- School of International Business, Qingdao Huanghai University, Qingdao, China
The pressing issues of climate change and the limited availability of non-renewable energy resources have created a growing need for sustainable energy alternatives. This study provides a comprehensive overview of the pressing need for sustainable energy solutions and the complex relationship between energy and the economy. The challenges and opportunities presented by the transition to sustainable energy sources are explored, including the need for investment in renewable energy technologies, policy changes to incentivize sustainable energy use, and the potential for job creation in the sustainable energy sector. On the other hand, it is recognized that there are considerable hurdles that need to be addressed, including the substantial initial expenses associated with establishing renewable energy systems, as well as the political and societal barriers to enacting change. The economic benefits of transitioning to sustainable energy, such as improved energy security, reduced dependence on fossil fuels, and the potential for increased economic growth, are evaluated. The complex relationship between energy and the economy is thoroughly analyzed, presenting a valuable contribution to the academic literature on sustainable energy. Furthermore, an inquiry is being made into the potential contribution of blockchain technology in advancing a sustainable energy landscape. This includes its ability to augment the effectiveness and openness of energy markets, as well as its capacity to assist in the assimilation of renewable energy resources. Hence, this research underscores the importance of transitioning to sustainable energy sources for their environmental and economic merits. The findings presented offer valuable insights to inform policy decisions and guide future research endeavors in this field. By promoting the advancement of sustainable energy technologies, this study contributes to the development of a more sustainable global economy.
1 Introduction
The significance of energy in the functioning of a nation’s economy and society cannot be overstated. Nevertheless, the bulk of global energy demand is still satisfied by non-renewable fossil fuels like oil, coal, and natural gas (Abban et al., 2022; Amin et al., 2022). Nonetheless, these sources are finite, contribute to environmental pollution and climate change, and are progressively more arduous and costly to extract. Consequently, an urgent and imperative need exists to shift towards sustainable energy alternatives that are both renewable and cleaner, capable of mitigating the detrimental impacts of climate change. This transition towards sustainable energy presents a spectrum of challenges and opportunities for the global economy. Fossil fuels have long served as the predominant energy source worldwide due to their high energy density and affordability. However, the combustion of these fuels has resulted in emissions that have caused escalating global temperatures, an increase in extreme weather events, and a myriad of other catastrophic environmental transformations (Ansari et al., 2022; Asif et al., 2022; Chenic et al., 2022). As a result, numerous scholars have extensively investigated this subject matter through the utilization of modeling, empirical investigations, and optimization techniques (Li et al., 2018; Xu et al., 2022; Tong et al., 2023; Xie et al., 2023; Yin et al., 2023).
The primary objective of every society is to achieve development in all its dimensions (Meinshausen et al., 2022). Access to high standards of energy production and consumption is a key indicator of a country’s success in achieving development. However, energy production and consumption, whether fossil or non-fossil, pose significant threats and challenges to the environment and sustainable development, despite their role in contributing to economic growth and development (Chien, 2022; Fang et al., 2022; Farghali et al., 2023). Sustainable energy can only be achieved through the integration of the environment, energy, and development sectors. Conversely, the absence of integration can lead to conflicts between the objectives of these sectors, which can hinder the achievement of sustainable development (Holechek et al., 2022; Ishaq et al., 2022; Islam et al., 2022). At the international level, policies related to energy and development have been largely aligned, while environmental policies have not. The misalignment between these factors can create difficulties and discord, and resolving this dilemma requires the development of effective solutions by the global community that strike a balance between energy supply security, economic advancement, and environmental safeguarding. Hence, the primary obstacle to sustainable development in the energy industry is to ensure that the advantages of energy services are extended to the world’s population and future generations without causing harm to the environment (Nnabuife et al., 2022; Meydani, 2023; Mohideen et al., 2023).
During the 1980s and 1990s, energy-related matters garnered considerable attention; however, environmental considerations within the energy sector were largely overlooked by governments. The intricate nature and diverse array of interests involved posed challenges in implementing substantial policy changes. Moreover, many countries possessing oil and gas resources faced constraints in terms of political, economic, and technological capacities, impeding their ability to transition towards sustainable development by reducing dependence on fossil fuels and transforming core economic strategies. The substantial reliance on non-renewable fossil fuels in global energy production and consumption has proven to be a significant obstacle in achieving sustainable development objectives (Kanwal et al., 2022; Jie et al., 2023; Kocak et al., 2023). Sustainable energy is characterized by a lower per capita production of greenhouse gases. However, unstable energy production and consumption patterns have led to several environmental problems, including climate change, acid rain, ozone depletion, nuclear radiation, urban air pollution, and marine pollution caused by oil transportation. Developing and developed countries have blamed each other for these issues, with developing countries accusing developed countries of environmental destruction due to excessive energy consumption resulting from increased demand, and developed countries accusing developing countries of environmental degradation due to increased consumption resulting from population growth (Nnabuife et al., 2022; Meydani, 2023). Both claims are valid, but research indicates that developing and developed countries face different challenges in relation to the environment and energy. Developing countries face energy resource scarcity and lack of access to energy, while developed countries face pollution and energy waste (Luo et al., 2013; Gielen et al., 2016). The international community eventually recognized that sustainable development cannot be achieved without sustainable energy (Adamowicz, 2022).
When discussions about the pollution caused by traditional energy sources arise, there is often a shift towards non-conventional energy sources (Ramzan et al., 2022; Mohideen et al., 2023). Lovin’s guidelines, introduced in 1972, provide a precise framework for establishing acceptable and conventional energy policies using both hard and soft methods. In Lovin’s model, the hard path emphasizes the rapid development of energy resources such as coal and nuclear energy, with sustainable energy production for low consumption and efficiency being highlighted. This approach involves extensive research on nuclear energy and rapid development of energy resources like coal. Lovin’s theory suggests that the hard path leads to the creation of elite technology, concentration of economic and political power, vulnerability to technological threats, and the potential for social and economic injustice and complexity (Lovins, 1974; Lovins, 1976). On the other hand, the soft energy path focuses on limiting energy production and maximizing efficiency in consumption. Soft energy leads to a small, decentralized system and is flexible, sustainable, and environmentally safe. The advantages of this approach are manifold and include the potential to extend energy generation beyond centralized production centers, self-sufficiency in energy supply, reduced reliance on public or private energy infrastructure, a preference for renewable energy over non-renewable sources, eliminating the use of uneven energy resources, prioritizing energy conservation, and employing low-risk technology that is suitable for energy sources with high risk factors (Takase et al., 2022; Yasmeen et al., 2022; Sharma et al., 2023).
Renewable energy technologies have experienced significant advancements in recent years, with increased efficiency and declining costs. They offer a promising pathway to a sustainable energy system, but significant investments and policy changes are still necessary to facilitate the transition. The high upfront costs associated with renewable energy infrastructure remain a significant barrier for many. Government policies and incentives that favor renewable energy development and use are crucial to overcoming the obstacles to adoption and accelerating the transition towards sustainability. Some key policies that can support renewable energy include tax incentives, direct subsidies, renewable energy mandates, and carbon pricing (Siddik et al., 2023; Zakaria et al., 2023). Through appropriate policies and economic support, renewable energy has the potential to surpass fossil fuels in competitiveness. The transition to renewable energy offers various economic advantages, including the creation of new job opportunities in the sustainable energy sector, enhanced energy security and independence, and long-term cost savings. The renewable energy industry is generating numerous employment opportunities in manufacturing, technology, installation, and related fields. Shifting towards locally available renewable sources also enables countries to achieve greater energy security and independence. While renewable energy technologies often involve initial high costs, their operating costs remain low as the sun or wind (as fuel sources) is freely available. Over time, these technologies can yield cost savings alongside environmental benefits. Nevertheless, the transition presents economic challenges that necessitate attention. Established interests striving to maintain the status quo may pose social and political obstacles. A successful transition requires initiatives such as worker retraining programs, support for affected communities, and effective communication with citizens and policymakers to highlight the advantages of renewable energy. Nonetheless, with the rapid decline in the costs of renewable technologies, the economic arguments in favor of sustainability and renewables are becoming more compelling over time (Takase et al., 2022; Yu et al., 2023; Zhang et al., 2023).
The implementation of blockchain technology presents a significant opportunity for advancing sustainable energy systems. This technology operates as a decentralized ledger that records transactions in a permanent and unalterable manner, thereby enabling transparent monitoring of energy production and consumption throughout the network. Numerous projects are leveraging blockchain to enable peer-to-peer energy trading between producers and consumers, monitor the origin of renewable energy generation, and facilitate innovative financing models for renewable energy projects. The intermittency of renewable energy sources poses a significant challenge for renewable energy. Blockchain can help address this by enabling decentralized energy trading networks. When a solar panel owner generates excess energy, they can sell it to neighboring buildings using smart contracts on the blockchain. Neighbors who need additional energy can buy it instantly in a transparent marketplace, resulting in minimal waste and improved overall efficiency and reliability of renewable energy (Afzal et al., 2022; Gawusu et al., 2022; Dwivedi et al., 2023). Several startups facilitating these energy trading networks have launched in New York, California, and European countries. Another promising application of blockchain for sustainable energy is renewable energy certification and tracking the origin of energy. Certification systems like Renewable Energy Certificates (RECs) help fund renewable energy projects by enabling businesses and individuals to purchase renewable energy credits. However, the current system for RECs involves cumbersome paperwork, administration fees, and a lack of transparency. Implementing RECs on an open blockchain platform can reduce costs, simplify the process, and provide a clear link between the generation and consumption of renewable energy. Several companies are piloting blockchain-based renewable energy certificate platforms (Juszczyk and Shahzad, 2022; Polas et al., 2022; Wu et al., 2022).
Although there has been a proliferation of research on sustainable energy and the potential of emerging technologies, there still exists a discernible gap in knowledge regarding the assimilation of blockchain technology in the shift towards sustainable energy alternatives. Additionally, the interplay between energy policy, economic factors, and technological advancements in facilitating this transition has not been comprehensively explored. Thus, the importance of this study lies in addressing this research gap and providing valuable insights into the challenges, opportunities, and implications of transitioning to sustainable energy sources, while emphasizing the role of blockchain technology and policy changes. The primary objective of this investigation is to examine the intricate facets of the worldwide energy milieu and assess the viability of blockchain technology in advancing sustainable energy solutions. The research methodology employed in this study is a qualitative research design, which includes an extensive literature review and content analysis. This systematic approach facilitates a thorough examination of the interconnections among energy, policy, technology, and the economy in the transition towards sustainable energy sources. The study’s findings have multiple practical implications, including offering evidence-based insights for policymakers and industry stakeholders in the formulation of sustainable energy strategies. Moreover, the research outcomes inform future studies in this field. These insights play a crucial role in enhancing policy effectiveness, encouraging investments in renewable energy technologies, and fostering the expansion of the sustainable energy sector. Ultimately, this study aims to advance the understanding of the role of blockchain technology and policy changes in facilitating the transition to sustainable energy sources, and the benefits and challenges associated with this transition, thereby contributing to the development of a more sustainable and resilient global economy.
2 Fundamental concept of the study and definitions
2.1 Sustainable energy and technologies
The term sustainable energy pertains to energy resources that can fulfill existing energy requirements while preserving the capacity of future generations to meet their own energy needs. This widely accepted definition is supported by scientific literature and the international community. The adoption of sustainable energy solutions is essential in curtailing the release of greenhouse gases into the environment and ameliorating the impacts of climate change. Moreover, it promotes energy security by decreasing reliance on fossil fuels and promoting energy independence. The development and implementation of sustainable energy technologies require a complex process that involves technological innovation, policy support, and public awareness (Chai and Zhang, 2010; Qazi et al., 2019).
Various sustainable energy technologies are currently being developed, including solar PV, wind turbines, geothermal energy, energy storage, smart grids, hydrogen fuel cells, and biofuels. Solar PV technology converts sunlight into electricity through solar panels and is rapidly advancing, becoming more efficient and cost-effective. Biofuels, derived from renewable biomass sources, are becoming more sustainable and environmentally friendly than traditional fossil fuels due to advances in technology. These technologies aim to provide a stable and reliable supply of electricity while balancing energy supply and demand, integrating renewable energy sources into the grid, and offering a clean source of energy for transportation and other applications. Also, they are expected to become more efficient, cost-effective, and widely adopted as research and development continue. They hold the potential to build a sustainable energy future, addressing the world’s growing energy needs while reducing the environmental impact of energy production and use (Chu and Majumdar, 2012; Vujanović et al., 2021).
2.2 Economic benefits of sustainable energy transition
Sustainable energy practices, policies, and technologies offer numerous economic benefits, including cost savings, job creation, and increased competitiveness. The adoption of renewable energy sources can reduce dependence on fossil fuels, which are susceptible to price volatility and supply disruptions. Renewable sources like solar and wind power have become cost-competitive with traditional sources in many regions, with costs expected to decrease further. Energy efficiency measures can also reduce energy costs by improving energy use efficiency and decreasing waste. The development and deployment of sustainable energy technologies create new job opportunities in industries such as renewable energy, energy efficiency, and energy storage. Businesses that adopt sustainable energy practices and technologies can reduce energy costs, enhance their reputation, and meet the growing demand for sustainable products and services, leading to increased market share and profitability (Bulavskaya and Reynès, 2018; Osorio-Aravena et al., 2021; Dong et al., 2022; Tirkolaee et al., 2022; Wang H. et al., 2023). The shift towards a sustainable energy future can have a positive impact on public health by decreasing air pollution, which has severe health consequences. The costs of air pollution to the environment and public health can be considerable, and the adoption of sustainable energy practices and technologies can help to minimize these costs. The integration of sustainable energy practices and technologies can also decrease the environmental impacts related to energy production and use, such as land use impacts and greenhouse gas emissions. The environmental costs of conventional energy sources can be significant, and the adoption of sustainable energy practices and technologies can help to address these costs and promote a more sustainable and resilient energy system. To conclude, the implementation of sustainable energy practices, policies, and technologies can offer numerous economic benefits, including job creation, cost savings, improved public health outcomes, and reduced environmental impacts. By leveraging technologies like blockchain to enhance the efficiency and transparency of energy systems, we can expedite the transition to an energy system that is both sustainable and resilient and that benefits the economy and the environment (Jenniches, 2018; Gielen et al., 2019; Ghasemi et al., 2021; Mirzaei et al., 2021).
2.3 Blockchain for sustainable energy
Distributed ledger technology, known as blockchain, facilitates secure, transparent, and tamper-proof recording of data and transactions. It operates by using a decentralized network of computers to validate and verify transactions, thereby eliminating intermediaries like banks or governments. The integration of blockchain technology presents significant potential in the shift towards a sustainable energy future, as it enables more efficient and transparent energy systems. It can address many of the challenges associated with the transition by enabling more effective management of energy systems, improving energy efficiency and reducing waste, and increasing transparency and accountability in energy systems (Arabian et al., 2022; Barenji and Nejad, 2022). Blockchain technology can provide a secure and transparent platform for tracking the production and consumption of energy and enables peer-to-peer energy trading (Wu and Tran, 2018; Ahl et al., 2020; Otoum et al., 2022; Goli, 2023). Additionally, blockchain-based smart contracts can automate energy transactions and incentivize energy conservation, leading to more efficient and sustainable energy use. Blockchain technology can help address this challenge by enabling the development of smart grid systems that use real-time data to optimize energy production, storage, and consumption. These systems can automate energy transactions and incentivize energy conservation through blockchain-based smart contracts, leading to more efficient and sustainable energy use (Andoni et al., 2019; Akram et al., 2020).
2.4 Policy changes for sustainable energy
This study highlights the significance of policy adjustments in facilitating the transition towards a sustainable energy future. Policy modifications encompass the development and implementation of new regulations and policies designed to promote sustainable energy practices and technologies. These policy changes can manifest at different levels, ranging from local to global, and can take various forms. Examples of policy changes that can advance sustainable energy practices include the implementation of incentives and targets for renewable energy adoption, the establishment of standards and incentives for energy efficiency improvements, and the enactment of policies that impose a price on carbon emissions (Kern and Smith, 2008; Kuzemko et al., 2016). According to scientific literature, policy changes are essential in promoting a sustainable energy future. As a result, policymakers must meticulously design policies that can achieve the desired outcomes while minimizing potential negative impacts. The use of blockchain technology can also be critical in enabling more efficient and transparent energy systems. Therefore, policymakers must prioritize policy changes that promote sustainable energy practices and technologies to expedite the transition to a sustainable and resilient energy system. The success of these policies depends on meticulous consideration of political, economic, and social factors, along with the potential role of blockchain technology in facilitating more efficient and transparent energy systems (Streimikiene and Šivickas, 2008; Lu et al., 2020; Yildizbasi, 2021).
3 Research methodology
The current research employs a qualitative research methodology to investigate the potential of blockchain technology in promoting the adoption of sustainable energy alternatives. The choice of a qualitative research design is deemed appropriate as it allows for a comprehensive understanding of complex social phenomena, specifically the global energy system and the transition towards sustainable energy sources. By utilizing a qualitative approach, this study can explore the intricate relationships between energy, sustainability, policy, technology, and the economy in an open-ended and flexible manner.
To gather data, an extensive review of pertinent scientific literature, policy documents, industry reports, and media articles was undertaken. The literature review encompassed various domains, including sustainable energy, renewable energy policy, energy economics, blockchain technology, and innovation. This comprehensive review provided a foundation for identifying key themes, challenges, opportunities, and arguments concerning the transition towards sustainable energy sources and the potential role of blockchain technology and policy changes in facilitating this transition.
In this research study, the analysis primarily relied on secondary data obtained from reliable sources. Through this analysis, the study aimed to identify and examine the main themes and insights related to the transition towards sustainable energy sources and the potential of blockchain technology. By drawing upon a wide range of sources, this study sought to gain a deeper understanding of the challenges, opportunities, and arguments surrounding the integration of blockchain technology in the pursuit of sustainable energy alternatives.
The collected data underwent qualitative content analysis, which involved identifying key concepts, themes, and arguments. The data was categorized based on focal areas such as sustainable energy technologies, economic factors, policy issues, blockchain applications, challenges, and opportunities. Through this coding process, relationships between categories and overarching themes were established. The study synthesized the significant findings and insights, leading to a comprehensive discussion of various aspects. These included the current state of the global energy system, the imperative need for transitioning to sustainable energy sources, challenges and opportunities associated with the transition, the role of policy changes and blockchain technology in facilitating the transition, and the implications for the economy.
To minimize bias, the data collection and analysis process incorporated multiple perspectives from diverse and reputable sources. Various viewpoints were considered to ensure a comprehensive analysis. The study substantiated its findings with evidence from the literature, while also considering alternative explanations or counterarguments.
The research process was transparently documented, allowing for scrutiny of the logical reasoning behind the analysis and conclusions. Additionally, member checking was conducted by presenting the preliminary findings to experts in the fields of energy policy and blockchain technology. Their feedback was incorporated, further enhancing the credibility, transferability, dependability, and confirmability of the study.
Consequently, this study adopted a qualitative research design that encompassed an extensive literature review and content analysis to comprehensively investigate the research topic and fulfill the study objectives. By employing this methodology, a systematic and rigorous process was established to gain profound insights into the intricate relationships among energy, policy, technology, and the economy within the context of transitioning towards sustainable energy sources.
4 Finding and discussion
4.1 Exploring the environmental impacts of sustainable energy
The overconsumption of fossil fuels has resulted in an increase in air pollution and global warming, which has propelled climate change to the forefront of public discourse (Leiserowitz, 2007; Perera, 2018). As a result, there is a growing demand for alternative energy sources, particularly renewable energy. However, research has indicated that renewable energy sources may also have negative environmental impacts (Al-Shetwi, 2022; Rahman et al., 2022). This section focuses on the environmental effects of solar energy, wind energy, and hydroelectric systems, including their impact on air pollution, soil quality, noise levels, and wildlife.
4.1.1 Environmental impacts of solar energy
Solar energy is acknowledged as the most significant renewable energy source due to its simplicity of deployment and eco-friendly characteristics in contrast to other energy sources. There are various methods of converting direct solar energy into useable energy, such as solar heating systems, building systems, and photovoltaic systems. The installation of solar energy production systems requires large ground components that can absorb a significant amount of solar energy without being too expensive. It is ideal that these components are not placed in agricultural or forested areas, and they should be located near population centers to reduce transportation costs and energy loss. The northwestern region of the unit is the ideal location for the central system due to its high sunlight exposure (Xu et al., 2021; Jiang J. et al., 2022; Cai et al., 2022; Yu and Zhou, 2023). However, the effects of large-scale solar units on desert ecosystems need to be investigated. Additionally, the construction and equipment protection of solar units utilize many materials such as glass, cement, and steel. During the construction phase, pollution effects need to be studied, as it is estimated that the amount of materials required for solar units is greater than that of fossil-fuel units. Photovoltaic-based units use unconventional and toxic materials such as cadmium sulfide, which is flammable. Large-scale use of solar energy creates significant problems in terms of water pollution due to the use of anti-icing agents, anti-corrosion agents, and metals that enter the water during system washing. The use of herbicides to prevent weed growth around collectors also indirectly leads to water pollution (Mahajan, 2012; Hosenuzzaman et al., 2015; Tawalbeh et al., 2021).
The environmental impacts of solar energy production systems are significant and include permanent land use during the unit’s operation, as well as the production of non-renewable materials such as insulation and glass. Photovoltaic-based systems also produce toxic materials such as cadmium and arsenic. Other adverse effects include damage to the landscape, eye damage due to reflection of solar radiation, and land erosion and compaction, wind deflection, and increased potential for soil evaporation. An analysis conducted in 1977 compared the particulate pollution associated with the construction of a solar unit to that of oil or coal-based units producing the same amount of energy. The study found that solar units produced significantly less particulate matter. It is important to carefully consider the costs, hazardous waste, and land use associated with solar energy production compared to other forms of energy. Although there is potential for large-scale replacement of nuclear and fossil-fuel units with solar units, the risks posed by solar technologies, such as safety and health risks, must also be taken into account. For photovoltaic-based units, the most significant negative effect is water consumption for cooling, which can lead to the destruction of surface and underground units. This can also destroy the habitats of soil-dwelling organisms and other animals that live in the desert. Additionally, the energy produced must be transported to residential and industrial centers, resulting in significant energy loss during transmission (Hernandez et al., 2014; Mahmud et al., 2018; Sánchez-Pantoja et al., 2018).
Solar energy is deemed an eco-friendly energy option for temperature regulation through heating and cooling. Research has shown that the only negative consequence of this method is the potential for urban aesthetic issues. In some cases, compatibility issues may arise between solar systems and trees near homes, and the extensive use of collectors installed on roofs can alter reflection and have minor impacts on weather patterns. However, these changes are not considered significant threats to the environment. The only environmental risk associated with solar energy for heating and cooling is during the construction of the equipment in factories (Kumar et al., 2020; Rabaia et al., 2021; Tang et al., 2022; Yavari et al., 2022).
4.1.2 Environmental impacts of wind energy
Wind energy is considered the least risky among energy sources due to its lack of need for a cooling system. However, wind energy has its disadvantages, such as noise pollution, interference with nature, and reduction of the area’s aesthetic appeal. Wind turbines may also cause damage to the ecosystem by reducing wind speed, leading to warmer lakes and decreased surface evaporation. Nonetheless, the environmental effects of wind turbines are not significant. Risks to human safety during construction and operation are similar to those in other industries, with small wind turbines in densely populated areas posing a greater risk to human health. Wind turbines can pose a risk to birds since they may not be able to avoid the high-speed blades. There are two types of pollution associated with wind turbines: machine noise, which can be mitigated through proper design and sound insulation measures, and rotational noise generated by the vortex flow of air. Additionally, wind motion can produce noise, which is often perceived as pleasant and enjoyable, particularly at higher wind speeds (Wang and Wang, 2015; Dhar et al., 2020; Nazir et al., 2020). However, low-frequency and subsonic sounds may cause vibrations in houses and metal structures, particularly in turbines that react with the tower based on blades. Wind turbines may also create signals that interfere with television waves and cause disturbances in rainfall and surface evaporation from the ground due to wind movement resulting from windmills on the ground. Despite limited cases of such disturbances reported in 1995, the impact is not considered significant. Energy storage or auxiliary systems are required for wind energy, though it should be noted that these systems are more vulnerable to wind energy. Auxiliary facilities are used in high-risk situations (Etheridge, 2000). Distributed wind energy systems are considered more environmentally compatible than other energy sources if wind turbines are scattered throughout the country’s agricultural lands and connected to a network. In this scenario, only a small portion of the turbines would be considered undesirable (Jaber, 2013; Mendecka and Lombardi, 2019).
4.1.3 Environmental impacts of large and small hydroelectric projects
While large hydroelectric energy production projects are considered renewable energy sources, micro and small water systems are classified as unconventional energy sources. Before discussing the potential effects of small and micro water systems projects, it is important to briefly examine the main findings of experiments conducted by scientists. Although environmental impacts are expected to be smaller and different in smaller systems (Egré and Milewski, 2002; Abbasi and Abbasi, 2011; Başkaya et al., 2011).
Hydroelectric energy production projects have been extensively studied alongside thermal units in terms of their environmental impacts. While some experts believe that hydroelectric units, particularly large ones, have negative effects on the environment and have the greatest destructive impact compared to other renewable energy sources, there is not a complete consensus. In the 1950s, when only a few of these units were in use worldwide, it was believed that this energy source was the cleanest form of energy compared to other sources. Water is one of the most expensive and even the most expensive natural resource, and dams provide this water in abundant quantities and in a way that can be used several times. Dams provide the possibility of water use throughout the year (for public use, fishing, and recreation). After electricity is obtained from water, it can be used for irrigating agricultural lands downstream of the dam. During this process, it is possible to recharge underground water resources. This energy and these benefits will be obtained without creating any smoke from thermal units or any hazardous waste from nuclear power plants (Jumani et al., 2017; Nautiyal and Goel, 2020; Oladosu et al., 2021). However, now, after 50 years, such units are considered hazardous by some experts. The most important environmental effects caused by large hydroelectric units are storing rainfall in the area, creating an artificial lake, reducing water flow downstream, and changing the flow of the river. Changes in the water flow can have various environmental effects, including increased water evaporation and potential soil leakage, displacement of small aquatic organisms, formation of distinct temperature layers, alterations in habitat and food availability, and reduction of inhabitable lands due to the creation of artificial lakes. Moreover, there is a risk of nutrient accumulation in the lake area and downstream river regions. Organisms located at river mouths may experience negative impacts due to the mixing of saltwater and the diminished flow of freshwater. Reproduction of organisms, fish, and other aquatic conditions are affected by changes in river flow and move towards the river’s border areas. Increased water stagnation and human activities in the lake area lead to increased deforestation and reduced animal habitats. Often, water-borne diseases increase in these areas. The latest research shows an increase in the production of greenhouse gases such as methane from the lakes created by human-made dams. Some researchers believe that the amount of gas emitted by these units is comparable to the gases emitted in fossil fuel units, although there are differences between the greenhouse gases produced by humans. This recent issue is more significant in terms of weight and size than other problems resulting from hydroelectric systems (Pinho et al., 2007; Pang et al., 2015).
Small and micro-hydro systems are created by constructing numerous small dams with low heads or generators placed in the path of water flow. China leads other countries in having small hydro units, with approximately 100,000 units in rural areas. In 1980, the Philippines generated 4 MW of electricity from these units. However, these units are not without their problems, and their production in kilowatts is not significantly higher than centralized energy units. Some of the environmental impacts associated with these units include hindering animal movement, increasing water evaporation due to slow movement, reducing river boundaries and animal habitats, which incurs high costs to create such habitats elsewhere. Access roads must be built to reach these units, which in turn harms the environment. These units also contribute to environmental degradation by producing sediment and accumulating nutrients, which is a significant problem in large and small hydro units, leading to a decrease in the depth and size of the space. The production of greenhouse gases is another problem associated with these units, as they act as shallow water reservoirs that emit gases such as methane. Therefore, the environmental impacts of small and dispersed hydro units are significant and influential in all cases, and more attention should be paid to their potential negative effects (Gleick, 1992; Hennig et al., 2013; Zeleňáková et al., 2018).
Figure 1 presents an outline of the environmental consequences of renewable energy sources, with particular emphasis on wind energy, solar energy, and hydroelectric systems. The figure highlights the potential adverse effects of these energy sources on greenhouse gas emissions, wildlife habitats, noise pollution, water pollution, and land use. A thorough evaluation of the costs and benefits of each energy source, as well as careful consideration of these impacts, is crucial when transitioning to renewable energy sources.
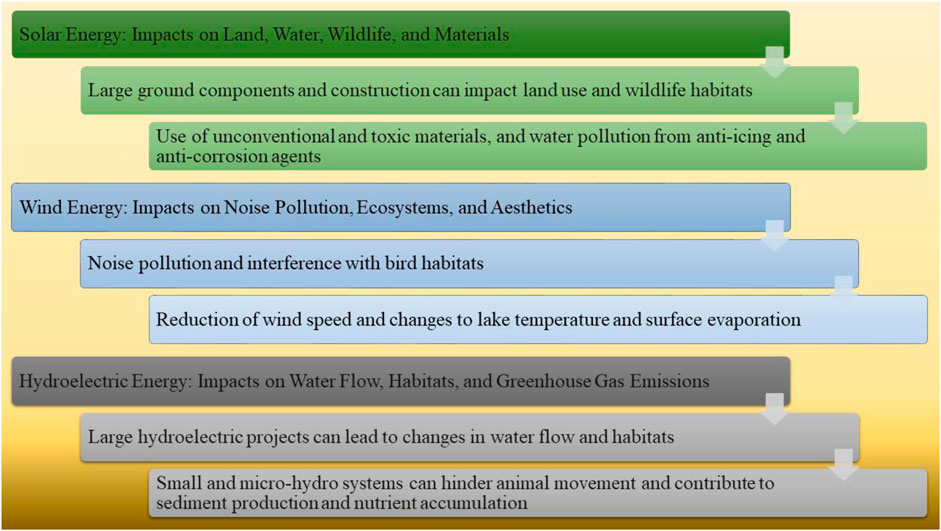
FIGURE 1. Assessing the environmental impacts of renewable energy sources: a comprehensive overview of solar, wind, and hydroelectric energy.
4.2 Challenges and opportunities in the transition to sustainable energy sources
The shift towards sustainable energy sources poses both opportunities and obstacles. One of the most significant obstacles is the requirement for investment in renewable energy technologies, as the current energy infrastructure is heavily reliant on traditional energy sources. The adoption of sustainable energy sources necessitates substantial investment in renewable energy technologies such as hydro, wind, solar, and geothermal, which is essential in ensuring their accessibility and affordability, facilitating widespread implementation (Liu L. et al., 2022; Chen, 2022; Guo B. et al., 2023; Liu et al., 2023). Another significant challenge is policy changes to incentivize sustainable energy use. Governments play a critical role in promoting sustainable energy use through policy changes. These policies create a favorable environment for renewable energy technologies to thrive, making them more accessible and affordable for consumers. However, implementing these policies can be challenging, requiring political will and public support (Dominković et al., 2018; Hassan et al., 2019; Ghasemi et al., 2022; Al-Housani et al., 2023; Ibeanu et al., 2023).
Despite these challenges, the transition to sustainable energy sources presents significant opportunities, such as job creation in the sustainable energy sector. The sustainable energy sector is an industry that is expanding rapidly, offering significant potential for generating new jobs. Job opportunities in the sustainable energy sector range from manufacturing and installation to research and development, offering a wide range of opportunities for workers with diverse skill sets. The shift towards sustainable energy sources also presents an opportunity for a more environmentally-friendly world. The adoption of sustainable energy sources like hydro, wind, and solar can mitigate the adverse environmental effects of traditional energy sources, resulting in a cleaner and healthier environment. Additionally, renewable energy technologies are inexhaustible and do not produce greenhouse gas emissions (Armaroli and Balzani, 2007; Magar et al., 2023; Owusu and Asumadu-Sarkodie, 2016; Bahlouli et al., 2023).
The sustainable energy sector offers promising prospects for job creation, yet it faces various challenges. One of the significant challenges is the skills gap, requiring a highly skilled workforce with specialized technical skills, including engineering, science, and technology. Nonetheless, the sustainable energy sector is relatively new, and many workers may not have prior experience in this field. As a result, companies may face challenges finding experienced workers, leading to longer training periods and additional costs. Another challenge is the uncertainty of government policies, such as tax incentives and renewable energy standards, which can make it difficult for companies to plan and invest in their workforce. Moreover, funding challenges pose a significant hurdle for the sustainable energy sector, as it requires significant investment to develop and expand (Vidadili et al., 2017; Pérez et al., 2019; Bayulgen, 2020; Ishaq et al., 2022). Many companies may not have the resources to invest in expanding their workforce, and securing funding can be difficult due to the high risk associated with the sector. Additionally, the competition with established industries, such as oil and gas, makes it challenging for the sustainable energy sector to attract workers and establish a foothold in the job market. To overcome these challenges, a collaborative effort between governments, businesses, and educational institutions is required to create the necessary skills, policies, and funding to support job creation in this critical sector. This effort can include upskilling workers in specialized technical skills, providing training and education programs to develop experience, and creating more predictable and supportive government policies. Furthermore, funding opportunities and incentives can be developed to support the growth of the sustainable energy sector and reduce the risk associated with investing in it (Erat et al., 2021; Kabeyi and Olanrewaju, 2022; Guo L. et al., 2023; Japir Bataineh et al., 2023).
Academic institutions have a crucial role to play in filling the skills gap in the sustainable energy sector. To tackle this challenge, there are a variety of approaches that educational institutions can take to narrow this gap. One such step is to offer targeted programs tailored to the specific needs of the sustainable energy sector. These initiatives aid students in acquiring the technical skills and understanding necessary to excel in the sustainable energy sector, such as sustainable energy management or renewable energy engineering. Collaborating with industry partners can also help ensure that educational programs align with current and future industry needs, enabling students to acquire the necessary skills and knowledge to work in the sustainable energy sector. In addition, hands-on training is crucial in the sustainable energy sector. Educational institutions can provide students with access to labs, workshops, and internships to gain practical experience working with renewable energy technologies. Furthermore, incorporating sustainability into existing programs, such as business or engineering, can help students understand the importance of sustainability in all fields. Continuing education programs can also help bridge the skills gap by providing established industry workers with the necessary skills and knowledge required to work in renewable energy. Finally, educational institutions can provide career services to help students and graduates find jobs in the sustainable energy sector, including job fairs, networking opportunities, and career counseling (Di Somma and Graditi, 2002; Kyriakopoulos et al., 2022).
The sustainable energy sector holds a plethora of opportunities for job creation, but it is not without its challenges. One such challenge is the skills gap, which necessitates a workforce with specialized technical skills in engineering, science, and technology. Unfortunately, there is a shortage of skilled workers in this area, and the supply of qualified candidates does not always align with the demand, posing a significant challenge for companies seeking to expand their workforce. Another challenge is the limited experience of workers in the sustainable energy sector, a relatively new field. As a result, companies may face difficulties finding experienced workers, which can lead to lengthier training periods and additional expenses. Moreover, government policies play a crucial role in job creation in the sustainable energy sector. Furthermore, the sustainable energy sector requires substantial investment to develop and expand, and securing funding can be challenging due to the high risk associated with the sector. Consequently, many companies may not have the resources to invest in expanding their workforce. Furthermore, the sustainable energy sector faces competition from established industries such as oil and gas, which have a well-established workforce and infrastructure. This competition can make it difficult for the sustainable energy sector to attract workers and establish a foothold in the job market (Sakellariou and Mulvaney, 2013; Sen and Ganguly, 2017; Inês et al., 2020).
In order to attract workers to the sustainable energy sector, businesses can implement a number of strategies to compete with established industries. These strategies include providing competitive salaries and benefits packages that are comparable to those offered by established industries. Companies can also offer training and development opportunities to help workers build the necessary skills and knowledge required to work in the sustainable energy sector. This can include on-the-job training, mentorship programs, and professional development opportunities. Highlighting the impact of the work being done in the sustainable energy sector can also be an effective strategy to attract workers. This emphasizes the importance of creating a sustainable future and reducing the environmental impact of energy production, which can be instrumental in attracting workers who value environmental sustainability. Furthermore, partnering with educational institutions to develop programs that prepare students for careers in the sustainable energy sector can be an effective means of recruiting new talent. This includes providing internships, mentorship programs, and other opportunities for students to gain hands-on experience in the field. Finally, businesses can connect with the community to enhance consciousness of the importance of sustainability and to advocate for the advantages of working in the sustainable energy sector. This includes participating in community events, hosting workshops, and partnering with local organizations to support sustainability initiatives. These strategies can help companies effectively compete with established industries and attract workers who are passionate about creating a sustainable future (Stolten and Scherer, 2013; Surendra et al., 2014; Quitzow et al., 2019).
The shift towards a sustainable energy infrastructure is not without its difficulties. One of the primary hurdles is the considerable initial costs required for constructing sustainable energy infrastructure. Although renewable energy has long-term cost advantages, governments, investors, and businesses must be willing to make substantial financial commitments to develop new sustainable energy initiatives and modernize existing infrastructure. Another critical challenge is the political and social resistance to change (Hu et al., 2021; Luo et al., 2023; Wu et al., 2023; Yi et al., 2023). The fossil fuel industry has a strong presence in many countries, which may resist efforts to transition to renewable energy. Additionally, local communities may be resistant to large-scale renewable energy projects due to concerns about potential impacts such as noise pollution or changes to the landscape. Technical impediments also represent a significant challenge to the adoption of sustainable energy. Aslo, there is a need for more research and development in renewable energy technologies to improve their efficiency, reduce costs, and enhance their scalability. Technological advancements will be critical to the continued growth and adoption of renewable energy (Bose et al., 2019; Pietrosemoli and Rodríguez-Monroy, 2019; Popescu et al., 2022). Figure 2 provides a comprehensive overview of the obstacles and possibilities in the shift towards sustainable energy alternatives. The figure shows the need for investment in renewable energy technologies, policy changes to incentivize sustainable energy use, and the potential for job creation in the sustainable energy sector. It is important to consider these challenges and opportunities when transitioning to sustainable energy sources and to carefully evaluate the costs and benefits of each energy source.
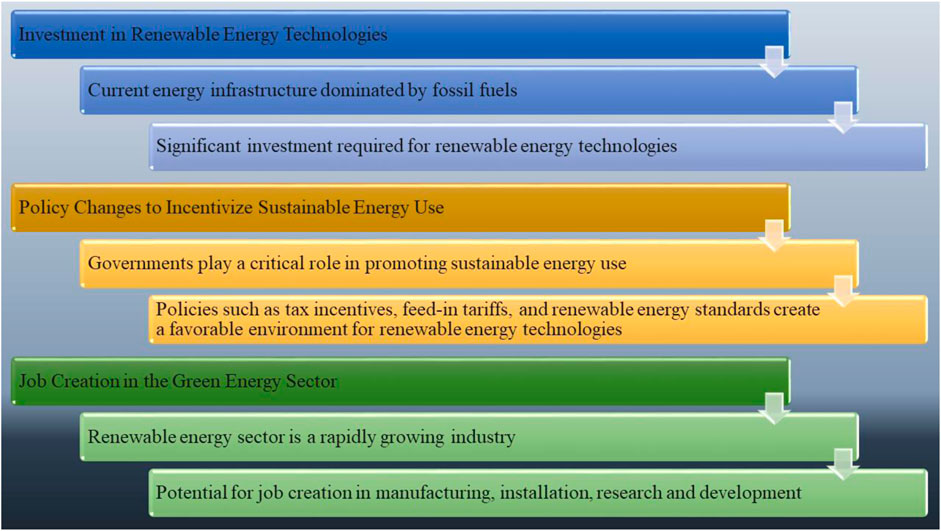
FIGURE 2. Challenges and opportunities in the transition to sustainable energy sources: investment in renewable energy technologies, policy changes to incentivize sustainable energy use, and job creation in the sustainable energy sector.
Table 1 compares the challenges, opportunities, advantages, and disadvantages of transitioning to sustainable energy sources in developed and developing countries (Herzog et al., 2001; Verbruggen et al., 2010; Broman and Robèrt, 2017; Safari et al., 2019; Hoang et al., 2021; Mourtzis et al., 2022; Neacsa et al., 2022; Tian et al., 2022; Usman et al., 2022). Attaining a sustainable and resilient future through sustainable energy alternatives is a global challenge that necessitates cooperation from individuals, businesses, and governments. Although there are similarities in the challenges and opportunities associated with the transition, regional differences must be taken into account. The table highlights the significant differences in challenges, opportunities, advantages, and disadvantages between developed and developing countries. Recognizing these regional disparities is crucial in designing effective policies and strategies to promote sustainable energy solutions and foster a more sustainable and resilient future.
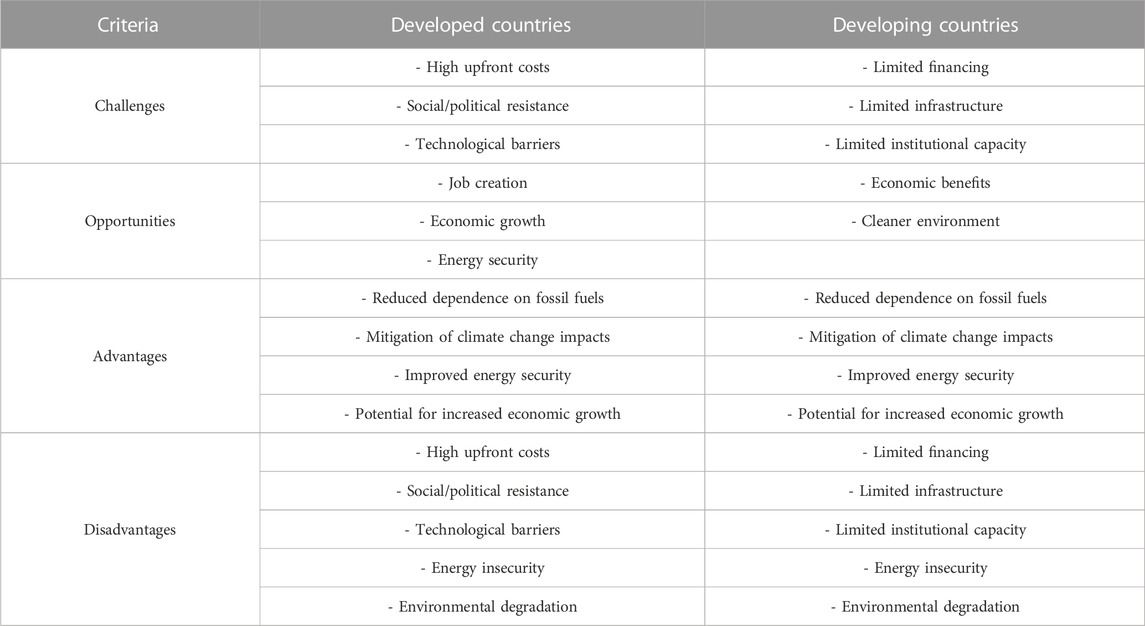
TABLE 1. Comparing challenges, opportunities, advantages, and disadvantages of the transition to sustainable energy sources in developed and developing countries.
In developed countries, the transition towards sustainable energy sources is accompanied by various challenges, including substantial initial investments, resistance from social and political entities, and technological barriers. Despite these obstacles, this transition also offers opportunities for job creation, economic growth, and enhanced energy security. The adoption of sustainable energy alternatives in developed nations yields multiple benefits, such as mitigating the impacts of climate change, reducing dependence on traditional energy sources, and stimulating economic progress. However, this transition is not without drawbacks, as it involves high upfront costs, encounters opposition from social and political forces, and may contribute to environmental degradation. Conversely, developing countries confront distinct challenges as they strive to transition to sustainable energy sources, including limitations in financial resources, inadequate infrastructure, and institutional capacity constraints. Despite these difficulties, transitioning to sustainable energy sources in developing nations also presents opportunities for economic advantages, environmental improvements, and enhanced energy security. However, this process brings disadvantages in the form of limited financing options, insufficient infrastructure, energy insecurity, and potential environmental degradation (Press and Arnould, 2009; Peeters, 2012; Nejad and Kashan, 2019; Majid, 2020; Wang H. et al., 2023).
4.3 Economic benefits of the transition to sustainable energy
The shift towards sustainable energy sources has the potential to yield substantial economic advantages, such as enhanced energy security, decreased reliance on traditional energy sources, and a rise in economic growth. This section will provide a detailed explanation of these benefits. One of the key economic advantages of adopting sustainable energy alternatives is the enhancement of energy security. By diversifying the energy mix and decreasing reliance on a solitary energy source, countries can bolster their energy security and decrease their susceptibility to supply interruptions. Sustainable energy sources like solar and wind power are readily accessible and can be sourced locally, resulting in decreased necessity for transporting energy resources over long distances. This can help to mitigate the risk of supply disruptions due to geopolitical tensions or natural disasters. Another economic benefit of transitioning to sustainable energy is the potential for reduced dependence on fossil fuels. This can improve their energy security and reduce the economic risks associated with fossil fuel dependency. Furthermore, the transition to sustainable energy can spur economic growth. The creation and implementation of renewable energy technologies can generate novel job opportunities and stimulate economic activity in the sustainable energy field. This can result in the emergence of new industries and the expansion of new markets for sustainable energy commodities and services. Additionally, the transition to sustainable energy can lead to cost savings over the long term, as renewable energy sources are generally cheaper than fossil fuels once the initial investment is made (Perelman, 1980; Merven et al., 2019; Siampour et al., 2021; Wang et al., 2022; Wang X. et al., 2023).
The adoption of sustainable energy alternatives is instrumental in reducing the economic costs associated with climate change. The combustion of conventional energy sources emits greenhouse gases, which contribute to global warming and the exacerbation of climate-related impacts, including more frequent and severe weather events. By reducing reliance on traditional energy sources, countries can help mitigate the economic consequences of climate change, such as infrastructure damage, increased insurance premiums, and decreased productivity. Therefore, transitioning to sustainable energy is an essential step towards building a more resilient and sustainable economy. Another notable economic advantage of adopting sustainable energy alternatives is the potential for enhanced energy efficiency. Sustainable energy sources, such as solar and wind power, are intrinsically more efficient than traditional energy sources, as they convert a greater percentage of the energy input into practical energy. This can help to reduce energy waste and lower energy costs. Additionally, the adoption of sustainable energy alternatives can encourage the implementation of energy-efficient technologies, such as energy-efficient appliances and smart meters, which can further decrease energy consumption and expenses. This can result in substantial economic benefits for individuals, businesses, and governments, while also diminishing greenhouse gas emissions (Tirkolaee et al., 2020a; Cantarero, 2020; Liu H. et al., 2022; Wang and Razzaq, 2022).
The adoption of sustainable energy alternatives can have a significant impact in decreasing the economic expenses related to air pollution. Fossil fuels, when burned, release pollutants into the air, contributing to significant health impacts and increased healthcare costs. Through the adoption of sustainable energy alternatives, nations can diminish their dependence on traditional energy sources, resulting in decreased air pollution and associated health expenses. Consequently, this can lead to noteworthy economic benefits for individuals, businesses, and governments. Another significant economic benefit of transitioning to sustainable energy is the potential for increased energy independence. Furthermore, renewable energy sources are abundant and widely available, reducing the need for countries to rely on foreign energy sources and thereby lowering their vulnerability to geopolitical tensions (Van Der Schoor and Scholtens, 2015; Chen J. et al., 2023).
The shift towards sustainable energy alternatives can also yield noteworthy economic advantages for rural communities. Renewable energy can be located in rural areas, creating new economic opportunities and stimulating local economic growth. This can help to create new jobs and generate income for rural communities. Moreover, renewable energy projects can provide a new source of income for farmers and landowners, as they can lease their land for renewable energy projects and receive regular payments. Furthermore, transitioning to sustainable energy can help to attract investment and improve a country’s competitiveness. Investors and businesses are increasingly looking for opportunities to invest in sustainable energy projects, and countries that have a strong commitment to sustainability and renewable energy are likely to be more attractive to these investors. This can lead to increased investment in sustainable energy projects, creating new jobs and economic growth opportunities. Additionally, countries that are investing in sustainable energy are likely to be more competitive in the global economy, as they will have lower energy costs and a more diversified and secure energy mix. This can help to attract new businesses and industries to the country, further stimulating economic growth and job creation (Kemp and Loorbach, 2006; Barbir, 2009; Salam and Khan, 2018; Tirkolaee et al., 2020b).
The relationship between energy and the economy is a complex one with many facets. Energy is a crucial input for economic growth and development. Nevertheless, the manners in which energy is generated, dispersed, and consumed can result in significant effects on both the economy and society. Energy’s impact on the economy is mainly through its role as an input for production. It is used in various economic activities such as manufacturing, transportation, agriculture, and services. The cost and accessibility of energy exert substantial influence on production costs and the competitive edge of industries, particularly energy-intensive sectors like manufacturing. The pivotal role of energy in shaping frameworks for economic growth and progress is of great significance. Historically, access to affordable and abundant energy sources has been a crucial catalyst for economic development, particularly in economies undergoing industrialization. Additionally, the connection between energy and the economy is influenced by political and institutional factors. Energy policy choices, such as subsidies and regulations, can significantly shape the distribution of economic advantages and costs. Moreover, aspects such as vested interests, international trade, and resource allocation can influence the political economy of energy production and consumption. Energy’s role in shaping patterns of social inequality and vulnerability is also important. Access to reliable and affordable energy services is a fundamental aspect of meeting basic human needs such as heating, lighting, and cooking, as well as accessing education, healthcare, and other services. However, patterns of energy access and consumption can be shaped by economic and social inequalities, leading to higher energy costs and exposure to energy-related risks such as energy poverty, energy insecurity, and environmental pollution for low-income households and marginalized communities (Kelly-Richards et al., 2017; Bogdanov et al., 2021; Kabeyi and Olanrewaju, 2022). Technical aspects also have a notable impact on shaping the correlation between energy and the economy. However, the adoption and diffusion of new energy technologies can be influenced by factors such as financing, regulation, and cost-benefit analysis. Therefore, the relationship between energy and the economy is a complex one with many facets, shaped by a range of economic, social, political, institutional, and technological factors. Understanding this relationship is essential for developing effective policies and strategies for achieving sustainable and equitable economic development while addressing the environmental and social challenges associated with energy production and consumption. Further research is needed to deepen our understanding of this complex relationship and identify effective strategies for promoting sustainable energy transitions and building more resilient and inclusive economies (Quitzow et al., 2019; Emna et al., 2022; Neacsa et al., 2022; Zhou et al., 2022; Zhu et al., 2023).
Figure 3 shows an overview of the economic benefits associated with transitioning to sustainable energy. The figure emphasizes the potential for enhanced energy security, decreased reliance on fossil fuels, job creation and economic growth, cost savings and improved energy efficiency, reduced economic costs related to climate change and air pollution, augmented energy independence, economic advantages for rural communities, and improved competitiveness and investment attraction. Each category represents a core aspect in which transitioning to sustainable energy could generate considerable economic benefits, and comprehending these benefits is pivotal in developing effective policies and strategies for achieving sustainable and equitable economic growth while addressing the environmental and social challenges linked with energy production and consumption.
4.4 The role of blockchain technology in the transition towards sustainable energy
The equilibrium of smart grids can be disturbed by changes in consumer behavior. Blockchain technology can provide solutions to integrate new disruptors into the existing industrial structure of the electricity market. By enabling peer-to-peer transactions, blockchain technology can enhance consumer empowerment, but it also poses challenges to the existing regulatory frameworks in the industry (Brilliantova and Thurner, 2019; Jiang S. et al., 2022). Hence, it is crucial to design blockchain technology that addresses trust and regulatory structures in the industry. The electricity market is continuously changing, and blockchain technology offers solutions to integrate new disruptors into the existing industrial structure. Peer-to-peer (P2P) transactions using blockchain technology can increase consumer empowerment and challenge traditional regulatory frameworks in the industry. To address trust and regulatory structures, the text discusses four different approaches to designing blockchain technology for the electricity market: unlicensed, licensed, private, and a combination of both. Each approach has its own unique features and implications for consumer trust and regulatory structures in the industry. Comprehending the necessities of each strategy is crucial for the energy sector to assess the significance of developing blockchain technology for the electricity market. The article emphasizes that technological design is critical in determining the influence of commercial structures on consumer conduct and regulatory frameworks (Liu et al., 2021; Nygaard and Silkoset, 2022).
P2P transactions facilitate the direct exchange of electricity between consumers, eliminating the need for traditional intermediaries such as utility companies. This decentralized trading approach empowers consumers by granting them the authority to determine the price of electricity and enables them to sell any excess energy they generate. This level of control over energy consumption and production represents a significant advantage of P2P transactions. Furthermore, the utilization of blockchain technology enhances transparency and security within the energy market, thereby fostering consumer trust. Transactions are recorded in an immutable ledger, reducing the risk of fraudulent or erroneous transactions and promoting market efficiency. By leveraging blockchain for P2P transactions, consumers are afforded increased empowerment in the electricity market, allowing them to actively participate and make informed decisions regarding their energy consumption and production (Mannaro et al., 2017; Di Silvestre et al., 2020; Afzal et al., 2022; Chen W. et al., 2023).
The potential of blockchain technology lies in its ability to combat the problem of energy poverty in developing nations by boosting access to inexpensive and dependable energy sources. One of the primary hurdles in addressing energy poverty is the dearth of access to established financial systems and infrastructure, which makes it challenging to finance and distribute energy resources to underprivileged communities. Blockchain technology provides a decentralized platform for energy transactions, enabling peer-to-peer energy trading and facilitating the distribution of energy resources to underserved communities. Blockchain-based energy platforms can also address trust and transparency issues in the energy sector. By utilizing a tamper-proof and transparent ledger system, blockchain technology increases accountability and reduces the risk of fraud and corruption in energy transactions. This helps to establish trust between energy producers, distributors, and consumers, creating a more efficient and equitable energy market. Furthermore, blockchain technology can facilitate the integration of sustainable energy sources into the energy grid, which is critical in developing nations where sustainable energy sources like wind or solar may be more accessible and economical than conventional fossil fuel-based energy sources (Enescu et al., 2020; Mukherjee et al., 2021; Almutairi et al., 2022; Govindan, 2022).
The implementation of blockchain-based energy platforms in developing countries is accompanied by various challenges that need to be addressed. The first challenge pertains to limited infrastructure, which includes insufficient access to reliable electricity, inadequate internet connectivity, and a lack of necessary hardware to support blockchain-based energy platforms. These infrastructure limitations can impede the deployment and maintenance of blockchain technology in developing countries. The second challenge revolves around the scarcity of technical expertise required for the successful implementation of blockchain technology. Proficiency in areas such as software development, cryptography, and cybersecurity is vital for the development, deployment, and upkeep of blockchain-based energy platforms. The limited availability of technical expertise in these domains poses difficulties in incorporating blockchain technology in developing countries. The third challenge involves the absence of a comprehensive regulatory framework that supports the deployment of blockchain technology in developing countries. The lack of regulatory guidelines creates ambiguity, heightening the risk of non-compliance, deterring investment, and impeding the growth of blockchain-based energy platforms. The fourth challenge concerns the economic viability of blockchain-based energy platforms in developing countries. Due to low levels of energy consumption and limited access to financing, attracting investments and achieving the economies of scale necessary for the financial sustainability of blockchain-based energy platforms can be challenging. Lastly, social and cultural factors play a significant role in the adoption of blockchain-based energy platforms in developing countries. Some communities may exhibit skepticism towards new technologies or have a preference for traditional energy sources, thereby creating obstacles to the widespread adoption of blockchain-based energy platforms. Addressing these challenges is crucial to ensure the successful implementation of blockchain-based energy platforms in developing countries. By overcoming these obstacles, the potential benefits of blockchain technology in enhancing energy access, efficiency, and transparency can be harnessed to support sustainable development and address energy challenges in these regions (Giungato et al., 2017; Truby, 2018; Aybar-Mejía et al., 2021; Popkova et al., 2023).
The licensed approach to designing blockchain technology for the electricity market involves the use of licensed and regulated intermediaries to facilitate transactions between producers and consumers. This approach aims to provide a higher level of trust and security than unlicensed approaches, while still enabling the benefits of blockchain technology. In the licensed approach, licensed intermediaries act as trusted third parties to validate transactions and ensure compliance with regulatory requirements. These intermediaries are usually regulated by government agencies and must adhere to specific standards for security, transparency, and integrity. Additionally, they may be required to maintain records of transactions and provide reports to regulators. The primary advantage of the licensed approach is the higher level of trust and security it provides compared to unlicensed approaches. By utilizing licensed intermediaries, consumers can have greater confidence in the integrity of transactions and the regulatory compliance of market participants. This can help to reduce the risk of fraudulent and illegal activities in the electricity market. However, the licensed approach has some drawbacks. For instance, the use of licensed intermediaries can increase transaction costs and reduce market efficiency. Furthermore, the regulatory requirements for licensed intermediaries can be complex and may vary across different jurisdictions, making it challenging to implement a standardized approach to blockchain-based energy platforms (Svetec et al., 2019; Yildizbasi, 2021; Lei et al., 2022).
Thus, blockchain technology holds the potential to make a substantial impact in promoting a sustainable energy future by augmenting the efficiency and transparency of energy markets and streamlining the incorporation of sustainable energy sources. The technology can aid in addressing some of the primary challenges confronting the worldwide energy sector, such as energy security, climate change, and sustainable growth. As mentioned, one of the ways that blockchain technology can enhance the efficiency and transparency of energy markets is through peer-to-peer energy trading. This allows consumers to determine electricity prices and sell any excess energy they generate, giving them greater control over their energy consumption and production. The transparency and security afforded by blockchain technology can also increase consumer trust in the market, as transactions are recorded in a tamper-proof ledger, reducing the risk of fraudulent or inaccurate transactions and increasing market efficiency. Blockchain technology can also contribute to promoting a sustainable energy future by simplifying the integration of sustainable energy sources into the energy grid. The technology can aid in addressing the obstacles presented by the sporadic nature of sustainable energy sources and the insufficiency of energy storage capacity. Decentralized energy systems can be built on blockchain-based energy platforms that allow for the seamless integration of sustainable energy sources. These platforms can streamline the effective administration and synchronization of energy resources, enabling the maximization of energy production and consumption. For example, blockchain-based energy platforms can be used to create virtual power plants that aggregate sustainable energy sources and use energy storage systems to smooth out fluctuations in energy supply and demand. Lastly, blockchain technology can have a pivotal function in promoting sustainable development by enhancing access to energy resources in underprivileged communities. Blockchain-based energy platforms provide a decentralized platform for energy transactions, enabling P2P energy trading and facilitating the distribution of energy resources to underserved communities. This is particularly beneficial in developing countries where traditional energy infrastructure may be lacking or unreliable (Sweeney et al., 2020; Ante et al., 2021; Wünsche and Fernqvist, 2022; Mao et al., 2023).
The application of blockchain technology in the energy industry could face various potential challenges or limitations. Scalability is a major challenge, where an increase in the number of transactions on a blockchain can lead to slower transaction times and higher transaction fees, making blockchain-based energy platforms less efficient and less cost-effective than traditional energy systems. Another challenge is the technical complexity of blockchain technology, which necessitates specialized technical expertise to develop and maintain, making it difficult for energy companies and regulators to adopt and implement blockchain-based energy platforms. Interoperability is another issue of concern, as there are presently numerous distinct blockchain platforms, each with its distinct features and stipulations, making the consolidation of various blockchain-based energy platforms with one another and with conventional energy systems challenging. Furthermore, the highly regulated nature of the energy sector can create regulatory challenges that require new or updated regulations to ensure compliance with existing laws and regulations, leading to uncertainty and delaying the adoption of blockchain-based energy platforms. The security and privacy of user data are also significant concerns, and any breach of this data could result in significant risks for both consumers and market participants. Furthermore, there could be apprehensions about the storage and dissemination of confidential energy data on a public blockchain network. Furthermore, while blockchain technology can promote energy efficiency in certain contexts, it also requires significant energy consumption, particularly with proof-of-work consensus mechanisms, creating concerns about the environmental sustainability of blockchain-based energy platforms. In conclusion, the implementation of blockchain technology in the energy sector requires careful consideration of potential challenges and drawbacks, including scalability, technical complexity, interoperability, regulatory challenges, data privacy and security, and energy consumption. Addressing these challenges will require collaboration between stakeholders across different sectors, such as governments, energy companies, technology providers, and regulators (Wang and Su, 2020; Wang et al., 2021; Juszczyk and Shahzad, 2022).
Policy frameworks play a pivotal role in supporting the implementation of energy systems based on blockchain technology. The successful integration of blockchain platforms into the energy sector necessitates the establishment of specific policy measures and regulatory frameworks. Standards aimed at ensuring interoperability between blockchain platforms and existing energy infrastructure are crucial for facilitating seamless integration and optimizing the exchange of data. Regulations governing peer-to-peer energy trading, smart contracts, and the protection of customer data are vital for ensuring equitable and transparent transactions while safeguarding individual privacy. By offering incentives such as tax credits, governments can stimulate investments in blockchain energy projects, thereby fostering innovation and encouraging the widespread adoption of this technology. The integration of renewable energy certificates and carbon trading systems with blockchain platforms enhances the transparency and accountability of renewable energy markets. Policies that support decentralized energy production, smart grid infrastructure, and net metering are instrumental in effectively integrating distributed energy resources into the existing energy landscape. Additionally, the establishment of blockchain sandboxes provides controlled testing environments where policymakers can evaluate the feasibility and impact of new regulatory frameworks. By implementing these comprehensive policy frameworks, governments can foster an enabling environment for the successful deployment of blockchain-based energy systems, thereby promoting transparency, efficiency, and sustainability within the energy sector.
Figure 4 depicts the primary advantages and potential applications of blockchain technology in the energy sector. The first category, P2P Energy Trading, elucidates how blockchain technology can empower consumers, enhance transparency, and augment security in the energy market. The second category, Integration of Renewable Energy Sources, illustrates how blockchain technology can enable the seamless integration of renewable energy sources, energy storage systems, and smart grid technologies. The third category, Addressing Energy Poverty, explores how blockchain technology can offer a decentralized platform for energy transactions, enabling peer-to-peer energy trading and facilitating the distribution of energy resources to underserved communities. Finally, the fourth category, Challenges and Potential Drawbacks, highlights the primary challenges and potential drawbacks associated with implementing blockchain technology in the energy sector, such as scalability, technical complexity, regulatory, data privacy and security, and environmental sustainability concerns. Comprehending these advantages and challenges is imperative in determining the significance of designing blockchain technology for the electricity market and promoting a sustainable energy future.
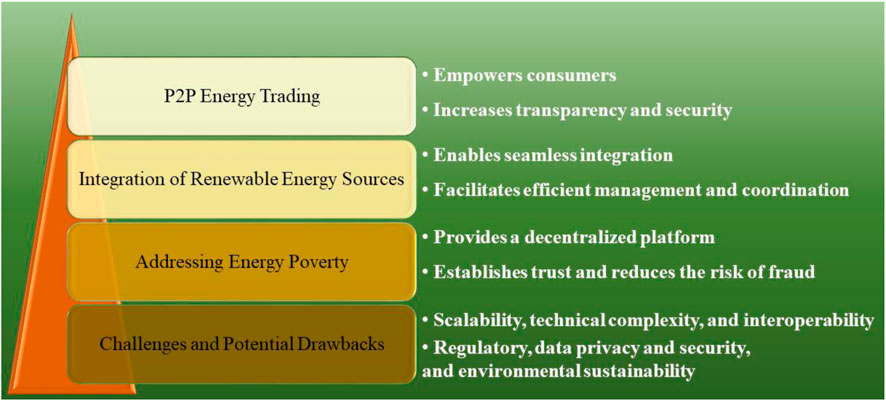
FIGURE 4. Potential benefits and challenges of implementing blockchain technology in the energy sector.
5 Conclusion
A qualitative research methodology was used in this study, which involved an extensive literature review and content analysis, enabling an in-depth exploration of the research topic and addressing the study objectives. The objective of the study was to comprehensively investigate the challenges, opportunities, and role of blockchain technology in facilitating the transition towards sustainable energy sources. The transition towards sustainable energy is essential for environmental and economic reasons, as it reduces dependence on fossil fuels, mitigates the impacts of climate change, and promotes economic growth. Thus, the findings from this study can inform policy decisions and future research to promote sustainable energy solutions.
Several challenges were identified in the transition to sustainable energy, including high upfront costs, social and political resistance, and technological barriers. However, opportunities such as job creation, economic benefits, and a cleaner environment also exist. Blockchain technology has the potential to enable more efficient and transparent energy markets through peer-to-peer transactions and a distributed ledger. It can also facilitate the integration of renewable energy sources. However, addressing challenges such as scalability, technical complexity, and security risks is necessary for blockchain technology to effectively contribute to a sustainable energy transition. Policy changes that incentivize sustainable energy use and investment in renewable energy technologies are also crucial enablers.
The transition to sustainable energy sources offers promising economic prospects, including improved energy security, reduced dependence on imports, and potential for increased economic growth. However, achieving sustainable development requires policies that balance energy supply, economic growth, and environmental protection. The sustainable energy sector faces the challenge of attracting and developing a skilled workforce to meet growing demand. Educational institutions have an important role to play in bridging the skills gap and preparing students for careers in sustainable energy.
Also, addressing the skills gaps and implementing effective training strategies is crucial for building a proficient workforce in the renewable energy sector. The identified skills gaps encompass technical proficiencies, understanding of renewable energy technologies, engineering expertise, data analytics skills, and knowledge of regulations and policy frameworks. Educational institutions play a vital role in developing these skills through targeted sustainable energy programs, cross-disciplinary courses, apprenticeships, and vocational training. Integrating renewable energy and blockchain topics into mainstream engineering and business curriculums ensures that graduates are well-equipped for the evolving energy landscape. Reskilling programs are also essential for enabling a smooth transition for individuals from fossil fuel-related occupations to renewable energy careers. By addressing these skills gaps and implementing comprehensive training strategies, stakeholders can foster a competent workforce capable of driving the successful implementation of renewable energy technologies and the integration of blockchain platforms. This, in turn, will contribute to the advancement of sustainable energy systems and the achievement of global climate goals.
Looking towards the future, there are several promising trends that have the potential to accelerate the transition towards sustainable energy systems. Advancements in renewable energy technologies, coupled with declining costs, are expected to drive their widespread adoption on a global scale. Policy frameworks at various levels of governance have the capacity to evolve progressively, becoming more supportive of sustainability initiatives, as public concern over climate change continues to grow. The emergence of potentially disruptive technologies such as blockchain, artificial intelligence, and advanced data analytics holds promise for unlocking new capabilities and business models within the energy sector. Furthermore, the green energy workforce is anticipated to experience significant expansion, supported by targeted training initiatives offered by educational institutions and industry partners. Innovative financing mechanisms can play a pivotal role in making the economics of sustainability more viable, while community-based approaches offer creative solutions to overcome local resistance. By comprehensively capitalizing on these opportunities, a future can be envisioned where affordable, decentralized, and clean energy empowers societies worldwide. To translate these promising trends into tangible reality, further rigorous interdisciplinary research and enhanced collaboration among diverse stakeholders will be crucial.
In conclusion, transitioning to sustainable energy sources is crucial for environmental and economic sustainability but faces significant challenges that require concerted efforts from governments, companies, and educational institutions. The utilization of promising technologies such as blockchain and policy changes incentivizing renewable energy can help enable a sustainable energy transition. This transition offers promising economic benefits and prospects for job creation in the sustainable energy sector. However, addressing the skills gap through targeted training programs and continued education is critical to realize the full potential of the sustainable energy transition. The insights from this study can inform policies and strategies to promote sustainable energy solutions and build a more sustainable and resilient economy.
Data availability statement
The raw data supporting the conclusion of this article will be made available by the authors, without undue reservation.
Author contributions
YL: Conceptualization, Data curation, Formal Analysis, Funding acquisition, Investigation, Methodology, Project administration, Resources, Supervision, Validation, Visualization, Writing—original draft, Writing—review and editing.
Funding
The author(s) declare that no financial support was received for the research, authorship, and/or publication of this article.
Acknowledgments
The authors would like to express their sincere appreciation to all those who provided valuable support and assistance during the research process.
Conflict of interest
The authors declare that the research was conducted in the absence of any commercial or financial relationships that could be construed as a potential conflict of interest.
Publisher’s note
All claims expressed in this article are solely those of the authors and do not necessarily represent those of their affiliated organizations, or those of the publisher, the editors and the reviewers. Any product that may be evaluated in this article, or claim that may be made by its manufacturer, is not guaranteed or endorsed by the publisher.
References
Abban, O. J., Hongxing, Y., Nuta, A. C., Dankyi, A. B., Ofori, C., and Cobbinah, J. (2022). Renewable energy, economic growth, and CO2 emissions contained Co-movement in african oil-producing countries: A wavelet based analysis. Energy Strategy Rev. 44, 100977. doi:10.1016/j.esr.2022.100977
Abbasi, T., and Abbasi, S. (2011). Small hydro and the environmental implications of its extensive utilization. Renew. Sustain. energy Rev. 15, 2134–2143. doi:10.1016/j.rser.2010.11.050
Adamowicz, M. (2022). Green deal, green growth and green economy as a means of support for attaining the sustainable development goals. Sustainability 14, 5901. doi:10.3390/su14105901
Afzal, M., Li, J., Amin, W., Huang, Q., Umer, K., Ahmad, S. A., et al. (2022). Role of blockchain technology in transactive energy market: A review. Sustain. Energy Technol. Assessments 53, 102646. doi:10.1016/j.seta.2022.102646
Ahl, A., Yarime, M., Goto, M., Chopra, S. S., Kumar, N. M., Tanaka, K., et al. (2020). Exploring blockchain for the energy transition: opportunities and challenges based on a case study in Japan. Renew. Sustain. energy Rev. 117, 109488. doi:10.1016/j.rser.2019.109488
Akram, S. V., Malik, P. K., Singh, R., Anita, G., and Tanwar, S. (2020). Adoption of blockchain technology in various realms: opportunities and challenges. Secur. Priv. 3, e109. doi:10.1002/spy2.109
Al-Housani, M. I., Koç, M., and Al-Sada, M. S. (2023). Investigations on entrepreneurship needs, challenges, and models for countries in transition to sustainable development from resource-based economy—Qatar as a case. Sustainability 15, 7537. doi:10.3390/su15097537
Al-Shetwi, A. Q. (2022). Sustainable development of renewable energy integrated power sector: trends, environmental impacts, and recent challenges. Sci. Total Environ. 822, 153645. doi:10.1016/j.scitotenv.2022.153645
Almutairi, K., Hosseini Dehshiri, S. J., Hosseini Dehshiri, S. S., Hoa, A. X., Arockia Dhanraj, J., Mostafaeipour, A., et al. (2022). Blockchain Technology application challenges in renewable energy supply chain management. Environ. Sci. Pollut. Res. 30, 72041–72058. doi:10.1007/s11356-021-18311-7
Amin, M., Shah, H. H., Fareed, A. G., Khan, W. U., Chung, E., Zia, A., et al. (2022). Hydrogen production through renewable and non-renewable energy processes and their impact on climate change. Int. J. hydrogen energy 47, 33112–33134. doi:10.1016/j.ijhydene.2022.07.172
Andoni, M., Robu, V., Flynn, D., Abram, S., Geach, D., Jenkins, D., et al. (2019). Blockchain technology in the energy sector: A systematic review of challenges and opportunities. Renew. Sustain. energy Rev. 100, 143–174. doi:10.1016/j.rser.2018.10.014
Ansari, M. A., Akram, V., and Haider, S. (2022). A link between productivity, globalisation and carbon emissions: evidence from emissions by coal, oil and gas. Environ. Sci. Pollut. Res. 29, 33826–33843. doi:10.1007/s11356-022-18557-9
Ante, L., Steinmetz, F., and Fiedler, I. (2021). Blockchain and energy: A bibliometric analysis and review. Renew. Sustain. Energy Rev. 137, 110597. doi:10.1016/j.rser.2020.110597
Arabian, M., Ghadiri Nejad, M., and Barenji, R. V. (2022). Blockchain technology in supply chain management: Challenge and future perspectives, industry 4.0: Technologies, applications, and challenges. Springer, 201–220.
Armaroli, N., and Balzani, V. (2007). The future of energy supply: challenges and opportunities. Angew. Chem. Int. Ed. 46, 52–66. doi:10.1002/anie.200602373
Asif, M., Salman, M. U., Anwar, S., Gul, M., and Aslam, R. (2022). Renewable and non-renewable energy resources of Pakistan and their applicability under the current scenario in Pakistan. OPEC Energy Rev. 46, 310–339. doi:10.1111/opec.12230
Aybar-Mejía, M., Rosario-Weeks, D., Mariano-Hernández, D., and Domínguez-Garabitos, M. (2021). An approach for applying blockchain technology in centralized electricity markets. Electr. J. 34, 106918. doi:10.1016/j.tej.2021.106918
Bahlouli, K., Lotfi, N., and Ghadiri Nejad, M. (2023). A new multi-heuristic method to optimize the ammonia–water power/cooling cycle combined with an HCCI engine. Sustainability 15, 6545. doi:10.3390/su15086545
Barbir, F. (2009). Transition to renewable energy systems with hydrogen as an energy carrier. Energy 34, 308–312. doi:10.1016/j.energy.2008.07.007
Barenji, R. V., and Nejad, M. G. (2022). Intelligent and fuzzy techniques in aviation 4.0. Theory and Applications, 411–430.Blockchain applications in UAV-towards aviation 4.0
Başkaya, Ş., Başkaya, E., and Sari, A. (2011). The principal negative environmental impacts of small hydropower plants in Turkey. Afr. J. Agric. Res. 6, 3284–3290.
Bayulgen, O. (2020). Localizing the energy transition: town-level political and socio-economic drivers of clean energy in the United States. Energy Res. Soc. Sci. 62, 101376. doi:10.1016/j.erss.2019.101376
Bogdanov, D., Ram, M., Aghahosseini, A., Gulagi, A., Oyewo, A. S., Child, M., et al. (2021). Low-cost renewable electricity as the key driver of the global energy transition towards sustainability. Energy 227, 120467. doi:10.1016/j.energy.2021.120467
Bose, S., Dong, G., Simpson, A., Bose, S., Dong, G., and Simpson, A. (2019). Financing clean technology innovation and the transition to renewable energy. Financial Ecosyst. Role Finance Achiev. Sustain., 339–368.
Brilliantova, V., and Thurner, T. W. (2019). Blockchain and the future of energy. Technol. Soc. 57, 38–45. doi:10.1016/j.techsoc.2018.11.001
Broman, G. I., and Robèrt, K.-H. (2017). A framework for strategic sustainable development. J. Clean. Prod. 140, 17–31. doi:10.1016/j.jclepro.2015.10.121
Bulavskaya, T., and Reynès, F. (2018). Job creation and economic impact of renewable energy in The Netherlands. Renew. Energy 119, 528–538. doi:10.1016/j.renene.2017.09.039
Cai, T., Dong, M., Chen, K., and Gong, T. (2022). Methods of participating power spot market bidding and settlement for renewable energy systems. Energy Rep. 8, 7764–7772. doi:10.1016/j.egyr.2022.05.291
Cantarero, M. M. V. (2020). Of renewable energy, energy democracy, and sustainable development: A roadmap to accelerate the energy transition in developing countries. Energy Res. Soc. Sci. 70, 101716. doi:10.1016/j.erss.2020.101716
Chai, Q., and Zhang, X. (2010). Technologies and policies for the transition to a sustainable energy system in China. Energy 35, 3995–4002. doi:10.1016/j.energy.2010.04.033
Chen, J., Huang, S., and Kamran, H. W. (2023a). Empowering sustainability practices through energy transition for sustainable development goal 7: the role of energy patents and natural resources among European union economies through advanced panel. Energy Policy 176, 113499. doi:10.1016/j.enpol.2023.113499
Chen, W., Zou, W., Zhong, K., and Aliyeva, A. (2023b). Machine learning assessment under the development of green technology innovation: A perspective of energy transition. Renew. Energy 214, 65–73. doi:10.1016/j.renene.2023.05.108
Chen, Y. (2022). Research on collaborative innovation of key common technologies in new energy vehicle industry based on digital twin technology. Energy Rep. 8, 15399–15407. doi:10.1016/j.egyr.2022.11.120
Chenic, A. Ș., Cretu, A. I., Burlacu, A., Moroianu, N., Vîrjan, D., Huru, D., et al. (2022). Logical analysis on the strategy for a sustainable transition of the world to green energy—2050. Smart cities and villages coupled to renewable energy sources with low carbon footprint. Sustainability 14, 8622. doi:10.3390/su14148622
Chien, F. (2022). How renewable energy and non-renewable energy affect environmental excellence in N-11 economies? Renew. Energy 196, 526–534. doi:10.1016/j.renene.2022.07.013
Chu, S., and Majumdar, A. (2012). Opportunities and challenges for a sustainable energy future. nature 488, 294–303. doi:10.1038/nature11475
Dhar, A., Naeth, M. A., Jennings, P. D., and El-Din, M. G. (2020). Perspectives on environmental impacts and a land reclamation strategy for solar and wind energy systems. Sci. total Environ. 718, 134602. doi:10.1016/j.scitotenv.2019.134602
Di Silvestre, M. L., Gallo, P., Guerrero, J. M., Musca, R., Sanseverino, E. R., Sciumè, G., et al. (2020). Blockchain for power systems: current trends and future applications. Renew. Sustain. Energy Rev. 119, 109585. doi:10.1016/j.rser.2019.109585
Di Somma, M., and Graditi, G. (2022). Challenges and opportunities of the energy transition and the added value of energy systems integration. Technol. Integr. energy Syst. Netw., 1–14. doi:10.1002/9783527833634.ch1
Dominković, D. F., Bačeković, I., Pedersen, A. S., and Krajačić, G. (2018). The future of transportation in sustainable energy systems: opportunities and barriers in a clean energy transition. Renew. Sustain. Energy Rev. 82, 1823–1838. doi:10.1016/j.rser.2017.06.117
Dong, F., Li, Y., Gao, Y., Zhu, J., Qin, C., and Zhang, X. (2022). Energy transition and carbon neutrality: exploring the non-linear impact of renewable energy development on carbon emission efficiency in developed countries. Resour. Conservation Recycl. 177, 106002. doi:10.1016/j.resconrec.2021.106002
Dwivedi, A., Agrawal, D., Jha, A., and Mathiyazhagan, K. (2023). Studying the interactions among industry 5.0 and circular supply chain: towards attaining sustainable development. Comput. Industrial Eng. 176, 108927. doi:10.1016/j.cie.2022.108927
Egré, D., and Milewski, J. C. (2002). The diversity of hydropower projects. Energy policy 30, 1225–1230. doi:10.1016/s0301-4215(02)00083-6
Emna, O., Chtourou, N., and Bazin, D. (2022). Technological, economic, institutional, and psychosocial aspects of the transition to renewable energies: A critical literature review of a multidimensional process. Renew. Energy Focus 43, 37–49. doi:10.1016/j.ref.2022.08.004
Enescu, F. M., Bizon, N., Onu, A., Răboacă, M. S., Thounthong, P., Mazare, A. G., et al. (2020). Implementing blockchain technology in irrigation systems that integrate photovoltaic energy generation systems. Sustainability 12, 1540. doi:10.3390/su12041540
Erat, S., Telli, A., Ozkendir, O. M., and Demir, B. (2021). Turkey’s energy transition from fossil-based to renewable up to 2030: milestones, challenges and opportunities. Clean Technol. Environ. Policy 23, 401–412. doi:10.1007/s10098-020-01949-1
Etheridge, D. (2000). Unsteady flow effects due to fluctuating wind pressures in natural ventilation design—Mean flow rates. Build. Environ. 35, 111–133. doi:10.1016/s0360-1323(99)00007-4
Fang, J., Gozgor, G., Mahalik, M. K., Mallick, H., and Padhan, H. (2022). Does urbanisation induce renewable energy consumption in emerging economies? The role of education in energy switching policies. Energy Econ. 111, 106081. doi:10.1016/j.eneco.2022.106081
Farghali, M., Osman, A. I., Mohamed, I. M., Chen, Z., Chen, L., Ihara, I., et al. (2023). Strategies to save energy in the context of the energy crisis: A review. Environ. Chem. Lett. 21, 2003–2039. doi:10.1007/s10311-023-01591-5
Gawusu, S., Zhang, X., Ahmed, A., Jamatutu, S. A., Miensah, E. D., Amadu, A. A., et al. (2022). Renewable energy sources from the perspective of blockchain integration: from theory to application. Sustain. Energy Technol. Assessments 52, 102108. doi:10.1016/j.seta.2022.102108
Ghasemi, M., Nejad, M. G., and Aghaei, I. (2021). Knowledge management orientation and operational performance relationship in medical tourism (overview of the model performance in the COVID-19 pandemic and post-pandemic era). Health Serv. Manag. Res. 34, 208–222. doi:10.1177/0951484820971438
Ghasemi, M., Nejad, M. G., Alsaadi, N., Abdel-Jaber, M. t., Ab Yajid, M. S., and Habib, M. (2022). Performance measurment and lead-time reduction in epc project-based organizations: A mathematical modeling approach. Math. Problems Eng. 2022, 1–15. doi:10.1155/2022/5767356
Gielen, D., Boshell, F., Saygin, D., Bazilian, M. D., Wagner, N., and Gorini, R. (2019). The role of renewable energy in the global energy transformation. Energy strategy Rev. 24, 38–50. doi:10.1016/j.esr.2019.01.006
Gielen, D., Boshell, F., and Saygin, D. (2016). Climate and energy challenges for materials science. Nat. Mater. 15, 117–120. doi:10.1038/nmat4545
Giungato, P., Rana, R., Tarabella, A., and Tricase, C. (2017). Current trends in sustainability of bitcoins and related blockchain technology. Sustainability 9, 2214. doi:10.3390/su9122214
Gleick, P. H. (1992). Environmental consequences of hydroelectric development: the role of facility size and type. Energy 17, 735–747. doi:10.1016/0360-5442(92)90116-h
Goli, A. (2023). Integration of blockchain-enabled closed-loop supply chain and robust product portfolio design. Comput. Industrial Eng. 179, 109211. doi:10.1016/j.cie.2023.109211
Govindan, K. (2022). Tunneling the barriers of blockchain technology in remanufacturing for achieving sustainable development goals: A circular manufacturing perspective. Bus. Strategy Environ. 31, 3769–3785. doi:10.1002/bse.3031
Guo, B., Feng, Y., and Hu, F. (2023a). Have carbon emission trading pilot policy improved urban innovation capacity? Evidence from a quasi-natural experiment in China. Environ. Sci. Pollut. Res., 1–14. doi:10.1007/s11356-023-25699-x
Guo, L., Nowzari, R., Saygin, H., Al-Bahrani, M., Nejad, M. G., and Baghaei, S. (2023b). Experimental investigation and two-factor factorial analysis of a solar air heater with scrap wire meshes and cans as energy storage components. J. Energy Storage 70, 107998. doi:10.1016/j.est.2023.107998
Hassan, S. S., Williams, G. A., and Jaiswal, A. K. (2019). Moving towards the second generation of lignocellulosic biorefineries in the EU: drivers, challenges, and opportunities. Renew. Sustain. Energy Rev. 101, 590–599. doi:10.1016/j.rser.2018.11.041
Hennig, T., Wang, W., Feng, Y., Ou, X., and He, D. (2013). Review of Yunnan's hydropower development. Comparing small and large hydropower projects regarding their environmental implications and socio-economic consequences. Renew. Sustain. Energy Rev. 27, 585–595. doi:10.1016/j.rser.2013.07.023
Hernandez, R. R., Easter, S., Murphy-Mariscal, M. L., Maestre, F. T., Tavassoli, M., Allen, E. B., et al. (2014). Environmental impacts of utility-scale solar energy. Renew. Sustain. energy Rev. 29, 766–779. doi:10.1016/j.rser.2013.08.041
Herzog, A. V., Lipman, T. E., and Kammen, D. M. (2001). Renewable energy sources, Encyclopedia of life support systems (EOLSS). Forerunn. Volume-‘Perspectives Overv. life support Syst. Sustain. Dev., 76.
Hoang, A. T., Nižetić, S., Olcer, A. I., Ong, H. C., Chen, W.-H., Chong, C. T., et al. (2021). Impacts of COVID-19 pandemic on the global energy system and the shift progress to renewable energy: opportunities, challenges, and policy implications. Energy Policy 154, 112322. doi:10.1016/j.enpol.2021.112322
Holechek, J. L., Geli, H. M., Sawalhah, M. N., and Valdez, R. (2022). A global assessment: can renewable energy replace fossil fuels by 2050? Sustainability 14, 4792. doi:10.3390/su14084792
Hosenuzzaman, M., Rahim, N. A., Selvaraj, J., Hasanuzzaman, M., Malek, A. A., and Nahar, A. (2015). Global prospects, progress, policies, and environmental impact of solar photovoltaic power generation. Renew. Sustain. energy Rev. 41, 284–297. doi:10.1016/j.rser.2014.08.046
Hu, F., Xi, X., and Zhang, Y. (2021). Influencing mechanism of reverse knowledge spillover on investment enterprises’ technological progress: an empirical examination of Chinese firms. Technol. Forecast. Soc. Change 169, 120797. doi:10.1016/j.techfore.2021.120797
Ibeanu, C., Ghadiri Nejad, M., and Ghasemi, M. (2023). Developing effective project management strategy for urban flood disaster prevention project in EDO state capital, Nigeria. Urban Sci. 7, 37. doi:10.3390/urbansci7020037
Inês, C., Guilherme, P. L., Esther, M.-G., Swantje, G., Stephen, H., and Lars, H. (2020). Regulatory challenges and opportunities for collective renewable energy prosumers in the EU. Energy Policy 138, 111212. doi:10.1016/j.enpol.2019.111212
Ishaq, H., Dincer, I., and Crawford, C. (2022). A review on hydrogen production and utilization: challenges and opportunities. Int. J. Hydrogen Energy 47, 26238–26264. doi:10.1016/j.ijhydene.2021.11.149
Islam, M. M., Irfan, M., Shahbaz, M., and Vo, X. V. (2022). Renewable and non-renewable energy consumption in Bangladesh: the relative influencing profiles of economic factors, urbanization, physical infrastructure and institutional quality. Renew. Energy 184, 1130–1149. doi:10.1016/j.renene.2021.12.020
Jaber, S. (2013). Environmental impacts of wind energy. J. Clean Energy Technol. 1, 251–254. doi:10.7763/jocet.2013.v1.57
Japir Bataineh, M. A., Ghasemi, M., and Ghadiri Nejad, M. (2023). The role of green training in the ministry of education’s corporate environmental performance: A mediation analysis of organizational citizenship behavior towards the environment and moderation role of perceived organizational support. Sustainability 15, 8398. doi:10.3390/su15108398
Jenniches, S. (2018). Assessing the regional economic impacts of renewable energy sources–A literature review. Renew. Sustain. Energy Rev. 93, 35–51. doi:10.1016/j.rser.2018.05.008
Jiang, J., Zhang, L., Wen, X., Valipour, E., and Nojavan, S. (2022a). Risk-based performance of power-to-gas storage technology integrated with energy hub system regarding downside risk constrained approach. Int. J. Hydrogen Energy 47, 39429–39442. doi:10.1016/j.ijhydene.2022.09.115
Jiang, S., Jakobsen, K., Bueie, J., Li, J., and Haro, P. H. (2022b). A tertiary review on blockchain and sustainability with focus on Sustainable Development Goals. IEEE access 10, 114975–115006. doi:10.1109/access.2022.3217683
Jie, H., Khan, I., Alharthi, M., Zafar, M. W., and Saeed, A. (2023). Sustainable energy policy, socio-economic development, and ecological footprint: the economic significance of natural resources, population growth, and industrial development. Util. Policy 81, 101490. doi:10.1016/j.jup.2023.101490
Jumani, S., Rao, S., Machado, S., and Prakash, A. (2017). Big concerns with small projects: evaluating the socio-ecological impacts of small hydropower projects in India. Ambio 46, 500–511. doi:10.1007/s13280-016-0855-9
Juszczyk, O., and Shahzad, K. (2022). Blockchain technology for renewable energy: principles, applications and prospects. Energies 15, 4603. doi:10.3390/en15134603
Kabeyi, M. J. B., and Olanrewaju, O. A. (2022). Sustainable energy transition for renewable and low carbon grid electricity generation and supply. Front. Energy Res. 9, 1032. doi:10.3389/fenrg.2021.743114
Kanwal, S., Mehran, M. T., Hassan, M., Anwar, M., Naqvi, S. R., and Khoja, A. H. (2022). An integrated future approach for the energy security of Pakistan: replacement of fossil fuels with syngas for better environment and socio-economic development. Renew. Sustain. Energy Rev. 156, 111978. doi:10.1016/j.rser.2021.111978
Kelly-Richards, S., Silber-Coats, N., Crootof, A., Tecklin, D., and Bauer, C. (2017). Governing the transition to renewable energy: A review of impacts and policy issues in the small hydropower boom. Energy Policy 101, 251–264. doi:10.1016/j.enpol.2016.11.035
Kern, F., and Smith, A. (2008). Restructuring energy systems for sustainability? Energy transition policy in The Netherlands. Energy policy 36, 4093–4103. doi:10.1016/j.enpol.2008.06.018
Kocak, E., Ulug, E. E., and Oralhan, B. (2023). The impact of electricity from renewable and non-renewable sources on energy poverty and greenhouse gas emissions (GHGs): empirical evidence and policy implications. Energy 272, 127125. doi:10.1016/j.energy.2023.127125
Kumar, N. M., Chopra, S. S., and Rajput, P. (2020). Life cycle assessment and environmental impacts of solar PV systems, Photovoltaic solar energy conversion. Elsevier, 391–411.
Kuzemko, C., Lockwood, M., Mitchell, C., and Hoggett, R. (2016). Governing for sustainable energy system change: politics, contexts and contingency. Energy Res. Soc. Sci. 12, 96–105. doi:10.1016/j.erss.2015.12.022
Kyriakopoulos, G. L., Streimikiene, D., and Baležentis, T. (2022). Addressing challenges of low-carbon energy transition. MDPI, 5718.
Lei, Y.-T., Ma, C.-Q., Mirza, N., Ren, Y.-S., Narayan, S. W., and Chen, X.-Q. (2022). A renewable energy microgrids trading management platform based on permissioned blockchain. Energy Econ. 115, 106375. doi:10.1016/j.eneco.2022.106375
Leiserowitz, A. (2007). International public opinion, perception, and understanding of global climate change. Hum. Dev. Rep. 2008, 31.
Li, Q.-K., Lin, H., Tan, X., and Du, S. (2018). H∞ consensus for multiagent-based supply chain systems under switching topology and uncertain demands. IEEE Trans. Syst. Man, Cybern. Syst. 50, 4905–4918. doi:10.1109/tsmc.2018.2884510
Liu, H., Khan, I., Zakari, A., and Alharthi, M. (2022b). Roles of trilemma in the world energy sector and transition towards sustainable energy: A study of economic growth and the environment. Energy Policy 170, 113238. doi:10.1016/j.enpol.2022.113238
Liu, J., Lv, J., Dinçer, H., Yüksel, S., and Karakuş, H. (2021). Selection of renewable energy alternatives for green blockchain investments: A hybrid IT2-based fuzzy modelling. Archives Comput. Methods Eng. 28, 3687–3701. doi:10.1007/s11831-020-09521-2
Liu, L., Tang, Y., and Liu, D. (2022a). Investigation of future low-carbon and zero-carbon fuels for marine engines from the view of thermal efficiency. Energy Rep. 8, 6150–6160. doi:10.1016/j.egyr.2022.04.058
Liu, X., Li, Z., Fu, X., Yin, Z., Liu, M., Yin, L., et al. (2023). Monitoring house vacancy dynamics in the pearl river delta region: A method based on NPP-viirs night-time light remote sensing images. Land 12, 831. doi:10.3390/land12040831
Lovins, A. B. (1976). Energy strategy: the road not taken. Foreign Aff. 55, 65. doi:10.2307/20039628
Lovins, A. B. (1974). World energy strategies: facts, issues and options for policies that transcend today's ‘ad-hocracy. Bull. Atomic Sci. 30, 14–32. doi:10.1080/00963402.1974.11458114
Lu, Y., Khan, Z. A., Alvarez-Alvarado, M. S., Zhang, Y., Huang, Z., and Imran, M. (2020). A critical review of sustainable energy policies for the promotion of renewable energy sources. Sustainability 12, 5078. doi:10.3390/su12125078
Luo, J., Zhuo, W., and Xu, B. (2023). Optimal NGO size of human resources and governance quality of entrepreneurship in circular economy. Management Decision.The bigger, the better?
Luo, L., Tang, Q., and Lan, Y. C. (2013). Comparison of propensity for carbon disclosure between developing and developed countries: A resource constraint perspective. Account. Res. J. 26, 6–34. doi:10.1108/arj-04-2012-0024
Magar, V., Peña, A., Hahmann, A. N., Pacheco-Rojas, D. A., García-Hernández, L. S., and Gross, M. S. (2023). Wind energy and the energy transition: challenges and opportunities for Mexico. Sustainability 15, 5496. doi:10.3390/su15065496
Mahajan, B. (2012). Negative environment impact of solar energy. Environmental Science and Policy, 1–6.
Mahmud, M. P., Huda, N., Farjana, S. H., and Lang, C. (2018). Environmental impacts of solar-photovoltaic and solar-thermal systems with life-cycle assessment. Energies 11, 2346. doi:10.3390/en11092346
Majid, M. (2020). Renewable energy for sustainable development in India: current status, future prospects, challenges, employment, and investment opportunities. Energy, Sustain. Soc. 10, 1–36.
Mannaro, K., Pinna, A., and Marchesi, M. (2017). “Crypto-trading: blockchain-oriented energy market,” in 2017 AEIT International Annual Conference, Cagliari, Italy, 20-22 September 2017 (IEEE), 1–5.
Mao, Q., Ma, X., and Sun, Y. (2023). Study of impacts of blockchain technology on renewable energy resource findings. Renew. Energy 211, 802–808. doi:10.1016/j.renene.2023.05.038
Meinshausen, M., Lewis, J., McGlade, C., Gütschow, J., Nicholls, Z., Burdon, R., et al. (2022). Realization of Paris Agreement pledges may limit warming just below 2 C. Nature 604, 304–309. doi:10.1038/s41586-022-04553-z
Mendecka, B., and Lombardi, L. (2019). Life cycle environmental impacts of wind energy technologies: A review of simplified models and harmonization of the results. Renew. Sustain. Energy Rev. 111, 462–480. doi:10.1016/j.rser.2019.05.019
Merven, B., Hartley, F., and Arndt, C. (2019). Quantifying the macro-and socio-economic benefits of a transition to renewable energy in South Africa.
Meydani, A. (2023). “Development planning policies for renewable electricity generation in competition with fossil electricity,” in 2023 8th International Conference on Technology and Energy Management (ICTEM), Mazandaran, Babol, Iran, Islamic Republic of, 08-09 February 2023 (IEEE), 1–7.
Mirzaei, N., Nejad, M. G., and Fernandes, N. O. (2021). Combining line balancing methods and discrete event simulation: A case study from a metalworking company. Int. J. Industrial Eng. Manag. 12, 14–24. doi:10.24867/ijiem-2021-1-273
Mohideen, M. M., Subramanian, B., Sun, J., Ge, J., Guo, H., Radhamani, A. V., et al. (2023). Techno-economic analysis of different shades of renewable and non-renewable energy-based hydrogen for fuel cell electric vehicles. Renew. Sustain. Energy Rev. 174, 113153. doi:10.1016/j.rser.2023.113153
Mourtzis, D., Angelopoulos, J., and Panopoulos, N. (2022). A literature review of the challenges and opportunities of the transition from industry 4.0 to society 5.0. Energies 15, 6276. doi:10.3390/en15176276
Mukherjee, A. A., Singh, R. K., Mishra, R., and Bag, S. (2021). Application of blockchain technology for sustainability development in agricultural supply chain: justification framework. Operations Manag. Res. 15, 46–61. doi:10.1007/s12063-021-00180-5
Nautiyal, H., and Goel, V. (2020). Sustainability assessment of hydropower projects. J. Clean. Prod. 265, 121661. doi:10.1016/j.jclepro.2020.121661
Nazir, M. S., Ali, N., Bilal, M., and Iqbal, H. M. (2020). Potential environmental impacts of wind energy development: A global perspective. Curr. Opin. Environ. Sci. Health 13, 85–90. doi:10.1016/j.coesh.2020.01.002
Neacsa, A., Rehman Khan, S. A., Panait, M., and Apostu, S. A. (2022). “The transition to renewable energy—a sustainability issue?,” in Energy transition: Economic, social and environmental dimensions (Springer), 29–72.
Nejad, M. G., and Kashan, A. H. (2019). An effective grouping evolution strategy algorithm enhanced with heuristic methods for assembly line balancing problem. J. Adv. Manuf. Syst. 18, 487–509. doi:10.1142/s0219686719500264
Nnabuife, S. G., Ugbeh-Johnson, J., Okeke, N. E., and Ogbonnaya, C. (2022). Present and projected developments in hydrogen production: A technological review*. Carbon Capture Sci. Technol. 3, 100042. doi:10.1016/j.ccst.2022.100042
Nygaard, A., and Silkoset, R. (2022). Sustainable development and greenwashing: how blockchain technology information can empower green consumers. Bus. Strategy Environ. doi:10.1002/bse.3338
Oladosu, G. A., Werble, J., Tingen, W., Witt, A., Mobley, M., and O'Connor, P. (2021). Costs of mitigating the environmental impacts of hydropower projects in the United States. Renew. Sustain. Energy Rev. 135, 110121. doi:10.1016/j.rser.2020.110121
Osorio-Aravena, J. C., Aghahosseini, A., Bogdanov, D., Caldera, U., Ghorbani, N., Mensah, T. N. O., et al. (2021). The impact of renewable energy and sector coupling on the pathway towards a sustainable energy system in Chile. Renew. Sustain. Energy Rev. 151, 111557. doi:10.1016/j.rser.2021.111557
Otoum, S., Al Ridhawi, I., and Mouftah, H. (2022). A federated learning and blockchain-enabled sustainable energy trade at the edge: A framework for industry 4.0. IEEE Internet Things J. 10, 3018–3026. doi:10.1109/jiot.2022.3140430
Owusu, P. A., and Asumadu-Sarkodie, S. (2016). A review of renewable energy sources, sustainability issues and climate change mitigation. Cogent Eng. 3, 1167990. doi:10.1080/23311916.2016.1167990
Pang, M., Zhang, L., Ulgiati, S., and Wang, C. (2015). Ecological impacts of small hydropower in China: insights from an emergy analysis of a case plant. Energy policy 76, 112–122. doi:10.1016/j.enpol.2014.10.009
Peeters, J. (2012). The place of social work in sustainable development: towards ecosocial practice. Int. J. Soc. Welf. 21, 287–298. doi:10.1111/j.1468-2397.2011.00856.x
Perelman, L. J. (1980). Speculations on the transition to sustainable energy. Ethics 90, 392–416. doi:10.1086/292170
Perera, F. (2018). Pollution from fossil-fuel combustion is the leading environmental threat to global pediatric health and equity: solutions exist. Int. J. Environ. Res. public health 15, 16. doi:10.3390/ijerph15010016
Pérez, M. D. L. E. M., Scholten, D., and Stegen, K. S. (2019). The multi-speed energy transition in europe: opportunities and challenges for EU energy security. Energy Strategy Rev. 26, 100415. doi:10.1016/j.esr.2019.100415
Pietrosemoli, L., and Rodríguez-Monroy, C. (2019). The Venezuelan energy crisis: renewable energies in the transition towards sustainability. Renew. Sustain. Energy Rev. 105, 415–426. doi:10.1016/j.rser.2019.02.014
Pinho, P., Maia, R., and Monterroso, A. (2007). The quality of Portuguese environmental impact studies: the case of small hydropower projects. Environ. Impact Assess. Rev. 27, 189–205. doi:10.1016/j.eiar.2006.10.005
Polas, M. R. H., Kabir, A. I., Sohel-Uz-Zaman, A. S. M., Karim, R., and Tabash, M. I. (2022). Blockchain technology as a game changer for green innovation: green entrepreneurship as a roadmap to green economic sustainability in Peru. J. Open Innovation Technol. Mark. Complex. 8, 62. doi:10.3390/joitmc8020062
Popescu, C., Panait, M., Palazzo, M., and Siano, A. (2022). Energy transition in European union—challenges and opportunities. Energy Transition Econ. Soc. Environ. Dimensions, 289–312.
Popkova, E. G., Bogoviz, A. V., Lobova, S. V., Vovchenko, N. G., and Sergi, B. S. (2023). Blockchain, sustainability and clean energy transition. Glob. Transitions 5, 64–78. doi:10.1016/j.glt.2023.04.002
Press, M., and Arnould, E. J. (2009). Constraints on sustainable energy consumption: market system and public policy challenges and opportunities. J. Public Policy & Mark. 28, 102–113. doi:10.1509/jppm.28.1.102
Qazi, A., Hussain, F., Rahim, N. A., Hardaker, G., Alghazzawi, D., Shaban, K., et al. (2019). Towards sustainable energy: A systematic review of renewable energy sources, technologies, and public opinions. IEEE access 7, 63837–63851. doi:10.1109/access.2019.2906402
Quitzow, R., Thielges, S., Goldthau, A., Helgenberger, S., and Mbungu, G. (2019). Advancing a global transition to clean energy–the role of international cooperation. Economics 13, 20190048. doi:10.5018/economics-ejournal.ja.2019-48
Rabaia, M. K. H., Abdelkareem, M. A., Sayed, E. T., Elsaid, K., Chae, K.-J., Wilberforce, T., et al. (2021). Environmental impacts of solar energy systems: A review. Sci. Total Environ. 754, 141989. doi:10.1016/j.scitotenv.2020.141989
Rahman, A., Farrok, O., and Haque, M. M. (2022). Environmental impact of renewable energy source based electrical power plants: solar, wind, hydroelectric, biomass, geothermal, tidal, ocean, and osmotic. Renew. Sustain. Energy Rev. 161, 112279. doi:10.1016/j.rser.2022.112279
Ramzan, M., Raza, S. A., Usman, M., Sharma, G. D., and Iqbal, H. A. (2022). Environmental cost of non-renewable energy and economic progress: do ICT and financial development mitigate some burden? J. Clean. Prod. 333, 130066. doi:10.1016/j.jclepro.2021.130066
Safari, A., Das, N., Langhelle, O., Roy, J., and Assadi, M. (2019). Natural gas: A transition fuel for sustainable energy system transformation? Energy Sci. Eng. 7, 1075–1094. doi:10.1002/ese3.380
Sakellariou, N., and Mulvaney, D. (2013). Engineers and the renewable energy transition: challenges and opportunities. J. Prof. Issues Eng. Educ. Pract. 139, 12–18. doi:10.1061/(asce)ei.1943-5541.0000128
Salam, M. A., and Khan, S. A. (2018). Transition towards sustainable energy production–A review of the progress for solar energy in Saudi Arabia. Energy Explor. Exploitation 36, 3–27. doi:10.1177/0144598717737442
Sánchez-Pantoja, N., Vidal, R., and Pastor, M. C. (2018). Aesthetic impact of solar energy systems. Renew. Sustain. Energy Rev. 98, 227–238. doi:10.1016/j.rser.2018.09.021
Sen, S., and Ganguly, S. (2017). Opportunities, barriers and issues with renewable energy development–A discussion. Renew. Sustain. Energy Rev. 69, 1170–1181. doi:10.1016/j.rser.2016.09.137
Sharma, G. D., Verma, M., Taheri, B., Chopra, R., and Parihar, J. S. (2023). Socio-economic aspects of hydrogen energy: an integrative review. Technol. Forecast. Soc. Change 192, 122574. doi:10.1016/j.techfore.2023.122574
Siampour, L., Vahdatpour, S., Jahangiri, M., Mostafaeipour, A., Goli, A., Shamsabadi, A. A., et al. (2021). Techno-enviro assessment and ranking of Turkey for use of home-scale solar water heaters. Sustain. Energy Technol. Assessments 43, 100948. doi:10.1016/j.seta.2020.100948
Siddik, A. B., Khan, S., Khan, U., Yong, L., and Murshed, M. (2023). The role of renewable energy finance in achieving low-carbon growth: contextual evidence from leading renewable energy-investing countries. Energy 270, 126864. doi:10.1016/j.energy.2023.126864
Streimikiene, D., and Šivickas, G. (2008). The EU sustainable energy policy indicators framework. Environ. Int. 34, 1227–1240. doi:10.1016/j.envint.2008.04.008
Surendra, K., Takara, D., Hashimoto, A. G., and Khanal, S. K. (2014). Biogas as a sustainable energy source for developing countries: opportunities and challenges. Renew. Sustain. Energy Rev. 31, 846–859. doi:10.1016/j.rser.2013.12.015
Svetec, E., Nađ, L., Pašičko, R., and Pavlin, B. (2019). “Blockchain application in renewable energy microgrids: an overview of existing technology towards creating climate-resilient and energy independent communities,” in 2019 16th international conference on the European Energy Market (EEM), Ljubljana, Slovenia, 18-20 September 2019 (IEEE), 1–7.
Sweeney, C., Bessa, R. J., Browell, J., and Pinson, P. (2020). The future of forecasting for renewable energy. Wiley Interdiscip. Rev. Energy Environ. 9, e365. doi:10.1002/wene.365
Takase, M., Aboah, M., and Kipkoech, R. (2022). A review on renewable energy potentials and energy usage statistics in Ghana. Fuel Commun. 11, 100065. doi:10.1016/j.jfueco.2022.100065
Tang, A., Alsultany, F. H., Borisov, V., Mohebihafshejani, A., Goli, A., Mostafaeipour, A., et al. (2022). Technical, environmental and ranking analysis of using solar heating: A case study in South Africa. Sustain. Energy Technol. Assessments 52, 102299. doi:10.1016/j.seta.2022.102299
Tawalbeh, M., Al-Othman, A., Kafiah, F., Abdelsalam, E., Almomani, F., and Alkasrawi, M. (2021). Environmental impacts of solar photovoltaic systems: A critical review of recent progress and future outlook. Sci. Total Environ. 759, 143528. doi:10.1016/j.scitotenv.2020.143528
Tian, J., Yu, L., Xue, R., Zhuang, S., and Shan, Y. (2022). Global low-carbon energy transition in the post-COVID-19 era. Appl. energy 307, 118205. doi:10.1016/j.apenergy.2021.118205
Tirkolaee, E. B., Goli, A., and Mirjalili, S. (2022). Circular economy application in designing sustainable medical waste management systems. Environ. Sci. Pollut. Res. 29, 79667–79668. doi:10.1007/s11356-022-20740-x
Tirkolaee, E. B., Goli, A., and Weber, G.-W. (2020b). Fuzzy mathematical programming and self-adaptive artificial fish swarm algorithm for just-in-time energy-aware flow shop scheduling problem with outsourcing option. IEEE Trans. fuzzy Syst. 28, 2772–2783. doi:10.1109/tfuzz.2020.2998174
Tirkolaee, E. B., Goli, A., Weber, G.-W., and Szwedzka, K. (2020a). A novel formulation for the sustainable periodic waste collection arc-routing problem: A hybrid multi-objective optimization algorithm. Logist. Operations Manag. Recycl. Reuse, 77–98.
Tong, D., Sun, Y., Tang, J., Luo, Z., Lu, J., and Liu, X. (2023). Modeling the interaction of internal and external systems of rural settlements: the case of guangdong, China. Land Use Policy 132, 106830. doi:10.1016/j.landusepol.2023.106830
Truby, J. (2018). Decarbonizing bitcoin: law and policy choices for reducing the energy consumption of blockchain technologies and digital currencies. Energy Res. Soc. Sci. 44, 399–410. doi:10.1016/j.erss.2018.06.009
Usman, M., Jahanger, A., Makhdum, M. S. A., Balsalobre-Lorente, D., and Bashir, A. (2022). How do financial development, energy consumption, natural resources, and globalization affect arctic countries' economic growth and environmental quality? An advanced panel data simulation. Energy 241, 122515. doi:10.1016/j.energy.2021.122515
Van Der Schoor, T., and Scholtens, B. (2015). Power to the people: local community initiatives and the transition to sustainable energy. Renew. Sustain. energy Rev. 43, 666–675. doi:10.1016/j.rser.2014.10.089
Verbruggen, A., Fischedick, M., Moomaw, W., Weir, T., Nadaï, A., Nilsson, L. J., et al. (2010). Renewable energy costs, potentials, barriers: conceptual issues. Energy policy 38, 850–861. doi:10.1016/j.enpol.2009.10.036
Vidadili, N., Suleymanov, E., Bulut, C., and Mahmudlu, C. (2017). Transition to renewable energy and sustainable energy development in Azerbaijan. Renew. Sustain. Energy Rev. 80, 1153–1161. doi:10.1016/j.rser.2017.05.168
Vujanović, M., Wang, Q., Mohsen, M., Duić, N., and Yan, J. (2021). Recent progress in sustainable energy-efficient technologies and environmental impacts on energy systems. Appl. Energy 283, 116280. doi:10.1016/j.apenergy.2020.116280
Wang, X., Chen, G., Afshan, S., Awosusi, A. A., and Abbas, S. (2023b). Transition towards sustainable energy: the role of economic complexity, financial liberalization and natural resources management in China. Resour. Policy 83, 103631. doi:10.1016/j.resourpol.2023.103631
Wang, H., Ghasemi, M., Ghadiri Nejad, M., and Khandan, A. (2023a). Assessing the potential growth of Iran’s hospitals with regard to the sustainable management of medical tourism. Health & Soc. Care Community 2023, 1–12. doi:10.1155/2023/8734482
Wang, Q., Li, R., and Zhan, L. (2021). Blockchain technology in the energy sector: from basic research to real world applications. Comput. Sci. Rev. 39, 100362. doi:10.1016/j.cosrev.2021.100362
Wang, Q., and Su, M. (2020). Integrating blockchain technology into the energy sector—From theory of blockchain to research and application of energy blockchain. Comput. Sci. Rev. 37, 100275. doi:10.1016/j.cosrev.2020.100275
Wang, S., and Wang, S. (2015). Impacts of wind energy on environment: A review. Renew. Sustain. Energy Rev. 49, 437–443. doi:10.1016/j.rser.2015.04.137
Wang, Z., and Razzaq, A. (2022). Natural resources, energy efficiency transition and sustainable development: evidence from BRICS economies. Resour. Policy 79, 103118. doi:10.1016/j.resourpol.2022.103118
Wang, Z., Yen-Ku, K., Li, Z., An, N. B., and Abdul-Samad, Z. (2022). The transition of renewable energy and ecological sustainability through environmental policy stringency: estimations from advance panel estimators. Renew. Energy 188, 70–80. doi:10.1016/j.renene.2022.01.075
Wu, B., Gu, Q., Liu, Z., and Liu, J. (2023). Clustered institutional investors, shared ESG preferences and low-carbon innovation in family firm. Technol. Forecast. Soc. Change 194, 122676. doi:10.1016/j.techfore.2023.122676
Wu, J., and Tran, N. K. (2018). Application of blockchain technology in sustainable energy systems: an overview. Sustainability 10, 3067. doi:10.3390/su10093067
Wu, Y., Wu, Y., Cimen, H., Vasquez, J. C., and Guerrero, J. M. (2022). Towards collective energy community: potential roles of microgrid and blockchain to go beyond P2P energy trading. Appl. Energy 314, 119003. doi:10.1016/j.apenergy.2022.119003
Wünsche, J. F., and Fernqvist, F. (2022). The potential of blockchain technology in the transition towards sustainable food systems. Sustainability 14, 7739. doi:10.3390/su14137739
Xie, X., Jin, X., Wei, G., and Chang, C.-T. (2023). Monitoring and early warning of SMEs’ shutdown risk under the impact of global pandemic shock. Systems 11, 260. doi:10.3390/systems11050260
Xu, X., Lin, Z., Li, X., Shang, C., and Shen, Q. (2022). Multi-objective robust optimisation model for MDVRPLS in refined oil distribution. Int. J. Prod. Res. 60, 6772–6792. doi:10.1080/00207543.2021.1887534
Xu, X., Wang, C., and Zhou, P. (2021). GVRP considered oil-gas recovery in refined oil distribution: from an environmental perspective. Int. J. Prod. Econ. 235, 108078. doi:10.1016/j.ijpe.2021.108078
Yasmeen, R., Tao, R., Jie, W., Padda, I. U. H., and Shah, W. U. H. (2022). The repercussions of business cycles on renewable & non-renewable energy consumption structure: evidence from OECD countries. Renew. Energy 190, 572–583. doi:10.1016/j.renene.2022.03.138
Yavari, R., Zaliwciw, D., Cibin, R., and McPhillips, L. (2022). Minimizing environmental impacts of solar farms: A review of current science on landscape hydrology and guidance on stormwater management. Environ. Res. Infrastructure Sustain. 2, 032002. doi:10.1088/2634-4505/ac76dd
Yi, H., Meng, X., Linghu, Y., and Zhang, Z. (2023). Can financial capability improve entrepreneurial performance? Evidence from rural China. Econ. research-Ekonomska istraživanja 36, 1631–1650. doi:10.1080/1331677x.2022.2091631
Yildizbasi, A. (2021). Blockchain and renewable energy: integration challenges in circular economy era. Renew. Energy 176, 183–197. doi:10.1016/j.renene.2021.05.053
Yin, L., Wang, L., Li, T., Lu, S., Yin, Z., Liu, X., et al. (2023). U-Net-STN: A novel end-to-end lake boundary prediction model. Land 12, 1602. doi:10.3390/land12081602
Yu, B., and Zhou, X. (2023). Urban administrative hierarchy and urban land use efficiency: Evidence from Chinese cities. International Review of Economics & Finance.
Yu, C., Moslehpour, M., Tran, T. K., Trung, L. M., Ou, J. P., and Tien, N. H. (2023). Impact of non-renewable energy and natural resources on economic recovery: empirical evidence from selected developing economies. Resour. Policy 80, 103221. doi:10.1016/j.resourpol.2022.103221
Zakaria, Z., Kamarudin, S. K., Salehmin, M. N. I., Ahmad, N. N. R., Aminuddin, M. A., Hanapi, I. H., et al. (2023). Energy scenario in Malaysia: embarking on the potential use of hydrogen energy. Int. J. Hydrogen Energy. doi:10.1016/j.ijhydene.2023.05.358
Zeleňáková, M., Fijko, R., Diaconu, D. C., and Remeňáková, I. (2018). Environmental impact of small hydro power plant—A case study. Environments 5, 12. doi:10.3390/environments5010012
Zhang, J., Ullah, S., and Khan, K. (2023). The prominence of fossil energy resources in ecological sustainability of BRICS: the key role of institutional worth. Front. Environ. Sci. 10, 1084314. doi:10.3389/fenvs.2022.1084314
Zhou, X., Jia, M., Altuntaş, M., Kirikkaleli, D., and Hussain, M. (2022). Transition to renewable energy and environmental technologies: the role of economic policy uncertainty in top five polluted economies. J. Environ. Manag. 313, 115019. doi:10.1016/j.jenvman.2022.115019
Keywords: sustainable energy, blockchain technology, renewable energy technologies, economic benefits, policy changes
Citation: Lv Y (2023) Transitioning to sustainable energy: opportunities, challenges, and the potential of blockchain technology. Front. Energy Res. 11:1258044. doi: 10.3389/fenrg.2023.1258044
Received: 13 July 2023; Accepted: 28 August 2023;
Published: 14 September 2023.
Edited by:
Alireza Goli, University of Isfahan, IranReviewed by:
Erfan Babaee Tirkolaee, University of Istinye, TürkiyeMokhtar Mohammadi, Lebanese French University, Iraq
Copyright © 2023 Lv. This is an open-access article distributed under the terms of the Creative Commons Attribution License (CC BY). The use, distribution or reproduction in other forums is permitted, provided the original author(s) and the copyright owner(s) are credited and that the original publication in this journal is cited, in accordance with accepted academic practice. No use, distribution or reproduction is permitted which does not comply with these terms.
*Correspondence: Yongjun Lv, lvyj@qdhhc.edu.cn