiss, single polarized light, biotite intracrystalline dissolution pores and feldspar dissolution pores along cleavage.
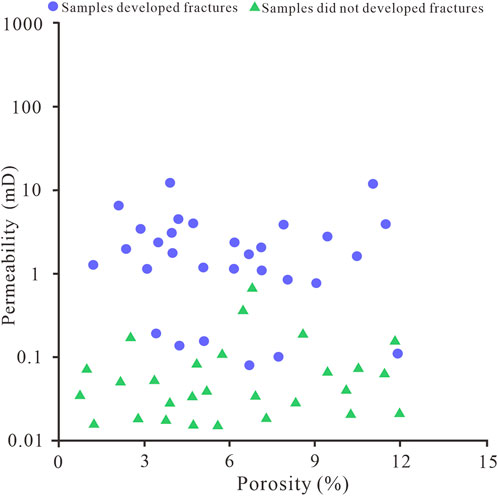
FIGURE 11. Relationship of permeability and porosity in metamorphic rock reservoirs. Analyzed by Physical Property Testing (N = 58).
In general, metamorphic rocks must undergo fracture and dissolution before they can become favorable reservoirs (Guo et al., 2016). For dense metamorphic rocks, the depth of weathering and leaching during the exposure period of buried hills is limited, and it is difficult to effectively transform them. Therefore, in order to form an effective reservoir in the inner part of the buried hill, it is first necessary to form fractures by strong tectonic movement. Fractures can become a channel for weathering and leaching to expand to the bedrock, thus forming dissolution fractures (Chai and Yin, 2021). These results imply that the development degree of fractures determines the distribution of favourable reservoirs.
6 Conclusion
The tectonic fractures of metamorphic rock buried hill reservoir in Bozhong 19–6 condensate gas field mainly develop shear fractures, and the development of tensile fractures is less. The fracture filling degree is high, and the filling materials mainly include clay, carbonate, silicate and other minerals. The dip angle of fractures is mainly high angle fractures and vertical fractures. The strike of tectonic fractures is scattered. There are four groups of tectonic fractures in EW, NE-SW, NW-SE and NNW-SSE oriented.
Lithology is the internal factor that determines the difference of fracture development. In rocks with relatively high content of light minerals, the degree of fracture development increases with the increase of composition and structural heterogeneity. Under the same stress condition, the thinner the rock thickness is, the more developed the fractures are. The vertical difference of single rock thickness is an important factor to cause the vertical heterogeneity of fracture development degree in metamorphic rock reservoir. When the porosity and permeability of the reservoir are in a suitable combination, the mechanical properties of the rock and the extension ability of the fractures can achieve the optimal combination and promote the development of the fractures. Indosinian periods and Yanshanian periods are the main periods of fractures formation. The early tectonic fractures were reactivated in Himalayan epoch, and the multi-tectonic movements caused the complex fractures system.
Fractures in metamorphic rock reservoirs connect dispersed pores and improve the effectiveness of reservoirs. The existence of fractures increases the permeability of metamorphic rock reservoirs by 1–2 orders of magnitude, which is the key to improving the seepage capacity of metamorphic rock reservoirs. It provides effective space for the accumulation and migration of oil and gas, and plays an important role in the formation of metamorphic rock reservoirs.
Data availability statement
The raw data supporting the conclusion of this article will be made available by the authors, without undue reservation.
Author contributions
ZL: Methodology, Writing–review and editing, Funding acquisition. QC: Funding acquisition, Writing–review and editing. XL: Data curation, Funding acquisition, Resources, Writing–review and editing. LZ: Writing–review and editing. WL: Project administration, Supervision, Writing–review and editing. GZ: Writing–original draft, Writing–review and editing.
Funding
The author(s) declare financial support was received for the research, authorship, and/or publication of this article. The major science and technology project of CNOOC (China) Co., Ltd. High-efficiency development technology of complex oil and gas reservoirs in the middle and deep layers of Bohai Sea (CNOOC-KJ 135 ZDXM 36 TJ 03 TJ-GD 2020-01); Tianjin branch of CNOOC (China) Co., Ltd. Unveiled the project Research on the main controlling factors of fracture development and favorable reservoir distribution of different types of buried hills in Bohai (ZZKY-2022-TJ-JG-02).
Conflict of interest
Authors ZL, QC, XL, LZ, and WL were employed by the CNOOC China Limited.
The remaining author declares that the research was conducted in the absence of any commercial or financial relationships that could be construed as a potential conflict of interest.
Publisher’s note
All claims expressed in this article are solely those of the authors and do not necessarily represent those of their affiliated organizations, or those of the publisher, the editors and the reviewers. Any product that may be evaluated in this article, or claim that may be made by its manufacturer, is not guaranteed or endorsed by the publisher.
References
Aydin, A. (2000). Fractures, faults, and hydrocarbon entrapment, migration and flow. Mar. Petroleum Geol. 17 (7), 797–814. doi:10.1016/S0264-8172(00)00020-9
Bazalgette, L., Petit, J.-P., Amrhar, M., and Ouanaïmi, H. (2010). Aspects and origins of fractured dip-domain boundaries in folded carbonate rocks. J. Struct. Geol. 32 (4), 523–536. doi:10.1016/j.jsg.2010.03.002
Cao, X., Li, S., Xu, L., Guo, L., Liu, L., Zhao, S., et al. (2015). Mesozoic–Cenozoic evolution and mechanism of tectonic geomorphology in the central North China Block: constraint from apatite fission track thermochronology. J. Asian Earth Sci. 114, 41–53. doi:10.1016/j.jseaes.2015.03.041
Chai, Y., and Yin, S. (2021). 3D displacement discontinuity analysis of in-situ stress perturbation near a weak faul. Adv. Geo-Energy Res. 5 (3), 286–296. doi:10.46690/ager.2021.03.05
Chen, A. (1998). Geometric and kinematic evolution of basement-cored structures: intraplate orogenesis within the Yanshan Orogen, northern China. Tectonophysics 292 (1–2), 17–42. doi:10.1016/S0040-1951(98)00062-6
Cheng, Y., Wu, Z., Lu, S., Li, X., Lin, C., Huang, Z., et al. (2018). Mesozoic to cenozoic tectonic transition process in zhanhua sag, Bohai Bay Basin, east China. Tectonophysics 730, 11–28. doi:10.1016/j.tecto.2018.02.010
Cui, J., Zhang, J., and Zhang, H. (2013). Features of the carboniferous volcanic rocks fracture reservoirs in Hongshanzui oilfield, Junggar Basin. J. Earth Sci. 24 (6), 997–1007. doi:10.1007/s12583-013-0397-z
Dai, X., Tang, H., Li, H., Zhang, Y., Xu, P., Wang, P., et al. (2021). Characteristics and formation mechanism of the fractures in Archaean buried hill: a case study in the BZ19-6 Block, Bohai Bay Basin, China. Geol. J. 56 (4), 2240–2257. doi:10.1002/gj.4052
Ding, W., Li, C., Li, C., Xu, C., Jiu, K., Zeng, W., et al. (2012). Fracture development in shale and its relationship to gas accumulation. Geosci. Front. 3 (1), 97–105. doi:10.1016/j.gsf.2011.10.001
Ding, W., Zhu, D., Cai, J., Gong, M., and Chen, F. (2013). Analysis of the developmental characteristics and major regulating factors of fractures in marine–continental transitional shale-gas reservoirs: a case study of the Carboniferous–Permian strata in the southeastern Ordos Basin, central China. Mar. Petroleum Geol. 45, 121–133. doi:10.1016/j.marpetgeo.2013.04.022
Eig, K., and Bergh, S. G. (2011). Late Cretaceous–Cenozoic fracturing in Lofoten, North Norway: tectonic significance, fracture mechanisms and controlling factors. Tectonophysics 499 (1–4), 190–205. doi:10.1016/j.tecto.2010.12.002
Ezati, M., Azizzadeh, M., Riahi, M. A., Fattahpour, V., and Honarmand, J. (2018). Characterization of micro-fractures in carbonate Sarvak reservoir, using petrophysical and geological data, SW Iran. J. Petroleum Sci. Eng. 170, 675–695. doi:10.1016/j.petrol.2018.06.058
Fan, C., Xu, C., Li, C., Liu, A., Li, H., Hou, J., et al. (2021). Identification and prediction of allo-source overpressure caused by vertical transfer: example from an HTHP gas reservoir in the ledong slope in the yinggehai basin. Geofluids 2021, 1–20. doi:10.1155/2021/6657539
Feng, W., Wang, F., Jiang, T., Zhao, X., Guan, J., Ma, X., et al. (2020). Origin and accumulation of petroleum in deep precambrian reservoir in baxian sag, Bohai Bay Basin, China. Mar. Petroleum Geol. 120, 104541. doi:10.1016/j.marpetgeo.2020.104541
Gong, L., Gao, S., Liu, B., Yang, J., Fu, X., Xiao, F., et al. (2021). Quantitative prediction of natural fractures in shale oil reservoirs. Geofluids 2021, 1–15. doi:10.1155/2021/5571855
Guo, P., Yao, L., and Ren, D. (2016). Simulation of three-dimensional tectonic stress fields and quantitative prediction of tectonic fracture within the Damintun Depression, Liaohe Basin, northeast China. J. Struct. Geol. 86, 211–223. doi:10.1016/j.jsg.2016.03.007
Huang, C. H., Zhu, H. Y., Wang, J. D., Han, J., Zhou, G. Q., and &Tang, X. H. (2022). AFEM-DFN model for the interaction and propagation of multi-cluster fractures during variable fluid-viscosity injection in layered shale oil reservoir. Petroleum Sci. 19 (6), 2796–2809. doi:10.1016/j.petsci.2022.06.007
Huang, S., Li, L., Vleugels, J., Wang, P., and Van Der Biest, O. (2003). Thermodynamic prediction of the nonstoichiometric phase Zr1–zCezO2–x in the ZrO2–CeO1.5–CeO2 system. J. Eur. Ceram. Soc. 23 (1), 99–106. doi:10.1016/S0955-2219(02)00073-0
Ju, W., and Sun, W. (2016). Tectonic fractures in the lower cretaceous xiagou formation of qingxi oilfield, jiuxi basin, NW China part one: characteristics and controlling factors. J. Petroleum Sci. Eng. 146, 617–625. doi:10.1016/j.petrol.2016.07.042
Lander, R. H., and Laubach, S. E. (2015). Insights into rates of fracture growth and sealing from a model for quartz cementation in fractured sandstones. Geol. Soc. Am. Bull. 127 (3–4), 516–538. doi:10.1130/B31092.1
Lavenu, A. P. C., Lamarche, J., Gallois, A., and Gauthier, B. D. M. (2013). Tectonic versus diagenetic origin of fractures in a naturally fractured carbonate reservoir analog (Nerthe anticline, southeastern France). AAPG Bull. 97 (12), 2207–2232. doi:10.1306/04041312225
Li, A., Ding, W., Wang, R., He, J., Wang, X., Sun, Y., et al. (2017). Petrophysical characterization of shale reservoir based on nuclear magnetic resonance (NMR) experiment: a case study of Lower Cambrian Qiongzhusi Formation in eastern Yunnan Province, South China. J. Nat. Gas Sci. Eng. 37, 29–38. doi:10.1016/j.jngse.2016.11.034
Li, H., Qin, Q., Zhang, B., Ge, X., Hu, X., Fan, C., et al. (2020). Tectonic fracture Formation and distribution in ultradeep marine carbonate gas reservoirs: a case study of the maokou formation in the jiulongshan gas field, sichuan basin, southwest China. Energy and Fuels 34 (11), 14132–14146. doi:10.1021/acs.energyfuels.0c03327
Li, H., Yu, F., Wang, M., Wang, Y., and Liu, Y. (2022). Quantitative prediction of structural fractures in the Paleocene lower Wenchang formation reservoir of the Lufeng Depression. Adv. Geo-Energy Res. 6 (5), 375–387. doi:10.46690/ager.2022.05.03
Li, S., Zhao, G., Dai, L., Zhou, L., Liu, X., Suo, Y., et al. (2012). Cenozoic faulting of the Bohai Bay Basin and its bearing on the destruction of the eastern north China craton. J. Asian Earth Sci. 47, 80–93. doi:10.1016/j.jseaes.2011.06.011
Lianbo, Z., and Xiang-Yang, L. (2009). Fractures in sandstone reservoirs with ultra-low permeability: a case study of the upper triassic yanchang formation in the ordos basin, China. AAPG Bull. 93 (4), 461–477. doi:10.1306/09240808047
Liu, C., Xie, Q., Wang, G., Zhang, C., Wang, L., and Qi, K. (2016). Reservoir properties and controlling factors of contact metamorphic zones of the diabase in the northern slope of the Gaoyou Sag, Subei Basin, eastern China. J. Nat. Gas Sci. Eng. 35, 392–411. doi:10.1016/j.jngse.2016.08.070
Liu, J., Ding, W., Yang, H., Dai, P., Wu, Z., and Zhang, G. (2023b). Natural fracturesand rock mechanical stratigraphy evaluationin huaqing area, OrdosBasin: a quantitative analysis basedon numerical simulation. EarthScience 48 (7), 2572–2588. doi:10.3799/dqkx.2022.234
Liu, J., Ding, W., Yang, H., and Liu, Y. (2021). Quantitative multiparameter prediction of fractured tight sandstone reservoirs: a case study of the yanchang formation of the ordos basin, Central China. SPE J. 26 (05), 3342–3373. doi:10.2118/205495-PA
Liu, J., Mei, L., Ding, W., Xu, K., Yang, H., and Liu, Y. (2023a). Asymmetric propagation mechanism of hydraulic fracture networks in continental reservoirs. GSA Bull. 135 (3–4), 678–688. doi:10.1130/B36358.1
Liu, J., Yang, H., Xu, K., Wang, Z., Liu, X., Cui, L., et al. (2022). Genetic mechanism of transfer zones in rift basins: insights from geomechanical models. GSA Bull. 134 (9–10), 2436–2452. doi:10.1130/B36151.1
Luo, J., Morad, S., Liang, Z., and Zhu, Y. (2005). Controls on the quality of Archean metamorphic and Jurassic volcanic reservoir rocks from the Xinglongtai buried hill, western depression of Liaohe basin, China. AAPG Bull. 89 (10), 1319–1346. doi:10.1306/05230503113
Lyu, W., Zeng, L., Zhou, S., Du, X., Xia, D., Liu, G., et al. (2019). Natural fractures in tight-oil sandstones: a case study of the upper triassic yanchang formation in the southwestern ordos basin, China. AAPG Bull. 103 (10), 2343–2367. doi:10.1306/0130191608617115
Maerten, L., Maerten, F., and Lejri, M. (2018). Along fault friction and fluid pressure effects on the spatial distribution of fault-related fractures. J. Struct. Geol. 108, 198–212. doi:10.1016/j.jsg.2017.10.008
Maréchal, J. C., Dewandel, B., and Subrahmanyam, K. (2004). Use of hydraulic tests at different scales to characterize fracture network properties in the weathered-fractured layer of a hard rock aquifer: FRACTURE NETWORK PROPERTIES IN HARD ROCK. Water Resour. Res. 40 (11). doi:10.1029/2004WR003137
Nelson, R. A., and Mold, E. P. (2000). Production characteristics of the fractured reservoirs of the La Paz field. Maracaibo basin, venezuela. AAPG Bull. 84. doi:10.1306/8626C393-173B-11D7-8645000102C1865D
Ortega, O. J., Marrett, R. A., and Laubach, S. E. (2006). A scale-independent approach to fracture intensity and average spacing measurement. AAPG Bull. 90 (2), 193–208. doi:10.1306/08250505059
Pei, L., Wang, X., Wang, Q., Zhang, Q., Luo, H., and Liu, W. (2022). Origin, accumulation and secondary alteration of natural gas around Qingshui Sub-sag, Liaohe Depression, China: insights from molecular and isotopic composition. Mar. Petroleum Geol. 135, 105390. doi:10.1016/j.marpetgeo.2021.105390
Salah, M. G., and Alsharhan, A. S. (1998). The Precambrian basement: a major reservoir in the rifted basin, Gulf of Suez. J. Petroleum Sci. Eng. 19 (3–4), 201–222. doi:10.1016/S0920-4105(97)00024-7
Tong, K., Zhao, C., Lü, Z., Zhang, Y., Zheng, H., Xu, S., et al. (2012). Reservoir evaluation and fracture chracterization of the metamorphic buried hill reservoir in Bohai Bay Basin. Petroleum Explor. Dev. 39 (1), 62–69. doi:10.1016/S1876-3804(12)60015-9
Wang, G., Li, S., Wu, Z., Suo, Y., Guo, L., and Wang, P. (2019). Early paleogene strike-slip transition of the tan–Lu fault zone across the southeast Bohai Bay Basin: constraints from fault characteristics in its adjacent basins. Geol. J. 54 (2), 835–849. doi:10.1002/gj.3344
Wang, J., Xie, H.-P., Matthai, S. K., Hu, J.-J., and Li, C.-B. (2023). The role of natural fracture activation in hydraulic fracturing for deep unconventional geo-energy reservoir stimulation. Petroleum Sci. 20, 2141–2164. S1995822623000079. doi:10.1016/j.petsci.2023.01.007
Wang, N., Xu, Y.-H., Wang, F.-L., Liu, Y., Huang, Q., and Huang, X. (2022). Identification and geochemical significance of unusual C24 tetracyclic terpanes in Shahejie Formation source rocks in the Bozhong subbasin, Bohai Bay Basin. Petroleum Sci. 19 (5), 1993–2003. doi:10.1016/j.petsci.2022.03.025
Wang, Q., Hao, F., Niu, C., Zou, H., Miao, Q., Yin, J., et al. (2021). Origins and deep petroleum dynamic accumulation in the southwest part of the Bozhong depression, Bohai Bay Basin: insights from geochemical and geological evidences. Mar. Petroleum Geol. 134, 105347. doi:10.1016/j.marpetgeo.2021.105347
Wang, R., Ding, W., Zhang, Y., Wang, Z., Wang, X., He, J., et al. (2016). Analysis of developmental characteristics and dominant factors of fractures in Lower Cambrian marine shale reservoirs: a case study of Niutitang formation in Cen’gong block, southern China. J. Petroleum Sci. Eng. 138, 31–49. doi:10.1016/j.petrol.2015.12.004
Wang, W., Yi, J., Shan, X., Zhang, X., Liu, X., Liu, P., et al. (2022). Characteristics of fractures development and its controlling factors within the buried hill reservoirs from the Archaean metamorphic basement in the Bozhong Sag, Bohai Bay Basin, Eastern China. Front. Earth Sci. 10, 935508. doi:10.3389/feart.2022.935508
Wang, X., Wang, R., Ding, W., Yin, S., Sun, Y., Zhou, X., et al. (2017). Development characteristics and dominant factors of fractures and their significance for shale reservoirs: a case study from Є1b2 in the Cen’gong block, southern China. J. Petroleum Sci. Eng. 159, 988–999. doi:10.1016/j.petrol.2017.08.007
Wang, Y., Zhou, L., Liu, S., Li, J., and Yang, T. (2018). Post-cratonization deformation processes and tectonic evolution of the North China Craton. Earth-Science Rev. 177, 320–365. doi:10.1016/j.earscirev.2017.11.017
Wang, Z., Xiao, S., Wang, F., Tang, G., Zhu, L., and Zhao, Z. (2021). Phase behavior identification and formation mechanisms of the BZ19-6 condensate gas reservoir in the deep Bozhong sag, Bohai Bay Basin, eastern China. Geofluids 2021, 1–19. doi:10.1155/2021/6622795
Xu, C., Yu, H., Wang, J., and Liu, X. (2019). Formation conditions and accumulation characteristics of Bozhong 19-6 large condensate gas field in offshore Bohai Bay Basin. Petroleum Explor. Dev. 46 (1), 27–40. doi:10.1016/S1876-3804(19)30003-5
Xu, S., Hao, F., Xu, C., Zou, H., Zhang, X., Zong, Y., et al. (2019). Hydrocarbon migration and accumulation in the northwestern Bozhong subbasin, Bohai Bay Basin, China. J. Petroleum Sci. Eng. 172, 477–488. doi:10.1016/j.petrol.2018.09.084
Ye, T., Chen, A., Niu, C., Luo, J., and Hou, M. (2022). Effective fractures linked with tectonic reactivation and multiple genetic fluids in the ultradeep Paleozoic carbonate buried hills of the Bozhong sag, North China. Mar. Petroleum Geol. 140, 105642. doi:10.1016/j.marpetgeo.2022.105642
Ye, T., Chen, A., Niu, C., Wang, Q., and Guo, L. (2020). Characteristics and vertical zonation of large-scale granitic reservoirs, a case study from Penglai oil field in the Bohai Bay Basin, North China. Geol. J. 55 (12), 8109–8121. doi:10.1002/gj.3932
Ye, T., Chen, A., Niu, C., and Wang, Q. B. (2021). Structural, petrophysical and lithological characterization of crystalline bedrock buried-hill reservoirs: a case study of the southern jinzhou oilfield in offshore Bohai Bay Basin, north China. J. Petroleum Sci. Eng. 196, 107950. doi:10.1016/j.petrol.2020.107950
Yi, J., Li, H., Shan, X., Hao, G., Yang, H., Wang, Q., et al. (2022). Division and identification of vertical reservoir units in archaeozoic metamorphic buried hill of Bozhong sag, Bohai Bay Basin, east China. Petroleum Explor. Dev. 49 (6), 1282–1294. doi:10.1016/S1876-3804(23)60349-0
Yin, S., Ding, W., Zhou, W., Shan, Y., Wang, R., Liu, J., et al. (2016). Logging assessment of tight clastic rock reservoir fractures via the extraction of effective pore aspect ratios: a case study of lower Permian strata in the southern Qinshui Basin of eastern China. J. Nat. Gas Sci. Eng. 36, 597–616. doi:10.1016/j.jngse.2016.11.001
Yin, S., Han, C., Wu, Z., and Li, Q. (2019). Developmental characteristics, influencing factors and prediction of fractures for a tight gas sandstone in a gentle structural area of the Ordos Basin, China. J. Nat. Gas Sci. Eng. 72, 103032. doi:10.1016/j.jngse.2019.103032
Yu, F., and Koyi, H. (2016). Cenozoic tectonic model of the Bohai Bay Basin in China. Geol. Mag. 153 (5–6), 866–886. doi:10.1017/S0016756816000492
Zeng, L., Gong, L., Guan, C., Zhang, B., Wang, Q., Zeng, Q., et al. (2022). Natural fractures and their contribution to tight gas conglomerate reservoirs: a case study in the northwestern Sichuan Basin, China. J. Petroleum Sci. Eng. 210, 110028. doi:10.1016/j.petrol.2021.110028
Zeng, W., Zhang, J., Ding, W., Zhao, S., Zhang, Y., Liu, Z., et al. (2013). Fracture development in Paleozoic shale of Chongqing area (South China). Part one: fracture characteristics and comparative analysis of main controlling factors. J. Asian Earth Sci. 75, 251–266. doi:10.1016/j.jseaes.2013.07.014
Zhao, X., Jin, F., Wang, Q., and Bai, G. (2015a). Buried-hill play, Jizhong subbasin, Bohai Bay basin: a review and future propespectivity. AAPG Bull. 99 (01), 1–26. doi:10.1306/07171413176
Keywords: tectonic fractures, deep metamorphic rock, buried hill, faulted basin, main controlling factors
Citation: Liu Z, Cheng Q, Liao X, Zhang L, Liu W and Zhang G (2024) Characteristics and controlling factors of tectonic fractures within the buried hill reservoirs from the Archaean metamorphic basement: a case study in the Bozhong 19–6 condensate gas field, Bohai Bay Basin. Front. Energy Res. 12:1309037. doi: 10.3389/fenrg.2024.1309037
Received: 07 October 2023; Accepted: 03 January 2024;
Published: 12 January 2024.
Edited by:
Peng Tan, CNPC Engineering Technology R & D Company Limited, ChinaReviewed by:
Hu Li, Southwest Petroleum University, ChinaZhipeng Huo, Northeastern University at Qinhuangdao, China
Copyright © 2024 Liu, Cheng, Liao, Zhang, Liu and Zhang. This is an open-access article distributed under the terms of the Creative Commons Attribution License (CC BY). The use, distribution or reproduction in other forums is permitted, provided the original author(s) and the copyright owner(s) are credited and that the original publication in this journal is cited, in accordance with accepted academic practice. No use, distribution or reproduction is permitted which does not comply with these terms.
*Correspondence: Guanjie Zhang, emdqMTk5Njg4QDE2My5jb20=