- 1School of Environmental Science and Engineering, Suzhou University of Science and Technology, Suzhou, Jiangsu, China
- 2Jiangsu Key Laboratory of Micro and Nano Heat Fluid Flow Technology and Energy Application, School of Physical Science and Technology, Suzhou University of Science and Technology, Suzhou, China
1 Introduction
With the rapid development of the world economy, the energy crisis and environmental issues have become two important issues facing humanity. Developing and utilizing renewable and clean energy is an effective way to relieve the pressure of the energy crisis and environmental issues. The energy received daily from the sun is sufficient to supply all the energy needed on the earth for a year (Chapo, 2008) and about 80% of the solar energy reaching the earth is absorbed by the ocean (Malik et al., 2020). Therefore, ocean energy has attracted wide attention due to its abundant resources, clean and stable characteristics. Ocean energy can be divided into ocean thermal energy, salinity energy, tidal energy, current energy and wave energy. The above types of ocean energy have the potential to be used as alternatives to traditional fossil energy.
Ocean thermal energy is the energy contained in the temperature difference between the surface warm seawater and the deep cold seawater. Between 25°N and 25°S, the surface seawater temperature is above 26°C (Nithesh et al., 2016), and the deep seawater temperature at 900 m below the sea surface is maintained at 4°C–6°C (Yang and Yeh, 2014). The reserve of ocean thermal energy is second only to wave energy in all ocean energy. Ocean thermal energy can be utilized in many forms, such as power generation (Rajagopalan and Nihous, 2013), refrigeration (Bian et al., 2019), hydrogen generation (Khanmohammadi et al., 2020) and desalination of seawater (Park et al., 2014). Ocean Thermal Energy Conversion (Abbreviated as OTEC) (Rajagopalan and Nihous, 2013) technology utilizes the temperature difference between the surface warm seawater and the deep cold seawater to generate electricity through heat exchangers and turbines. If all the ocean thermal energy is used for power generation, 87,600 TWh of electricity can be generated every year, which is more than five times the annual global electricity demand (Khan et al., 2017). Due to the enormous potential of ocean thermal energy in power generation, many coastal countries, including the United States (Mitsui et al., 1983), China (Yang and Yeh, 2014), and Japan (Johnson, 1989; Marti et al., 2064) have conducted research on ocean thermal energy conversion technology.
In this paper, the development status of OTEC technology is introduced in detail, and the potential challenges and development prospects of OTEC technology in future applications are discussed, so as to provide reference for the development and utilization of renewable energy.
2 Development status of OTEC technology
The OTEC system can be divided into three basic types: open cycle, closed cycle (Sherwood, 1995) and hybrid cycle (Heydt, 1993). The open cycle was first proposed by Georges Claude, who established an open cycle power generation device with an output power of 22 kW in 1929 (Claude, 1930). The thermal efficiency of open cycle was only about 0.7% (Takazawa et al., 1996) This system consisted of flash evaporator, turbine, electric generator, condenser and seawater pump, but due to site selection and construction technology defects, the system failed to achieve net power output. Figure 1A shows the schematic of the open cycle system. The surface warm seawater is pumped into the flash evaporator by a warm seawater pump, where the warm seawater is vaporized. After vaporization, the steam enters the turbine to drive the generator for power generation, and then enters the condenser and is condensed into fresh water by deep cold seawater. The structure of the open cycle is simple, and seawater is used as the working fluid, whcih has the advantages of environmental protection, pollution-free, and can produce fresh water while generating electricity. However, its disadvantages are also obvious: the flash evaporator volume is huge and the sea water pump has high power consumption.
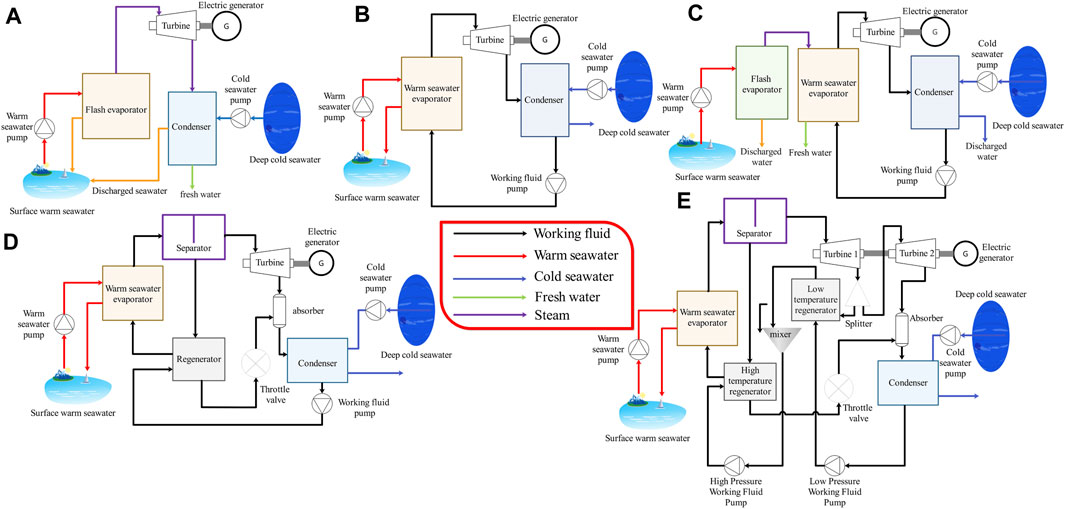
FIGURE 1. Schematic of the (A) open cycle, (B) closed cycle, (C) hybrid cycle, (D) Kalina cycle, and (E) Uehara cycle.
The closed cycle was first proposed by D'Arsonval in 1881 (D’arsonval, 1881), this system consisted of evaporator, turbine, condenser, electric generator, working fluid pump and seawater pumps. The thermal efficiency of closed cycle was about 2.1% (Flynn and Cicchetti George, 1997). Figure 1B shows the schematic of the closed cycle system. The surface warm seawater is sent into the evaporator by the warm seawater pump to exchange heat with the working fluid, and the working fluid is vaporized in the evaporator. The vaporized working fluid enters the turbine and drives the electric generator to generate electricity. And then the working fluid enters the condenser and exchanges heat with the cold seawater. Finally, the condensed working fluid is pumped into the evaporator to complete a full cycle. Compared to the open cycle, the closed cycle has a smaller equipment size and lower construction costs.
The hybrid cycle was first proposed by Vega et al., in 1989 (Vega, 2002). Figure 1C shows the schematic of the hybrid cycle system. The thermal efficiency of hybrid cycle was 1.6% (Panchal and Bell, 1987). The warm seawater is pumped into the flash evaporator by the warm seawater pump, and then flash to generate steam. Steam enters the evaporator and exchanges heat with the working fluid. The working fluid is evaporated in the evaporator, while the steam is condensed into fresh water. The evaporated working fluid enters the turbine and drives the generator to generate electricity, and then the working fluid enters the condenser for heat exchange with cold seawater. The condensed working fluid is pumped into the evaporator to exchange heat with steam, and the cycle continues (Heydt, 1993). The advantages of the hybrid cycle are high system efficiency and the ability to generate fresh water while generating electricity. However, the hybrid cycle has the disadvantages of too complex structure and low economy.
Early ocean thermal energy conversion systems were all Rankine cycles with simple structure, and the closed cycle is the most widely used system (Chen et al., 2010). However, the thermal efficiency of Rankine cycle was only about 3% (Kleute et al., 2009), because the temperature difference between surface warm seawater and deep cold seawater was only 15–25°C (Jung et al., 2016; Ikegami et al., 2018). Therefore, the researchers began to conduct further research and exploration on more efficient ocean thermal energy conversion technology. Karina cycle was proposed by Karina in 1981 and the ammonia-water mixture was used as the working fluid (ZhangHeZhang, 2012). Compared with the Rankine cycle, the Karina cycle has an additional subsystem for fractionation. Figure 1D shows the schematic of the Karina cycle system. The basic ammonia solution becomes a two-phase mixed working fluid after absorbing heat in evaporator. After entering the separator, the two-phase mixed working fluid is divided into ammonia-poor solution and ammonia-rich vapor. Ammonia-rich vapor drives the turbine to generate electricity. The low concentration ammonia-poor solution enters the regenerator to preheat the mixed working fluid delivered by the working fluid pump, and then enters the absorber after depressurization through the throttle valve. Subsequently, the ammonia-poor solution and ammonia-rich vapor are mixed in the absorber to become a two-phase mixed working fluid again. Then the two-phase mixed working fluid enters the condenser and condenses into the basic ammonia solution. The basic ammonia solution is then pumped by the working fluid pump into the regenerator to preheat, and finally enters the evaporator to complete a Karina cycle. Karina cycle uses the ammonia-water mixture as the working fluid, it can achieves a better temperature match between the cycle and the heat and cold sources. Therefore, the output power of the turbine is improved, and the thermal efficiency of the cycle can be up to 4.5% (Kalina, 1984).
The Uehara cycle was proposed by Haruo Uehara in 1994 and is also a power cycle system using ammonia-water mixture as the working fluid (Uehara, 1995). Figure 1E shows the schematic of the Uehara cycle system. The basic ammonia solution becomes two-phase mixed working fluid after absorbing heat in evaporator, and then the two-phase mixed working fluid enters the separator and is divided into ammonia-poor solution and ammonia-rich vapor. The ammonia-poor solution enters the high temperature regenerator to preheat the working fluid pumped by the high pressure working fluid pump, and then enters the throttle valve for depressurization. In addition, ammonia-rich vapor drives the first turbine to generate electricity, and then a part of the ammonia-rich vapor is extracted into the low-temperature regenerator to heat the working fluid pumped by the low-pressure working fluid pump, and the remaining ammonia-rich vapor enters the secondary turbine to continue generate electricity. The ammonia-rich vapor from the secondary turbine and the ammonia-poor solution after depressurization are mixed into a two-phase mixed working fluid in the absorber. The two-phase mixed working fluid enters the condenser to release heat and is transported into the low temperature regenerator by the low pressure working fluid pump. The working fluid from the low-temperature regenerator and the ammonia-rich vapor from the first turbine are mixed into basic ammonia solution in the mixer. The basic ammonia solution is pumped into the high temperature regenerator by the high pressure working fluid pump to preheat, and finally enters the evaporator to complete a full cycle. Compared to the Kalina cycle, the Uehara cycle is equipped an extraction regenerative cycle, the temperature of the working fluid entering the evaporator is increased, and the heat absorption of the system is reduced. Therefore, the thermal efficiency of the Uehara cycle is higher than that of the Rankine cycle and the Kalina cycle, and its value can reach 4.97% (Uehara et al., 1998). However, the disadvantage of the Uehara cycle is also obvious, the complexity of the Uehara cycle is much higher than that of the Rankine cycle and the Karina cycle.
3 Opportunities and challenges
With the economic development and population growth, the energy demand of human will continue to increase, which brings opportunities and challenges to the application and development of OTEC technology. Therefore, considering the research status and practical application requirements of OTEC technology, the following aspects need to be further studied in the future:
Low energy conversion efficiency is the biggest problem in the development of OTEC technology. This is because the energy conversion efficiency of the OTEC technology is limited by the small temperature difference between cold seawater and warm seawater (YamadaHoshiIkegami, 2006). The temperature difference between the surface warm seawater and deep cold seawater is only about 20°C even in the tropics (Yang and Yeh, 2014; Nithesh et al., 2016). For example, the thermal efficiency of the Uehara cycle can only reach 4.97%. Therefore, more attention should be paid to improving the thermal efficiency of OTEC technology. Ocean thermal energy can be effectively combined with other forms of low-grade heat sources, such as solar energy (Yamada et al., 2009) and industrial waste heat (Kim et al., 2009), to improve the thermal efficiency of the system. Solar energy and industrial heat can be used to heat the surface warm seawater, which increases the temperature difference between warm seawater and cold seawater, thus improving the system efficiency.
2) At present, OTEC technology has the problem of poor economy. There are many utilization ways of ocean thermal energy, such as power generation, refrigeration, hydrogen production, aquaculture of aquatic products and crops, seawater desalination. Therefore, the ocean thermal energy can be comprehensively utilized. According to the principle of cascade utilization, the surface warm seawater and deep cold seawater can be fully utilized for power generation, refrigeration, seawater desalination and deep water aquaculture, which will greatly improve the economic benefits and play a positive role in reducing carbon emissions.
Author contributions
EH contributed to conception of the study. WC wrote the first draft of the manuscript. All authors listed have made a substantial, direct, and intellectual contribution to the work and approved it for publication.
Funding
This work is supported by National Natural Science Foundation of China (No. 52106023).
Conflict of interest
The authors declare that the research was conducted in the absence of any commercial or financial relationships that could be construed as a potential conflict of interest.
Publisher’s note
All claims expressed in this article are solely those of the authors and do not necessarily represent those of their affiliated organizations, or those of the publisher, the editors and the reviewers. Any product that may be evaluated in this article, or claim that may be made by its manufacturer, is not guaranteed or endorsed by the publisher.
References
Bian, Y., Pan, J., Liu, Y., Zhang, F., Yang, Y., and Arima, H. (2019). Performance analysis of a combined power and refrigeration cycle. Energy Convers. Manag. 185, 259–270. doi:10.1016/j.enconman.2019.01.072
Chapo, R. (2008). Solar energy overview. http://ezinearticles.com.
Chen, H., Goswami, D. Y., and Stefanakos, E. K. (2010). A review of thermodynamic cycles and working fluids for the conversion of low-grade heat. Renew. Sustain. energy Rev. 14 (9), 3059–3067. doi:10.1016/j.rser.2010.07.006
Claude, G. (1930). Power from the tropical seas. Mech. Eng. 52 (12), 1039–1044. https://cir.nii.ac.jp/crid/1572543024001021312.
D'arsonval, J. A. (1881). Utilization des Forces Naturelles, Avenir de l'electricite. Le. Revenue Sci. 17, 370–372. https://cir.nii.ac.jp/crid/1571698599542132224.
Flynn, R., and Cicchetti George, J. (1997). Ocean thermal energy conversion(OTEC)system. Renew. Energy 11 (2), 276–290. doi:10.1016/S0960-1481(97)84537-5
Heydt, G. T. (1993). An assessment of ocean thermal energy conversion as an advanced electric generation methodology. Proc. IEEE 81 (3), 409–418. doi:10.1109/5.241487
Ikegami, Y., Yasunaga, T., and Morisaki, T. (2018). Ocean thermal energy conversion using double-stage Rankine cycle. J. Mar. Sci. Eng. 6 (1), 21. doi:10.3390/jmse6010021
Johnson, F. A. (1989). “A 250 KW closed cycle land based Ocean Thermal energy plant,” in ocean energy recovery (Reston, Virginia, United States: American Society of Civil Engineers), 247–256. https://cedb.asce.org/CEDBsearch/record.jsp?dockey=0067587.
Jung, J. Y., Lee, H. S., Kim, H. J., Yoo, Y., Choi, W. Y., and Kwak, H. Y. (2016). Thermoeconomic analysis of an ocean thermal energy conversion plant. Renew. energy 86, 1086–1094. doi:10.1016/j.renene.2015.09.031
Kalina, A. I. (1984). Combined-cycle system with novel bottoming cycle. J. Eng. Gas. Turbines Power 106. doi:10.1115/1.3239632
Khan, N. D., Kalair, A., Abas, N., and Haider, A. (2017). Review of ocean tidal, wave and thermal energy technologies. Renew. Sustain. Energy Rev. 72, 590–604. doi:10.1016/j.rser.2017.01.079
Khanmohammadi, S., Baseri, M. M., and AhmadiAl-RashedAfrand, P. A. M. (2020). Proposal of a novel integrated ocean thermal energy conversion system with flat plate solar collectors and thermoelectric generators: Energy, exergy and environmental analyses. J. Clean. Prod. 256, 120600. doi:10.1016/j.jclepro.2020.120600
Kim, N. J., Ng, K. C., and Chun, W. (2009). Using the condenser effluent from a nuclear power plant for Ocean Thermal Energy Conversion (OTEC). Int. Commun. Heat Mass Transf. 36 (10), 1008–1013. doi:10.1016/j.icheatmasstransfer.2009.08.001
Kleute, B. J., Dodoros, G., Verpoort, J., Harb, Y. S., and Hemmes, K. (2009). Design of an 10 MW OTEC power plant. mechanical maritime and materials engineering. https://repository.tudelft.nl/islandora/object/uuid:27c5ddc1-d783-47e4-938b-e7d68bff1753?collection=research.
Malik, M. Z., Musharavati, F., Khanmohammadi, S., Baseri, M. M., Ahmadi, P., and Nguyen, D. D. (2020). Ocean thermal energy conversion (OTEC) system boosted with solar energy and TEG based on exergy and exergo-environment analysis and multi-objective optimization. Sol. Energy 208, 559–572. doi:10.1016/j.solener.2020.07.049
Marti, J. A., Plocek, T. J., Laboy, M. A., and Ruiz, O. E. Ocean Thermal Energy Conversion: Strategies for system integration and implementation using commercially-available components and technology, Proceeding of the Offshore Technology Conference , Houston, Texas, USA. 2010, May.doi:10.4043/20644-MS
Mitsui, T., Ito, F., Seya, Y., and Nakamoto, Y. (1983). Outline of the 100 kw OTEC pilot plant in the republic of naure. IEEE Trans. power apparatus Syst. PAS-102 (9), 3167–3171. doi:10.1109/TPAS.1983.318124
Nithesh, K. G., Chatterjee, D., Oh, C., and Lee, Y. H. (2016). Design and performance analysis of radial-inflow turboexpander for OTEC application. Renew. Energy 85, 834–843. doi:10.1016/j.renene.2015.07.018
Panchal, C. B., and Bell, K. J. (1987). Simultaneous production of desalinated water and power using a hybrid-cycle OTEC plant. J. Sol. Energy Eng. 109. doi:10.1115/1.3268193
Park, S. S., Kim, W. J., KimJeon, Y. H. Y. H., and Kim, N. J. (2014). A study on the solar-otec convergence system for power generation and seawater desalination. iccc 34 (2), 73–81. doi:10.7836/kses.2014.34.2.073
Rajagopalan, K., and Nihous, G. C. (2013). Estimates of global Ocean Thermal Energy Conversion (OTEC) resources using an ocean general circulation model. Renew. Energy 50, 532–540. doi:10.1016/j.renene.2012.07.014
Sherwood, W. G. (1995). Renewable energy from the oceans: A guide to OTEC. Oxford, United Kingdom: Oxford University Press, 446. doi:10.1016/0029-8018(95)90035-7
Takazawa, H., Amano, M., and Tanaka, T. (1996). Performance characteristics of barometric type open-cycle OTEC system. IEEJ Trans. Power Energy 116 (5), 525–530. doi:10.1002/(SICI)1520-6556
Uehara, H., Ikegami, Y., and Nishida, T. (1998). Performance analysis of OTEC system using a cycle with absorption and extraction processes. Transaction JSME, Ser. B 64 (624), 2750–2755. doi:10.1299/kikaib.64.2750
Uehara, H. (1995). The present status and future of ocean thermal energy conversion. Int. J. Sol. energy 16 (4), 217–231. doi:10.1080/01425919508914278
Vega, L. A. (2002). Ocean thermal energy conversion primer. Mar. Technol. Soc. J. 36 (4), 25–35. doi:10.4031/002533202787908626
Yamada, N., Hoshi, A., and Ikegami, Y. (2009). Performance simulation of solar-boosted ocean thermal energy conversion plant. Renew. Energy 34 (7), 1752–1758. doi:10.1016/j.renene.2008.12.028
YamadaHoshiIkegami, N. A. Y. (2006). Thermal efficiency enhancement of ocean thermal energy conversion (otec) using solar thermal energy. Proc. Symposium Environ. Eng. 16, 404–407. doi:10.2514/6.2006-4130
Yang, M. H., and Yeh, R. H. (2014). Analysis of optimization in an OTEC plant using organic Rankine cycle. Renew. Energy 68, 25–34. doi:10.1016/j.renene.2014.01.029
Keywords: ocean thermal energy conversion, Rankine cycle, Uehara cycle, Kalina cycle, opportunities and challenges
Citation: Chen W and Huo E (2023) Opportunities and challenges of ocean thermal energy conversion technology. Front. Energy Res. 11:1207062. doi: 10.3389/fenrg.2023.1207062
Received: 17 April 2023; Accepted: 10 May 2023;
Published: 18 May 2023.
Edited by:
Xiangdong Liu, Yangzhou University, ChinaReviewed by:
Gang Chen, Zhengzhou University of Light Industry, ChinaLiu Jinyu, Chongqing University, China in collaboration with reviewer GC
Copyright © 2023 Chen and Huo. This is an open-access article distributed under the terms of the Creative Commons Attribution License (CC BY). The use, distribution or reproduction in other forums is permitted, provided the original author(s) and the copyright owner(s) are credited and that the original publication in this journal is cited, in accordance with accepted academic practice. No use, distribution or reproduction is permitted which does not comply with these terms.
*Correspondence: Erguang Huo, aHVvZXJndWFuZ0AxMjYuY29t