- 1Energy, Exergy and Environment Research Group (EEERG), Department of Mechanical Engineering, University of Calabar, Calabar, Cross River State, Nigeria
- 2Energy and Thermofluid Research Group, Department of Mechanical Engineering, Faculty of Engineering, University of Port Harcourt, Choba, Rivers State, Nigeria
- 3Tasmanian School of Business and Economics, University of Queensland, Brisbane, Australia
- 4Centre for Climate Change and Development, Alex Ekueme Federal University, Ndufu, Ebonyi State, Nigeria
- 5School of Policy Studies, University of Bristol, Bristol, United Kingdom
- 6Regional Leading Research Centre for Smart Energy System, Kyungpook National University, Sangju, Republic of Korea
- 7Department of Convergence and Fusion System Engineering, Kyungpook National University, Sangju, Republic of Korea
- 8The Department of Mechanical Engineering, Delta State University, Abraka, Delta State, Nigeria
- 9Department of Energy Convergence and Climate Change, Kyungpook National University, Daegu, Republic of Korea
The buildings sector is responsible for over 36% of total global end-use energy utilization and nearly 40% of the total indirect and direct carbon emissions. Low-carbon or zero-energy buildings remain the only option to lessen the sector’s energy consumption and CO2 emissions. The current systematic study examines low-carbon buildings under deep decarbonization scenarios in selected global south regions from 2010 to 2021. The study was channelled by the PRISMA (“Preferred Reporting Items for Systematic reviews and Meta-Analyses”) review process, which identified 29 related articles from Scopus, Web of Science., and Google Scholar databases. The identified critical drivers of emissions were population, gross domestic product, dwelling characteristics, and urbanization. The dwelling characteristics contributed about 12% and 27% to the total CO2 emissions in the selected regions. The population varies between 23% and 27% across the areas. Specific findings were made for inclusion in the Nigeria model while the general results were observed and further studies proposed. Total investment from the private and public sectors was identified as key to achieving the transition process of decarbonization in the building sector.
1 Introduction
1.1 Global energy consumption and CO2 emissions
The synthesis report on the aggregate consequence of Nationally Determined Contributions (NDC) identifies emission gaps more significant than 10 GtCO2eq amid the world GHG emissions based on the synopsis of NDCs in COP21 and the target pathways of 2°C (UNEP, 2017). On this note, the Paris proclamation has appealed to countries for more robust or ambitious decarbonization targets, to completely decarbonize the global economy by 100% with green energy by 2050 and net-zero emissions by the mid-century to keep the world on the trajectory of realizing below 1.5°C of warming (IPCC, 2018). Additionally, in 2017 the aggregate global energy consumption increased by 29%, reaching approximately 26,700 TWh in 2018. The international production profile increased by 30% from 2016 to 2018. Based on the current global population growth scenario and socioeconomic activities, there are strong indications from the United Nations human development index that global energy will reach 100 GJ per person in the coming years. Fossil fuels will dominate the latter, contributing around 75% of the world’s electricity demand in 2018, thus increasing CO2 emissions (REN21, 2020; IEA, 2019; Zahraoui et al., 2021). Residential buildings specifically account for about 27% of the global final energy utilization and about 17% of the global CO2 emissions (Nejat et al., 2015; Güneralp et al., 2017). However, the energy consumption level in the residential building sector grew by 14% between the years 2000 and 2010. This increase in energy consumption is estimated to be sustained due to demographic growth, economic expansion, and urbanization. Conversely, the global energy utilization for space heating and cooling is likely to reach a 40% increase by the mid-century, depending on some uncertainties (Eom et al., 2012a; Leibowicza et al., 2018). However, these tendencies are predominantly evident in developing economies, whose energy utilization levels are projected to converge near the consumption levels for the developed countries arising from an increase in household demand, employment, incomes rise, and increase in energy activities. Furthermore, the 2020 global energy consumption status report indicates that the building sector’s carbon emissions were highest in 2019 (IEA, 2020). The report shows that the overall global final energy use in the building sector was unchanged in 2019 when compared to the consumption levels in the preceding year, carbon emissions from the activities of buildings, which have risen to their maximum level hitherto at about 10 GtCO2, or 28% of the overall global energy-related CO2 emissions (Figures 1A, B). Similarly, with the addition of emissions from building construction, the share of CO2 emissions rose to 38% of the overall global energy-related carbon emissions (Figure 1B). The latter underscores the significance of multiple approaches to aggressively moderate energy demand from the building sector whereas decarbonizing the power sector besides implementing materials strategies that decrease the carbon emissions lifecycle (IEA, 2020).
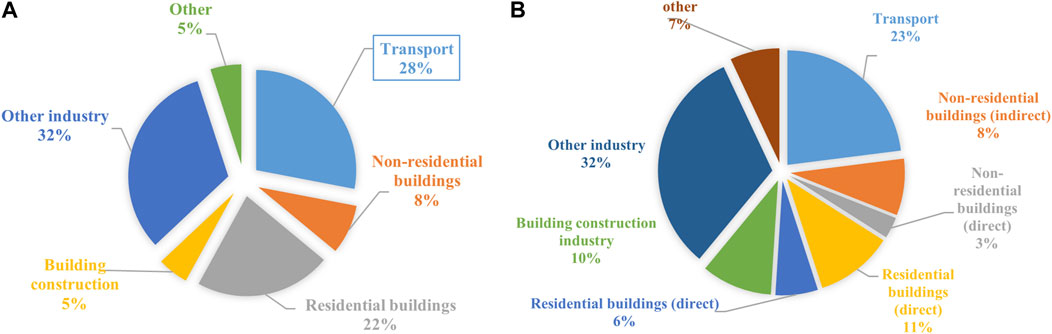
FIGURE 1. (A) Global share of final energy consumption in buildings and construction, (B) Share of emissions from buildings and construction, 2019. Sources: (IEA 2020).
Buildings remain a critical area that lacks detailed mitigation strategies despite their significance to global carbon emissions. A report from (IEA, 2020) indicates that most nations have not submitted their second NDC. However, out of the 136 countries that submitted their NDC, only 53 nations remarked on energy efficiency in buildings. At the same time, 38 explicitly call out energy codes in buildings, indicating the prominence of energy efficiency in building for the future of our climate. Additionally, to achieve a zero-carbon building, construction should be done using suitable certification stock that drives carbon emissions in the built sector (IEA, 2020).
1.2 Research questions and paper structure
In building a relevant systematic review, the present study was channelled by the following research questions: 1) What is the current knowledge in the open literature on deep decarbonization in buildings in the global south? 2) What are the identified key drivers of emissions in the building sector in the global south countries 3) what are the technological options for low Emissions in buildings? 4) What gaps or contributions in the literature can assist Nigeria in building its decarbonization pathways? The study was focused on low-income countries in the global south since their energy consumption is heterogeneous. Such circumstances are likely familiar to Nigeria. In this background, the knowledge gap may contribute to crafting the Nigerian scenarios since the level of activities in these regions parallels the building sector. Therefore, the remaining sections of the paper are structured in the following broad perspective 1) the methodology, including the PRISMA (“Preferred Reporting Items Systematic Reviews and Meta-Analysis”) procedure used, 2) systematic reviews and amalgamation of the scientific studies to identify, select and evaluate appropriate research on low carbon building under deep decarbonization scenarios in the Global South and 3) identification of gaps and future research primacies.
1.3 Objective of the study
The energy services needed within the building sector are varied. As the socioeconomic status of the households improves, the progression of the energy utilization is in two dimensions: 1) The services provided by the energy and 2) the required energy carrier to satisfy these services. These concentric dimensions usually are studied together to accomplish an all-inclusive understanding of the consequence of the building sector on the combined climate change and economic development problems. However, despite existing studies on low carbon building (LCB), scenarios, and deep decarbonization pathways (DDP), efforts toward a systematically comparative appraisal of these studies in developing economies are lacking. The present study endeavours to narrow the breach in understanding by identifying different approaches, policies, and scenarios to achieve LCB. Thus, the study presents a systematic literature review on LCB under deep decarbonization scenarios in selected global south regions (Asia, Latin America, and Africa), emphasizing Nigeria. The findings from this study will assist Nigeria in developing its scenarios in the proposed DDP project since Nigeria shear the same economic development features as the regions in question.
2 Model-based studies in building decarbonization pathways
On the other hand, more than a few earlier studies on energy modeling in the building have incorporated depictions of energy supply, service-based demands, and end-use technologies to describe decarbonization pathways and energy flow trajectories. Some of these studies have been followed by scholars at the “Joint Global Change Research Institute using the Global Change Assessment Model” (GCAM), an integrated valuation framework. The GCAM permits the demand in the energy service to react to the endogenous service costs by stipulating price elasticities of the demand. In the GCAM model, the formulation is carried out by building the demand for energy service according to the evolving fluctuations in the demography, per capita revenue or income, and floor space per capita. However, individual researchers have concentrated precisely on the residential or the building sectors such as China (Eom et al. 2012b), India (Chaturvedi et al., 2014), and the United States of America (Kyle et al., 2010; City of Austin, 2015).
Furthermore, the TIMES model has been used to examine the progression of building energy utilization in China (Shi et al., 2016) and decarbonization pathways for the Canadian building sector (Vaillancourt et al., 2017). In the Canadian studies, three concurrent transformations necessary for cost-effectively actualized decarbonization in buildings were identified: electrification of the end-uses, decarbonization of the electricity supply, and efficiency improvements. The decarbonization scenarios are aimed at 60% emissions reduction by 2050 compared to the 1990 levels. At the global level, the Global Energy Assessment (Johansson et al., 2012) explores three alternative sets of transformation pathways to develop scenarios and energy consumption patterns in the building sector. Two integrated assessment models include the MESSAGE (“Model of Energy Supply Strategy Alternatives and their General Environmental Impacts”). In addition, the “World Energy Scenarios of the World Energy Council” (World Energy Council, 2016) presented three investigative scenarios demonstrating alternative energy transition futures dating to 2060 and based on the Global Multi-Regional MARKAL energy model.
Additionally, some building models have been domesticated to solve and model peculiarities—the CBEM (“China’s building energy consumption model”). The CBEM model is a bottom-up approach that comprises five modules (Guo et al., 2021). These include a building-stock module, northern-urban heating, commercial and public buildings, urban-residential buildings, and rural residential buildings. The CBEM was applied to model energy consumption and carbon emissions in China’s building sector spanning 2050 for different conditions and scenarios. Other models include the RMEG (Residential Energy Model Global) (Ekholm et al., 2010), a stylized “bottom-up” system model approach that differentiates between one energy function and the other how the specific demands for the energy functions are met. These have been applied to several studies (Daioglou, 2010; Daioglou et al., 2012).
2.1 Energy service demands and end-use technologies in the building sector
Some critical energy functions (and related drivers) play a crucial role in building energy utilization. Such functions comprise space cooling and heating, water heating, appliances, lighting, and others (Newton and Tucker, 2011). Modeling these core energy functions will assist in understanding the dynamics and likely impending trends in the building sector. The building energy amenity or service demands can be affected by human behavior, climate, and economic growth. Some building energy demand amenities can be met by limited energy. However, the building sector’s end-use energy demand and technologies vary from country to country and region to region, even within the same country. Different countries have developed specific models to handle their end-use energy demand and specific end-use technologies. The studies in (Shi et al., 2015) presented the end-use energy service and the structure of the China building sector in TIMES (See Figure 2). The structure contains the supply side, with coal, natural gas, LPG., electricity biomass, and geothermal as the energy carries. At the same time, the service demands and the end-uses are boilers, air conditioners, fluorescent, and incandescent. The end-use energy demand was modeled based on the climatic zone; as such, demand may vary due to variations in ambient conditions. The studies of (Leibowicza et al., 2018) in the United States building sector presented seven energy demand services (Figure 3).
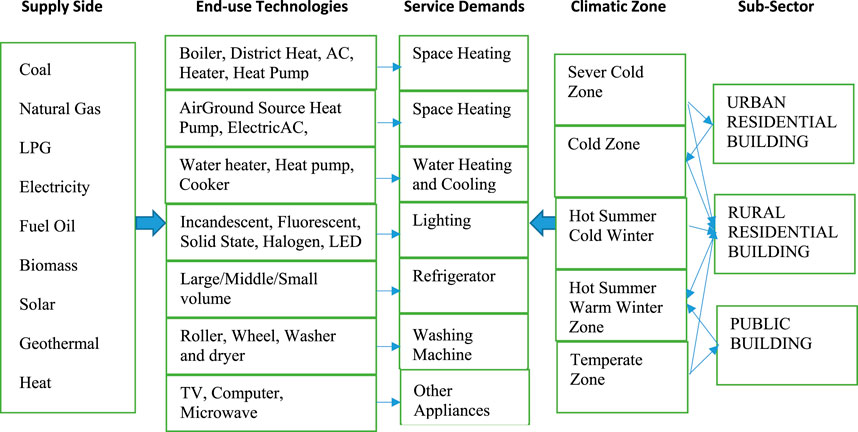
FIGURE 2. Energy service demands and end-use technologies in the building sector in China TIMES (Shi et al., 2015)
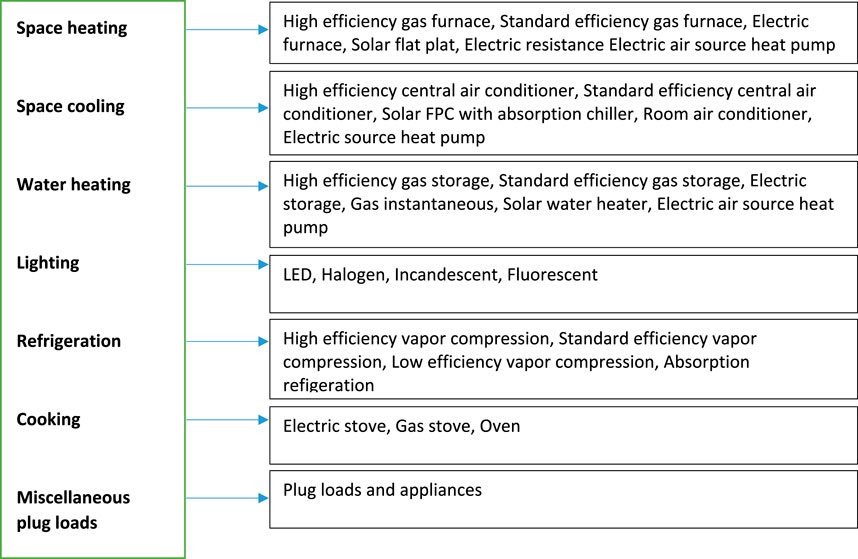
FIGURE 3. Energy service demands and end-use technologies in the building sector in China TIMES (Daioglou, 2010)
The energy service demand type included a list of specific end-use technologies. Also, some demand types and alternate end-use choices are essentially different technologies; for example, the model provided fluorescent, halogen, incandescent, and LED. alternatives in the lighting. These lighting choices or options consume Electricity. Other demand types comprise end-use technologies that use different inputs to allow fuel switching. For example, the technologies that fulfil space heating requirements need solar energy, Electricity, or natural gas. Additionally, comparing energy service demand for the cases in (Shi et al., 2015; Leibowicza et al., 2018) shows an apparent disparity in their energy service demands. Thus, different conditions or scenarios will be required to address specifics. The latter is why the building energy modeling is performed based on country-specific energy service demand.
2.2 Energy policies progression in the building sector
In recent times energy-related disquiets have widened to comprise GHG emissions, fossil-fuel diminution, global warming, and the advancement in green energy sources. Thus, building energy efficiency is the foundation for policy development and a promising instrument for Kyoto protocol compliance (Brounen et al., 2012). Energy efficiency likewise plays an essential part in the perspective of sustainable growth. It creates pathways for energy savings and CO2 reduction without destabilizing the wellbeing of the building inhabitants (Pilkington et al., 2011; Wada et al., 2012). Additionally, several procedures have been undertaken to lift energy policies globally. About 118 nations in 2010 have engaged different energy strategies at the state, sectorial, and countrywide levels (Martinot, 2012); most of these strategies have been market transformation (MT) driven, defined as the enduring achievement of energy-efficient technologies within the marketplace. The classification of measures to actualize MT has been categorized twofold: the entry of new technologies and enhancing their competitiveness. Based on the category, the most recurrently applied strategies include the 1) BECs (“Building energy codes”). The BEC is the primary regulatory instrument used during the design phase by policymakers to reduce building energy demand in the long term. The BEC. set the least building energy requirement at the design phase, 2) setting standards for electronic equipment with minimum energy requirement and high energy efficiency levels, and 3) Raised public awareness through labels on equipment and educating clients regarding the use of energy-efficient appliances and long-term energy use on buildings, 4) Motivations, in terms of incentives (financial and non-financial) such as tax benefits, and promoting energy-efficient residences (IEA, 2023). Over 32 countries in the world have applied the BECs at varying strictness. For example, in countries like Russia, Korea, India, Chile, and Tunisia, BEC is obligatory. Still, not all building stock aspects are affected (IEA, 2013). Nonetheless, this is still challenging in most developing economies or low-income countries.
2.3 CO2 emission profiles of the selected global south countries
The total number of countries selected from the global south was fifteen. In Asia, four countries were selected: Pakistan, India, Indonesia, Thailand, and Malaysia. Other countries in Latin America are Argentina, Mexico, Columbia, and Costa Rica. Similarly, in Africa, Nigeria, Algeria, Morocco, and South (Figure 4). Their Emission per capital in 2018 is also presented in Figure 4, wherein Asia: Pakistan, Malaysia, and Thailand had the highest EPC of 1.04, 7.6, and 3.7 respectively (Sandu et al., 2019; Climatewatchdata, 2021; Worldometers, 2021). For India, the EPC was 1.80 in 2018, a reduction of about 5.8% from 2016 values. For Pakistan, in 2016, the EPC was 0.87, with total CO2 emission of over 170 million tons. The EPC value increased to 1.04 in 2018, amounting to 16.34%. In Africa, Nigeria’s total CO2 emissions in 2016 stood at over 74 million tons with an EPC of 0.77. The EPC increased to 0.7 in 2018, culminating in a 42% increase. Other EPC. s data for respective countries are shown in Figure 4. The emissions trend is bound to have increased in recent times. Specific emission data for the building sector are usually not separated from the aggregate emissions. However, the rising values in EPCs is a growing concern and call for adequate emission tracking and documentation of sector-by-sector emission records for planning purposes.
3 Methodology
The PRISMA’s systematic procedure was employed, which comprises resources from Web of science (WoS), Scopus, and Google Scholar, to run the systematic review. First, beginning from the exclusion and eligibility criteria, followed by other steps, the identification, screening, eligibility, data abstraction, and evaluation. The procedure applied to retrieve journal articles and papers related to low carbon building under deep decarbonization scenarios in the global south regions is discussed in this section.
3.1 PRISMA
The review process followed the PRISMA statement. The PRISMA offers the following advantages: It lucidly defines the research questions and offers methodical research. It identifies both inclusion and exclusion criteria. And finally, it tries to scrutinize a more expansive database of scientific compendiums in a well-defined time. It allows for rigorous exploration of terms associated with low carbon building under deep decarbonization scenarios in selected global south regions. Systematic review minimizes predisposition by applying straightforward methods and identifying gaps and novel directions for future research. These methods are extensively used in health and related research (Ford and Pearce, 2010; Ford et al., 2011); nevertheless, they are not common in environmental science and climate change studies. In this context, the systematic literature review (SLR) is applied for monitoring the drivers of CO2 emissions, deep decarbonization pathways, and policies in the building sector for selected global south regions. However, the absence of detailed studies on deep decarbonization pathways in developing areas makes it difficult to reproduce the research and apply such methods within regions with standard energy demand features. A systematic review will provide implicit information for improving studies in this respect in the global south.
3.2 Resources
The review process depends on three focal journal databases–Google Scholar, Web of Science (WoS), and Scopus. Google scholar is an extensive database that publishes about two million articles annually. The WoS is a robust database comprising over 33,000 journals covering over 256 disciplines containing a wide variety of subjects associated with climate change, deep decarbonization of the building sector, residential energy utilization, environmental studies, and social sciences (Sierra-Correa and Kintz, 2015; Shaffril et al., 2018; Emodi et al., 2021). Furthermore, it is included over a century of wide-ranging back file and citation index data, confirmed and ranked by Clarivate Analytics in three specific measures: papers, citations, and citations per paper. Furthermore, the Scopus database is the most extensive citation and abstract database of peer-reviewed research literature containing over 22,800 journals on different topics and areas of science, engineering, energy, and built and environmental sciences from about 5,000 publishers (Sierra-Correa and Kintz, 2015; Shaffril et al., 2018).
3.3 Eligibility and exclusion criteria
The eligibility and the exclusion criterion are first determined. Its first begins with the type of literature. Journal articles with empirical and qualitative data, grey literature, and conference proceedings were included. At the same time, systematic reviews, book series, and book chapters are excluded. Secondly, to avoid ambiguity and difficulty in interpretation, non-English publications are excluded. Thirdly, a study period of 12 years study was selected, ranging between 2010 and 2021. The study was done to observe the advancement of research on the subject in question. Based on the set of objectives, the studies only included deep decarbonization pathways, scenarios, and emission drivers in the low-income economies in the global south were considered (Table 1).
3.4 Systematic review process
Four different levels of the systematic review procedure were involved at this point. The systematic review was conducted in October 2021. The first stage identified the keywords for the search procedure related to studies on low carbon building and deep decarbonization in the building sector. The search query are (“buildings” OR “house*” OR “public” OR “residential” OR “commercial”) AND (“decarbonisation “OR “decarbonization” OR “low carbon” OR “low emission” OR “zero emission” OR “transition”) AND (“pathways” OR “scenarios” OR “policy*”). For Scopus and Web of Science databases, the search was set to retrieve potential relevant publications within “Article title, Abstract, Keywords”. The search query returned 14,594 and 10,702 potentially relevant articles from Scopus and Web of Science databases, respectively. For Google Scholar, the initial output gave 404,000 articles, this was because the search parameters on the Google Scholar database was set to retrieve results where the words occur “anywhere in the article” which lead to the initial huge number of articles; as the only other option of “in the title of the article” would have been insufficient. After preliminary scanning of the Google scholar output the number was reduced to 719 potentially relevant articles. The second procedure involves data screening. At this stage, out of the 20,314 available articles eligible for review, 18,504 articles were removed.
Furthermore, the complete article papers were accessed in the third stage eligibility phase. After careful analysis of the articles, 1,745 were excluded. Some articles did not center on deep decarbonization scenarios and were not within the countries of research interest. The final stage of the review led to 29 articles included in the systematic review and the analysis (see Figure 5).
3.5 Abstraction of data and evaluation
The selected articles for eligibility were evaluated. The emphasis was on specific studies that addressed the research questions. First, the data extraction was performed by reading the abstracts and the entire article to substantiate relevant major and minor subjects. However, the qualitative evaluation was implemented by recognizing topics associated with the deep decarbonization scenario in the building sector of the global south regions. Different decarbonization pathways, policies, and emission drivers for the studied countries were identified and categorized.
4 Results
4.1 Characteristics of the reviewed studies
4.1.1 Methodology applied by reviewed literature
The rigorous search was conducted based on PRISMA (Preferred Reporting Items for Systematic Reviews and Meta-Analyses) to obtain articles or subject titles associated with low carbon building under deep decarbonization in the building sector for global south countries (See Figure 5). Furthermore, to advance significant inputs from low carbon building under deep decarbonization (LCBDD) in global south countries, the formulated research questions as presented in Section 1.2 (§1.2) were considered. Few records on LCBDD addressed the building sector (Residential and commercial), while two records were found addressing building design codes to improve building efficiency (Shukla et al., 2015).
4.1.2 Articles published by countries
The reviewed studies covered fourteen global South countries classified into Africa, Latin America, and Asia. The percentage of countries contributing to the total SLR. is presented in Figure 6. The highest studies contributing to the SLR are from Nigeria; about 21% of studies were from Nigeria, 17% from India, while studies from Mexico, Pakistan, and Costa Rica contributed 7%. The rest contributed between 3% and 4% to the overall SLR. However, 32% of the studies reviewed considered the building sector only in a single study, and 28% had considered building and commercial sectors in a single survey. In comparison, 40% had considered all sector inclusion in one study.
4.1.3 Articles published by journals
The total numbers of papers for inclusion are 29, as presented in Table 2, with paper classification ranging from B1-B29. Of the 29 papers, 55.17% are journal papers, 27.57% are technical reports, and 7% are conference papers. In Asia, most of the studies are journal papers, about 50%, while studies published in technical information and conferences are 37.5% and 50%, respectively. Similarly, 31.25% of papers for Latin America were Journal publications, and 62.5% were technical reports. 31.25% of articles were Journal papers in Africa, and technical papers constituted about 37.5%. More studies are carried out in Latin America, Asia, and Africa. Studies in Latin America and Asia had funding, while most African studies are self-funded research. About 12.5% of studies had funding, and 87.5% were non-funded. However, this underscores the low state-holders participation in climate change mitigation research in Africa.
4.1.4 Scenario characteristics and adapted models
The studied specifications B1-B27 (Table 2) use different combinations of trends to include a range of assumptions for the developed scenarios. Table 4 summarises the techniques and the adapted models used by other core scenario-based studies. The SLR show that about 72.22% of the studies used the LEAP model. Studies B1, B2, B8, B16, and B28 used R.E.U., Energy plus, EXSS, NESCAL 2005, and ELENA, respectively, and constituted about 5.55% utilization of each of the total studies. Scenarios ranged from simple to robust depending on the studies’ peculiarities and objectives. The scenario ranking is presented in Table 3. However, studies B7, B12, B4, B16, and B19 had considered varied scenarios ranked in that order.
4.2 Findings and lessons learned for Nigeria’s residential sector decarbonization
4.2.1 Drivers of emissions: Unravelling influences
The drivers of emissions vary across the considered locations. Figure 7 presents the various contributions to emissions in Asia, Latin America, and Africa, respectively. Asia, which includes Pakistan, Indonesia, Malaysia, India, and Thailand, had 19 drivers of emissions. These include population, GDP growth rate, per capita income, household size, and energy consumption. Thailand considered gross output while Pakistan considered floor space area, and other countries in Asia did not consider any of the characteristics. Conversely, India had included more different drivers such as gender, dwelling types, and household fractions using various energy sources. Therefore, it can be inferred drivers like Population, GDP, and number per household are very influential drivers of emissions in Asia, constituting about 27%, 27%, and 6%, respectively.
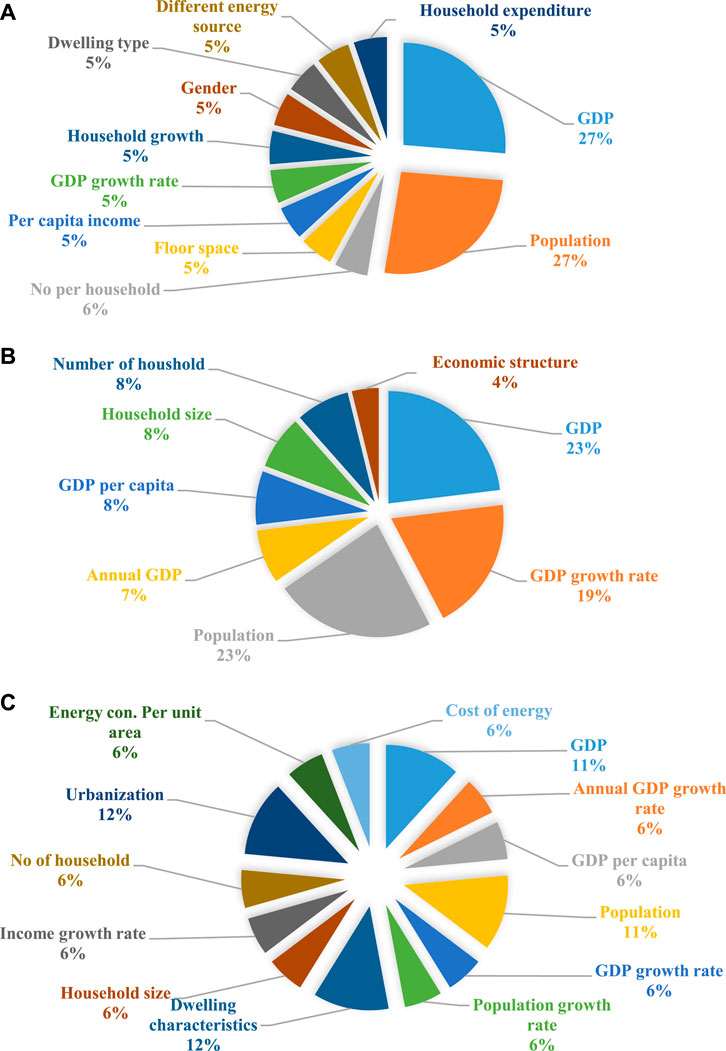
FIGURE 7. (A) Contribution of different emission drivers to the overall emissions in the considered Asian studies; (B) Contribution of different emission drivers to the overall emission in the considered Latin America studies; and (C) Contribution of different emission drivers to the overall emissions in the considered African studies.
For Latin America (LA.) (Brazil, Argentina, Columbia, Ecuador, Mexico, and Costa Rica), a total of 26 emission drivers were across countries ranging from GDP, GDP growth rate, Population, Annual GDP, GDP per capita, household size, number of households and economic structure. Brazil and Mexico considered drivers such as annual GDP growth rate and economic systems different from other countries. The economic structure contributed about 4% to the total emission drive in LA and 33.33% to the emission drive in Mexico. Similarly, the annual GDP growth rate contributed about 7% to the total emission drive and about 33% to the emission drive in Mexico. Therefore, the primary emission drivers in the region are GDP, GDP growth rate, and population, which stood at 23%, 19%, and 23%, respectively.
For Africa, 17 emission drivers were observed in ten studies. The drivers were spread across Nigeria, Algeria, Morocco, and South Africa. The major emission drivers were population (11%), GDP (11%), urbanization rate (12%), and dwelling characteristics (12%). However, two countries, Nigeria and Morocco, considered dwelling characteristics that contributed about 25% to the total emission drive in the countries. In contrast, dwelling characteristics and annual GDP contributed approximately 11% to the entire emission drive in Nigeria and 25% to South Africa.
For specific countries like Algeria, energy cost per unit area is a solid driving force of emissions. Urbanization, population, GDP, household growth rate, and economic structure are growing emissions forces, for others. Similarly, the cost of energy consumption per unit area considered by Algeria alone contributed about 50% to the emission drive in Algeria and about 6% to the total emission drive in countries. Additionally, comparing the whole studies for the three global south regions, population, GDP, GDP growth rate, and urbanization posed a significant challenge in emission drive.
4.2.2 Underlying causes: A roadmap for decarbonization
According to a review of the selected literature, it could be inferred that the residential sector of many Global South nations face a web of drivers that contribute to emissions ranging from various socioeconomic, cultural, technical, and policy variables. To proffer any decarbonization roadmap requires understanding the underlying issues connected with emissions in these regions and highlighting the common traits they share. These common emission driver characteristics include:
4.2.2.1 Energy sources and access
It was discovered that the availability of stable and cost-effective energy resources remains a concern in many countries across the Global South, forcing households to rely primarily on traditional biomass fuels such as wood, dung, and crop wastes for heating and cooking. However, inefficient combustion of these fuels produces significant pollutants, contributing to environmental concerns.
4.2.2.2 Urbanization and population growth
Nations in the Global South are experiencing rapid urbanization and population growth which is consequently driving up housing demand, resulting in the construction of new structures. This expansion in turn leads to increased energy consumption, both directly for heating and cooling and indirectly for the manufacture of building materials. Additionally, this expansion in population and urbanization leads to land use changes for building constructions resulting in deforestation of natural habitats
4.2.2.3 Low-income housing
These type of households often lack access to modern energy services and rely on inefficient and polluting cooking stoves, lighting, and heating techniques, and are characteristic most residential structures in countries of the Global South. These households are disproportionately affected by indoor air pollution, which lead to health issues and increased emissions.
4.2.2.4 Inefficient technology
Appliances used in households in these countries are usually obsolete and inefficient causing higher energy demand and emissions. These are everyday household appliances including heaters, lighting, and stoves for cooking. This loop of inefficiencies is maintained by a lack of access to contemporary and more efficient technologies.
4.2.2.5 Behavioral practices
High energy consumption and emission trends can be influenced by cultural customs and norms. For instance, in Nigeria there is a preference for cooking using firewood because of the belief that it adds a peculiar taste to the food. Cohabitation of big families also increase energy use and emissions.
4.2.2.6 Lack of awareness
There is also the issue of limited access to information and education about the environmental effects of certain activities, which consequently hampers the adoption of energy-efficient habits and technologies.
4.2.2.7 Income inequality
The kind of energy source and equipment used in a household are impacted by income level. Due to their financial limitations, low-income households cannot invest in energy-efficient devices, which results in higher emissions.
4.2.2.8 Weak regulatory framework
Inadequate laws in some regions, as well as weak enforcement mechanisms in some other places, result in inferior building practices and the adoption of inefficient technologies. Additionally, inconsistent policies and lack of incentives by government poses challenges contributing to greater emissions
4.2.3 Tailored challenges and opportunities for Nigeria
The review’s lessons shed light on unique issues Nigeria has in its decarbonization journey. Rapid urbanization and rising housing demand, combined with inefficient technology, all lead to increased energy use and emissions. Nigeria may address these concerns by promoting energy-efficient appliances, clean cooking efforts, and sustainable building techniques.
4.2.4 Technology options for decarbonization
The various studies have defined technology pathways to reduce the emission trajectories in the regions of consideration. The primary technologies in the residential sector include electric stoves and heaters, electrical appliances, refrigeration/air-conditioning, kerosene stoves, and heating devices. The studies show that Mexico, Argentina, Colombia, Ecuador, and Costa Rica had a 21% fall in residential sector emissions from 2015–2050 and a 13% improvement in energy efficiency (Groves et al., 2020). The latter was due to the change in technology that shifted from the GHG biomass cooking and heating to a cleaner use of N. G., LPG cookstoves, and Electricity in the residential sector. It is also observed that the residential and service sectors offer prospects in the transition to low-carbon buildings. Most studies opined that the primary concern should be the complete phase-out of low-efficient incandescent bulbs and injecting the sector with efficient cooking devices. In addition, minimum efficient energy standards for domestic appliances such as refrigerators, air conditioners, and television are worth considering. Furthermore, renewable energy systems, including rooftop solar photovoltaic systems, are promising technologies for reducing carbon footprint.
4.2.5 Technology options: Potential prioritization of technologies per region
Decarbonization efforts in the Global South’s residential sector can be adapted to specific locations in order to create sustainable and cleaner energy consumption patterns. Countries in Asia, such as India and Thailand, can focus on boosting the use of efficient cook stoves, solar water heaters, and rooftop solar panels. In Latin America, Brazil and Argentina can prioritize the growth of clean cooking technologies such as induction cooktops and biogas digesters. Meanwhile, African countries such as Nigeria and Morocco should promote the adoption of energy-efficient appliances, off-grid solar solutions, and better building designs. These technologies can be adapted to all the regions and it is also important to highlight that raising awareness about energy conservation and gaining access to clean and inexpensive energy sources are critical components of decarbonizing the residential sector in all regions.
4.2.6 Technology options for Nigeria’s decarbonization
In order to achieve decarbonization, technological approaches must be customized to Nigeria’s specific circumstances. While electric stoves and heaters are being proposed as viable solutions, it is critical to prioritize solutions that are compatible with Nigeria’s electricity situation. Off-grid solar solutions, energy-efficient appliances, LPG cook stoves and environmentally friendly building designs are emerging as attractive avenues for Nigeria.
4.2.7 Policy options for decarbonization
Different policies and options for decarbonization have been proposed across the regions of studies, which include.
1. The development of assessment standards and passive ultra-low energy technology guidelines for buildings.
2. Lateral progression of economic expansion to be followed with high penetration of energy-efficient devices and little population growth.
3. The effective building envelope should be a primary objective focused on the materials used for buildings to reduce heat transfer interactions through walls. Thus improving overall building efficiency.
4. Incandescent bulbs are replaced with low-energy bulbs; solar cookers, and electric stoves to replace LPG stoves for cooking.
5. Reduction in energy intensity, urbanization rates, per capita floor spaces, and gross domestic product.
4.2.8 Policy options: Proposed for Nigeria and the global south
To significantly contribute to larger sustainable development goals and climate mitigation efforts in Nigeria’s residential sector and consequently in the Global South the following specific policy interventions can provide an enabling environment for seamless decarbonisation (Organi sation for Economic Co-operation and Development, 2022; Center on Global Energy Policy, 2023; United Nations, 2023):
4.2.8.1 Financial incentives and subsidies
Lowering rates for renewable energy use or providing financial support for the purchase of energy-efficient appliances and solar installations are examples of this. Subsidies, incentives, and tax breaks should also be used to encourage adoption.
4.2.8.2 Energy efficiency standards and labelling
This can be achieved by setting and enforcing energy-saving requirements for appliances and buildings; and also implementing mandated labeling systems to assist consumers in making educated decisions about energy-efficient products.
4.2.8.3 Clean cooking initiatives
Programs to showcase clean cooking solutions and offering access to affordable and efficient cook stoves fueled by renewable energy sources.
4.2.8.4 Building codes and standards
The government of Nigeria and respective global south nations should not only create, but enforce building codes that place a premium on energy efficiency and sustainability, and also encourage the use of ecologically friendly building materials and designs that improve energy efficiency.
4.2.8.5 Microfinancing and loan facilities
Creating accessible lending programs and microfinancing options so that households can invest in renewable energy technologies without facing prohibitive costs.
4.2.8.6 Partnership and international support
Encouraging partnerships with international organizations, development organizations, and other nations to get access to technical know-how, knowledge exchange, and financial support for activities aimed at the transition to sustainable energy.
4.2.8.7 Waste management policies
Governments of Global South nations should enact and implement policies to encourage environmentally friendly waste management methods in the residential sector, such as recycling and composting. These methods can help to limit the amount of waste that ends up in landfills, which can generate potent greenhouse gas such as methane.
Furthermore, drawing on insights from global South evaluations is crucial for steering Nigeria’s decarbonization policies in the residential sector. Strengthening building codes to emphasize energy efficiency and embracing clean cooking technologies, alongside well-designed financial incentives, holds the key to a more sustainable future. Collaboration between public and private sectors, grounded in robust research and inclusive governance, will propel Nigeria and other Global South nations toward a greener and more prosperous path of development.
4.2.9 Economic impact and cost of decarbonization options
The deep decarbonization of the building sector has some far-reaching economic implications. Decarbonizing the building sector has to start with the entire energy sector down to the end-use sectors (buildings) (Commerce, 2022). Substantial variations of the energy and capital costs induced and the productivity upshot of energy efficiency are likely to influence the macroeconomic stability over the period significantly. Decarbonization costs comprise the infrastructure and the equipment required to provide zero-carbon energy. And also the costs associated with the provision of clean Electricity. The latter will increase the expenditures on clean energy fuels and Electricity by energy consumers and the expenses for upgrading infrastructure and equipment for households and services, such as appliances and new power generating plants. However, from the reviewed literature In Argentina, the cost of decarbonization from the power generation to the end-use inclusive is considered at 1.5 Billion USD in 2050 with CO2 carbon capture for the Hard Path scenario.
Similarly, with ENL scenario will require a total cost overrun at a discount of 12% of 10.2 Billion 2015USD in present value. In contrast, the Hard Path scenario would require an overall cost overrun of 3.5 Billion USD (Lallana Francisco et al., 2021). Studies from Nigeria show that decarbonization costs for the proposed three scenarios: Low-Carbon Moderate Scenario (LCM), Low-Carbon Advanced Scenario (LCA) and Green Optimistic Scenario (GO) are 36.62, 31.32, and 30.61 USD million, respectively, while LCA and GO scenarios were USD 31.32 and USD 30.61 million, respectively (Dioha et al., 2019). In executing the strategies with the LCM scenario, 2.73 USD, million can be saved, whereas 42 USD million can be saved in maintaining the policies in the household sector. Furthermore, extra funds will be spent on energy efficiency policy for the household sector in the LCA scenario.
From the review a constant theme that could be inferred is that decarbonizing the residential sector in Global South nations would necessitate considerable investments and governmental assistance to promote long-term economic, environmental, and social growth. Here are some of the economic impacts of decarbonization options in the residential sector which are applicable to Nigeria (Leung, 2018; Organi sation for Economic Co-operation and Development, 2022; Center on Global Energy Policy, 2023; United Nations, 2023):
4.2.9.1 Upfront costs
One major impediment to decarbonizing in the residential sector in Global South nations is the initial cost of investment. This is because decarbonizing the residential sector necessitates large initial investment in energy-efficient technologies, renewable energy systems, and sustainable waste management techniques.
4.2.9.2 Long payback periods
Payback periods for investments in energy-efficient technologies and renewable energy systems can be lengthy, discouraging households from making these purchases. To decrease this bottleneck and stimulate the adoption of these technologies, governments might offer incentives such as tax credits, subsidies, and net metering programs.
4.2.9.3 Job creation and local economy
The transition to cleaner energy sources and technologies has the potential to encourage local employment growth, particularly in the fabrication, installation, and maintenance of renewable energy systems and energy-efficient products. This has the potential to boost the local economy and lower unemployment rates.
4.2.9.4 Externalities and health costs
The economic benefits of decarbonizing the residential sector go beyond just monetary considerations. Cleaner technologies can reduce air pollution and improve public health outcomes, resulting in lower healthcare expenditures and a higher quality of life.
4.2.9.5 Split incentives
Which occur when the misalignment of costs and benefits between landlords and tenants impedes the adoption of energy-efficient technology and renewable energy systems, which frequently leads to underinvestment, particularly in the rental housing sector.
4.2.10 Economic implications for Nigeria
Decarbonizing Nigeria’s building sector involves both economic and societal factors. Lessons from different regions stress the high initial investment costs of energy-efficient devices. These upfront expenditures must be assessed in Nigeria against the potential benefits of local employment creation, improved public health, and a lower carbon impact.
4.3 Lessons learned for Nigeria from the review
This review offer valuable lessons for Nigeria’s journey towards decarbonizing the residential sector. The key lessons learned include:
4.3.1 Tailored approach for Nigeria
The findings highlight the importance of a targeted approach to addressing Nigeria’s emissions drivers. Given Nigeria’s distinctive characteristics, such as population, urbanization rate, and dwelling types, it is critical to establish specialized techniques that are contextually appropriate
4.3.2 Energy access and efficiency
Nigeria can learn from global South energy access and efficiency issues by promoting stable, cost-effective resources for homes, promoting energy-efficient appliances, and promoting renewable energy sources particularly in urbanizing areas to help reduce emissions.
4.3.3 Clean cooking initiatives
Recognizing the prevalence of inefficient and polluting cooking stoves in the regions reviewed, Nigeria can benefit from clean cooking initiatives, promoting LPG stoves and solar cookers to reduce emissions, improve indoor air quality, and public health.
4.3.4 Urbanization and land use
As a result of Nigeria’s fast urbanization, housing demand and its implications for energy use must be carefully considered. Learning from other regions, sustainable building techniques, and energy-efficient technologies can all help to reduce the environmental impact of urban growth and land use changes.
4.3.5 Policy framework
Lessons from decarbonization policy options in global south nations underscores the necessity of a strong regulatory framework. To design effective policy interventions adapted to its local context, Nigeria can learn from successful policies in other regions, such as energy efficiency standards, sustainable energy subsidies, and building codes.
4.3.6 Economic and social implications
This review emphasizes that initiatives to reduce carbon emissions have economic, environmental, and social components. Thus, Nigeria should be prepared for initial investment costs, but should also recognize the potential for local job creation, improved public health, and general economic growth connected with the transition to sustainable energy.
5 Discussion
5.1 Summary of study outcomes and implications
Climate change remains a global concern and must be tackled with all forms of adaptation procedures to reduce its impact. This review has endeavoured to methodically examine the current literature on the low-carbon building under deep decarbonization scenarios in global south regions with specific findings that will assist Nigeria in the ongoing deep decarbonization project. A rigorous review has resulted in 29 papers from three databases related to scenario-based studies, energy transition, and deep decarbonization. The results indicate that few studies addressed deep decarbonization in the global south. Still, most countries have only submitted their proposed NDCs.
Furthermore, 56 scenarios were considered, resulting in the identification of 62 emission drivers for the entire global south. Asia, Latin America, and Africa contributed about 30.65%, 41.94%, and 27.42% of the emission drivers, respectively. The significant drivers of emissions in Asia were population, GDP in Latin America, GDP, economic structure, and population. In Africa, dwelling characteristics, urbanization, and population were trending. Comparing the entire region, population growth and urbanization movement are trending. Ecological scarcities facilitate the Urbanization rate due to climate change as people can no longer sustain their livelihood in the rural communities. However, all the studied scenarios in the global south are very close as they address the following: 1) Increase in the use of clean and renewable energy in the building sector, 2) Development of more energy-efficient, emerging low carbon technology in the building sector and 3) Pursuit of green economy, through the circular economy and blue economy principles and activities. However, these objectives are far from archiving. The regions may lack adequate financial investment and stakeholders’ participation in the decarbonization process.
5.1.1 Specific findings related to developing low carbon building (LCB) in Nigeria
One of the specifics of this study was to apply the findings to assisting Nigeria in developing her scenarios in the ongoing DDP project since the study regions shear lateral economic development features with Nigeria. The total reviewed papers in Nigeria were seven (B5, B11, B12, B13, B16, and B18) Table 4. The study shows that the energy use in the Nigerian building sector is expansive and heterogeneous across the regions. Thus Nigeria will require a robust or ambitious scenario to achieve the needed decarbonization targets and pursue the intended green economy. The following were lacking in the Nigeria study. However, if implemented will be in line with the IPCC guidelines to avoid double counting.
1. Lack of multi-criteria decision analysis
2. Lack of economic options for chosen pathways
3. Non-inclusion of household purchasing power
4. The gap between building performance and modeling
5. Lack of building envelopes and codes
6. Emission saving potentials from chosen pathways or renewable options penetration
7. Under the building sector, data for the commercial sector are scare
8. Non-inclusion of climate factors like temperature. This factor is essential, especially in Nigeria, with different weather variations and heterogeneous energy demands.
9. The level of education should be an inclusion factor based on Nigeria’s peculiarities.
10. Unemployment rate
11. CO2 decoupling analysis is imperative and should be based on macroeconomic indicators.
5.1.2 Building Nigeria’s decarbonization pathway for the residential sector
Based on the literature reviewed valuable contributions to guide Nigeria in developing effective decarbonization strategies for its residential sector can be inferred. These insights can strengthen policies for Nigeria’s residential sector and they include:
5.1.2.1 Energy efficiency standards
Nigeria government must enforce strict energy efficiency standards for appliances, buildings, and equipment. This will engender the use of energy efficient technologies and reduce consumption and associated emissions;
5.1.2.2 Building codes
Enforcement of building codes requiring new constructions and renovations to adhere to laid down energy-efficient and sustainable design principle.
5.1.2.3 Financial incentives
Incentivize households to invest in energy-efficient appliances, solar panels, and renewable systems with the aid of subsidies and tax credits;
5.1.2.4 Net metering
Implementing net metering policies which allow for sale of excess renewable energy by households back to the grid will encourage renewable energy adoption and aid decarbonization;
5.1.2.5 Awareness campaigns
There is need to launch public awareness campaigns to educate households on the benefits of energy efficiency and renewable energy, as well as increase knowledge on energy efficient technology alternatives with the goal of encouraging behavioral changes and technological adoption;
5.1.2.6 Rural electrification
There is need to improve rural energy access with off-grid renewable solutions so as to reduce reliance on the consumption of traditional biomass fuels.
5.1.2.7 Research and development
Invest in research and development for energy-efficient residential buildings.
5.1.2.8 Capacity building
This entails building a solid crop of professionals from the local population capable of installing, maintaining and operating energy-efficient systems;
5.1.2.9 Regulatory framework
A legal backing for a comprehensive regulatory framework describing the duties and responsibilities of various stakeholders and the process of implementing decarbonization measures.
5.1.2.10 Data collection and monitoring
Develop robust data systems to track energy consumption, emissions reduction, and policy effectiveness, which will aid in enabling evidence-based decision-making in the residential sector.
5.1.3 General gaps in the literature review and future research
This systematic literature review (SLR) indicates that despite the increasing number of literature regarding low carbon energy scenarios of different economic sectors, specific studies or standalone studies on deep decarbonization of the entire building sector are lacking. Studies have concentrated on the residential sector, scarcely on the commercial sector. At the same time, the building construction industry and building material structure are with little concern. Thus, there seem to be gaps in the literature that requires improvement. However, only eight studies examined the commercial sectors, not as a standalone commercial sector. Furthermore, the studies did not disaggregate the specific drivers from the residential sector. The energy consumption patterns for the two sectors are not the same, and thus this could be misleading.
Additionally, in analyzing the sectors, only two studies consider energy consumption per floor area and dwelling characteristics. Figure 7 shows that the two drivers contributed about 5% to the entire region’s emission, indicating that the value will be higher for a specific country. The latter should be paramount, especially in developing economies without uniformity in building architecture. Conversely, no study considered the building construction industry. The global contribution of CO2 emissions from the building construction industry in 2019 was 10% (IEA, 2020). This is worrisome as the sector’s carbon emissions have recently increased.
Similarly, only one study examined building codes and building envelopes. Thus research should concentrate on this aspect to ascertain the quantitative impact of building envelope on achieving efficiency and decarbonization. Finally, the study included climatic factors (Temperature variations) across locations; this may be very necessary for areas with temperature variations as this will affect energy demand positively or negatively.
5.1.4 Enabling low-carbon infrastructure amidst barriers
Studies on the political economy of infrastructure (Bielenberg et al., 2016) conclude that many of the problems in the decision-making process around investments in infrastructure generally (cities) are circumscribed by a lack of solid institutions where interest groups, experts, politicians, and representatives of local communities are not informed or included in discussions about policy options for such investment. In transiting to low carbon building infrastructure, there is a need for a policy of total inclusion and full participation in the decision process. However, developing economies will face numerous additional barriers: weak governance, lack of expertise, poor revenue base, weak regulatory frameworks, poor credit, lack of political will, and currency and macroeconomic risk. The government will need a long-term budgeting plan for these uncertainties, establish technical institutions, and make effective policies backed by laws. Additionally, it is not easy to transition to low-carbon building without the high investment and involvement of the private sector. Nonetheless, this may not occur without the public sector’s lively participation. The public sector is responsible for providing part of the investment, framework, and policies needed to secure the process and make it attractive (Intergovernmental Panel on Climate Change, 2014).
6 Conclusion
This article presents a systematic literature review on the decarbonisation of the building sector in selected global south countries—A case for Nigeria to provide the previous and current status of research on low carbon building under deep decarbonization scenarios in the global south with specific finds to assist the Nigeria DDP project. Sixty-two emission drivers from the building sector were identified based on analysis from fifty-six scenarios that were observed across the study regions. The scenarios were focused on addressing the following: the increase in clean and renewable energy utilization in the building sector, the development of efficient and low carbon technologies in the sector, and achieving a green economy through deep decarbonization of the building sector. The aftermaths of the research indicate that the critical emission drivers were population, GDP, economic structure, dwelling characteristics, and urbanization. Suggestions were made for further study. Findings that will assist the Nigerian model included multi-criteria decision analysis and inclusion of household purchasing power, building envelopes, building codes, and unemployment. Finally, the building construction sector was a concern as there was limited studies that considered the inclusion of this sector.
Data availability statement
The original contributions presented in the study are included in the article/Supplementary Material, further inquiries can be directed to the corresponding author.
Author contributions
FA: Writing–original draft, Conceptualization, Formal Analysis, Methodology. CN: Formal Analysis, Writing–original draft, Methodology, Data curation. NE: Data curation, Formal Analysis, Writing–original draft, Software. CO: Data curation, Software, Visualization, Writing–review and editing. OD: Data curation, Software, Writing–review and editing, Formal Analysis, Resources. AO: Writing–review and editing, Funding acquisition, Supervision, Validation. KO: Formal Analysis, Resources, Visualization, Writing–review and editing. DS: Writing – original draft, Writing – reviewing and editing, Interpretation of data, Methodology, Data curation and Visualization and Funding Acquisition J-SH: Writing – original draft, Writing – reviewing and editing, Interpretation of data, Data curation and Visualization and Funding Acquisition
Funding
This work was supported by the National Research Foundation of Korea (NRF), a grant funded by the Korean government Ministry of Science and ICT (MSIT) (No. NRF-2021R1A5A8033165); the Korea Institute of Energy Technology Evaluation and Planning (KETEP) and the Ministry of Trade, Industry & Energy (MOTIE) of the Republic of Korea (No. 20224000000150).
Conflict of interest
The authors declare that the research was conducted in the absence of any commercial or financial relationships that could be construed as a potential conflict of interest.
Publisher’s note
All claims expressed in this article are solely those of the authors and do not necessarily represent those of their affiliated organizations, or those of the publisher, the editors and the reviewers. Any product that may be evaluated in this article, or claim that may be made by its manufacturer, is not guaranteed or endorsed by the publisher.
References
Africancityenergy (2015). Energy scenarios for cape town, South Africa. SAMPET PROJECT. https://www.africancityenergy.org/getfile.php?id=121&category=1.Accessed (August 24, 2021).
Akinwale, Y. O. (2017). Descriptive analysis of building indigenous low-carbon innovation capability in Nigeria. Afr. J. Sci. Technol. Innovation Dev. 10, 601–614. doi:10.1080/20421338.2017.1366131
Ali, G., Abbas, S., Pan, Y. C., Chen, Z. M., Hussain, J., Sajjad, M., et al. (2019). Urban environment dynamics and low carbon society: multi-criteria decision analysis modeling for policy makers. Sustain. Cities Soc. 51, 101763. doi:10.1016/j.scs.2019.101763
Bashir, S., Ahmad, I., and Ahmad, S. R. (2018). Low-emission modeling for energy demand in the household sector: A study of Pakistan as a developing economy. Sustainability 10, 3971. doi:10.3390/su10113971
Bataille, C., Waisman, C. B. H., Briand, Y., Adrien, J. S., Vogt-Schilb, M. J., Delgado, R., et al. (2020). Net-zero deep decarbonization pathways in Latin America: challenges and opportunities. Energy Strategy Rev. 30, 100510. doi:10.1016/j.esr.2020.100510
Bielenberg, A., Kerlin, M., Oppenheim, J., and Roberts, M. (2016). Financing change: How to mobilize private sector financing for sustainable infrastructure. New York, NY, USA: McKinsey Center for Business and the Environment.
Brounen, D., Kok, N., and Quigley, J. M. (2012). Residential energy use and conservation: economics and demographics. Eur. Econ. Rev. 56, 931–945. doi:10.1016/j.euroecorev.2012.02.007
Buira, D., Tovilla, J., Farbes, J., Jones, R., Haley, B., and Gastelum, D. (2021). A whole-economy deep decarbonization pathway for Mexico. Energy Strategy Rev. 33, 100578. doi:10.1016/j.esr.2020.100578
Cardoso, M. B., and González, A. D. (2019). Residential energy transition and thermal efficiency in an arid environment of northeast Patagonia, Argentina. Energy Sustain. Dev. 50, 82–90. doi:10.1016/j.esd.2019.03.007
Center on Global Energy Policy (2023). Decarbonizing the global buildings sector: efficiency, electrification, and equity. Center on global energy policy. https://www.energypolicy.columbia.edu/publications/decarbonizing-the-global-buildings-sector-efficiency-electrification-and-equity/.
Chaturvedi, V., Eom, J., Clarke, L. E., and Shukla, P. R. (2014). Long term building energy demand for India: disaggregating end use energy services in an integrated assessment modeling framework. Energy Policy 64, 226–242. doi:10.1016/j.enpol.2012.11.021
City of Austin (2015). Austin community climate plan. http://austintexas.gov/sites/default/files/files/Sustainability/OOS_AustinClimatePlan_032915_SinglePages.pdf (Accessed November 17, 2021).
Climatewatchdata (2021). Climate watch data. https://www.climatewatchdata.org/ghg-emissions.
Commerce (2022). Impacts-of-Decarbonization-Pathways-Modeling. https://www.commerce.wa.gov/wp-content/uploads/2021/01/Appendix-E-Economic. Accessed February 17.
Daioglou, V. (2010) Residential energy use scenarios M.Sc Thesis. Utrecht, Netherlands: Utrecht University, 1–163.
Daioglou, V., Ruijven, B. J. V., and V Vuuren, D. P. (2012). Model projections for household energy use in developing countries. Energy 37, 601–615. doi:10.1016/j.energy.2011.10.044
Dakwale, V. A., and Ralegaonkar, R. V. (2012a). Review of carbon emission through buildings: threats, causes and solution. Int. J. Low-Carbon Technol. 7 (7), 143–148. doi:10.1093/ijlct/ctr032
Dakwale, V. A., and Ralegaonkar, R. V. (2012b). Review of carbon emission through buildings: threats, causes and solution. Int. J. Low-Carbon Technol. 7, 143–148. doi:10.1093/ijlct/ctr032
Dioha, M. O., Emodi, N. V., and Dioha, E. C. (2019). Pathways for low carbon Nigeria in 2050 by using NECAL2050. Renew. Energy Focus 29, 63–77. doi:10.1016/j.ref.2019.02.004
Dioha, M. O., and Emodi, N. V. (2019). Investigating the impacts of energy access scenarios in the Nigerian household sector by 2030. Resources 8, 127. doi:10.3390/resources8030127
Dioha, M. O. (2018). Modelling the impact of Nigeria household energy policies on energy consumption and CO2 emissions. Eng. J. 22 (6), 1–19. doi:10.4186/ej.2018.22.6.1
Ekholm, T., Krey, V., Shonali, P., and Riahi, K. (2010). Determinants of household energy consumption in India. Energy Policy 38, 5696–5707. doi:10.1016/j.enpol.2010.05.017
Emodi, N. V., Emodi, C., Murthy, G. P., and Emodi, A. S. A. (2017). Energy policy for low carbon development in Nigeria: A LEAP model application. Renew. Sustain. Energy Rev. 68, 247–261. doi:10.1016/j.rser.2016.09.118
Emodi, N. V., Lovell, H., Clinton Levitt, C., and Franklin, E. (2021). A systematic literature review of societal acceptance and stakeholders’ perception of hydrogen technologies. Int. J. hydrogen energy 46, 30669–30697. doi:10.1016/j.ijhydene.2021.06.212
Eom, J., Clarke, L., Kim, S. H., Kyle, P., and Patel, P. (2012a). China's building energy demand: long-term implications from a detailed assessment. Energy 46 (1), 405–419. doi:10.1016/j.energy.2012.08.009
Eom, J., Clarke, L., Kim, S. H., Kyle, P., and Patel, P. (2012b). China's building energy demand: long-term implications from a detailed assessment. Energy 46 (1), 405–419. doi:10.1016/j.energy.2012.08.009
Ford, J. D., Berrang-Ford, L., and Paterson, J. (2011). A systematic review of observed climate change adaptation in developed nations. Clim. Change 106, 327–336. doi:10.1007/s10584-011-0045-5
Ford, J. D., and Pearce, T. (2010). What we know, do not know, and need to know about climate change vulnerability in the western Canadian arctic: A systematic literature review. Environ. Res. Lett. 5 (014008), 014008. doi:10.1088/1748-9326/5/1/014008
Ghedamsi, R., Settou, N., Gouareh, A., Khamouli, A., Saif, N., Recioui, B., et al. (2016). Modeling and forecasting energy consumption for residential buildings in Algeria using bottom-up approach. Energy Build. 121, 309–317. doi:10.1016/j.enbuild.2015.12.030
Groves, D., James, S., Molina-Perez, E., Calvo, C., Víctor- Gallardo, L., Guido, G-Z., et al. (2020). The benefits and costs of decarbonizing Costa Rica’s economy. https://www.rand.org/pubs/research_reports/RRA633-1/visualization.html.
Gul, M., and Qureshi, W. A. (2013). Long term electricity demand forecasting in residential sector of Pakistan. IEEE, 1–7. doi:10.1109/PESGM.2012.6512285
Güneralp, B., Zhou, Y., Ürge-Vorsatz, D., Gupta, M., Yu, S., Patel, P. L., et al. (2017). Global scenarios of urban density and its impacts on building energy use through 2050. Proc. Natl. Acad. Sci. 114 (34), 8945–8950. doi:10.1073/pnas.1606035114
Guo, S. D., Yan, S., Hu, Y., and Zhang, (2021). Modelling building energy consumption in China under different future scenarios. Energy 214 (1), 119063. doi:10.1016/j.energy.2020.119063
Ibitoye, F. I. (2013). The millennium development goals and household energy. Berlin, Germany: Springer.
IEA (2020). Global share of buildings and construction final energy and emissions 2019. https://www.iea.org/reports/world-energy-outlook-2020.
IEA, 2023 Sustainable buildings centre. International energy agency (IEA). Available from: http://www.sustainablebuildingscentre.org/.
IEA (2013). Tracking clean energy progress 2013. https://www.iea.org/reports/tracking-clean-energy-progress-2013.
Intergovernmental Panel on Climate Change (2014). Climate change 2014: Mitigation of climate change: Working group III contribution to the fifth assessment report of the intergovernmental panel on climate change. Cambridge, England: Cambridge University Press.
IPCC (2018). Global Warming of 1.5°C.An IPCC Special Report on the impacts of global warming of 1.5°C above pre-industrial levels and related global greenhouse gas emission pathways. https://www.ipcc.ch/site/assets/uploads/sites/2/2019/06/SR15_Full_Report_High_Res.pd.
Ismail, N. A., Rahman, M. M., Abuelnour, M. A., Jabbar, I., and Saat, A. “Long term energy development pathways for Nigeria: A scenario based analysis,” in Proceedings of the International Conference on Industrial Engineering and Operations Management, Dubai, UAE, March 2020.
Johansson, T. B., Nakicenovic, N., Patwardhan, A., Gomez-Echeverri, L., Arent, D. J., Banerjee, R., et al. (2012). Global energy assessment: toward a sustainable future. Accessed http://www.iiasa.ac.at/web/home/research/Flagship-Projects/Global-Energy Assessment/GEA-Summary-web.pdf.
Kumar, M. (2020).An analysis of optimal and near-optimal decarbonisation strategies for Malaysia using a hybrid model Doctoral thesis (Ph.D). London: UCL University College. https://discovery.ucLAc.uk/id/eprint/10107834/.
Kyle, P., Clarke, L., Rong, F., and Smith, S. J. (2010). Climate policy and the long-term evolution of the U.S. Buildings Sector. Energy J. 31 (2), 145–172. doi:10.5547/issn0195-6574-ej-vol31-no2-6
Lallana, F., Bravo, G., Treut, G. L., Julien, L. E., Nadal, G., and Nicol, D. S. (2021a). Exploring deep decarbonization pathways for Argentina. Energy Strategy Rev. 36, 100670. doi:10.1016/j.esr.2021.100670
Lallana, Francisco, Bravo, Gonzalo, Le Treut, Ga¨elle, Julien, Lefevre, Nadal, Gustavo, and Sbroiavacca, Nicolas Di (2021b). Exploring deep decarbonization pathways for Argentina. Energy Strategy Rev. 36, 100670. doi:10.1016/j.esr.2021.100670
Lèbre, L. R., Claudio, G. E. G., and William, W. (2015). Pathways to deep decarbonization in Brazil. http://deepdecarbonization.org/wp-content (Accessed October 8, 2021).
Leibowicza, B. D., Lanhamb, C. M., Brozynskia, M. T., Vázquez-Cantelic, J. R., Castillo, N. C., and Nagyc, C. (2018a). Optimal decarbonization pathways for urban residential building energy services. Appl. Energy 230, 1311–1325. doi:10.1016/j.apenergy.2018.09.046
Leibowicza, D. B., Lanhamb, M. C., Brozynskia, T. M., Vázquez-Cantelic, R. J., Nicolás, C. C., and Nagy, Z. (2018b). Optimal decarbonization pathways for urban residential building energy services. Appl. Energy 230, 1311–1325. doi:10.1016/j.apenergy.2018.09.046
Leung, J. (2018). Climate innovation 2025. https://www.c2es.org/document/decarbonizing-u-s-buildings/.
Martinot, E. (2012). Renewables 2012 global status report. https://www.ren21.net/Portals/0/documents/Resources/GSR2012_low%20res_FINAL.pdf.
Moher, D., Liberati, A., Tetzlaff, J., and Altman, D. G. (2009). The PRISMA group. Preferred reporting items for systematic reviews and meta-analyses: the PRISMA statement. PLOS Med. 6 (7), 6. doi:10.1136/bmj.b2535
Nejat, P., Jomehzadeh, F., Taheri, M. M., Gohari, M., and Muhd, M. Z. (2015). A global review of energy consumption, CO 2 emissions and policy in the residential sector (with an overview of the top ten CO 2 emitting countries). Renew. Sustain Energy Rev. 43, 843–862. doi:10.1016/j.rser.2014.11.066
Newton, P. W., and Tucker, N. S. (2011). Pathways to decarbonizing the housing sector: A scenario analysis. Build. Res. Inf. 39 (1), 34–50. doi:10.1080/09613218.2010.531085
Organisation for Economic Co-operation and Development (2022). Building decarbonisation in cities and regions. Organisation for Economic Co-operation and Development. https://www.oecd.org/cfe/cities/energy-efficiency-cities.htm.
Pilkington, B., Roach, R., and Perkins, J. (2011). Relative benefits of technology and occupant behaviour in moving towards a more energy efficient, sustainable housing paradigm. Energy Policy 39, 4962–4970. doi:10.1016/j.enpol.2011.06.018
Planning Commission (2011). Low Carbon strategies for inclusive growth. New Delhi, India: Government of India.
REN21 (2020). Renewables 2020 global status report 2020. Available online: http://www.ren21.net/ (accessed on May 20, 2020).
Ruijven, B. J. V., Detlef, P. V., Vries, B. J. M., Isaac, M., Sluijs, J. P. V., Lucas, P. L., et al. (2011). Model projections for household energy use in India. Energy Policy 39, 7747–7761. doi:10.1016/j.enpol.2011.09.021
Sandu, S., Yang, M., Mahlia, I. M. T., Wongsapai, W., Ong, C. H., Putra, N., et al. (2019). Energy-related CO2 emissions growth in ASEAN countries: trends, drivers and policy implications. Energies 12, 4650. doi:10.3390/en12244650
Shaffril, H. A. M., Krauss, S. E., and Samsuddin, S. F. (2018). A systematic review on Asian's farmers' adaptation practices towards climate change. Sci. Total Environ. 644, 683–695. doi:10.1016/j.scitotenv.2018.06.349
Shi, J., Chen, W., and Yin, X. (2016). Modelling building's decarbonization with application of China TIMES model. Appl. Energy 162, 1303–1312. doi:10.1016/j.apenergy.2015.06.056
Shi, J., Chen, W., and Yin, X. (2015). Modelling building’s decarbonization with application of China TIMES model. Appl. Energy 162, 1303–1312. doi:10.1016/j.apenergy.2015.06.056
Shukla, Y., Rawal, R., and Shnapp, S.“Residential buildings in India: energy use projections and savings potentials,” in Proceedings of the European Energy Efficiency Conference, Club Belambra Les Criques, Presqu’île de Giens, June 2015.
Sierra-Correa, P. C., and Kintz, J. R. C. (2015). Ecosystem-based adaptation for improving coastal planning for sea-level rise: A systematic review for mangrove coasts. Mar. Policy 51, 385–393. doi:10.1016/j.marpol.2014.09.013
UNEP (2017). The emissions gap report 2017. Nairobi: United Nations Environment Programme UNEP. available at www.unenvironment.org/resources/emissions-gap- report.
United Nations Framework Convention on Climate Change (2019). COMMIT project (Climate policy assessment and Mitigation Modeling to integrate national and global Transition pathways. Long-term, Low-emission Pathways in Australia, Brazil, Canada, China, EU, India, Indonesia, Japan, Republic of Korea, Russia, and United States. http://unfccc.int/focus/long-term_strategies/items/9971.php. February 2019 COMMIT.
United Nations (2023). Key priorities in charting decarbonization pathways in least developed countries. https://unctad.org/system/files/official-document/aldc2022d10_en.pdf.
Vaillancourt, K., Bahn, O., Frenette, E., and Sigvaldason, O. (2017). Exploring deep decarbonization pathways to 2050 for Canada using an optimization energy model framework. Appl. Energy 195, 774–785. doi:10.1016/j.apenergy.2017.03.104
Villamar, D., Soria, R., Imperio, M., Carvajal, P., Rochedo, P., Szklo, A., et al. “Efficiency and affordability: new energy markets in latin america,” in Proceedings of the 7th ELAEE Buenos Aires 2019 Decarbonization, Buenos Aires, Argentina, March 2019.
Wada, K., Akimoto, K., Sano, F., Oda, J., and Homma, T. (2012). Energy efficiency opportunities in the residential sector and their feasibility. Energy 48, 5–10. doi:10.1016/j.energy.2012.01.046
Winyuchakrit, P., Limmeechokchai, B., Matsuoka, Y., Gomi, K., Kainuma, M., Fujino, J., et al. (2011). Thailand's low-carbon scenario 2030: analyses of demand side CO2 mitigation options. Energy Sustain. Dev. 15, 460–466. doi:10.1016/j.esd.2011.09.002
World Energy Council (2016). “World energy scenarios 2016 – the grand transition,” in Collaboration with accenture Strategy and the (Würenlingen, Switzerland: Paul Scherrer Institut). https://www.worldenergy.org/publications/entry/world-energy-scenarios-2016-the-grand-transition (Accessed November, 2021).
Worldometers (2021). CO2 emissions per capita. https://www.worldometers.info/co2-emissions/co2- emissions-per-capita/.
Zahraoui, Y., Khan, M. R. B., Ahmed, M., AlHamrouni, I., and Mekhilef, S. (2021). Current status, scenario, and prospective of renewable energy in Algeria: A review. Energies 14, 2354. doi:10.3390/en14092354
Keywords: residential sector, decarbonization, Nigeria, building sector, global south
Citation: Abam FI, Nwachukwu CO, Emodi NV, Okereke C, Diemuodeke OE, Owolabi AB, Owebor K, Suh D and Huh J-S (2023) A systematic literature review on the decarbonisation of the building sector—a case for Nigeria. Front. Energy Res. 11:1253825. doi: 10.3389/fenrg.2023.1253825
Received: 06 July 2023; Accepted: 04 September 2023;
Published: 19 September 2023.
Edited by:
Nallapaneni Manoj Kumar, City University of Hong Kong, Hong Kong SAR, ChinaReviewed by:
Gudina Terefe Tucho, Jimma University, EthiopiaAliyu Barau, Bayero University Kano, Nigeria
Copyright © 2023 Abam, Nwachukwu, Emodi, Okereke, Diemuodeke, Owolabi, Owebor, Suh and Huh. This is an open-access article distributed under the terms of the Creative Commons Attribution License (CC BY). The use, distribution or reproduction in other forums is permitted, provided the original author(s) and the copyright owner(s) are credited and that the original publication in this journal is cited, in accordance with accepted academic practice. No use, distribution or reproduction is permitted which does not comply with these terms.
*Correspondence: Jeung-Soo Huh, anNodWhAa251LmFjLmty