- 1School of Energy and Environment, City University of Hong Kong, Kowloon, Hong Kong SAR, China
- 2Center for Research and Innovation in Science, Technology, Engineering, Arts, and Mathematics (STEAM) Education, HICCER—Hariterde International Council of Circular Economy Research, Palakkad, Kerala, India
- 3Swiss School of Business and Management Geneva, Genève, Switzerland
- 4Department of Computer, Control and Management Engineering, Sapienza University of Rome, Roma, Italy
- 5Department of Civil and Environmental Engineering, Indian Institute of Technology Patna, Bihar, India
- 6Centre for Plant and Environmental Biotech, Amity Institute of Biotechnology, Amity University, Noida, India
- 7Czech Technical University in Prague, University Centre for Energy Efficient Buildings, Buštěhrad, Czech Republic
- 8Department of Industrial and Information Engineering and Economics, University of L’Aquila, L’Aquila, Italy
Editorial on the Research Topic
Sustainable planning and lifecycle thinking of energy infrastructure
1 Energy infrastructure
Energy infrastructure refers to the systems, structures, and facilities that are designed to generate, transmit, and distribute energy (Bridge et al., 2018). Energy infrastructure is a critical component of modern societies and is essential for powering homes, businesses, and industries (Goldthau, 2014; Pandey, 2020). The types of energy infrastructure vary depending on the energy source and other things in its value chain. For example, traditional utilities like gas and pipelines, power generation systems for fossil fuels and renewable ones, electrical transmission lines, electrical metering and distribution systems, smart systems, storage facilities, advanced electric and electronic systems, and various types of power control systems (Bridge et al., 2018; Pandey, 2020). In addition, today’s energy infrastructure includes many directly or indirectly related industrial systems and numerous interdependent sectors like transportation (electric vehicles) (Nallapaneni, 2022a), food and agriculture (farm machinery, drying, agrivoltaics) (Chand et al., 2021; Nallapaneni et al., 2021; Poonia et al., 2022).
Energy infrastructure can also refer to the policies, regulations, and institutions that govern energy production and consumption (Goldthau, 2014; Bridge et al., 2018). These can include things like energy conservation programs, renewable energy standards, and energy market regulations. Furthermore, energy communities represent a new social model for the energy transition (D’Adamo et al., 2022). Overall, energy infrastructure is a complex and multifaceted system that plays a crucial role in the functioning of modern societies and is a key driver of global economic growth and competitiveness.
2 Importance of planning energy infrastructure and its implementation
Planning is crucial for energy infrastructure because the development and operation of energy infrastructure can have significant environmental, economic, and social impacts. They are often large-scale and require long-term investments; if we want to list the reasons; there are several reasons, some are listed below.
2.1 Environmental impact
Energy infrastructure projects can have significant environmental impacts, including land-use changes, habitat destruction, air and water pollution, and others. Proper planning can help identify potential environmental impacts and mitigate them through the use of cleaner technologies, siting decisions, and other measures.
2.2 Cost-effectiveness
Energy infrastructure projects are often large and expensive, so careful planning is needed to ensure that they are cost-effective. This includes identifying the most efficient and effective technologies, optimizing the project design, and ensuring that the project is built and operated in a way that maximizes its energy output and minimizes its operating costs.
2.3 Social acceptance
Energy infrastructure projects can also have significant social impacts, including displacement of communities, impacts on cultural resources, and impacts on public health and safety. Proper planning can help identify potential social impacts and develop strategies to mitigate them, including engaging with local communities, developing public health and safety plans, and providing adequate compensation and support for affected communities.
2.4 Regulatory compliance
Energy infrastructure projects are subject to a range of regulatory requirements, including environmental permits, land-use approvals, and safety regulations. Proper planning is essential to ensure that the project is designed and implemented in compliance with all applicable regulations and requirements.
2.5 Interdependencies
Energy infrastructure projects have seen many technological advancements in recent years, especially from an application point of view in various other infrastructure sectors. As a result, systems like renewable-powered desalination (Vishnupriyan et al., 2021), agrivoltaics (Nallapaneni et al., 2021), grapevoltaics (Nallapaneni and Chopra, 2021a; Kumar and Chopra, 2023), vehicle-integrated photovoltaics (Mamun et al., 2022), floating solar (Kumar et al., 2022), urban wind turbines (Dilimulati et al., 2018), integrated renewables-battery energy storage systems (Cucchiella et al., 2017) and others have become popular and created interdependencies in the context of climate change mitigation and decarbonization (Zhongming et al., 2018). Such interdependencies suggest the need for effective planning and better implementation of energy infrastructure to ensure the competitiveness of the energy and the other dependent sectors.
To summarise, proper planning is essential for energy infrastructure projects to ensure that they are designed and implemented in a way that is sustainable, cost-effective, and beneficial to society as a whole. However, when we think of planning and implementation, planning based on conventional feasibility studies is insufficient and should be from a life-cycle perspective (Kumar et al., 2023). Also, Sustainable Development Goal 7 (SDG7) advocates having energy infrastructure developments confined to set targets and indicators, broadly promoting the goal “ensure access to affordable, reliable, sustainable and modern energy for all” (UN SDG, 2022). On the other side, the energy infrastructure’s technology and policy drivers are rapidly changing, whereas the regulatory framework and permitting systems surrounding the energy infrastructure are currently designed for another age, making it difficult for implementation, modernization, expansion, decommissioning, and end-of-life (Nallapaneni, 2021; D’Adamo et al., 2023; Molla et al., 2023). Considering all such considerations, it is better to apply “sustainable planning and life-cycle thinking” to the energy infrastructure.
3 Approaches for sustainable planning and lifecycle thinking of energy infrastructure
There are several approaches for planning energy infrastructure, depending on factors such as the type of energy infrastructure being planned, the project’s scale, and the project’s goals. Some common approaches include.
3.1 Integrated resource planning (IRP)
IRP is a comprehensive planning process that considers all potential resources, including renewable energy, demand management, and energy efficiency measures. This approach aims to balance the cost, reliability, and environmental impacts of different resource options to meet the needs of customers (Beecher, 1995).
3.2 Scenario planning
Scenario planning involves developing multiple scenarios for the future based on different assumptions about energy demand, prices, and technological advancements. This approach helps planners identify potential risks and opportunities and develop strategies to address them (Ringland, 1998).
3.3 Stakeholder engagement
Stakeholder engagement involves involving a diverse group of stakeholders in the planning process, including community members, industry experts, and policymakers. This approach can help ensure that the infrastructure meets the needs of all stakeholders and that their concerns are addressed (Kujala et al., 2022).
3.4 Cost-benefit analysis (CBA)
CBA is a method of evaluating the costs and benefits of different energy infrastructure options. This approach can help identify the most cost-effective options for meeting energy needs (Layard, 1994).
3.5 Techno-economic assessment (TEA)
TEA is a method used to evaluate the feasibility and economic viability of energy infrastructure projects, such as renewable energy generation, transmission, and distribution. The TEA approach takes into consideration various factors such as the availability of natural resources, energy demand and consumption patterns, environmental impact, and regulatory framework. This can help make informed decisions on which energy infrastructure projects to pursue based on their economic and technical viability and their potential to meet the energy needs of the community while minimizing environmental impact (Burk, 2018).
3.6 Life cycle assessment (LCA)
LCA is a method of assessing the environmental impacts of different energy infrastructure options throughout their entire life cycle, from raw material extraction to disposal. This approach can help identify the most environmentally sustainable options (Hauschild et al., 2018).
3.7 Integrated TEA and LCA
Integrated TEA and LCA is a comprehensive approach that combines the evaluation of the economic and technical aspects of a technology or project with an analysis of its environmental impact over the entire life cycle of the product or service (Yuan et al., 2022). By integrating TEA and LCA, decision-makers can better evaluate the overall sustainability of a technology or project by considering not only its financial and technical feasibility but also its environmental impact. The combined approach enables the identification of trade-offs between economic and environmental goals and helps to identify opportunities for improvements in both areas.
3.8 Design in line with SDG7
Design in line with SDG7 refers to the practice of designing energy infrastructure and its systems and service following the targets of SDG7, i.e., to ensure access to affordable, reliable, sustainable, and modern energy for all. It was first proposed by (Kumar et al., 2020; Nallapaneni and Chopra, 2021b) for designing hybrid microgrids, see Figure 1A.
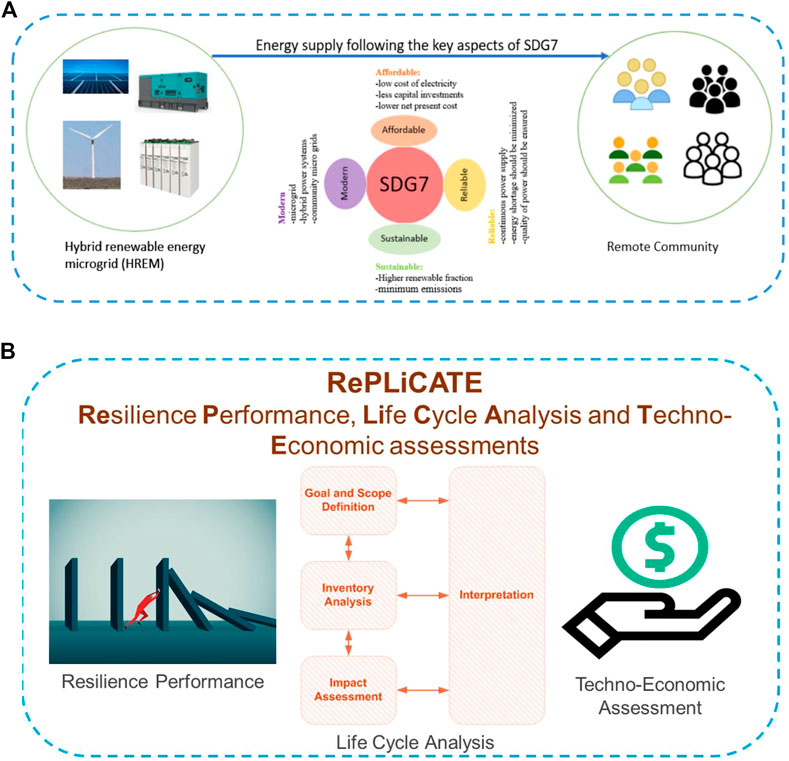
FIGURE 1. Approaches for Sustainable Planning and Lifecycle Thinking of Energy Infrastructure. (A) Energy Systems Design in line with SDG7. Adopted from authors own sources (Kumar et al., 2020; Nallapaneni and Chopra, 2021b); (B) RePLiCATE Approach. Adopted from authors own sources (Nallapaneni, 2021; Nallapaneni, 2022b).
3.9 RePLiCATE approach
RePLiCATE stands for Resilience Performance Life Cycle Analysis and Techno-Economics, Figure 1B. It is a framework proposed for the first time by (Nallapaneni, 2021) in his doctoral thesis, later in his young scientist talk in the HKICE Young Scientist Seminar Series at the Hong Kong Institute for Clean Energy, City University of Hong Kong, for sustainable and resilient planning of advanced clean energy systems (Nallapaneni, 2022b). RePLiCATE combines LCA, TEA, and resilience assessment to evaluate the environmental, economic, and social impacts of advanced clean energy systems across their life cycle stages (Nallapaneni, 2021; Nallapaneni, 2022b). The framework aims to provide decision-makers with a comprehensive understanding of the trade-offs between different performance indicators and identify sustainable and resilient strategies for energy infrastructure planning (Nallapaneni and Chopra, 2021a; Kumar and Chopra, 2023). Furthermore, RePLiCATE can also be integrated with the various SDGs to understand the energy infrastructure performance on a regional level based on the principles and methods as proposed by (D’Adamo et al., 2021).
4 Discussion on the research topic collection
This Research Topic in Frontiers in Energy Research intends to collect contributions that enable the sustainable transformation of energy infrastructure during its implementation, modernization, expansion, decommissioning, and end-of-life from a science, technology, engineering, and policy perspective. The overall response from the research, academic, and industry community for this Research Topic was amazing. A total of 22 articles were published and briefly fall under energy infrastructure dedicated to the power sector and other interdependent and dependent sectors.
4.1 Energy and supporting infrastructure dedicated to power sector
In energy infrastructure planning, first and foremost is to know the influential factors that influence the intentions of energy use and type of energy use, etc. Nazir & Tian (2022) investigated various factors influencing the intention to use renewable energy in energy infrastructure, such as social media exposure, relative advantage, ease of use, awareness, cost, attitude, and purchase intention, especially in Pakistan. The study uses the theory of planned behavior and the unified theory of acceptance and proposes hypotheses that are tested using structured questionnaires on a sample of 497 respondents. The findings show that attitude has a significant mediating effect on the relationship between purchase intention and factors such as social media exposure, relative advantage, ease of use, awareness, and cost. The study suggests that marketing approaches can be used to enhance customer purchase intention and help make decisions in the renewable energy sub-sector.
After understanding the intentions of use, energy systems design and placing them in the network either in a decentralized or centralized way matters. This phase is crucial where system design and related parameters or optimization that affect overall feasibility is given more importance. For instance, in (1). Navothna & Thotakura (2022) analyzes the performance of a 1 MWp solar photovoltaic (PV) plant in GITAM (Deemed to be University) in Andhra Pradesh, India. The study calculates efficiency, capacity factor, and performance ratio using simulated data and analyzes the PV modules’ light-induced degradation and degradation rate. (2). Desai et al. proposed the adoption of bifacial solar panels in rooftop PV systems in India and investigated the feasibility. The goal is to enhance energy generation as compared to mono-facial solar panels. The study presents the technical and economic advantages of using bifacial Si-modules in a typical 5-kW single-phase solar rooftop PV system. The study finds that bifacial modules generate more than 10% excess energy compared to the poly c-Si systems. Finally, the study finds that an optimized bifacial system tilted between 15° and 20° will reduce the levelized cost of electricity (LCOE) by 5.5% as compared to the traditional poly c-Si system. (3). Same et al. (2022) proposed a hybrid renewable energy system (HRESs) and a control system for a refugee camp to provide affordable, reliable, and clean energy. The techno-economic feasibility of the system was evaluated under load following, cycle charging, and predictive control strategy. The results showed that the predictive control strategy was the most suitable, with the lowest cost and highest eco-friendliness and energy efficiency. The total net present cost for electrification of the refugee camp was $3,809,822.54, and the cost of electricity generated was $0.2018 per kWh. The hybridization of the diesel generator under the predictive control strategy could avoid 991,240.32 kg of emissions annually. (4). Zhou et al. investigated the impact of displacement selections and heat exchanger arrangement on the performance of a 300 MW geothermal-aided power generation (GAPG) power plant. The results showed that using geo-fluid to displace high-grade extraction steam only can prevent silica scaling in the heat exchanger system, which can improve the performance of the GAPG plant. Additionally, the parallel arrangement of the heat exchanger is more effective than the series arrangement in terms of additional power output. The study also found that the GAPG plant has the potential to reduce carbon dioxide emissions by 13%, which highlights the environmental benefits of this technology. (5). Sivadanam et al. address the impact of inverter-based resources (IBRs) on the synchronous inertia of interconnected power systems (IPSs) and the need for faster frequency response requirements. The study proposes a stochastic and iterative-based optimization for load frequency control (LFC) using a hybrid energy storage system (HESS) with plug-in hybrid electric vehicles (PHEVs) and a superconducting magnetic energy storage (SMES) device. Two types of tie lines are investigated with optimal parameters recorded using particle swarm optimization (PSO) and biogeography-based optimization (BBO). The proposed BBO-based LFC ensures precision and accuracy, indicating improved IPS dynamic performance in smart cities. (6). Hussien et al. propose a method to minimize torque ripples in a surface-mounted permanent magnet synchronous motor using the variable switching frequency technique of pulse width modulation. The goal is to achieve high-performance operation of a motor-generator set. The effectiveness of the proposed control strategies is confirmed through obtained results to ensure controllability.
While developing infrastructure, it is crucial to check on the government subsidies and policies, if not atleast its outcome how the policy is performing to realise the climate goals. Wang et al. investigated the impact of government subsidies on pollution reduction in power companies in China. A fixed-effects panel data model was used to investigate the influence of subsidies on pollution reduction and how the mechanism works. The study found that subsidies can significantly reduce pollution, especially sulfur dioxide emissions and soot. Additionally, the results indicated that subsidies encourage power companies to reduce emissions through end-of-pipe control and green innovation. The study also found that subsidies have a better effect on regions with stronger environmental regulations, less economically developed regions, big-scale companies, and companies with low slack.
Other key aspect in infrastructure is its management, security and risk. For instance, in (1). Kong et al. described the design of a security risk management and control system for power trading institutions based on a Bayesian network to reduce the impact of risk on power trading projects. The risk evaluation is performed and effectively identifies risks; overall the system has high-risk management and control efficiency, with near-practical application value. (2). Zhang et al. proposed a simulation method to study the impact of the spot market on safety risks and generation benefits in the evolving electricity market considering actual cascade hydropower station (CHS). The hydraulic power matching among the CHSs is examined and the proposed method can help in making bidding decisions in spot transactions and can be useful during the transition period of electricity reform. This can also provide insights into the impact of new modes of a transaction on the electricity market and can help in identifying potential risks and opportunities. (3). Li and Hu (2022) addressed the Research Topic of accurately measuring and managing energy risk, which is critical for economic development and energy security. Instead of Value at Risk (VaR) method that is commonly available, this study proposes the use of Conditional VaR (CVaR) based on non-parametric kernel (NPK) framework. The results indicate that the NPK framework backed CVaR method is more effective in measuring actual energy risk and in carrying out risk hedging. Overall, the paper offers a more accurate and effective method to measure and manage energy risk, which is essential for promoting economic growth and energy security.
4.2 Energy and supporting infrastructure for interdependent and dependent sectors
Developing energy and supporting infrastructure from the context of interdependent and dependent sectors will lead to many new research areas. For instance, the Research Topic of co-producing energy and water is investigated by Murillo-Alvarado and Cardenas-Gil (2022) where they propose a mathematical optimization model to determine the feasibility of installing solar power generation plants and two scenarios are analyzed; one with variation in the operating time of the thermal storage system and the other with the incorporation of the rainwater harvesting system. Wu and Chul-Soo (2023) explored another application, i.e., the integration of solar photovoltaic collectors with vertical-green balconies in old high-rise buildings for water heating application. The study aims to conduct a preliminary research study on such integration possibilities with old buildings and explore various benefits such as water heating, energy-saving rate. The study suggests that the process-specific rationalization plan can be applied in future urban architecture renovation.
From a transportation sector application and its electrification point of view, (1). Chakraborty et al. presented the importance of sustainable mobility and the use of hydrogen fuel-based vehicular technologies to reduce greenhouse gas (GHG) emissions in the transportation sector. The study also provides an overview of various technologies with a different perception. (2). Tian et al. focuses on the effective utilization of coal liquefaction residue (CLR) through fluidized bed pyrolysis process that introduces green hydrogen and green oxygen to the process, achieving near-complete utilization of carbon and hydrogen elements in the CLR and producing high-quality liquid fuels and syngas. This new process can achieve almost near-zero carbon dioxide (CO2) emission in the entire unit which can facilitate sustainable development and improve the efficiency of coal conversion technology. (3). Chowdary and Sura (2022) proposes a solution to address concerns about charging infrastructure for electric vehicles (EVs) by designing and modeling a grid-connected photovoltaic-based microgrid. The study aims to analyze a hypothetical EV population charging while considering realistic EV loads and simulating the charging process. The study also analyzes the impact of weather parameters on power production and the impact of scaled EV sessions on microgrid power balances. The proposed solution could help address concerns about charging infrastructure for EVs and promote the adoption of renewable energy sources in the transportation sector. (4). Though renewables support transportation sector, there are some incidents where energy infrastructure may be considered as a risk. For instance, the wind turbines may be considered as potential obstructions to air traffic. Weigel et al. presented a multi-aspect holistic evaluation of aircraft detection lighting systems (ADLS) using a framework previously developed by the authors based on multi-criteria analysis (MCA), LCA, and expert interviews. The study presents information on potential implementation risks, bottlenecks, and levers for life cycle improvement.
From a supporting infrastructure for energy infrastructure, i.e., pipeline and gas network point of view, (1). Wang et al. used a stochastic process analysis of Research Topic evolution to trace and predict the technology trend of coal slurry pipeline transportation from patent texts. The study provides directions for sustainable research and development (R&D) activities in coal slurry pipeline transportation technology, facilitating interdisciplinary discussions and providing objective data for future decision-making by scientists and R&D managers in this field. (2). Wang et al. examined the impact of China’s third-party access policy on the efficiency of its natural gas network. They identify that the policy has resulted in a decoupling of gas trade and transport, which has led to underutilization of transmission resources due to the contractual arrangements between entry and exit capacities in the commercial network. To address this Research Topic, the authors propose a mathematical programming with equilibrium constraints (MPEC) model that integrates the allocations of commercial capacity and physical flows. Overall, the study highlights the importance of considering both commercial and physical aspects of natural gas transportation when designing policies to support the sustainable development of energy mix and low-carbon targets.
From an energy technology implementation and policy realisation to achieve decoupling and sustainability point of view, (1). Ao et al. examined the mediating role of innovation in the circular economy approach of energy-related enterprises in managing waste resources and reducing carbon emissions. They show that waste resource utilization positively affects the quality of new energy products, but it does not significantly impact the innovation of these products. On the other hand, carbon reduction behavior by companies in developing countries positively impacts new energy product innovation, but it does not significantly affect product quality. The study validates the existence of a relationship between waste resource utilization, new energy product innovation, and product quality. The findings can help energy-related enterprises improve their new energy product development activities and contribute to reducing waste and carbon emissions. (2). Xie et al. investigated the relationship between carbon emissions and economic growth in Fujian Province, China, based on evidence, as part of China’s “double carbon” target to reduce emissions while achieving economic growth. The study emphasizes the importance of the concept of ecological civilization in achieving the “double carbon goal” and the need for accelerating measures that guide the integration of urban and rural areas with critical infrastructure where energy is considered one critical commodity. (3). Han et al. examined the decoupling of China’s CO2 emissions and economic growth using the environmental Kuznets curve model for long-term decoupling and the Tapio model for short-term decoupling. They showed that the influence of industrial and energy structures inhibited CO2 emissions, but population structure may endorse them. The study recommends that China should strengthen and optimize its energy structure to match its industrial structure.
5 Conclusion
The Research Topic “Sustainable Planning and Life Cycle Thinking of Energy Infrastructure” explored various aspects of planning and implementing sustainable energy infrastructure. Based on the received response in this call, we covered a broad range of Research Topic related to energy infrastructure planning, including renewable energy systems, energy storage, grid integration, and policy impact and role of energy in decarbonization. Apart from planning, the Research Topic also focused on the need to adopt a life cycle thinking approach to energy infrastructure development; this approach involves considering the entire life cycle of energy systems, from production and distribution to end-of-life disposal. By taking a holistic view of energy infrastructure, it is possible to identify opportunities to reduce environmental impacts, improve resource efficiency, and enhance social and economic sustainability. However, the response towards life cycle thinking of energy infrastructure in this call was not too much. Also, from the approaches mentioned in Section 3, the point of view for sustainable planning and lifecycle thinking of energy infrastructure in the received response from various authors was only limited to fewer approaches.
To conclude, the articles included in the Research Topic provided insights into the strategies and technologies that can be employed to promote sustainable energy infrastructure. For instance, several articles explored the use of renewable energy sources, such as solar, geothermal, hydrogen, and wind power, to reduce greenhouse gas emissions and promote energy independence. Other articles discussed the importance of energy storage technologies, such as batteries and pumped hydro; mobility, such as hydrogen- and electric-based, and their integration into the grid. Few other articles focused on technology implementation and policy support in realizing economic growth and decarbonization. In addition, the Research Topic emphasized the importance of considering social and economic factors when planning energy infrastructure. For instance, several articles discussed the role of community engagement and participation in energy infrastructure decision-making. Others explored the potential for energy infrastructure to create new jobs and economic opportunities in local communities.
Overall, the Research Topic collected interesting articles and highlighted the need for a comprehensive, integrated approach like RePLiCATE (Figure 1B) to energy infrastructure planning and development following SDG7 (Figure 1A) and other applicable tools or techniques as per the changing global policies. We strongly believe that by considering technical, environmental, social, and economic factors under the RePLiCATE approach, it is possible to create sustainable energy systems that meet the needs of present and future generations.
Author contributions
All authors listed have made a substantial, direct, and intellectual contribution to the work and approved it for publication.
Acknowledgments
The Research Topic Editors would like to take the opportunity to thank the authors who responded to the call. We are also deeply indebted to the reviewers whose input was indispensable to select the published papers.
Conflict of interest
The authors declare that the research was conducted in the absence of any commercial or financial relationships that could be construed as a potential conflict of interest.
Publisher’s note
All claims expressed in this article are solely those of the authors and do not necessarily represent those of their affiliated organizations, or those of the publisher, the editors and the reviewers. Any product that may be evaluated in this article, or claim that may be made by its manufacturer, is not guaranteed or endorsed by the publisher.
References
Bridge, G., Özkaynak, B., and Turhan, E. (2018). Energy infrastructure and the fate of the nation: Introduction to special issue. Energy Res. Soc. Sci. 41, 1–11. doi:10.1016/j.erss.2018.04.029
Burk, C. (2018). “Techno-economic modeling for new technology development,” in Chemical engineering progress, 43–52.
Chand, A. A., Prasad, K. A., Mar, E., Dakai, S., Mamun, K. A., Islam, F. R., et al. (2021). Design and analysis of photovoltaic powered battery-operated computer vision-based multi-purpose smart farming robot. Agronomy 11 (3), 530. doi:10.3390/agronomy11030530
Cucchiella, F., D’Adamo, I., and Gastaldi, M. (2017). The economic feasibility of residential energy storage combined with PV panels: The role of subsidies in Italy. Energies 10, 1434. doi:10.3390/en10091434
D’Adamo, I., Ferella, F., Gastaldi, M., Ippolito, N. M., and Rosa, P. (2023). Circular solar: Evaluating the profitability of a photovoltaic panel recycling plant. Waste Manag. Res., 734242X221149327. 0734242X221149327. doi:10.1177/0734242X221149327
D’Adamo, I., Gastaldi, M., Imbriani, C., and Morone, P. (2021). Assessing regional performance for the sustainable development goals in Italy. Sci. Rep. 11, 24117. doi:10.1038/s41598-021-03635-8
D’Adamo, I., Gastaldi, M., and Morone, P. (2022). Solar collective self-consumption: Economic analysis of a policy mix. Ecol. Econ. 199, 107480. doi:10.1016/j.ecolecon.2022.107480
Dilimulati, A., Stathopoulos, T., and Paraschivoiu, M. (2018). Wind turbine designs for urban applications: A case study of shrouded diffuser casing for turbines. J. Wind Eng. Industrial Aerodynamics 175, 179–192. doi:10.1016/j.jweia.2018.01.003
Goldthau, A. (2014). Rethinking the governance of energy infrastructure: Scale, decentralization and polycentrism. Energy Res. Soc. Sci. 1, 134–140. doi:10.1016/j.erss.2014.02.009
Hauschild, M. Z., Rosenbaum, R. K., and Olsen, S. I. (2018). Life cycle assessment. Cham: Springer International Publishing. Available at: https://doi.org/10.1007/978-3-319-56475-3Book.
Kujala, J., Sachs, S., Leinonen, H., Heikkinen, A., and Laude, D. (2022). Stakeholder engagement: Past, present, and future. Bus. Soc. 61 (5), 1136–1196. doi:10.1177/00076503211066595
Kumar, N. M., Chakraborty, S., Yadav, S. K., Singh, J., and Chopra, S. S. (2022). Advancing simulation tools specific to floating solar photovoltaic systems–Comparative analysis of field-measured and simulated energy performance. Sustain. Energy Technol. Assessments 52, 102168. doi:10.1016/j.seta.2022.102168
Kumar, N. M., Chopra, S. S., Chand, A. A., Elavarasan, R. M., and Shafiullah, G. M. (2020). Hybrid renewable energy microgrid for a residential community: A techno-economic and environmental perspective in the context of the SDG7. Sustainability 12 (10), 3944. doi:10.3390/su12103944
Kumar, N. M., and Chopra, S. S. (2023). Integrated techno-economic and life cycle assessment of shared circular business model based blockchain-enabled dynamic grapevoltaic farm for major grape growing states in India. Renew. Energy 209, 365–381. doi:10.1016/j.renene.2023.03.064
Kumar, N. M., Islam, S., Podder, A. K., Selim, A., Bajaj, M., and Kamel, S. (2023). Lifecycle-based feasibility indicators for floating solar photovoltaic plants along with implementable energy enhancement strategies and framework-driven assessment approaches leading to advancements in the simulation tool. Front. Energy Res. 11, 1075384. doi:10.3389/fenrg.2023.1075384
Mamun, K. A., Islam, F. R., Haque, R., Chand, A. A., Prasad, K. A., Goundar, K. K., et al. (2022). Systematic modeling and analysis of on-board vehicle integrated novel hybrid renewable energy system with storage for electric vehicles. Sustainability 14 (5), 2538. doi:10.3390/su14052538
Molla, A. H., Shams, H., Harun, Z., Kasim, A. N. C., Nallapaneni, M. K., and Rahman, M. N. A. (2023). Evaluation of end-of-life vehicle recycling system in India in responding to the sustainability paradigm: An explorative study. Sci. Rep. 13 (1), 4169. doi:10.1038/s41598-023-30964-7
Nallapaneni, M. K. (2021). Leveraging blockchain and smart contract technology for sustainability and resilience of circular economy business models. City University of Hong Kong. Doctoral DissertationPhD-SEE-55632135.
Nallapaneni, M. K., and Chopra, S. S. (2021). “Blockchain-enabled dynamic grapevoltaic farms for selected wine risk regions on a global level and the potential opportunities for symbiotic industrial networks,” in 6th AIEE energy symposium current and future challenges to energy security: The energy transition, a pathway from (Milan, Italy.
Nallapaneni, M. K., Chopra, S. S., and D'Adamo, I. (2021). “Agrivoltaics for the co-production of soybeans-solar photovoltaics energy and the opportunities for developing synergetic industrial networks in India,” in 5th international conference on alternative fuels, energy and environment (ICAFEE 2021): Future and challenges: Future and challenges.
Nallapaneni, M. K., and Chopra, S. S. (2021). “Design and analysis of microgrids in line with SDG7 for residential communities in the global south,” in International symposium on sustainable systems and technology (ISSST 2021). ISSST-2021.
Nallapaneni, M. K. (2022). “RePLiCATE: A framework for sustainable and resilient planning of advanced clean energy systems,” in HKICE young scientist seminar series Hong Kong Institute for Clean Energy, City University of Hong Kong. HKICE_ YS20221125, 1.
Nallapaneni, M. K. (2022). “U.S.A.’s interstate highway modernization and financing issues: Could road-integrated wind and solar photovoltaics offer support?,” in Nallapaneni’s self archive, 0001. doi:10.13140/RG.2.2.30563.63523/3
Pandey, V. (2020). “Energy infrastructure for sustainable development,” in Affordable and clean energy, 1–13.
Poonia, S., Singh, A. K., Jain, D., Kumar, N. M., and Singh, D. (2022). Techno-Economic Analysis of Integrated Solar Photovoltaic Winnower-Cum Dryer for Drying Date Palm Fruit. Sustainability 14 (20), 13686. doi:10.3390/su142013686
UN SDG (2022). Goal 7- Ensure access to affordable, reliable, sustainable and modern energy for all. United Nations. Available at: https://sdgs.un.org/goals/goal7.
Vishnupriyan, J., Arumugam, D., Kumar, N. M., Chopra, S. S., and Partheeban, P. (2021). Multi-criteria decision analysis for optimal planning of desalination plant feasibility in different urban cities in India. J. Clean. Prod. 315, 128146. doi:10.1016/j.jclepro.2021.128146
Yuan, X., Kumar, N. M., Brigljević, B., Li, S., Deng, S., Byun, M., et al. (2022). Sustainability-inspired upcycling of waste polyethylene terephthalate plastic into porous carbon for CO2 capture. Green Chem. 24 (4), 1494–1504. doi:10.1039/d1gc03600a
Keywords: energy infrastructure, renewable energy, smart microgrids, circular economy, SDG7, clean energy policy, sustainable and resilient energy systems, integrated energy infrastructures
Citation: Kumar NM, D'Adamo I, Hait S, Priya A, Kichou S and Gastaldi M (2023) Editorial: Sustainable planning and lifecycle thinking of energy infrastructure. Front. Energy Res. 11:1196826. doi: 10.3389/fenrg.2023.1196826
Received: 30 March 2023; Accepted: 11 April 2023;
Published: 18 April 2023.
Edited and reviewed by:
Michael Carbajales-Dale, Clemson University, United StatesCopyright © 2023 Kumar, D'Adamo, Hait, Priya, Kichou and Gastaldi. This is an open-access article distributed under the terms of the Creative Commons Attribution License (CC BY). The use, distribution or reproduction in other forums is permitted, provided the original author(s) and the copyright owner(s) are credited and that the original publication in this journal is cited, in accordance with accepted academic practice. No use, distribution or reproduction is permitted which does not comply with these terms.
*Correspondence: Nallapaneni Manoj Kumar, bmFsbGFwYW5lbmljaG93QGdtYWlsLmNvbQ==, bWFubGxhcGFuMi1jQG15LmNpdHl1LmVkdS5oaw==