- Forest Products Innovation Center of Excellence, Ethiopia Forestry Development, Addis Ababa, Ethiopia
Understanding the potential of biomass sources and their types, quality, and regional distribution is crucial for modern energy production. This study evaluates the biomethane energy potential of livestock waste from six different categories of livestock across all Ethiopian regions using livestock statistics (2020–21), standard procedures, publicly accessible data, and literature. To evaluate the bioenergy potentials, the amount of dry matter per head, collection efficiency, biogas yields of respective livestock waste, and the calorific value of biogas and biomethane were used. The total biomethane and corresponding bioenergy potential of livestock residues in Ethiopia are estimated to be 3,321 hm3 y-1 and 118,906 TJ y-1, respectively. The biomethane combustion in a combined heat and power system is expected to produce roughly 11.4 TWh y-1 of electricity and 13.9 TWh y-1 of thermal energy. The estimated electrical energy could supply 11%, 31%, and 81% of Ethiopia’s total primary energy consumption, production, and total electricity generated in Ethiopia in 2019, respectively. The finding demonstrates that biomethane-based energy production via anaerobic digestion based on livestock waste has the potential to meet a significant portion of Ethiopia’s current energy needs.
1 Introduction
Ethiopia has the fastest economic growth in the region, with 6.3% growth in FY 2020/21, and is the second most populous nation in Africa after Nigeria (World Bank, 2022). Ethiopia, however, has one of the lowest levels of energy supply and consumption per capita (Yalew and Woldie, 2021). Compared to the global average of 1.9 toe (ton oil equivalent), the primary energy supply per person is approximately 0.4 toe, while the primary energy consumption per person is roughly 0.07 toe (EIA, 2021; Yalew and Woldie, 2021). The low energy consumption per capita was attributed to low energy access in Ethiopian regions, as indicated by the relatively low electricity access of approximately 51.09% of the entire population in Ethiopian regions in 2020 (Our World in Data, 2022a; Macrotreds, 2022), with a 3.03% increase from 2019 (Macrotreds, 2022). The percentage in rural areas was 39.4%. In 2020, approximately 7.80% of the Ethiopian population had access to clean cooking fuels or technologies (Our World in Data, 2022a). Clean cookstoves are one example of a clean technology that can help minimize exposure to indoor air pollution, a major cause of death in low-income households. Several Ethiopian regions rely heavily on renewable energy sources to supply their energy needs, especially bioenergy in the form of solid biofuels like fuelwood, charcoal, and agricultural waste. This is demonstrated by the comparatively high share of renewable energy sources (including biomass, wind, solar, and geothermal) in Ethiopia’s actual total energy supply, which was approximately 88% in 2019 (IRENA, 2022). The hydro and marine industries supplied over 96% of the electricity generation in 2020 (IRENA, 2022). More than 90% of households cook with solid biomass fuels, mainly fuelwood, as cited in the work of Yalew and Woldie (2021). This leads to indoor air pollution (IAP), which in 2016 caused 3 million disability-adjusted life years (DALYs), 65 thousand premature deaths (Global Health Observatory, 2016), and 5% of all disease burden in Ethiopia (Sanbata, Asfaw, and Kumie, 2014). Due to the negative effects of IAP on human health and the environment as well as other factors (such as the nation’s rapid rates of urbanization, industrialization, and population growth), Ethiopia needs an energy system that is adaptable, modern, dependable, and affordable in order to meet its country’s rising energy needs (PDC, 2021).
Traditional solid biofuel burning for cooking, such as the use of fuelwood, is not a sustainable practice because of the negative effects on human health and the environment caused by air pollution. All the aforementioned problems are caused by the lack of modern, clean energy facilities. The use of biomass resources helps alleviate the problems if appropriate technology is used. In addition to energy production, biomass has many other applications (Li et al., 2020; Tolessa et al., 2020; Cao et al., 2021; Meng et al., 2023). The use of anaerobic digestion (AD) technology could be among the best ways to produce clean and renewable energy (like biogas) from organic biomass sources, including livestock waste. Biogas produced via AD processes consists primarily of methane (CH4) (40%–75%) and carbon dioxide (CO2) (15%–60%) (Al Mamun and Torii, 2015; Tolessa, 2022) (see Figure 1). AD technology has been recognized as one of the most important sources of clean and renewable energy that can improve the livelihoods of low-income rural societies, particularly in developing countries (Tolessa et al., 2020).
Numerous Ethiopian regions are recently implementing AD at the household level to meet their energy needs as a more sustainable solution. Since 2009, the National Biogas Program of Ethiopia (NBPE) has been in operation in the country (SNV, 2022). The initiative began in Ethiopia’s Tigray, Oromia, Amhara, and Southern Nations and Nationalities and Peoples’ (SNNP) regions before expanding to nine regions in 2017 with the addition of the Benishangul Gumuz, Afar, Somali, Gambella, and Sidama regions. The first two phases of the program (NBPE I and NBPE II) were funded by DGIS and the Ethiopian government, while the current program, the Biogas Dissemination and Scale-Up Programme of Ethiopia (NBPE+), is supported by the European Union (EU) and the Ethiopian government through the Ministry of Finance. The NBPE+ was developed and entered its implementation phase in 2017.
With a target of 36,000 installations of household-size and 40 larger-size digesters during a 63-month implementation period, the NBPE+ is operating in nine regions of Ethiopia (except Addis Ababa, Dire Dawa, and Harari) (SNV, 2022). These domestic biodigesters use animal wastes, human wastes (or domestic wastewater), agricultural residues, and food wastes as biomass feedstock for producing biogas (Roopnarain and Adeleke, 2017; Clemens et al., 2018). The generated biogas is then used as cooking fuel, providing a safer, healthier alternative to domestic fuelwood burning while simultaneously improving energy availability and security in many of these Ethiopian regions. Despite the enormous potential for producing biogas from a variety of substrates, including animal manure, municipal solid wastes, and agricultural residues (crop residues and animal manure) in Ethiopian regions, anaerobic digestion is not widely used in Ethiopia as expected, despite its small-scale presence (Rupf et al., 2016), compared to other low-to-middle-income countries (Mengistu et al., 2015; Tucho et al., 2016; Berhe et al., 2017). Among these biomass resources, livestock effluents will be the focus of this study, as this feedstock represents an abundant source of untapped bioenergy in Ethiopian regions. According to estimates, Ethiopia has Africa’s largest population of livestock (Solomon et al., 2003; Tilahun and Schmidt, 2012; Leta and Mesele, 2014). The livestock subsector makes a significant contribution to the national economy of Ethiopia and serves as the means of subsistence for many Ethiopians. Approximately 16.5% of the nation’s GDP and 35.6% of the agricultural GDP are contributed by the subsector (Leta and Mesele, 2014).
Understanding the potential of biomass sources is crucial for developing sustainable energy strategies and planning the use of biomass waste. Moreover, knowing where key feedstocks are expected to be available and understanding their types, quality, and energy potential can help determine the best sites to construct potential renewable biomass energy plants. Although there are many biogas models (fixed dome, floating drum, and balloon/plastic models) installed in Ethiopia, there are limited studies on the potential of Ethiopia’s biomass resources for producing biomethane (Tolessa, 2023b). Although some authors have studied the topic of biomass in Ethiopia, most of them have not assessed the potential of Ethiopia’s biomass resources for producing biomethane, in particular. The few studies that did consider this focused on specific chosen residues and/or locations (Tucho et al., 2017). For example, a recent study by Tolessa (2023a) focused primarily on crop residues for the production of bioenergy. Another study by Gabisa and Gheewala (2018) focused on estimating the bioenergy potential from various biomass residue sources, including the bioenergy potential from livestock; however, it only looked at the total bioenergy potential rather than the biomethane energy potential. Furthermore, studies on biomass resources for use in alternative and renewable energy applications were also undertaken in the country by Guta (2012) and Tucho et al. (2015). However, according to Mohammed, Atnaw, and Desta (2022), many other published papers in the country on biogas and renewable energy primarily examine the environmental advantages, adoption drivers, prospects, and socioeconomic and technical hurdles of Ethiopian biogas technology.
To the authors’ knowledge, the potential availability of a variety of livestock wastes for biomethane-based energy production in all the Ethiopian regions has not yet been reported. Therefore, the purpose of this study is to determine whether the biomass resources from livestock waste in Ethiopia’s regions are sufficient to make AD a feasible and sustainable technology. The goal of the study is to estimate and quantify the potential availability of livestock waste biomass feedstock resources that can be recovered and used to produce biomethane energy as well as the total potential for bioenergy, electrical energy, and thermal energy.
Overall, this study provides an assessment of the potential for the generation of biomethane from widely accessible livestock effluents produced by cattle, sheep, goats, horses, donkeys, and poultry in all regions of Ethiopia to address the data gap in the literature. The data gaps for bioenergy resources in Ethiopia include the availability and amount of each livestock category waste biomass feedstock resource and its regional distributions, as well as the corresponding bioenergy potential. The estimated bioenergy potential at the national, regional, and livestock category levels in this study is expected to aid policy decisions and regional bioenergy planning in the country. This study provides a baseline evaluation of the livestock residue biomass resource potential for bioenergy production. Such a baseline helps in identifying the biomass resources from animals that are still underutilized for the production of bioenergy. A comparable baseline can be established for other places in the country and elsewhere to quantify the bioenergy production potential from locally available biomass resources. As a foundation for future research and policy planning, this study may also assist investors and decision makers in maximizing the use of locally accessible resources derived from livestock residual biomass.
2 Methodology
For the current study, locally available livestock production data in Ethiopian regions were obtained from the Ethiopian Central Statistical Agency (CSA) (CSA, 2020) and used to estimate the potential of animal waste biomass resources across the country for energy production via anaerobic digestion technology. Manure waste from six types of farm animals was considered for the assessment. Livestock waste is an important component in the generation of biogas owing to its composition. The generation of livestock waste differs from country to country and from region to region in a country, owing to variations in animal weight and size and feed intake (Maithel, 2009; Mboumboue and Njomo, 2018).
Excreta produced by cattle, sheep, goats, horses, donkeys, and poultry were considered to estimate the energy potential of livestock effluents. Estimation of accurate values of livestock effluent’s dry matter, recoverable fraction, and chemical and physical properties is difficult. Thus, significant variations exist in the values reported by several authors (Gabisa and Gheewala, 2018; Mboumboue and Njomo, 2018; Tolessa et al., 2020; 2021). The production of livestock waste varies from country to country and region to region due to differences in animal weight, body shape, and feed intake. Thus, the amount of waste collected can be influenced by the region’s environment. The estimation of various waste parameters should be as specific as possible in relation to the study region. However, both locally and globally, these statistics are rarely available. Therefore, due to a lack of local data relevant to the researched regions, various waste parameters linked to animal manure were collected from comparable prior studies/literature, and the average value for each residue type was employed in order to evaluate the energy potential of livestock waste. This study has also covered many studied specific areas for livestock waste in many nations across the world to reflect more agricultural and climatic situations. For estimating livestock effluent energy potential, some indicative values of the waste parameters relevant to animal waste, including daily volatile solid generation per livestock and biogas yield per unit of volatile solid, were collected from the literature, as presented in Section 3.2. Then, the average values were used to estimate the amount of biogas that can be generated by each livestock category as the biogas yield of a particular livestock category waste depends on the dry matter organic fraction in the livestock waste and the associated waste management system. The dry matter (DM) of the effluent refers to the matter left after moisture content removal and might be achieved as the weight loss on heating to a temperature of 105 °C, whereas volatile solids (VSs) refers to the organic fraction of dry matter in livestock effluent (UNEP, 2013).
Equation 1 (UNEP, 2013; Gabisa and Gheewala, 2018; Mboumboue and Njomo, 2018) is used to calculate the quantity of livestock effluent biomass that can be collected for energy application.
where LW is the amount of livestock waste that can be recovered for energy use (kg y−1);
The annual biogas potential of a particular livestock waste in a particular Ethiopian region was estimated using Equation 2, and then, the yearly biomethane potential was calculated by assuming that the methane content of the biogas is approximately 60% (Tolessa et al., 2020; Tolessa, Goosen, and Louw, 2021b; Tolessa et al., 2021).
where
The energy potential of the biogas and biomethane recoverable from the AD of livestock waste generated was estimated using the following two equations (UNEP, 2013):
where
After the combustion of the biomethane in a combined heat and power (CHP) system, the available energy consists of thermal energy (Etherm) and electrical energy (Eelec) and is estimated using the following equation (Lübken et al., 2007; Bundhoo, Mauthoor, and Mohee, 2016):
where
3 Results and discussion
3.1 Livestock residue biomass resource potential
Figure 2 and Figure 3 show that the most common livestock in the agricultural systems of Ethiopia is cattle (70.3 M; 30% of the total livestock), poultry (57.0 M; 24% of the total livestock), goat (52.5 M; 22% of the total livestock), sheep (42.9 M; 18% of the total livestock), donkey (10.8 M; 5% of the total livestock), and horse (2.1 M; 1% of the total livestock), respectively. Cattle, goats, and sheep account for approximately 70% of the total livestock reared in the country. These three most significant livestock species significantly contribute to the country’s GDP, according to the work of Metaferia et al. (2011). The top three regions that farmed livestock in the country are Oromia (68.1 M; 29%), Amhara (58 M; 25%), and Somale (34.8; 15%). At individual livestock type levels, cattle are the dominant animal in the country. Among Ethiopian regions that farmed with cattle, the highest number owned per region was (25.5 M; 36%) in Oromia, followed by Amhara (17.3 M; 25%) and the SNNP Region (11.1 M; 16%). Leta and Mesele (2014) conducted the spatial and regional distribution study and reported that Oromia is the greatest producer of livestock, followed by Amhara and the SNNP Region. A linear trend of growth was also evident in the 5 year’s annual livestock production. Based on this livestock production data, the biomass resource availability analysis of livestock waste was conducted at both regional and national levels. The gross and recoverable biomass potential, available biogas, and biogas energy potential of livestock effluents were estimated across all regions of Ethiopia in 2020/21. The total biomethane and total bioenergy potential, as well as the available energy after combustion in a CHP system for thermal and electrical energies, were also estimated for each livestock type across all regions, as detailed in the following.
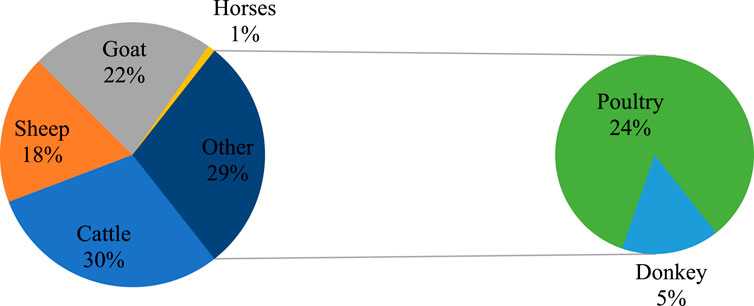
FIGURE 2. Percentage of livestock category in Ethiopia at the national level, drawn based on the data in Supplementary Table S1.
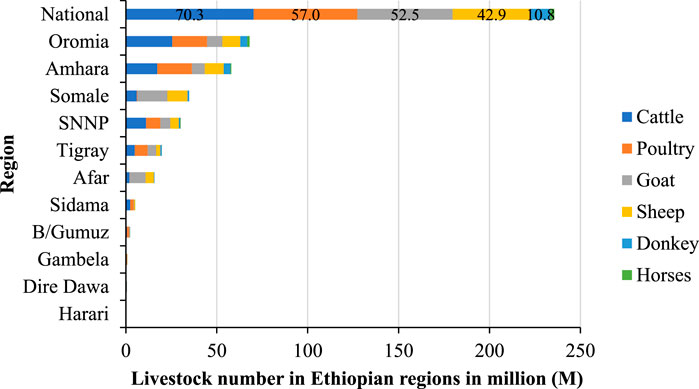
FIGURE 3. Comparison of the number of livestock in Ethiopia at national and regional levels, drawn based on the data in Supplementary Table S1.
3.2 Livestock residue biomass resource potential
3.2.1 Cattle
Over 70 million cattle were raised in Ethiopia in 2020/21 (CSA, 2020), with Oromia alone accounting for 36.3% of the cattle production in the country, followed by Amhara (24.6%) and the SNNP Region (15.8%). The average value of the reported amount of dry matter per head of cattle is approximately 1.86 kg d−1 h−1 (Y. S. Mohammed et al., 2013; Kemausuor et al., 2014; Shane, Gheewala, and Fungtammasan, 2016; Mboumboue and Njomo, 2018; Gabisa and Gheewala, 2018; Tolessa et al., 2021), with an average collection efficiency of approximately 43% (Bidart, Fröhling, and Schultmann, 2014; Kemausuor et al., 2014; Shane, Gheewala, and Fungtammasan, 2016; Gabisa and Gheewala, 2018; Tolessa et al., 2020). Due to the fact that animals in the country come from a variety of households and move across the area to graze in communal areas during the day and return to their respective areas at night, waste collection efficiency is poor (Gabisa and Gheewala, 2018). The volatile solid (VS) fraction of cattle waste has been previously studied, and the average volatile solid fraction reported is 0.93 kgVS kgDM−1 (Pereraa et al., 2005; UNEP, 2013; Mboumboue and Njomo, 2018; Tolessa, Goosen, and Louw, 2021a). Many studies have explored the AD of cattle waste due to its potential for the production of biogas. A biogas output on a VS of 0.20 m3 kg−1 was reported by Mboumboue and Njomo (2018) and UNEP (2015). According to Pereraa et al. (2005), dry cattle waste produced a biogas yield of 0.23 m3 kg−1. According to the information in Supplementary Table S2, Ethiopia could potentially produce 47,636 ktDM y−1 of gross biomass from cattle waste, of which 20,245 ktDM y−1 is recoverable. The amount of biogas recoverable and corresponding recoverable biogas energy from the AD of the cattle waste are estimated to be 3,962 hm3 y−1 and 79,247 TJ y−1, respectively, in Ethiopia. The total biomethane and total bioenergy potential from AD of cattle manure in Ethiopia are estimated to be 2,377 hm3 per year and 85,112 TJ y−1, respectively, corresponding to a total available energy of 65,196 TJ y−1 (35,747 TJ y−1 of thermal energy and 29,449 TJ y−1 of electrical energy). Oromia has the highest total biomethane and total bioenergy potential from livestock waste, as shown in Figure 4, followed by Amhara, the SNNP Region, Somale, and Tigray.
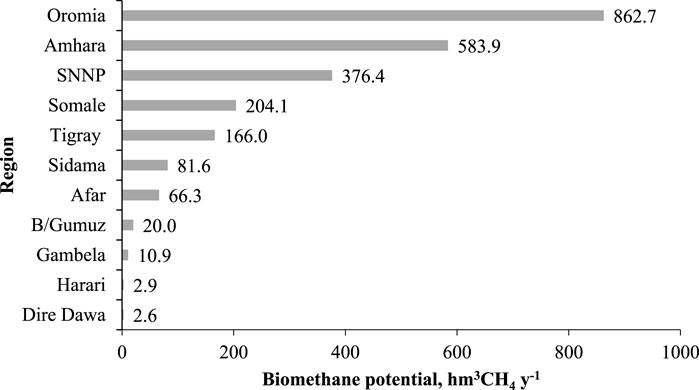
FIGURE 4. Biomethane production from cattle waste in Ethiopia, drawn based on the data in Supplementary Table S2.
3.2.2 Sheep
Sheep are raised mainly for meat, which is used as food for human consumption in Ethiopia. Approximately 43 million sheep were reared in Ethiopia in 2020/21, with Somale accounting for the highest number of sheep production (25.7%), followed by Amhara (24.2%) and Oromia (22.7%) (CSA, 2020). The amount of dry matter per head of sheep is reported to be 0.37 kg d−1 h−1 on average (Mohammed et al., 2013; Kemausuor et al., 2014; Shane, Gheewala, and Fungtammasan, 2016; Gabisa and Gheewala, 2018; Mboumboue and Njomo, 2018), with a collection efficiency of approximately 32% (Bidart, Fröhling, and Schultmann, 2014; Kemausuor et al., 2014; Shane, Gheewala, and Fungtammasan, 2016; Gabisa and Gheewala, 2018; Mboumboue and Njomo, 2018; Tolessa et al., 2021). The VS fraction of sheep waste has been previously studied, and the reported average volatile solid fraction is 0.91 kgVS kgDM−1 (UNEP, 2013; Mboumboue and Njomo, 2018). According to the information in Supplementary Table S3, following AD of sheep manure under mesophilic conditions, an average biogas yield of 0.28 m3 kg−1 has been reported in the literature (UNEP, 2013; Bidart, Fröhling, and Schultmann, 2014; Mboumboue and Njomo, 2018). The gross and recoverable biomass generated from sheep waste are estimated to be 5,819 ktDM y−1 and 1,852 ktDM y−1, respectively. Estimates for the amount of recoverable biogas and the associated biogas energy from AD of sheep waste in Ethiopia are 471 hm3 y−1 and 9,427 TJ y−1, respectively. The total biomethane and bioenergy potential of sheep manure are estimated to be 283 hm3 y−1 and 10,125 TJ y−1, respectively, in Ethiopia, corresponding to a total available energy of 7,755 TJ y−1 (4,252 TJ y−1 of thermal energy and 3,503 TJ y−1 of electrical energy). As illustrated in Figure 5, Somale, Amhara, and Oromia are the three Ethiopian regions with the highest biomethane potential from sheep manure.
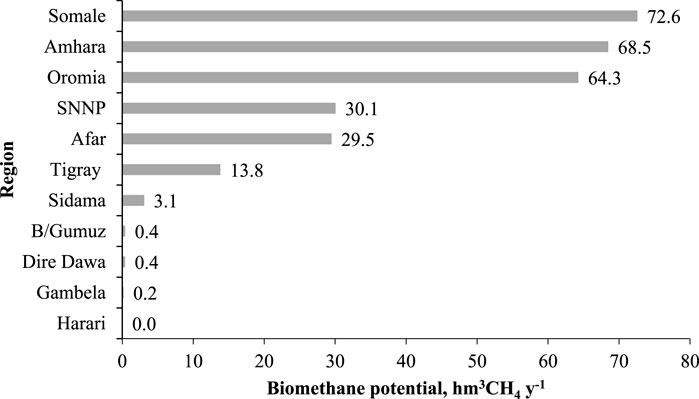
FIGURE 5. Biomethane production from sheep waste in Ethiopia, drawn based on the data in Supplementary Table S3.
3.2.3 Goats
In 2020/21, over 52 million goats were raised in Ethiopia, with Somale (31.4%), Afar (16.9%), Oromia (16.1%), and Amhara (13.4%) being the main producers (CSA, 2020). The amount of daily generated dry manure from goats is reported to be 0.42 kg d−1h−1 (Y. S. Mohammed et al., 2013; Kemausuor et al., 2014; Shane, Gheewala, and Fungtammasan, 2016; Mboumboue and Njomo, 2018; Gabisa and Gheewala, 2018; Tolessa et al., 2021), with an average collection efficiency of approximately 29% (Bidart, Fröhling, and Schultmann, 2014; Kemausuor et al., 2014; Shane, Gheewala, and Fungtammasan, 2016; Gabisa and Gheewala, 2018; Mboumboue and Njomo, 2018; Tolessa et al., 2020). The determined average VS fraction from goat waste is 0.60 kgVS kgDM−1 (Pereraa et al., 2005; UNEP, 2013; Mboumboue and Njomo, 2018), while the average biogas yield of 0.29 m3 kg−1 has been reported in previous studies (Pereraa et al., 2005; UNEP, 2013; Bidart, Fröhling, and Schultmann, 2014; Mboumboue and Njomo, 2018). Based on the data from Supplementary Table S4, the generated gross and recoverable biomass from goat waste are estimated to be 8,065 ktDM y−1 and 2,299 ktDM y−1, respectively. The recoverable biogas amount and corresponding biogas energy from AD of goat waste in Ethiopia are estimated to be 398 hm3 y−1 and 7967 TJ y−1, respectively. From goat waste, the estimated total biomethane and total bioenergy potential in Ethiopia are approximately 239 hm3 y−1 and 8,557 TJ y−1, respectively, corresponding to a total available energy of 6,554 TJ y−1 (3,594 TJ y−1 of thermal energy and 2,961 TJ y−1 of electrical energy). As demonstrated in Figure 6, the highest biomethane energy potential is estimated for Somale, followed by Afar, Oromia, and Amhara.
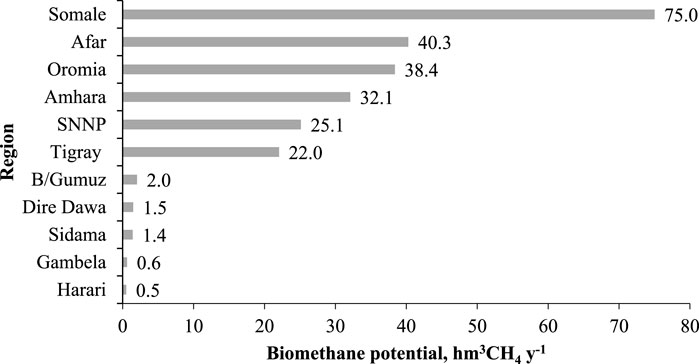
FIGURE 6. Biomethane production from goat waste in Ethiopia, drawn based on the data in Supplementary Table S4.
3.2.4 Horses
Horses are one of the livestock reared in some Ethiopian regions. In 2020/21, total horses raised in Ethiopia amounted to more than 2.1 million heads, with Oromia, Amhara, and the SNNP Region accounting for 61.0%, 22.8%, and 14.4% of the total production, respectively (CSA, 2020). Following horses’ production, horse manure is generated as waste that amounts to 3.15 kg d−1 h−1 of dry manure on average, as reported in the literature (Gabisa and Gheewala, 2018; Mboumboue and Njomo, 2018), with an average collection efficiency of approximately 31% (Bidart, Fröhling, and Schultmann, 2014; Gabisa and Gheewala, 2018; Mboumboue and Njomo, 2018). The VS fraction and the potential of biogas yield production from horse waste have been previously studied, and the average volatile solid fraction and biogas yield on VS reported are 0.88 kgVS kgDM−1 (UNEP, 2013; Mboumboue and Njomo, 2018) and 0.16 m3 kg−1 (UNEP, 2013; Bidart, Fröhling, and Schultmann, 2014; Mboumboue and Njomo, 2018), respectively. From the data reported in Supplementary Table S5, the total gross biomass generated from horse waste in Ethiopia is estimated to be 2,470 ktDM y−1, of which 766 ktDM y−1 is available as recoverable. The recoverable biogas amount and corresponding biogas energy from AD of horse waste are estimated to be 109 hm3 y−1 and 2180 TJ y−1, respectively, in Ethiopia. The total biomethane and total bioenergy potential from horse waste in Ethiopia approximate 65 hm3 per year and 2,341 TJ y−1, respectively, corresponding to a total available energy of 1,794 TJ y−1 (983 TJ y−1 of thermal energy and 810 TJ y−1 of electrical energy). As illustrated in Figure 7, Oromia, Amhara, and the SNNP Region are the three Ethiopian regions with the highest biomethane potential from horse waste.
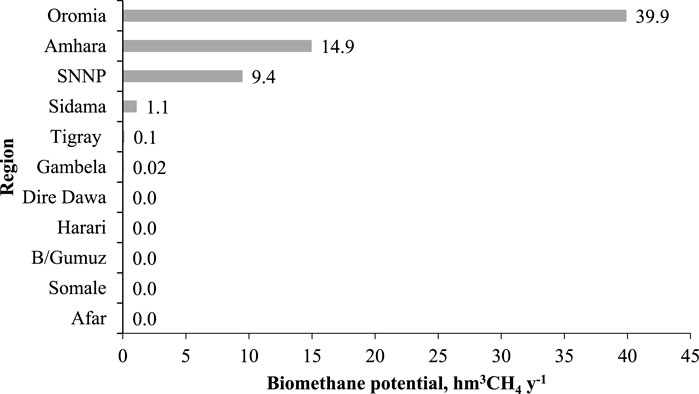
FIGURE 7. Biomethane production from horse waste in Ethiopia, drawn based on the data in Supplementary Table S5.
3.2.5 Donkeys
Donkeys provide a variety of services in Ethiopia, including the transportation of goods, particularly in rural areas. In 2020/21, approximately 10.79 million donkeys were raised in seven Ethiopian regions, with Oromia and Amhara accounting for over three-quarters of the total production in Ethiopia (CSA, 2020). The generated amount of daily dry manure from donkeys is assumed to be 3.0 kg d−1 h−1, with an assumed collection efficiency of approximately 31% (Bidart, Fröhling, and Schultmann, 2014). Studies on AD of donkey waste are relatively few in the literature. The determined average VS fraction from donkey waste is 0.88 kgVS kgDM−1, while the average biogas yield of 0.17 m3 kg−1 has been reported in a previous study (Bidart, Fröhling, and Schultmann, 2014). Based on the data from Supplementary Table S6, the gross and recoverable biomass generated from donkey waste are estimated to be 11,817 ktDM y−1 and 3,663 ktDM y−1, respectively. The amount of recoverable biogas and corresponding biogas energy from AD of the donkey waste in Ethiopia are estimated to be 529 hm3 y−1 and 10,590 TJ y−1, respectively. The biomethane and bioenergy potential from donkey waste in Ethiopia are estimated to be 65 hm3 y−1 and 2,341 TJ y−1, respectively, corresponding to a total available energy of 8,712 TJ y−1 (4,777 TJ y−1 of thermal energy and 3,935 TJ y−1 of electrical energy). The highest biomethane potential from donkey waste is determined for Oromia and Amhara to be 114.8 and 109.7 hm3 y−1, as presented in Figure 8.
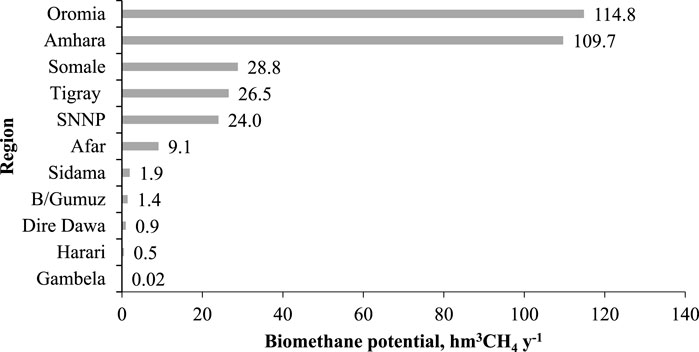
FIGURE 8. Biomethane production from donkey waste in Ethiopia, drawn based on the data in Supplementary Table S6.
3.2.6 Poultry
In 2020/21, Ethiopia raised approximately 57 million poultry, with Oromia and Amhara alone accounting for 34% and 33% of the country’s poultry production, respectively, followed by the SNNP Region (24.6%) and Tigray (15.8%) (CSA, 2020). Poultry was found in the country in the highest numbers after cattle, but it produces a small amount of manure per head. The reported amount of dry matter per poultry head is approximately 0.05 kg d−1 h−1 on average (Mohammed et al., 2013; Kemausuor et al., 2014; Shane, Gheewala, and Fungtammasan, 2016; Gabisa and Gheewala, 2018; Mboumboue and Njomo, 2018; Tolessa et al., 2021), with an average collection efficiency of approximately 68% (Kemausuor et al., 2014; Shane, Gheewala, and Fungtammasan, 2016; Gabisa and Gheewala, 2018; Mboumboue and Njomo, 2018; Tolessa et al., 2020). The VS fraction of poultry waste has been previously studied, and the average volatile solid fraction is 0.47 kgVS kgDM−1 (Pereraa et al., 2005; UNEP, 2013; Mboumboue and Njomo, 2018). Pereraa et al. (2005) and UNEP (2013) reported a biogas yield of 0.18 m3 kg−1 dry poultry waste on VS. According to the data in Supplementary Table S7, the gross biomass generated from poultry waste in Ethiopia is estimated to be 1,137 ktDM y−1, with 773 ktDM y−1 being recoverable. In Ethiopia, the amount of biogas recoverable and corresponding biogas energy from the AD of poultry waste are estimated to be 65 hm3 y−1 and 1,301 TJ y−1. The total biomethane and total bioenergy potential from AD of poultry waste are estimated to be 39 hm3 y−1 and 1,398 TJ y−1, respectively, corresponding to a total available energy of 1,071 TJ y−1 (587 TJ y−1 of thermal energy and 484 TJ y−1 of electrical energy). Oromia and Amhara have the highest total biomethane potential from poultry waste, as shown in Figure 9, followed by the SNNP Region, Somale, and Tigray.
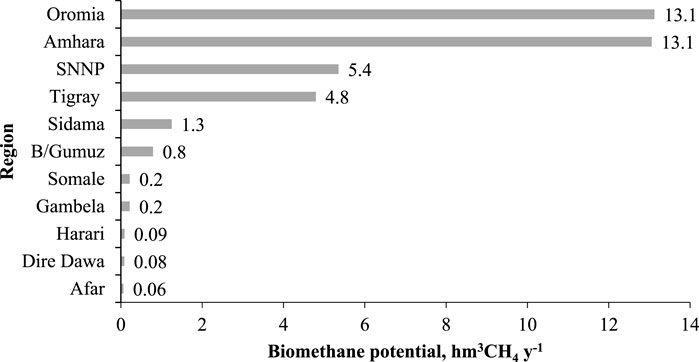
FIGURE 9. Biomethane production from poultry waste in Ethiopia, drawn based on the data in Supplementary Table S7.
3.3 Livestock waste biomass potential
3.3.1 Biogas and energy potential from animal waste biomass
Total recoverable livestock waste is estimated to be approximately 29,598 ktDM y−1 (as presented in Supplementary Table S8), indicating that 38% of gross manure produced is surplus and recoverable. The recoverable fraction of livestock waste can generate approximately 5,536 hm3 y−1 of biogas, which is equivalent to 110,713 TJ y−1 of bioenergy in Ethiopia. The total biogas potential available from recoverable livestock waste is primarily from cattle, with approximately 3,962 hm3 y−1 of biogas (71%). This high potential is due to the relatively large amount of waste generated by cattle (approximately 1.86 kg dry matter per head per day on average) and the large number of cattle in the country, which ranks fifth in the world as cited in the work of Gabisa and Gheewala (2018), resulting in a large amount of cattle waste recovered. Moreover, cattle were owned by the majority of Ethiopian households as part of their production system. Most family farms are located in rural areas of the country, and livestock are generally allowed to roam freely during the day. As a result, it is assumed that for half of the day, most of the produced manure is unrecoverable. However, livestock are generally kept close to the house during the day to prevent animal theft, which provides an excellent opportunity to facilitate manure recovery.
3.3.2 Biomethane and bioenergy potential comparison
3.3.2.1 Comparison among livestock waste potential
Among the livestock waste considered in this study, cattle waste has the highest biomethane potential estimated for Ethiopia, at 2,377 hm3 y−1, followed by donkey (318 hm3 y-1), sheep (283 hm3 y−1), and goat waste (239 hm3 y−1), as shown in Figure 10. After the combustion of the biomethane in a CHP system, this corresponds to an available energy of 65,196 TJ y−1 (cattle waste), 8,712 TJ y−1 (donkey waste), 7,755 TJ y−1 (sheep waste), and 6,554 TJ y−1 (goat waste) (see Supplementary Table S8). The high energy potential of livestock waste is due to the high production of this livestock in Ethiopia and the high methane yields of the residues, with cattle accounting for approximately 72% of the total. The residue with the lowest biomethane and bioenergy potential is poultry since it produces a small amount of waste per head on a daily basis.
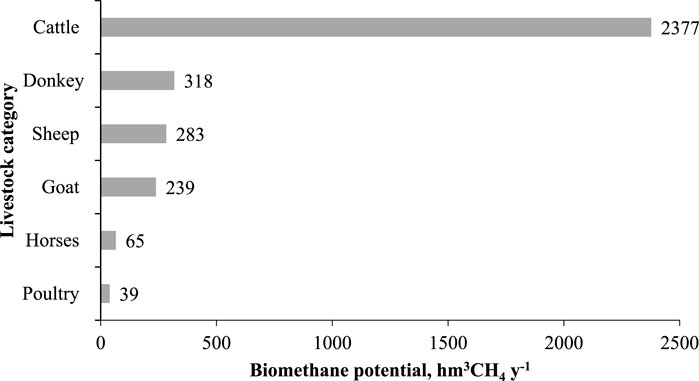
FIGURE 10. Biomethane production from livestock waste in Ethiopia (drawn based on the data in Supplementary Table S8).
3.3.2.2 Comparison among Ethiopian regions
The bioenergy potentials of livestock residues considered in this study vary significantly across Ethiopian regions. The bioenergy potential of Ethiopian regions is determined by their size and geographical locations. Oromia has the highest bioenergy potential, whereas Harari has the lowest energy potential. Overall, the major potential producers of biomass and bioenergy are Oromia, Amhara, and the SNNP Region. As shown in Figure 11, the total biomethane and corresponding bioenergy recoverable from AD of livestock residues are estimated to be 3,321 hm3 y−1 and 118,906 TJ y−1 (equivalent to 33 TWh), respectively. This accounted for approximately 89% and 31% of Ethiopia’s total primary energy production and consumption in 2019, respectively; the estimated Ethiopia’s total primary energy production and consumption were 37 TWh (or 133,200 TJ y−1) and 106 TWh (or 381,600 TJ y−1), respectively (EIA, 2021). In 2019, hydropower (95.83%), wind (3.75%), and other sources (0.42%) accounted for the majority of electricity generation in Ethiopia (Our World in Data, 2022b).
The energy produced by the burning of biomethane in a CHP system totals 91,082 TJ y−1 (25.3 TWh), of which 49,940 TJ y−1 (13.9 TWh) is thermal energy and 41,141 TJ y−1 (11.4 TWh) is electrical energy. However, the smaller the CHP capacity, the lower the electricity yield. As shown in Figure 12 and Figure 13, the estimated 11.4 TWh electrical energy suggests that AD of livestock residues could supply 81% and 11% of Ethiopia’s total electricity generation and total primary energy consumption in 2019, respectively. Thermal energy can be used to heat the substrates to the required digestion temperature (mesophilic or thermophilic temperatures), to maintain the operating temperature inside the reactors, and to reduce the digestate moisture content, which can then be used as feedstock in a composting process. Otherwise, thermal energy can be used in absorption chillers or refrigerators (Bundhoo and Surroop, 2019).
Estimating livestock effluent availability for AD application is critical for the sustainability of biomass supply. The estimated results show a significant biomass potential for the production of biogas and/or biomethane-based energy in the country, which can significantly improve energy access while minimizing traditional biomass use. Livestock waste is either directly applied to crops or farming fields as a biofertilizer, used in dried form for cooking, or left unused. Livestock waste biomass resources can be used to generate bioenergy if used correctly and with the appropriate processing technology (including type and scale of AD; further studies are recommended in the following paragraphs). Anaerobic digestion could be a viable option for generating bioenergy. The communal design type with a large-scale digester is more affordable for a family/farmer/household with more than 30 cattle (equivalent substrate), whereas for a family/farmer/household with two or three cows (equivalent substrate), a smaller household design type digester with a capacity of 4–12 cubic meters might be more affordable (Widodo et al., 2009; Putra, Liu, and Lund, 2017; Tolessa et al., 2020; 2021). The household-scale type digester is more advantageous for families/farmers/households since it can produce bio(gas/methane) with less initial capital outlay and ongoing maintenance (Vu et al., 2015; Tolessa et al., 2020; 2021). Additionally, household-scale digesters can be more desirable in rural areas with very low population densities where feedstock is easily accessible within a limited range for a small farm. This is appropriate for smallholder farms without the key issue of storage and transport. Currently, no households in Ethiopia’s rural areas use biogas to a significant extent. The use of biomass resources to provide clean and renewable energy to rural farming households can help alleviate the problems caused due to the lack of modern, clean energy facilities. It also increases the productive capacity of farming households.
Literature has paid little attention to the challenges impeding the implementation of anaerobic digestion in Africa, including Ethiopia, which include financial constraints, technical and infrastructural constraints, and weak legal and institutional frameworks (Kemausuor, Adaramola, and Morken, 2018). From a financial constraint perspective, the cost of establishing anaerobic digesters, particularly medium to large scale, is quite high, and this is frequently a major impediment to technology implementation in most developing countries (Capodaglio, Callegari, and Lopez, 2016). The incurred costs include costs related to permits, project feasibility studies (preparation studies), and land procurement, investment costs related to biogas plant construction and commissioning, grid network connection, and social/environmental clearances, and plant operating costs, which may include expenses associated with feed collection, transportation, and preparation. As previously stated and cited by Bundhoo and Surroop (2019), the costs of established commercial technologies with high automation are even higher.
Lack of awareness, knowledge, and technical experience about the process of AD and its potential for the generation of renewable energy may be technical barriers, reducing its visibility to potential investors and policymakers (Goemans, 2017; Muvhiiwa et al., 2017; Bundhoo and Surroop, 2019; Tolessa, 2022). Nevertheless, technical barriers are particularly associated with a lack of local technical experience in aspects linked to AD plant construction, commissioning, operation, and maintenance (LTS, 2017; Kemausuor, Adaramola, and Morken, 2018). Furthermore, in terms of poor legal and institutional frameworks, Ethiopia has renewable energy road maps, but due to a poor legal and institutional framework and lack of policy decisions, these targets will remain unmet. A biogas project implementation may be overdue owing to unassigned responsibilities at various project development levels due to a lack of a proper institutional setup (Kemausuor, Adaramola, and Morken, 2018). Similarly, the lack of government policy decisions frequently discourages large-scale AD plant construction due to a lack of guarantees, incentives, and sharing risk in Africa (LTS, 2017).
In Ethiopia, to encourage the implementation of medium-to large-scale AD, governments must first develop a roadmap for renewable energy, with biomass energy as a key component. As a result, governments must ensure that the mechanisms required to facilitate the development of the biomass energy sector are in place. Some recommendations to facilitate medium-to-large-scale development of AD in Ethiopia are based on the following barriers: policies and financial incentives, campaigns for training and public awareness, and the establishment of appropriate legal and institutional frameworks.
Based on some limitations of the current investigation, it is mainly suggested that future research focus on the exact availability of livestock effluent in Ethiopia. These future work’s aspects may include the specificity of waste generation by region, yearly variation in livestock waste biomass production, livestock waste fraction used as a biofertilizer, and region- and climate-dependent values for dry matter. Second, determining the livestock effluents’ biomethane potentials specific to Ethiopian regions opens the door to several potential future studies. Third, one aspect that has been overlooked in this study is co-digestion (simultaneous digestion of two or more substrates). In Ethiopia, livestock effluents are not the only suitable substrates available for AD application. Other substrates include, among others, process- and field-based crop residues, wastewater, and food wastes. As a result, while co-digestion is not covered in this study, it may be investigated in the future. Furthermore, future research could investigate the economics of various AD scales and methods in Ethiopian regions and conduct cost-benefit analyses. In addition to economics, the relationship between socioeconomic indicators and biomethane potential may be deemed as potential future work.
Overall, this study found that AD based on livestock waste biomass can contribute significantly to Ethiopia’s energy needs. The study’s findings revealed that if more emphasis is placed on bioenergy production from livestock waste, the existing energy access problem in many Ethiopian regions can be alleviated, assuming that the amount of required biomass for soil fertility is maintained. Furthermore, proper livestock waste biomass utilization to produce bioenergy in Ethiopian rural areas can substitute a high percentage of traditional solid biomass fuels used for cooking (approximately 90% of households) (Padam et al., 2018; Yalew and Woldie, 2021). Shifting from traditional solid fuels (such as wood, charcoal, and straw) to more efficient modern fuels, such as biomethane, can result in significant reductions in air pollution in households while also reducing pressure on natural resources.
Finally, the paper recommends that different AD technologies be adapted and/or developed to meet local needs (including the availability of feedstock: biomass and water). This could be carried out to investigate potential strategies for increasing biomass potential and utilizing resources more effectively (e.g., a study on whether the use of a community digester shared by households (centralized), household digester types (decentralized), or a large-scale digester is more feasible in local situations). Furthermore, future studies on the effective use of available resources for AD application in the country and across regions would be extremely beneficial to future project assessments. To realize the full potential of AD and assist farmers in better-utilizing farm residues, further research should focus on alternative approaches and usages, for example, natural biogas/bio-natural gas for vehicles, BioNGV (e.g., for conventional fuel substitution), in the context of the circular economy and economic development (Tolessa et al., 2021; Tolessa, 2022; Tolessa, Louw, and Goosen, 2022). As a result, farmers may be able to diversify their income and enhance their socioeconomic development.
Overall, future research on the exact distribution of locations, their availability, and the ecological and economic benefits of collecting and converting biomass feedstock at a national scale could be conducted to incorporate it into the mainstream energy sector. Further research on other wastes, such as crop residue, kitchen waste, abattoir waste, and wastewater, is also advised to maximize the biomass potential of the country and the regions. Future studies are also advised to develop and/or adapt the best AD technologies in accordance with regional conditions and demands by working with potential users and stakeholders, which could lower capital costs and, thereby, lessen the need for financial support. AD technology can be built on a variety of scales, and the size of a project has an impact on economies of scale that should be considered in the planning stages.
4 Conclusion
The availability of biomass resources remains a major concern in the bioenergy production portfolio, particularly for anaerobic digestion applications. Livestock residue biomass assessment in this study has revealed that there is substantial potential for biomass feedstock for AD applications in Ethiopia. Cattle waste produces the most biomethane (2,377 hm3 y−1) and bioenergy (85,112 TJ y−1) of the six livestock category effluents studied, followed by donkey and sheep wastes. The total biomethane and corresponding bioenergy potential from livestock effluents were calculated to be 3,321 hm3 y−1 and 118,906 TJ y−1, respectively, while biomethane combustion in a CHP system produces 49,940 TJ y−1 of thermal and 41,141 TJ y−1 of electricity energy. When comparing Ethiopian regions, Oromia has the highest potential for biomethane and bioenergy (40,568 TJ y−1), while Harari has the lowest (144 TJ y−1). Despite the enormous potential of anaerobic digestion in Ethiopia, its low uptake is attributed to several factors that, if addressed, could substantially accelerate its development and improve the generation of sustainable energy in Ethiopian regions. Critical elements of the Ethiopian region’s sustainable bioenergy industry include conducting research and development, creating a database for local biomass resources, and creating a unified bioenergy unit and policy with the participation and collaboration of all stakeholders. Additionally, decentralized model development, capacity training and awareness-raising, and value chain analysis of biomass feedstock are all crucial for the development of the country’s bioenergy sector. Establishing bioenergy generation will play a significant role in reducing energy poverty, reducing greenhouse gas emissions, and alleviating socioeconomic problems in general. It will also result in the employment of many young people, addressing society’s social problems. Promoting a good waste management system, afforestation, and a modern agricultural system will make a significant contribution to the country’s energy sector.
Data availability statement
The original contributions presented in the study are included in the article/Supplementary Material; further inquiries can be directed to the corresponding author.
Author contributions
AT conceived and designed the experiments; performed the experiments; analyzed and interpreted the data; contributed materials, analysis tools, or data; and wrote the paper.
Acknowledgments
The author would like to express his gratitude to the Ethiopian Statistics Service (ESS), Government of Ethiopia, and the Central Statistical Agency (CSA) for the data used during the study.
Conflict of interest
The author declares that the research was conducted in the absence of any commercial or financial relationships that could be construed as a potential conflict of interest.
Publisher’s note
All claims expressed in this article are solely those of the authors and do not necessarily represent those of their affiliated organizations, or those of the publisher, the editors, and the reviewers. Any product that may be evaluated in this article, or claim that may be made by its manufacturer, is not guaranteed or endorsed by the publisher.
Supplementary material
The Supplementary Material for this article can be found online at: https://www.frontiersin.org/articles/10.3389/fenrg.2023.1249327/full#supplementary-material
Abbreviations
AD, anaerobic digestion; BioNGV, bio-natural gas for Vehicles; CHP, combined heat and power; DALYs, disability-adjusted life years; DM, dry matter; EU, European Union; FY, fiscal year; GDP, gross domestic product; hm3, cubic hectometer; IAP, indoor air pollution; M, million; NBPE, The National Biogas Program of Ethiopia; SNNP, Southern Nations, Nationalities, and Peoples; TJ, terajoule; toe, ton (s) of oil equivalent; TWh, terawatt-hour; VS, volatile solid; and y: year.
References
Al Mamun, M. R., and Torii, Shuichi (2015). Enhancement of production and upgradation of biogas using different techniques-a review. Int. J. Earth Sci. Eng. 8 (02), 877–892.
Berhe, Melaku, Dana, Hoag, Tesfay, Girmay, and Keske, Catherine (2017). Factors influencing the adoption of biogas digesters in rural Ethiopia. Energy, Sustain. Soc. 7 (10), 1–13. doi:10.1186/s13705-017-0112-5
Bidart, Christian, Fröhling, Magnus, and Frank, Schultmann (2014). Livestock manure and crop residue for energy generation: macro-assessment at a national scale. Renew. Sustain. Energy Rev. 38, 537–550. doi:10.1016/j.rser.2014.06.005
Bundhoo, Zumar M. A., and Surroop, Dinesh (2019). Evaluation of the potential of bio-methane production from field-based crop residues in Africa. Renew. Sustain. Energy Rev. 115, 109357. doi:10.1016/j.rser.2019.109357
Bundhoo, Zumar M. A., Mauthoor, Sumayya, and Mohee., Romeela (2016). Potential of biogas production from biomass and waste materials in the small island developing state of Mauritius. Renew. Sustain. Energy Rev. 56, 1087–1100. doi:10.1016/j.rser.2015.12.026
Cao, Yingying, Chen, Dandan, Meng, Ye, Saravanamurugan, Shunmugavel, and Hu, Li (2021). Visible-light-driven prompt and quantitative production of lactic acid from biomass sugars over a N-TiO2 photothermal catalyst. Green Chem. 23 (24), 10039–10049. doi:10.1039/D1GC03057D
Capodaglio, Andrea G., Callegari, Arianna, and Virginia Lopez., Maria (2016). European framework for the diffusion of biogas uses: emerging technologies, acceptance, incentive strategies, and institutional-regulatory support. Sustain. Switz. 8 (4), 1–18. doi:10.3390/su8040298
Clemens, Harry, Bailis, Rob, Nyambane, Anne, and Ndung, Victoria (2018). Africa biogas partnership program: A review of clean cooking implementation through market development in east Africa. Energy Sustain. Dev. 46, 23–31. doi:10.1016/j.esd.2018.05.012
CSA (2020). Central statistical agency. Agricultural sample survey, volume II, report on livestock and livestock. https://www.statsethiopia.gov.et/wp-content/uploads/2021/05/REVISED_2013.LIVESTOCK-REPORT.FINAL-1.pdf.
EIA (2021). Energy information administration: ethiopia, U.S. Energy inf. Admin- istration 14. Available at: https://Www.Eia.Gov/International/Data/Country/ETH.
Gabisa, Elias W., and Gheewala, Shabbir H. (2018). Potential of bio-energy production in Ethiopia based on available biomass residues. Biomass Bioenergy 111, 77–87. doi:10.1016/j.biombioe.2018.02.009
Global Health Observatory (2016). Household air pollution: burden of disease, world health organ. Available at: https://Apps.Who.Int/Gho/Data/Node.Main.139?Lang = En.
Goemans, Roxanne (2017). The development of the biogas industry in South Africa: strategic niche management in an african institutional context. Delft, Netherlands: Delft University of Technology.
Guta, Dawit Diriba (2012). Assessment of biomass fuel resource potential and utilization in Ethiopia: sourcing strategies for renewable energies. Int. J. Renew. Energy Res. (IJRER) 2 (1), 131–139. http://www.iea.org/statistics/statisticssearch/report/?country=Kenya&product=electricityandheat.
IRENA (2022). International renewable energy agency, ENERGY PROFILE Ethiopia. Available at: https://Irena.Org/Data/Energy-Profiles (Accessed on November 8, 2022).
Kemausuor, Francis, Adaramola, Muyiwa S., and Morken, John (2018). A review of commercial biogas systems and lessons for Africa. Energies 11 (11), 2984–3021. doi:10.3390/en11112984
Kemausuor, Francis, Kamp, Andreas., and Østergård, Hanne (2014). Assessment of biomass residue availability and bioenergy yields in Ghana. Resour. Conservation Recycl. 86, 28–37. doi:10.1016/j.resconrec.2014.01.007
Leta, Samson, and Mesele, Frehiwot (2014). Spatial analysis of cattle and shoat population in Ethiopia: growth trend, distribution and market access. SpringerPlus 3, 310. doi:10.1186/2193-1801-3-310
Li, Hu, Wang, Changhong, Xu, Yufei, Yu, Zhaozhuo, Saravanamurugan, Shunmugavel, Wu, Zhilei, et al. (2020). Heterogeneous (de)Chlorination-Enabled Control of Reactivity in the Liquid-Phase Synthesis of Furanic Biofuel from Cellulosic Feedstock. Green Chem. 22 (3), 637–645. doi:10.1039/C9GC04092G
LTS (2017). Bioenergy for sustainable energy access in Africa: PO 7420, stakeholder mapping and literature review report. Penicuik, UK: Lts International Limited.
Lübken, Manfred, Wichern, Marc, Schlattmann, Markus, Gronauer, Andreas, and Horn, Harald (2007). Modelling the energy balance of an anaerobic digester fed with cattle manure and renewable energy crops. Water Res. 41, 4085–4096. doi:10.1016/j.watres.2007.05.061
Macrotreds, L. L. C. (2022). Ethiopia electricity access 2000-2022. Available at: https://Www.Macrotrends.Net/Countries/ETH/Ethiopia/Electricity-Access-Statistics (Accessed on November 10, 2022).
Maithel, Sameer (2009). Biomass energy resource assessment handbook prepared. Asian and pacific centre for transfer of technology of the united nations. Renewable Energy Cooperation-Network for the Asian Pacific.
Mboumboue, Edouard, and Njomo, Donatien (2018). Biomass resources assessment and bioenergy generation for a clean and sustainable development in Cameroon. Biomass Bioenergy 118, 16–23. doi:10.1016/j.biombioe.2018.08.002
Meng, Ye, Huang, Jinshu, Li, Jie, Jian, Yumei, Yang, Song, and Hu, Li (2023). Enzyme-mimicking single atoms enable selectivity control in visible-light-driven oxidation/ammoxidation to afford bio-based nitriles. Green Chem. 25 (11), 4453–4462. doi:10.1039/D3GC00968H
Mengistu, M. G., Simane, B., Eshete, G., and Workneh, T. S. (2015). A review on biogas technology and its contributions to sustainable rural livelihood in Ethiopia. Renew. Sustain. Energy Rev. 48, 306–316. doi:10.1016/j.rser.2015.04.026
Metaferia, Fitaweke, Cherenet, Thomas, Gelan, Ayele., and Gulilat, WondessenJemal Abdi Ali (2011). Areview to improve estimation of livestock contribution to the national GDP. Addia Ababa, Ethiopia: Ministry of Finance and Economic Development and Ministry of Agriculture.
Mohammed, Adem Siraj, Atnaw, Samson Mekbib, and Desta, Melaku (2022). The biogas technology development in Ethiopia: the status, and the role of private sectors, academic institutions, and research centers. Lect. Notes Energy 92, 227–243. doi:10.1007/978-981-19-6688-0_14
Mohammed, Y. S., Mokhtar, A. S., Bashir, N., and Saidur, R. (2013). An overview of agricultural biomass for decentralized rural energy in Ghana. Renew. Sustain. Energy Rev. 20, 15–25. doi:10.1016/j.rser.2012.11.047
Muvhiiwa, Ralph, Hildebrandt, Diane, Chimwani, Ngonidzashe, Ngubevana, Lwazi, and Matambo, Tonderayi (2017). The impact and challenges of sustainable biogas implementation: moving towards a bio-based economy. Energy, Sustain. Soc. 7 (1), 20. doi:10.1186/s13705-017-0122-3
Our World in Data, (2022a). Ethiopia: energy country profile. Avilable at: https://Ourworldindata.Org/Energy/Country/Ethiopia (Accessed on November 7, 2022).
Our World in Data, (2022b). Ethiopia: Energy country profile. Bio. Oxford, England: Global Change Data Lab.
Padam, Gouthami, Dana, Rysankova, Portale, Elisa, Koo, Bonsuk, Keller, Sandra, and Fleurantin, Gina (2018). Ethiopia – beyond connections: Energy access diagnostic report based on the multi-tier framework (English). Washington, D.C., USA: World Bank Group.
PDC (2021). Ten years development plan of Ethiopia (2021-2030). Addis Ababa: Planning and Development Commission. doi:10.13140/RG.2.2.13412.86407
Pereraa, K. K. C. K., Rathnasiria, P. G., Senaratha, S. A. S., Sugathapalaa, A. G. T., Bhattacharyab, S. C., and Abdul Salamb, P. (2005). Assessment of sustainable energy potential of non-plantation biomass resources in Sri Lanka. Biomass Bioenergy 29, 199–213. doi:10.1016/j.biombioe.2005.03.008
Putra, Surya, Liu, Zhen, and Lund, Mogens (2017). The impact of biogas technology adoption for farm households – empirical evidence from mixed crop and livestock farming systems in Indonesia. Renew. Sustain. Energy Rev. 74, 1371–1378. doi:10.1016/j.rser.2016.11.164
Roopnarain, Ashira, and Adeleke, Rasheed (2017). Current status, hurdles and future prospects of biogas digestion technology in Africa. Renew. Sustain. Energy Rev. 67, 1162–1179. doi:10.1016/j.rser.2016.09.087
Rupf, Gloria V., Bahri, Parisa A., De Boer, Karne, and Mchenry, Mark P. (2016). Broadening the potential of biogas in sub-saharan Africa: an assessment of feasible technologies and feedstocks. Renew. Sustain. Energy Rev. 61, 556–571. doi:10.1016/j.rser.2016.04.023
Sanbata, Habtamu, Araya, Asfaw, and Kumie, Abera (2014). Indoor air pollution in slum neighbourhoods of Addis Ababa, Ethiopia. Atmos. Environ. 89, 230–234. doi:10.1016/j.atmosenv.2014.01.003
Shane, Agabu, H Gheewala, Shabbir, Fungtammasan, Bundit, Silalertruksa, T., Bonnet, S., and Phiri, S. (2016). Bioenergy resource assessment for Zambia. Renew. Sustain. Energy Rev. 53, 93–104. doi:10.1016/j.rser.2015.08.045
SNV (2022). Biogas dissemination scale-up Programme (NBPE +) and the national biogas program of Ethiopia (NBPE). Available at: https://Snv.Org/Project/National-Biogas-Programme-Ethiopia.
Solomon, Ayele, Workalemahu, Assegid, Jabbar, M. A., Ahmed, M. M., and Hurissa, Belachew (2003). Livestock marketing in Ethiopia: A review of structure, performance and development initiatives. Socio-economics and policy research working paper 52. Nairobi, Kenya: ILRI (International Livestock Research Institute.
Tolessa, Amsalu (2023a). Bioenergy potential from crop residue biomass resources in Ethiopia. Heliyon 9 (2), e13572. doi:10.1016/j.heliyon.2023.e13572
Tilahun, Helina, and Schmidt, Emily (2012). Spatial analysis of livestock production patterns in Ethiopia: ethiopia strategy support program II (ESSP II), ESSP II working paper 44. http://essp.ifpri.info/files/2011/04/ESSP2_WP44_SpatialAnalysisLivestockProduction.pdf.
Tolessa, Amsalu, Bélières, Jean-François, Salgado, Paulo, Raharimalala, Sitrakiniaina, Louw, Tobias M., and Goosen, Neill J. (2021). Assessment of agricultural biomass residues for anaerobic digestion in rural vakinankaratra region of Madagascar. BioEnergy Res. 15, 1251–1264. doi:10.1007/s12155-021-10336-7
Tolessa, Amsalu (2022). PhD thesis. Stellenbosch, South Africa: Stellenbosch University.Development of a techno-economic analysis tool for anaerobic digestion in smallholder farming systems in the context of the water-energy-food nexus
Tolessa, Amsalu, Goosen, Neill J., and Louw, Tobias M. (2021b). Probabilistic simulation of biogas production from anaerobic Co-digestion using anaerobic digestion model No. 1: A case study on agricultural residue. Available at: https://ssrn.com/abstract=3935562.doi:10.2139/ssrn.3935562
Tolessa, Amsalu, Goosen, Neill J., and Louw, Tobias M. “Simulation of anaerobic digestion of agricultural substrates using anaerobic digestion model No.1,” in Proceedings of the Southern African Sustainable Energy Conference, 7th SASEC Conference, South Africa, Stellenbosch, November 2021a, 243–249.
Tolessa, Amsalu, Louw, Tobias M., and Goosen, Neill J. (2022). Probabilistic techno-economic assessment of anaerobic digestion predicts economic benefits to smallholder farmers with quantifiable certainty. Waste Manag. 138, 8–18. doi:10.1016/j.wasman.2021.11.004
Tolessa, Amsalu (2023b). Potential of biomethane-based energy production from livestock waste biomass resources in Ethiopia. SSRN 11. doi:10.2139/ssrn.4335665
Tolessa, Amsalu, Zantsi, Siphe, Louw, Tobias M., Greyling, Jan C., and Goosen, Neill J. (2020). Estimation of biomass feedstock availability for anaerobic digestion in smallholder farming systems in South Africa. Biomass Bioenergy 142, 105798. doi:10.1016/j.biombioe.2020.105798
Tucho, Gudina Terefe, Henri C MollUiterkamp, Anton J. M. Schoot, and Nonhebel, Sanderine (2016). Problems with biogas implementation in developing countries from the perspective of labor requirements. Energies 9 (750), 1–16. doi:10.3390/en9090750
Tucho, , Terefe, Gudina, and Nonhebel, Sanderine (2017). Alternative energy supply system to a rural village in Ethiopia. Energy, Sustain. Soc. 7, 33. doi:10.1186/s13705-017-0136-x
Tucho, , Terefe, Gudina, and Nonhebel, Sanderine (2015). Bio-wastes as an alternative household cooking energy source in Ethiopia. Energies 8, 9565–9583. doi:10.3390/en8099565
UNEP (2013). Technologies for converting waste agricultural biomass to energy. Osaka, Japan: Compiled by United Nation Environment Program Division of Technology, Industry and Economics International Environmental Technology Centre.
Vu, T. K. V., Vu, D. Q., Jensen, L. S., Sommer, S. G., and Bruun, S. (2015). Life cycle assessment of biogas production in small-scale household digesters in vietnam. Asian-Australasian J. Animal Sci. 28 (5), 716–729. doi:10.5713/ajas.14.0683
Widodo, Teguh Wikan, Ahmad, Asari, Ana, N., and Elita, R. (2009). Design and development of biogas reactor for farmer group scale. Indonesian J. Agric. 2 (2), 121–128.
World Bank (2022). The world bank in Ethiopia. Available at: https://Www.Worldbank.Org/En/Country/Ethiopia/Overview.
Keywords: anaerobic digestion, biogas, biomass, energy potential, biomethane, livestock waste
Citation: Tolessa A (2023) Potential of biomethane-based energy production from livestock waste biomass resources in Ethiopia. Front. Energy Res. 11:1249327. doi: 10.3389/fenrg.2023.1249327
Received: 28 June 2023; Accepted: 28 July 2023;
Published: 10 August 2023.
Edited by:
Hu Li, Guizhou University, ChinaReviewed by:
Samuel Lalthazuala Rokhum, National Institute of Technology, IndiaYe Meng, Guizhou University, China
Copyright © 2023 Tolessa. This is an open-access article distributed under the terms of the Creative Commons Attribution License (CC BY). The use, distribution or reproduction in other forums is permitted, provided the original author(s) and the copyright owner(s) are credited and that the original publication in this journal is cited, in accordance with accepted academic practice. No use, distribution or reproduction is permitted which does not comply with these terms.
*Correspondence: Amsalu Tolessa, YW1zYS50b2xhQGdtYWlsLmNvbQ==