Corrigendum: Assessing the viability of a grid-connected PV power plant in Mubi, Adamawa State, Nigeria
- 1Department of Mechanical Engineering, School of Engineering, Federal Polytechnic Mubi, Mubi, Nigeria
- 2Centre for Entrepreneurship Development, Federal Polytechnic Mubi, Mubi, Nigeria
- 3Regional Leading Research Center for Smart Energy System, Kyungpook National University, Sangju, Republic of Korea
- 4Department of Convergence and Fusion System Engineering, Kyungpook National University, Sangju, Republic of Korea
- 5Department of Entrepreneurship and Creative Industries, Canadian University Dubai, Dubai, United Arab Emirates
- 6Civil Engineering Department, University of Ottawa, Ottawa, ON, Canada
- 7Department of Energy Convergence and Climate Change, Kyungpook National University, Daegu, Republic of Korea
This paper is based on a techno-economic analysis and the environmental impact of a proposed 1 MW solar photovoltaic (PV) power plant at the main campus of the Federal Polytechnic Mubi (FPM) in north-eastern Nigeria. A photovoltaic power plant converts solar radiation into electricity that can be used as a source of electrical power to meet the daily energy requirements of homes, equipment, and all tertiary institutions. RETScreen Expert software was used to evaluate the techno-economic and environmental sustainability of installing a grid-connected PV power plant. The research results revealed that with an annual solar radiation of 5.74 kWh/m2/day, the maximum annual energy production was estimated to be 1,550.98 MWh. It was discovered that the maximum energy production in March was 146.89 MWh. The project’s profitability and economic sustainability were determined with a good internal rate of return (IRR) of 11.9% and a positive net present value (NPV) of $681,164. The proposed PV power plant has a simple payback period of 11.4 years. The maximum greenhouse gas (GHG) emission reduction is 670.9 tCO2, equivalent to 61.7 ha of forest-absorbing carbon emissions.
1 Introduction
Renewable energy is essential because of its vital role in producing energy, among several other energy sources used worldwide. Many countries are shifting from conventional fossil-fuel-based power generation to renewable, environmentally friendly energy sources (Same et al., 2022). Alternative and sustainable energy policies aim to address future energy demand challenges while simultaneously mitigating air pollution and combating climate change on a global scale. One primary renewable energy source is solar energy technology, which has advanced significantly in several aspects in recent years. Solar photovoltaic (PV) technology, which directly transforms solar radiation into electricity, has become one of the fastest-growing and most widely adopted renewable energy technologies worldwide. Recently, PV module prices have decreased by 80% and are expected to continue falling (al Garni et al., 2016; Renewable Energy Agency, 2014). According to Jamil et al. (2012), renewable energy is crucial to a country’s sustainable development, particularly in light of mounting international pressure to reduce carbon emissions. By leveraging energy storage devices, renewable energy can meet energy demand and provide a continuous supply of electricity (Pal et al., 2015).
Numerous studies have demonstrated that as the human population grows, so does energy consumption. It has been reported that many communities worldwide, particularly in developing countries, face economic and social challenges due to over two billion people lacking access to electricity (Martínez-Díaz et al., 2013; Akinyele et al., 2015a). Energy plays a fundamental role in our daily activities due to the increase in population, industrialization, and demand for rapid change in the standard of living of people throughout the globe compared with the last few decades. A conventional method of generating electricity is the thermal power plant, which depends on fossil fuels and contributes to greenhouse gas (GHG) emissions, leading to atmospheric pollution that causes acid rain (Raji et al., 2020). Aba et al. (2019) stated that the PV system was ranked as the third most sustainable renewable energy technology in the world. Bopp et al. (Kumi and Brew-Hammond, 2013) reported that due to energy challenges in Sub-Saharan Africa, between 2000 and 2014, more than 2000 factories were forced to shut down because of power outages. The outages of grid power in Africa led to the usage of gigantic diesel generators with rising fuel costs; operating and maintaining these electric generators caused hardship for most private businesses, enterprises, and companies and accelerated the shutting down of businesses (Adesanya and Schelly, 2019; Reuters, 2022).
Today, Nigeria ranks among the countries with high solar energy potential, ranging from 3.5 kWh/m2/day to 7.0 kWh/m2/day (Raji et al., 2020; Okoye et al., 2016). The Nigerian government has shown interest in renewable energy to eliminate blackouts in the national power supply. The northern states of Nigeria have 6 kWh/m2/day to 7.5 kWh/m2/day of direct irradiance, which is higher than the recommended value required for concentrating solar power technologies (Ogunmodimu and Okoroigwe, 2018). Nigeria has natural resources and excellent potential for future development if these resources are utilized (Soonmin et al., 2019). Adesanya and Schelly (2019) reported that the country’s vision was to be among the 20 economic superpowers worldwide by the end of 2020, but this goal could not be achieved due to an inadequate power supply to boost economic growth. Many businesses in Nigeria mainly depend on conventional power generation as their primary source of electricity. Jaime et al. (Ruiz-Cabrero et al., 2022) proposed that for Nigeria to develop, multi-dimensional growth and infrastructural development like a power generation plant should be considered as one of the five key areas to be addressed to provide a reliable and affordable power supply.
Okoye et al. (2016) reported that solar energy systems had been used to provide electricity for household and street lighting across Nigeria. Still, many people are unaware of the benefits of PV, and those who are discouraged because of the high installation costs. As Nigeria experiences insufficient electricity supply, it is imperative to explore alternative means of generating power and work toward reducing dependence on such methods by 2025. To achieve this goal, the government, educational institutions, researchers, and public and private companies must collaborate and promote environmentally friendly energy sources (Ong et al., 2011). To ensure environmental sustainability and long-term viability, the Nigerian government must prioritize efforts to enhance the efficiency of the power system by integrating alternative energy sources. Higher education institutions, such as universities, polytechnics, and research institutes with large land areas, can transform their environment into green, self-sustaining campuses.
There are many relevant studies on the simulation of the PV power system using free or paid energy simulation software such as Homer Pro, PVsyst, PV Watt, Solar-GIS, Solar Advisor Model, Solar Pro, Helioscope, PV Sol, and PV F-Chart (Shukla et al., 2016; Baitule and Sudhakar, 2017). In the work of Alsharif et al. (2017), the author conducted a techno-economic evaluation of a stand-alone power system based on the mobile communication system. The author carried out the research based on South Korean solar radiation data using HOMER software and obtained the result on the initial capital cost of the project by comparing the economic feasibility of the proposed solar system with a conventional energy system in urban and rural South Korea. Bhuyan et al. (2017) conducted a performance analysis of a 75 kW solar power plant in grid-connected and off-grid modes. The authors used a software application to estimate the load as an input function to obtain the proposed output. The authors concluded that the overall economic benefit of a grid-connected power solar plant lies in the reduction of the monthly electricity received from the grid and the reduction of diesel consumption required when the grid cannot provide electricity, making the PV plant a green energy contributor. Manoj Kumar et al. (2017) assessed a 1 MW solar PV power plant at the UMP campus in Malaysia; the authors used the PV Geographical Information System (PVGIS) and PV Watt software for their simulation. The result showed that 1390 MWh of electricity was generated with a GHG emission reduction of 818.71 t CO2. The authors concluded that the result provided 5% of the total energy required on campus and that both software programs agreed on the amount of predicted energy production.
Jumaat et al. (2018) modeled a 120-W monocrystalline photovoltaic module using MATLAB Simulink to estimate the efficiency and performance of the PV module. The input parameters for the study, which consist of solar irradiance and temperature in Parit Raja (1.86°N and 103.12°E), were obtained from RETScreen software. After comparing the theoretical I-V and P-V curves, the result was shown in the I-V and P-V curves, and it was concluded that the Su-Kam PV module works efficiently with a complete performance at standard test conditions. Afzal et al. (Muhammad Azeem et al., 2017) conducted a feasibility and financial analysis of solar and wind power plants. The authors developed the study through RETScreen Expert. The system consists of a PV and wind turbine project model with a combination of solar and wind energy to address the electricity shortfall in Pakistan. Three cases were considered for generating 10,000 MW by retaining the same electricity prices of $0.13/kWh throughout the simulation. The results showed an equity payback of 18 years, 19.2 years, and 18.5 years, respectively.
A feasibility study of a small stand-alone PV system was carried out by Thompson and Duggirala (2009) in Canada, and the economic study of the system was determined for south Kenora, Ontario, Canada. This project, which used RETScreen International 4.0, considered the economic and environmental costs of generating 100 kW of electric power after considering biomass, wind, and PV sources. The authors concluded that combining the two systems gave a payback period of 1.4 years compared to an existing hybrid. Liqun and Chunxia (Liqun and Chunxia, 2023) conducted a feasibility analysis of a hybrid wind-PV system in Dongwangsha on Chongming Island in Shanghai. The study used RETScreen software and National Aeronautics and Space Administration (NASA) data for analysis. The result revealed the percentage of electricity that could be generated by a wind turbine (82.1%) and PV (16.2%) with an equity payback of 1.8 years. The authors inferred that feasibility analysis is essential for selecting the appropriate hybrid renewable power system based on local weather conditions. An availability study of a grid-connected PV system for the rural area of Rajasthan was carried out by Nsafon et al. (2020). In their study, the authors determined the electrical energy demand for the village through a PV energy system using RETScreen Expert. The PV power plant’s proposed annual energy of 600 kW was evaluated using the online tools PVGIS and PV Watt. It was concluded that the result had a good agreement and encouraged the use of PV systems.
The main idea of this study is to assess the viability of a ground-mounted, 1 MW grid-connected PV system using RETScreen Expert software. RETScreen offers comprehensive functionality that allows users to assess the feasibility of renewable energy projects by evaluating technical, economic, and environmental aspects on a single platform. This holistic approach enables well-informed decision-making. This study involves conducting techno-economic and environmental analyses to determine the project’s viability. This research is essential as it aims to illustrate to the educational institutions’ administrations the potential impact of PV installation on reducing the campus’s carbon footprint from non-renewable energy sources. Several countries, including Nigeria, have implemented carbon neutrality programs to achieve decarbonization in response to environmental concerns. Ensuring consistent energy for the activities on Nigerian university campuses is expensive, with some institutions paying up to $140,000 per month, not including running diesel generators during sessions (Alabi, 2022). With this in mind, conducting a techno-economic feasibility assessment is critical to determine what additional generation can be added to the current power system to satisfy growing electrical needs, reduce operating costs, and transition to a cleaner and more reliable supply.
2 Materials and methods
2.1 Location of the study
The Federal Polytechnic Mubi (FPM), situated in Mubi, Adamawa State, Nigeria, was selected as the location for the study. FPM is 180 km from Yola, the capital of Adamawa State. Geographically, FPM is located in north-eastern Nigeria and has a total area of 750 ha. The school’s main campus, in Mubi, is geographically located at 10°16′44″N and 13°17′25″E, and the elevation above sea level is about 559 m. FPM has another campus in Yola. The school is ranked as one of the best polytechnics in northern Nigeria. FPM has four schools divided into seven departments. The staff and student population of the main campus is 25,000. The main campus consists of well-established facilities, infrastructure, and social amenities. As an institution, FPM promotes renewable and sustainable energy usage on campus, leading to the reduction of global warming and air pollution, and supports the federal government in promoting renewable energy in Nigeria. The selected campus regions are shown in Figure 1, and the site information is provided in Table 1.
2.2 Distribution of the proposed grid-connected PV power plant
The proposed 1 MW ground-mounted grid-connected solar PV power plant is novel in Nigeria because most solar systems in Nigeria are designed to supply power for 6 h–12 h per day, depending on the load required by the users (Akinyele et al., 2015a). The proposed 1 MW PV system is expected to meet the electricity demand of the Federal Polytechnic Mubi campus for 24 h for more than 20 years. The power plant is convenient with no interruption during operation and requires fewer operating and maintenance costs throughout the plant’s lifecycle. The installation of this proposed power plant will not only improve the standard of living of both the staff and students of the institution but also for nearby communities. All possibilities were considered when designing the proposed 1 MW ground-mounted PV power plant to meet the current energy needs and supply excess energy to nearby communities to support the Federal Government’s renewable energy program. Özbek and Çelik (2022) listed solar modules, inverters, mounting grid connections, and DC/AC cables as some of the critical components needed for grid-connected PV power plants with the specifications provided by manufacturers. The different types of solar modules produced presently are categorized based on the materials used by the manufacturing industries (Seme et al., 2019). The crystalline solar modules are widely used in large-scale PV power plant installations around the world. Four 250 kW inverters were selected for the 1 MW PV plant. The solar panels will be mounted at an optimal angle per the site conditions; the latitude of the particular location was chosen to obtain maximum orientation (Seme et al., 2019). The proposed 1 MW solar plant components and their respective specifications are shown in Figure 2. The excess power generated by the power plant would be supplied to the grid; in case of a power shortage, the system would receive and use electricity from the grid (Olanrewaju et al., 2019).
2.2.1 Proposed solar PV plant area
A typical 1 MW ground-mounted solar PV plant requires five acres of land area, and the space required can either be open land or on top of a building (Atsu et al., 2021). For this project, the PV plant’s total area is 0.6382 ha (6,382 m2) of the 750 ha acquired by the Federal Polytechnic Mubi campus.
2.2.2 Tilt angle optimization
Nigeria experiences a considerable amount of solar radiation because of its global location (Elhab, 2012). The tilt angle used in this proposed power plant is the value of the latitude of the site location, as it is the best way to obtain maximum absorption of solar radiation. The tilt angle considered for this PV plant is 10°16′44″. The simulation study was conducted using the RETScreen software tool to obtain the maximum optimized value.
2.2.3 PV solar panel used
The China Sunergy mono-si-CSUN200-72M solar PV module is used for the solar power plant with a power rating of 200 W, as shown in Table 2. To meet the requirements of the inverter, the PV panels will be connected in series to form a PV module string. A total of 426 panels are needed for the project. As mentioned previously, the maximum power (
where
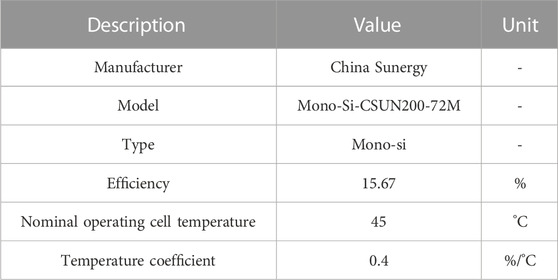
TABLE 2. Manufacturer specifications of China Sunergy mono-Si-CSUN200-72M under standard test conditions (STC).
Equation 2 was used to select the proposed plant’s suitable PV module.
2.2.4 Inverter
An inverter is a device that converts DC to AC. It is key to the safety, efficiency, and reliability of any PV system setup, and the sizing of the inverter for this study is given in Equation 3. The inverter is also designed to handle high voltage and current under adverse weather conditions. The inverter’s ingress protection and efficiency are the two major factors determining its lifespan (Owolabi et al., 2019). The Digital Luminous inverter with a rating capacity of 10 kVA/180 V was selected for this proposed 1-MW solar PV power plant; 125 pieces is needed. Hence, the efficiency of this inverter is 95%, and it would be connected to meet the proposed PV power plant requirement.
2.2.5 Economic analysis
The current study used RETScreen software to assess the grid-connected PV system’s techno-economic viability. It was also used to examine the effects of tilt angles on the PV power output. These measures of economic viability were assessed to evaluate the profitability and return on investment of the suggested PV project.
The capacity factor (CF) is the actual power generated by the PV system during the time under consideration compared to the amount of energy that could have been produced during the same time under continuous full-power operation. The capacity factor is expressed in Equation 4.
The net present value (NPV) is the after-tax cash flow for the year.
The levelized cost of energy (LCOE) is the average net present cost of electricity generation for a generator throughout its lifetime, which is calculated using Equation 6:
Simple payback (SP) measures how long it will take for a planned facility to recoup its initial investment in terms of savings or revenue. It is expressed in Equation 7.
Equity payback (EP) is simply the amount of time it takes to recoup the cost of an investment. It is expressed in Equation 8.
Annual life cycle savings (ALCS) represents the annual savings or revenue realized as a result of putting the proposed system into practice and is calculated using Equation 9.
where
2.3 Simulation software package
RETScreen Expert is a clean energy management software that enables professionals and decision-makers to identify the potential of energy efficiency and renewable energy projects. Natural Resources Canada developed the program on 30 April 1998 (Umar et al., 2018). The software integrates with many databases, such as a global climatic conditions database, the NASA satellite database, a benchmark database, a cost database, and a hydrology database. The software can perform energy, cost, emission, financial, sensitivity, and risk analyses (Khandelwal and Shrivastava, 2017). The simulation procedure is shown in Figure 3.
2.4 Solar radiation potential
Studies of potential solar radiation at the site where the PV power plant will be installed are important. Conditions like solar radiation, air temperature, and relative humidity vary from location to location. According to Giwa et al. (2017), solar radiation in Nigeria ranges from 12.6 MJ/m2/day to 25.2 MJ/m2/day in the coastal and northern areas, respectively. This study takes the selected location’s solar radiation potentials and air temperatures from the RETScreen Expert databases. The daily solar radiation horizontal (DSRH) and air temperature (AT) of the chosen area are shown in Figure 4. From the simulated results, the maximum solar radiation of 6.55 kWh/m2/day was obtained in March, followed by 6.36 kWh/m2/day in February. The lowest solar radiation of 4.75 kW/m2/day was obtained in August. Similarly, the maximum air temperature of 30°C was obtained in March, 29.5°C in April and 24.40°C in August.
3 Results and discussions
3.1 Monthly variation in energy generation
Monthly energy generation variations are clearly observed from simulated studies in RETScreen Expert software, as shown in Figure 5. The simulation revealed that the annual PV energy production was 1,505.98 MWh for an annual average solar irradiation of 5.74 kW/m2/day. March generated the maximum energy, 146.89 MWh, while the minimum was generated in August, 106.73 MWh.
3.2 Emission analysis
The proposed 1 MW PV power plant is not fully considered a zero-carbon power generation system. Thus, there is a need to estimate the CO2 t/kWh released by the plant. The proposed case against the coal-based power plant’s CO2 emissions is known as the base case, as shown in Figure 6. RETScreen Expert has generated GHG emissions from the PV plant. Similarly, RETScreen was used to calculate the revenue that can be generated from the sale of GHG reduction emissions. Transmission and distribution (T&D) losses of 16% were used as stated in Yakub et al. (2022), and a GHG emission factor of 0.433 tCO2/MWh was used in Nigeria’s base case electricity system. The software can calculate the selected location’s gross annual GHG emission reduction, which is the difference between the proposed case and the base case. The GHG emission for the base case is 721.4 tCO2/MWh, and the proposed case is 50.5 tCO2/MWh. Therefore, the site’s gross annual GHG emission reduction is 670.9 tCO2/MWh, which is equivalent to 61.7 ha of forest-absorbing carbon that will not be cut down for the project’s lifespan. The software also calculates the GHG reduction revenue, as shown in Figure 6. This is done to show how to generate revenue through carbon trading.
3.3 Financial analysis of the proposed 1MW solar PV power plant
Financial analysis is essential for the development and technical feasibility of any project. The financial analysis cannot be perfect due to various uncertainties associated with the calculation. Some of the parameters used in the study as input variables are standards obtained directly from the software’s built-in financial parameters, including the inflation rate, discount rate, reinvestment rate, debt ratio, and debt interest rate (Owolabi et al., 2019). However, the initial cost and the operation and maintenance cost were manually inputted, as shown in Table 3.
The software generated the outputs, such as the internal rate of return, net present value (NPV), annual life cycle savings, benefit-cost (B-C) ratio, debt service cost, energy production cost ($/kWh), payback period, and equity payback period, as shown in Table 4 from the input variables.
According to Yakub et al. (2022), the project’s feasibility and acceptability depend on the NPV, internal rate of return (IRR), and payback period. The NPV is the difference between the present value of cash inflows and the current value of cash outflows over time for a project. The result in Table 4 shows that if the NPV of the project is positive, then the project is economically viable and sustainable for investors (Yakub et al., 2022; Yaniktepe et al., 2017). The result also showed that the IRR, which reveals the project’s profitability, is higher than the required rate of return (Yakub et al., 2022). This also makes the project acceptable if the rate of return is equal to the discount rate. The solar power plant project has a payback period of 12 years, the time required to recoup the cost of the initial investment in the project. With this payback period, the solar PV plant makes financial sense at this location. The equity payback, or the number of years required to recoup the equity investment in the project from pre-tax cash flows reflecting inflation and debt payments, shown in Figure 7, is 10 years. The simulation results imply that the proposed project will yield interest in the chosen location for 13 of its anticipated 25 years of service.
3.4 Solar PV plant risk analysis
Risk analysis allows varying all parameters within a specific range before concluding the simulation (Owolabi et al., 2020). In this study, energy production cost was selected as the financial indicator, and its range is ±25% for all parameters involved. The software’s built-in Monte Carlo simulation was used to run 4,500 iterations. The result is displayed as the impact and distribution graphs shown in Figures 8, 9. The variation in the energy production cost yield results from the different parameters, as shown in Figure 8. The result revealed that the initial cost and the electricity exported to the grid connection significantly impact the proposed project in opposite directions. Thus, the higher the initial cost, the higher the energy production cost, while the more electricity exported to the grid, the lower the energy production cost. To exceed the 90% confidence level (Pr 90 value) of the energy production cost, the risk level was set to 20% by excluding 10% for the lower level and 10% for the upper level, as shown in Figure 9. According to the result obtained, the Pr 90 energy production cost was $107/MWh, which is slightly above the energy production cost of $93/MWh at the proposed site; this value revealed that the project is bankable and realistic (Akinyele et al., 2015b).
3.5 Performance comparison using RETScreen Expert Software
The performance of the proposed 1 MW solar PV power system for Federal Polytechnic Mubi, Main Campus, was compared using RETScreen Expert software. The results of this simulation study were compared with the few existing studies of plants of similar capacity. The result obtained from the present study is the same as the study of Bano and Rao (2016) in Jaipur, India, but slightly higher than the study of Manoj Kumar et al. (2017) and Yaniktepe et al. (2017), while the work of Kumi and Brew-Hammond (2013) and Kazem et al. (2017) was slightly higher than the present study. The study of Poullikkas (2009) had the highest annual energy generation, as shown in Table 5.
4 Conclusion
RETScreen Expert software was used to analyze the feasibility of installing a 1 MW grid-connected PV power plant at the Federal Polytechnic Main Campus in Adamawa State, Nigeria. This was performed by considering the project’s technical, financial, risk, and environmental impacts on the community. In this study, some parameters used as input to the software are the inflation rate, discount rate, location weather data, reinvestment rate, and debt interest rate. The simulation was performed to calculate the energy required to be exported to the grid, the amount of GHG emission reduction, the total initial cost, the GHG emission revenue over the lifetime of the project, the sales of energy exported to the grid, and the number of forest-equivalent hectares that would be needed to absorb the carbon from fossil-fuel-based methods of generating electricity. It was shown from the results that a maximum annual energy production of 1,550.98 MWh could be exported to the grid. In addition, the simulation result shows that the calculated payback period of the PV solar plant is 11.4 years, and its equity payback period is 10 years. The project showed a positive value of $677,471 for the NPV with an IRR of 11.9%, making the project viable and economically sustainable. In addition, the project reduced GHG emissions by 670.9 tCO2, equivalent to 61.7 ha of forest-absorbing carbon emissions. The findings also indicated a correlation between increased electricity export to the grid and decreased energy production costs at the Pr 90% level.
Data availability statement
The original contributions presented in the study are included in the article/Supplementary material; further inquiries can be directed to the corresponding authors.
Author contributions
RL: Conceptualization, Formal analysis, Writing—original draft. AKI: Writing—original draft Resources, Visualization. ABO: Data curation, Methodology, Software. AOY: Investigation, Methodology, Review. NNS: Review, Methodology, Formal analysis; AY: Methodology, Data curation; Visualization. NY: Data curation; Review. MK: Investigation, Software, Resources. DS: Funding acquisition, Supervision, Validation. J-SH: Supervision, Resources.
Funding
This work was supported by the National Research Foundation of Korea (NRF), a grant from the Ministry of Science and ICT (MSIT) of the Government of the Republic of Korea (NRF-2021R1A5A8033165), the Korea Institute of Energy Technology Evaluation and Planning (KETEP), and the Ministry of Trade, Industry, and Energy (MOTIE) of the Republic of Korea (20224000000150).
Conflict of interest
The authors declare that the research was conducted in the absence of any commercial or financial relationships that could be construed as a potential conflict of interest.
Publisher’s note
All claims expressed in this article are solely those of the authors and do not necessarily represent those of their affiliated organizations, or those of the publisher, the editors, and the reviewers. Any product that may be evaluated in this article, or claim that may be made by its manufacturer, is not guaranteed or endorsed by the publisher.
References
Aba, M., Ladeinde, A., Afimia, E., Tsai, H.-L., and Muhammad Lawan, S. (2019). Economic evaluation of hybrid renewable energy systems for electricity generation in Nigeria: A discounted cash flow analysis. J. Energy Res. Rev. 2 (2), 1–10. doi:10.9734/JENRR/2019/V2I230075
Adesanya, A. A., and Schelly, C. (2019). Solar PV-diesel hybrid systems for the Nigerian private sector: An impact assessment. Energy Policy 132, 196–207. doi:10.1016/j.enpol.2019.05.038
Akinyele, D. O., Rayudu, R. K., and Nair, N. K. C. (2015a). Development of photovoltaic power plant for remote residential applications: The socio-technical and economic perspectives. Appl. Energy 155, 131–149. doi:10.1016/J.APENERGY.2015.05.091
Akinyele, D. O., Rayudu, R. K., and Nair, N. K. C. (2015b). Development of photovoltaic power plant for remote residential applications: The socio-technical and economic perspectives. Appl. Energy 155, 131–149. doi:10.1016/j.apenergy.2015.05.091
al Garni, H., Kassem, A., Awasthi, A., Komljenovic, D., and Al-Haddad, K. (2016). A multicriteria decision making approach for evaluating renewable power generation sources in Saudi Arabia. Sustain. Energy Technol. Assessments 16, 137–150. doi:10.1016/J.SETA.2016.05.006
Alabi, M. (2022). High electricity tariff may cripple Nigerian universities – unilag VC. Available at: https://www.premiumtimesng.com/news/top-news/433270-high-electricity-tariff-may-cripple-nigerian-universities-unilag-vc.html (accessed September 01, 2022).
Alsharif, M. H. (2017). Techno-economic evaluation of a stand-alone power system based on solar power/batteries for global system for mobile communications base stations. Energies (Basel) 10, 392–393. doi:10.3390/EN10030392
Atsu, D., Seres, I., and Farkas, I. (2021). The state of solar PV and performance analysis of different PV technologies grid-connected installations in Hungary. Renew. Sustain. Energy Rev. 141, 110808. doi:10.1016/j.rser.2021.110808
Baitule, A. S., and Sudhakar, K. (2017). Solar powered green campus: A simulation study. Int. J. Low-Carbon Technol. 12 (4), 400–410. doi:10.1093/IJLCT/CTX011
Bano, T., and Rao, K. (2016). Performance analysis of 1MW grid connected photovoltaic power plant in Jaipur. India: IEEE, 165–170. doi:10.1109/ICEETS.2016.7582919
Bhuyan, S., Singh, K., Kumar, M. N., and Mishra, S. (2017). “A study on performance analysis of 75kWp grid connected solar power plant at MEMS,” in Proceedings of the 2017 International Conference on Computer, Electrical and Communication Engineering, Kolkata, India, 22-23 December 2017. doi:10.1109/ICCECE.2017.8526218
Eke, R., and Demircan, H. (2013). Performance analysis of a multi crystalline Si photovoltaic module under Mugla climatic conditions in Turkey. Energy Convers. Manag. 65, 580–586. doi:10.1016/j.enconman.2012.09.007
Elhab, B. R. (2012). Optimizing tilt angles and orientations of solar panels for Kuala Lumpur, Malaysia. Sci. Res. Essays 7 (42), 3758–3765. doi:10.5897/SRE12.241
Giwa, A., Alabi, A., Yusuf, A., and Olukan, T. (2017). A comprehensive review on biomass and solar energy for sustainable energy generation in Nigeria. Renew. Sustain. Energy Rev. 69, 620–641. doi:10.1016/j.rser.2016.11.160
Goura, R. (2014). Analyzing the on-field performance of a 1-megawatt-grid-tied PV system in South India. Int. J. Sustain. Energy 34, 1–9. doi:10.1080/14786451.2013.824880
Humada, A. M., Hojabri, M., Hamada, H. M., Samsuri, F. B., and Ahmed, M. N. (2016). Performance evaluation of two PV technologies (c-Si and CIS) for building integrated photovoltaic based on tropical climate condition: A case study in Malaysia. Energy Build. 119, 233–241. doi:10.1016/j.enbuild.2016.03.052
Jamil, M., Kirmani, S., Rizwan, M., Jamil, M., Kirmani, S., and Rizwan, M. (2012). Techno-economic feasibility analysis of solar photovoltaic power generation: A review. Smart Grid Renew. Energy 3 (4), 266–274. doi:10.4236/SGRE.2012.34037
Jumaat, S. A., Ab Majid, A. A. S., Abdullah, M. N., Radzi, N. H., Hamdan, R., and Salimin, S. (2018). Modeling of 120W monocrystalline photovoltaic module using MATLAB simulink. Indonesian J. Electr. Eng. Comput. Sci. 11 (1), 74–81. doi:10.11591/IJEECS.V11.I1.PP74-81
Kazem, H. A., Albadi, M. H., Al-Waeli, A. H. A., Al-Busaidi, A. H., and Chaichan, M. T. (2017). Techno-economic feasibility analysis of 1 MW photovoltaic grid connected system in Oman. Case Stud. Therm. Eng. 10, 131–141. doi:10.1016/J.CSITE.2017.05.008
Khandelwal, A., and Shrivastava, V. (2017). Viability of grid-connected solar PV system for a village of Rajasthan. undefined 22, 1–6. doi:10.1109/ICOMICON.2017.8279175
Kumi, E. N., and Brew-Hammond, A. (2013). Design and analysis of a 1 MW grid-connected solar PV system in Ghana. Nairobi, Kenya: African Technology Policy Studies Network.
Liqun, L., and Chunxia, L. (2023). Feasibility analyses of hybrid wind-PV-battery power system in Dongwangsha, Shanghai. Available at: http://pe.org.pl/articles/2013/1a/58.pdf (Accessed June 15, 2023).
Manoj Kumar, N., Sudhakar, K., and Samykano, M. (2017). Techno-economic analysis of 1 MWp grid connected solar PV plant in Malaysia. Int. J. ambient energy 40 (4), 434–443. doi:10.1080/01430750.2017.1410226
Martínez-Díaz, M., Villafáfila-Robles, R., Montesinos-Miracle, D., and Sudrià-Andreu, A. (2013). Study of optimization design criteria for standalone hybrid renewable power systems. Renew. Energy Power Qual. J. 1 (11), 1266–1270. doi:10.24084/REPQJ11.598
Muhammad Azeem, A., Abrar, A., Saqib, H., and Talat Farid, A. (2017). Pre-feasibility financial analysis of solar and wind power plants by RET Screen Expert, considering total energy shortfall in Pakistan. Acad. J. Sci. Res. 5 (9), 379–389. doi:10.15413/ajsr.2017.0420
Nsafon, B. E. K., Owolabi, A. B., Butu, H. M., Roh, J. W., Suh, D., and Huh, J. S. (2020). Optimization and sustainability analysis of PV/wind/diesel hybrid energy system for decentralized energy generation. Energy Strategy Rev. 32, 2020. doi:10.1016/J.ESR.2020.100570
Ogunmodimu, O., and Okoroigwe, E. C. (2018). Concentrating solar power technologies for solar thermal grid electricity in Nigeria: A review. Renew. Sustain. Energy Rev. 90, 104–119. doi:10.1016/j.rser.2018.03.029
Okoye, C. O., Taylan, O., and Baker, D. K. (2016). Solar energy potentials in strategically located cities in Nigeria: Review, resource assessment and PV system design. Renew. Sustain. Energy Rev. 55, 550–566. doi:10.1016/J.RSER.2015.10.154
Olanrewaju, F. O., Andrews, G. E., Li, H., and Phylaktou, H. N. (2019). Bioenergy potential in Nigeria. Chem. Eng. Trans. 74, 61–66. doi:10.3303/CET1974011
Ong, H. C., Mahlia, T. M. I., and Masjuki, H. H. (2011). A review on energy scenario and sustainable energy in Malaysia. Renew. Sustain. Energy Rev. 15 (1), 639–647. doi:10.1016/j.rser.2010.09.043
Owolabi, A. B., Nsafon, B. E. K., Huh, J. S., and Suh, D. (2019). Validating the techno-economic and environmental sustainability of solar PV technology in Nigeria using RETScreen Experts to assess its viability. Sustain. Energy Technol. Assessments 36, 100542. doi:10.1016/J.SETA.2019.100542
Owolabi, A. B., Emmanuel Kigha Nsafon, B., Wook Roh, J., Suh, D., and Huh, J. S. (2020). Measurement and verification analysis on the energy performance of a retrofit residential building after energy efficiency measures using RETScreen Expert. Alexandria Eng. J. 59 (6), 4643–4657. doi:10.1016/j.aej.2020.08.022
Özbek, N. S., and Çelik, Ö. (2022). Design and analysis of a novel adaptive learning control scheme for performance promotion of grid-connected PV systems. Sustain. Energy Technol. Assessments 52, 102045. doi:10.1016/j.seta.2022.102045
Pal, A. M., Das, S., and Raju, N. B. (2015). Designing of a standalone photovoltaic system for a residential building in gurgaon, India. Sustain. Energy 3, 14–24. doi:10.12691/RSE-3-1-3
Poullikkas, A. (2009). Parametric cost–benefit analysis for the installation of photovoltaic parks in the island of Cyprus. Energy Policy 37 (9), 3673–3680. doi:10.1016/J.ENPOL.2009.04.037
Raji, L., Amusat, R. O., Anjorin, O. J., Idris, M. H., and Issa, A. K. (2020). Predictions of daily horizontal solar radiation for rural development: The case of Mubi town, Adamawa state, Nigeria. Int. J. Appl. Technol. Res. 1 (1), 38–44. doi:10.35313/IJATR.V1I1.22
Renewable Energy Agency, I. (2014). REthinking Energy: Towards a new power system. [Online]. Available at: www.irena.org.
Reuters (2022). Nigerian power problems old and new frustrate Buhari's economy push | Reuters. Available at: https://www.reuters.com/article/nigeria-power-idUSL8N1CW56I (accessed September 05, 2022).
Ruiz-Cabrero, J., Boer, W., and Pita, M. (2022). Unlocking Nigeria's potential: The path to well-being. Available at: https://www.bcg.com/publications/2016/public-sector-globalization-unlocking-nigerias-potential (accessed September 05, 2022).
Same, N. N., Yakub, A. O., Kigha Nsafon, B. E., Owolabi, A. B., Mih, T. A., Suh, D., et al. (2022). Performance evaluation of renewable-based sustainable micro-grid under predictive management control strategy: A case study of gado refugee camp in Cameroon. Front. Energy Res. 10, 1274. doi:10.3389/FENRG.2022.987495
Seme, S., Sredenšek, K., Štumberger, B., and Hadžiselimović, M. (2019). Analysis of the performance of photovoltaic systems in Slovenia. Sol. Energy 180, 550–558. doi:10.1016/j.solener.2019.01.062
Shukla, A. K., Sudhakar, K., and Baredar, P. (2016). Design, simulation and economic analysis of standalone roof top solar PV system in India. Sol. Energy 136, 437–449. doi:10.1016/J.SOLENER.2016.07.009
Soonmin, H., Lomi, A., Okoroigwe, E. C., and Urrego, L. R. (2019). Investigation of solar energy: The case study in Malaysia, Indonesia, Colombia and Nigeria. Int. J. Renew. Energy Res. 9 (1), 86–95. doi:10.20508/IJRER.V9I1.8699.G7620
Thompson, S., and Duggirala, B. (2009). The feasibility of renewable energies at an off-grid community in Canada. Renew. Sustain. Energy Rev. 13, 2740–2745. doi:10.1016/j.rser.2009.06.027
Umar, N., Bora, B., Banerjee, C., and Panwar, B. S. (2018). Comparison of different PV power simulation softwares: Case study on performance analysis of 1 MW grid-connected PV solar power plant. Available at: www.ijesi.org||Volumewww.ijesi.org (Accessed September 05, 2022).
Yakub, A. O., Same, N. N., Owolabi, A. B., Nsafon, B. E. K., Suh, D., and Huh, J.-S. (2022). Optimizing the performance of hybrid renewable energy systems to accelerate a sustainable energy transition in Nigeria: A case study of a rural healthcare centre in kano. Energy Strategy Rev. 43, 100906. doi:10.1016/j.esr.2022.100906
Keywords: solar photovoltaic, RETScreen expert, solar energy, renewable energy, techno-economic, sustainability
Citation: Luqman R, Kehinde Issa A-J, Owolabi AB, Yakub AO, Same NN, Yahaya A, Yasin N, Kavgic M, Suh D and Huh J-S (2023) Assessing the viability of a grid-connected PV power plant in Mubi, Adamawa State, Nigeria. Front. Energy Res. 11:1205646. doi: 10.3389/fenrg.2023.1205646
Received: 14 April 2023; Accepted: 31 May 2023;
Published: 23 June 2023.
Edited by:
Nallapaneni Manoj Kumar, City University of Hong Kong, Hong Kong SAR, ChinaReviewed by:
Rasaq Lamidi, University of Birmingham, United KingdomAyodele Osalade, Ekiti State University, Nigeria
Aliu Olamide Oyedun, Hamburg University of Technology, Germany
Copyright © 2023 Luqman, Kehinde Issa, Owolabi, Yakub, Same, Yahaya, Yasin, Kavgic, Suh and Huh. This is an open-access article distributed under the terms of the Creative Commons Attribution License (CC BY). The use, distribution or reproduction in other forums is permitted, provided the original author(s) and the copyright owner(s) are credited and that the original publication in this journal is cited, in accordance with accepted academic practice. No use, distribution or reproduction is permitted which does not comply with these terms.
*Correspondence: Dongjun Suh, dongjunsuh@knu.ac.kr