- 1Department of Mechanical Engineering, Covenant University, Ota, Nigeria
- 2School of Chemical and Metallurgical Engineering, University of the Witwatersrand, Johannesburg, South Africa
- 3Department of Mechanical Engineering, Trinity College of Engineering and Research, Pune, India
The study focused on a forensic assessment of bioenergy utilization in developing countries, emphasizing how to improve bioenergy resources for a sustainable economy and development. First, a holistic review of energy sources and their impacts on sustainable development was highlighted. The study also looked at the relationships between various energy sources, both the supply and demand of energy, as the population statistics in developing countries. However, renewable energy is yet to be harnessed fully as it lagged by 0.7%. Shockingly, the African continent is backward in both the global energy supply and in tapping renewable energy sources, as only 6% is contributed. More so, applying a clean development mechanism (CDM) for energy utilization and control shows that net emission reduction and global temperature reduction of 1.5°C by 2030 is attainable. Thus, helping to realize low-cost and sustainable energy (SDG 7) and sustainable cities and communities (SDG11). Further, the assessment of the level of bioenergy availability and its utilization shows that 10% of the world’s energy is provided by bioenergy. Interestingly, it is available in every region in the form of biomass. The study is helpful for stakeholders, policymakers, and governments managing the affairs of the energy sector on the need to formulate policy that will guide effective bioenergy utilization and reduction in greenhouse gas emissions, especially in developing countries thereby, promoting sustainable economic growth.
1 Introduction
The cornerstone of the contemporary industrial economy is energy. Energy is a fundamental component of nearly all human activities. It promotes transportation, education, health, food storage and production, mineral extraction, and industrial development, among other functions (Østergaard et al., 2020). Without providing a sizable section of its population with at least some access to energy services, no nation has been able to develop its economy far above a subsistence level. Modern energy services are a powerful force for social and economic growth. People’s daily lives are influenced by their financial status and available energy resources. However, it is paramount to acknowledge that consumers will like to know what other services that energy can give apart from fuel and electricity (Johansson et al., 2012; Østergaard et al., 2020).
Energy is necessary for development, and renewable energy systems are required for sustainable development (Kartha and Larson, 2000; Johansson et al., 2012; Østergaard et al., 2020). Significant 21st-century challenges, including climate change, economic and social growth, human wellbeing, and international security, are addressed mainly by energy (Johansson et al., 2012). Energy has been recognized as a crucial resource for facilitating any country’s social and economic operations. As a result, issues related to sustainable development, including reducing poverty, advancing women, protecting the environment, and creating jobs, are strongly correlated with a lack of energy services (Kartha and Larson, 2000). There are still billions of individuals without privilege to up-to-date, essential energy facilities that must be met and developed. Emerging economies must also participate in the global switch to clean, low-carbon energy systems. Past development rates toward greater adeptness, carbon reduction, greater fuel variety, and decrease in pollutant emissions need further acceleration (Dilip and Marika, 2009). Concern about advancing renewable energy has been greatly rekindled by growing worries about global warming and worldwide energy safety caused by emissions of toxic gases released when fossil fuels are burned. These worries have also sparked a concerted response to the global push for a clean development mechanism, a fundamental policy recognized under the Kyoto Protocol. Residents outside of cities with little connection to centralized grid networks’ electricity have a great deal to hope for, thanks to the utilization of biomass for sustainable, dispersed generating electricity (Mohammed et al., 2014). Many difficulties are associated with the current rise in the human population worldwide. In emerging nations, where almost 2 billion persons do not have privilege to a modern energy source, electricity and energy demands are rising as nations and regions work to improve their economies.
Additionally, to satiate the rising demand for necessities by urban and rural residents, worldwide climatic change and the fickleness of using petroleum products have sparked the quest for sustainable and ecologically acceptable alternative forms of energy (Oyedepo et al., 2019; Buraimoh et al., 2020). This has caused a substantial rise in the usage of bioenergy as a remedy that can integrate energy security with environmental conservation without inherently jeopardizing human nutrition (Shahare et al., 2017). The world’s energy demand is currently being significantly reduced thanks to bioenergy, and its place in the future synthesis of energy supplies is likely to grow significantly. Bioenergy can help address issues with energy access, open doors for social and economic growth in rural areas, support local energy security, enhance resource and waste management, lessen greenhouse gas emissions, and have other progressive effects on the surroundings (Johansson et al., 2012).
This study aims to present the recent research in bioenergy technology, which will serve as a blueprint for sustainability by employing a clean development mechanism.
1.1 Global energy supply and demand
Fossil fuels has been the primary supply of energy for years right from the emergence of the Industrial Revolution. The primary energy sources on the planet are coal, oil, and gas. Coal, oil, and natural gas have significantly increased their primary energy supply since 2000, while renewable energy sources have seen a 48% growth. The pattern has remained consistent in recent years (Wilkinson and Herrera, 2010; Lebre La Rovere et al., 2011). Between 2016 and 2017, fossil fuels market increases more than that of renewable energy. Renewable energy is trailing (0.7%), whereas coal, oil, and natural gas contributed to the 1.6% rise in the primary power source between 2016 and 2017 (Pousa et al., 2007; HLPE, 2014; IEA, 2016). Most emerging countries use solid biofuels, especially South Asia and sub-Saharan Africa. Liquid biofuels for transportation make up 0.5% of the world’s total primary energy supply and 4% of the renewable energy available worldwide. Even though they have risen the fastest since 1990 (about 15% yearly) compared to other biofuels, biogases still only make up 1.5% of the world’s renewable energy supply. Although the growth rate of solid biofuels was relatively modest (1% per year), that of liquid biofuels was equally spectacular (11% per year) (IEA, 2014). In 2010, the world’s two largest bioenergy producers were China and India, which produced 20% and 17% of them, respectively (Bennamoun, 2022). Compared to about 25% in India, bioenergy makes up less than 10% of China’s total primary energy supply (TPES). Nigeria and the United States’ third and fourth most significant bioenergy producers, respectively, had a bioenergy contribution to total primary energy supply (TPES) of above 80% and below 4%, in 2010. This exemplifies the distinction between industrialized and developing nations: in OECD nations, bioenergy often makes up a small portion of the total primary energy supply (TPES), whereas in developing non-OECD nations, it typically serves as the principal energy source.
To achieve a 20% share of renewable energy consumption by 2020, the European Union (EU) recently set legally obligatory goals. Due to the complicated environmental effects of biofuels and the competition for land between the production of bioenergy feedstocks, food, feed, and biomaterials, there is concurrently growing concern about a sustainable and dependable supply of biofuels. Contrarily, there is much room for improvement in the efficiency of bioenergy production, which currently ranges from 5% to 15% of power and heat production. Given the uncertainties mentioned earlier, projections of bioenergy’s contribution to the TPES differ substantially, from less than 100 EJ annually to more than 400 EJ in 2050. Contrary to current expectations, agricultural bioenergy resources are predicted to be the primary resource (IEA, 2014).
Using quantitative projections, the Matsuo study (Matsuo et al., 2013) examines the interaction between Asia and the Middle East to determine energy supply and demand in Asia and other parts of the world until 2035. The authors developed an economic model to predict significant rise in Asian demand for fossil fuels. To maintain stability in the global energy supply and demand, it will be essential to continue making wise investments in resource development in the Middle East. The Middle East is anticipated to concentrate more on its exports of fossil fuels to Asia while its shipments to North America and Europe are predicted to fall. According to Matsuo (Matsuo et al., 2013), there will be an increase in interdependence between the major energy-producing and consuming regions (Matsuo et al., 2013; Yang et al., 2019; Rather et al., 2022).
1.2 Net zero emission, 1.5°C, and world energy
The report of IPCC Sixth Assessment presented a challenge of achieving reducing global temperature increase by 1.5°C and also reducing greenhouse gas emissions to net zero. This goal seems impossible due to the world’s vulnerable teeming population that is ravaged by the impact of climate change induced by fire, flood, and drought. A timeframe goal of 2030 by the sustainable development agenda is to reduce emissions drastically and provide clean energy. Clean energy is the corrector to climate change impacts (Maltsoglou et al., 2013; IEA, 2014; IEA, 2016; IRENA, 2022).
More and more nations are putting these technical advancements at the core of their strategy for combating climate change, as well as for economic growth, energy security, and universal access. Although there is a compelling commercial case for renewable energy, progress is still being stymied by entrenched impediments that were put in place by systems and institutions designed for the fossil fuel age. Reducing CO2 emissions by about 37 gigatonnes (Gt) from 2022 levels and attaining net-zero emissions in the energy sector by 2050 are necessary to regulate global warming to 1.5°C. Figure 1 presents the progress of energy systems (Maltsoglou et al., 2013; IEA, 2014; IEA, 2016; IRENA, 2022).
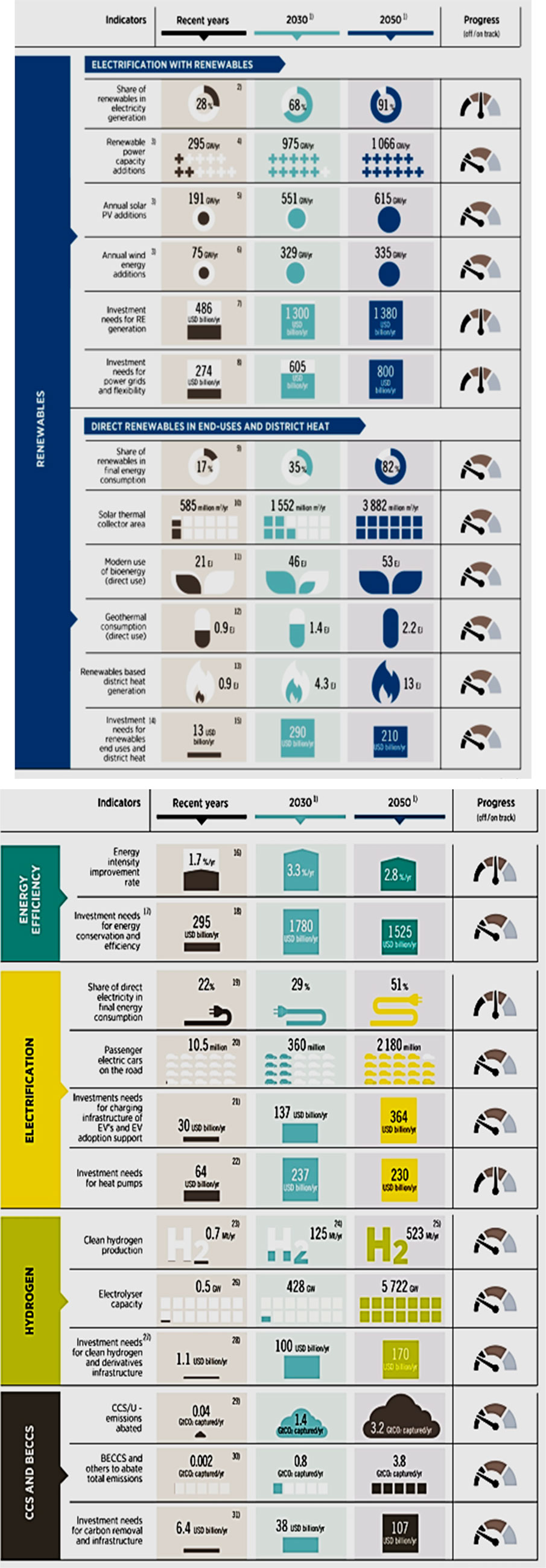
FIGURE 1. Cont’d: Monitoring the development of major energy system elements to reach the 1.5°C Scenario (Maltsoglou et al., 2013; IEA, 2014; IEA, 2016; IRENA, 2022).
To achieve the 1.5°C objective between 2023 and 2030, an average yearly expenditure is needed. All investment data from recent years is presented in USD as of today. Future investments are required in district heating, biofuels, and novel bio-based fuels. Future investments in energy efficiency and conservation will go toward energy recovery, chemical and mechanical recycling, and organic and bio-based plastics. Green hydrogen’s market share will increase from 40% in 2030 to 94% in 2050. Future expenditures are required in infrastructure, H2 stations, bunkering facilities, and electrolyzers. Hydrogen, other fuel supplies, power and heat, industry, and direct air capture of operational facilities are all included in the electrolyzer’s capacity to capture CO2 during the processing of natural gas (Maltsoglou et al., 2013; IEA, 2014; IEA, 2016; IRENA, 2022).
In the 1.5°C Scenario, power production will more than triple between 2020 and 2050, with renewable energy accounting for 91% of all electricity supply, up from 28% in 2020 as shown in Figure 2. Power generation based on coal and oil would see a steep fall over the following 10 years until being completely phased out by the middle of the century. Nuclear power plants would supply the remaining 4% of the world’s electricity requirements by 2050, with natural gas covering 5% of that total. The shift is characterized by a synergy between renewable energy sources that are becoming more accessible and the widespread use of electric technology for end-use applications, particularly in transportation and heating. The electrification of heat, transportation, and other end uses suggests that to achieve the 1.5°C target by the end of 2050, the world’s renewable power generation capacity will need to increase by a factor of approximately 12 (Maltsoglou et al., 2013; IEA, 2014; IEA, 2016; IRENA, 2022).
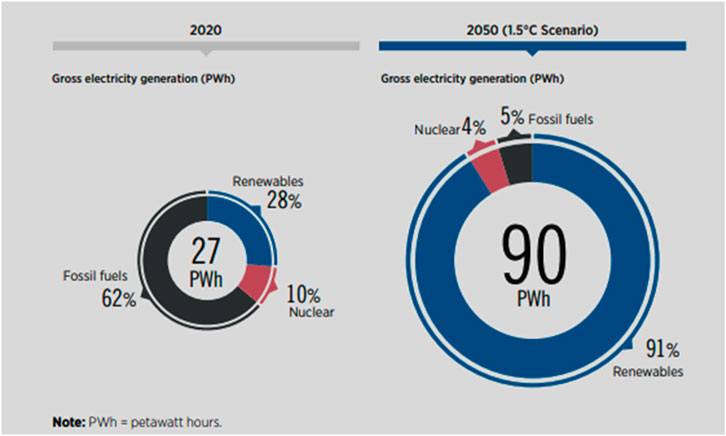
FIGURE 2. Trend of electricity generation from 2020 to 2050 in light of 1.5°C (IEA, 2014; IEA, 2016; IRENA, 2022).
By scaling up, the proportion of renewable energy in total final energy consumption (TFEC) would increase from 18% in 2020 to 82% by the year 2050. According to the 1.5°C Scenario, electricity will take over 50% of TFEC, making it the primary energy transporter as shown in Figure 3. The use of renewable energy, increases in energy efficiency, and electrification of end-use industries all contribute to this trend. By 2050, it is anticipated that modern biomass and hydrogen will contribute 16% and 14% of TFEC, respectively, to the global energy mix.
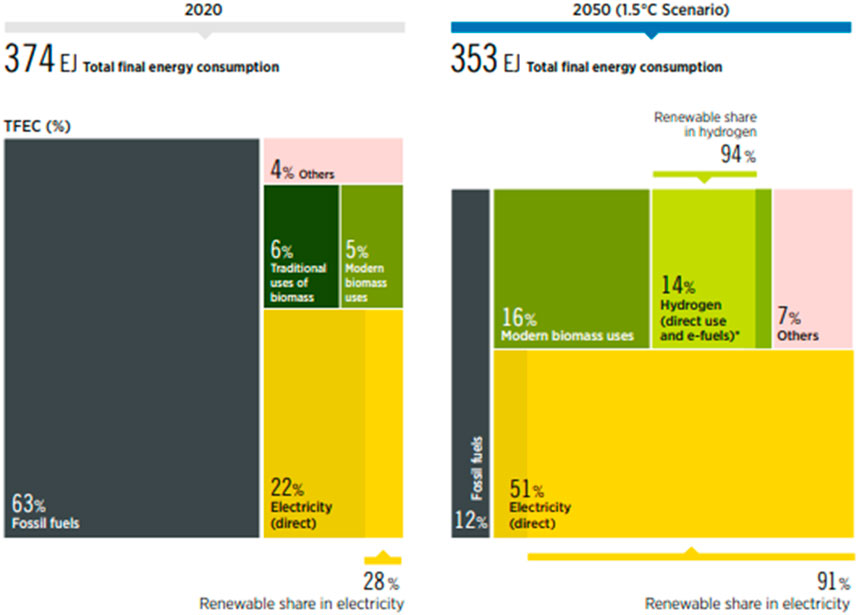
FIGURE 3. Total ultimate energy use between 2020 and 2050, broken down by energy carrier, under the 1.5°C Scenario (IEA, 2014; IEA, 2016; IRENA, 2022).
Especially noteworthy is the prediction that 94% of hydrogen production will originate from renewable sources, demonstrating a rising reliance on clean energy sources. The roadmap also indicates a potential trend toward decarbonization and a more sustainable energy future, with TFEC falling by 6% between 2020 and 2050 (IEA, 2014; IEA, 2016; IRENA, 2022).
Only energy consumption is depicted in Figure 3; non-energy uses are not included. In terms of energy usage, renewable sources account for 28% in 2020 91% in 2050 for electricity, 7% and 84% in 2050 for district heating, and 94% in 2050 for hydrogen (direct use and e-fuels) from renewable sources (IEA, 2014; IEA, 2016; IRENA, 2022).
Total hydrogen consumption is accounted for by hydrogen (direct usage and e-fuels). All forms of direct power generation, including nuclear, fossil fuel-based, and renewable energy sources, are included. The residential TFEC of solid biofuels in non-OECD nations is referred to as one of the traditional uses of biomass. Modern bioenergy applications include the use of liquid biofuels mostly in transportation but also in buildings, industry, and other end consumption, as well as solid biomass, biogas, and biomethane used in industry and buildings. Natural gas (used mostly in industry and transportation, and to a lesser extent in buildings), oil (used primarily in industry and transportation, and to a lesser extent in buildings), and coal (used in industry for making cement, chemicals, iron, and steel) will all still be considered fossil fuels in 2050 (IEA, 2014; IEA, 2016; IRENA, 2022). District heating and the use of alternative renewable energy sources are some others. Organization for Economic Co-operation and Development, exajoule, and total final energy consumption (TFEC) all stand for energy (IEA, 2014; IEA, 2016; IRENA, 2022).
Due to improved energy efficiency and the expansion of renewable energy sources, the global primary energy supply stays steady. By 2050, renewable energy will account for 77% of the primary energy supply, up from 16% in 2020. A combination of end-use electrification, renewable fuels, and direct applications would substantially alter the energy balance, increasing the share of renewable energy in the total primary energy supply by a net 61 percentage points. For the global climate targets to be met, this level of renewable energy penetration must be attained, which would require major financial and legislative assistance as well as ongoing innovation (Maltsoglou et al., 2013). The implementation of a variety of policy and technology measures, including many already-existing solutions, can help meet the 1.5°C climate objective (IEA, 2014; IEA, 2016; IRENA, 2022).
1.3 Energy consumption pattern in developing countries
A sufficient energy supply is a requirement for the growth of the economy, culture, and society in complex civilizations (Junginger et al., 2011). However, a lack of energy will impact all social and economic progress facets. In contrast, energy use is believed to be the primary underlying factor contributing to greenhouse gas emissions and climate change. The World Resources Institute estimates that the energy sector is responsible for 61.4% of all greenhouse gas emissions. According to Energy Information Agency predictions, population expansion and economic development will cause a significant rise in energy demand in the upcoming years (Suzuki, 2015). Most of this rise will occur in poorer countries, where from 2004 to 2040, energy consumption will rise from 46% to 58% of total consumption. Energy demand is anticipated to increase by 0.9% annually in developed nations, compared to 3% annually for emerging nations (Bakhtiar et al., 2020). Oil, coal, and gas, in particular, are responsible for a large portion of the increase in worldwide energy use (Ravindranath and Rao, 2011). Around 9.25 GTOE of fossil and renewable energy are now consumed globally, 3.8 of them in developing nations. It is anticipated that by 2050, global consumption will at least double and most likely quadruple. Energy consumption will rise faster in developing nations than in industrialized nations (Bößner et al., 2019). According to the World Energy Council, nearly one-third of the total primary energy consumed by developing nations will come from renewable sources, increasing from 1.09 to 3.23 GTOE (Gielen et al., 2019).
Energy consumption has increased along with economic expansion in developing nations (Chirambo, 2018; Ebhota and Jen, 2020). The energy demand of emerging countries nearly doubled between 2000 and 2015, from roughly 3,750 million tonnes (metric) to 7,000 million tonnes (metric) of oil equivalent (Oyedepo, 2012). Their GDP has increased by a factor of four since 2000. Demand in wealthy countries, however, remained unchanged during that time (Kumar and Samadder, 2017). Nearly six billion people in the developing world already consume most of the world’s energy, and over the next 15 years, their demand is anticipated to rise by another 30%. The consumer class in emerging countries is increasing and becoming wealthier, driving up energy consumption as they buy more goods and services that raise living standards (such as housing, transportation, healthcare, and education) and are driven in huge part by quickly growing economies (Balzani and Armaroli, 2010).
Developing countries must overcome challenges to secure a sufficient energy supply to meet their rising demand. Developing nations must amass tremendous amounts of new energy resources to meet this need. They will have to decide which fuels to provide, how much energy will be produced domestically and imported, and what kind of infrastructure will be used for energy production and distribution, among other strategic decisions. These decisions will be greatly influenced by various exogenous and endogenous variables, the cost, the accessibility of funds, and the inclinations for particular policies (such as those that address environmental consequences) (Chavez et al., 2020).
Many people also use energy-intensive forms of consumption, such as owning cars. After China, India is expected to surpass the United States as the world’s second-largest market for passenger cars. From 42 million units sold in 2017 to 58 million in 2024, light vehicle sales are anticipated to grow from 42 million units sold in 2017 to more than half of global sales in that year. This growth is expected in South America and Asia’s developing nations. As they try to satisfy these customers’ needs for goods and services, neighbourhood companies and enterprises are using more and more energy. Additionally, governments and the public sector typically use a lot of energy to provide various social, commercial, and administrative services (including security, utility-based power, healthcare, and education) (Nejat et al., 2015; Bharathiraja et al., 2018).
This demand’s energy mix will affect various nations in several different ways. The burning of indigenous coal versus imported natural gas for heating and the environmental issues associated with diesel use as opposed to electric vehicles are just a few examples of how different fuels have various properties. The sort of fuel demand will also depend on the source of the use. For instance, whereas electricity, which can be generated using renewable or fossil fuels, powers air conditioning services (which are anticipated to grow significantly throughout rising nations), heat for industrial processes has typically necessitated the burning of fossil energies (Petinrin and Shaaban, 2015).
Africa has myriads of challenges such as pollution, inadequate funding for clean energy, negative effect of COVID-19 and the Ukraine-Russia war affecting energy price and supply. Despite all of these challenges, the move to renewable energy globally offers new hope for the economic and social advancement of Africa. As of May 2022, nations that contributed negatively to over 70% emission of CO2 promised to reduce the emissions to a barest zero by 2050. 12 nations in African that were major contributors by 40% showed commitment to reducing CO2 emissions. With the cost of clean technologies falling and global investment changing, these goals are assisting in reorienting the global energy system (IRENA, 2022).
Africa needs affordable energy now more than anything else. The Sustainable Africa Scenario (Sovacool and Monyei, 2021; IRENA, 2022) estimates that it will take 90 million people per year, or three times as many as in recent years, to be connected to attain universal access to reasonably priced power by 2030. Nearly 600 million people globally that amount to 40% do not have access to electrical energy, mainly the Sub-Saharan Africa. It is worth emulating that Ghana, Rwanda, and Kenya are on track to attain full access to energy by 2030. Study reveals that for over 45% of those, expanding national grids is the most cost-effective and wisest solution (Sovacool and Monyei, 2021; IRENA, 2022).
Over the 2020–30 decade, the supply of contemporary primary energy will increase by 30 percent, whereas traditional cooking with bioenergy will be eliminated due to widespread access to modern fuels and technologies. Even so, the average worldwide energy consumption per person is still less than one-third (Sovacool and Monyei, 2021; IRENA, 2022). SAS plan to reduce resident energy demand by 2030 in sub-Saharan Africa by ensuring that everyone has access to cleaner, more efficient energy sources for cooking, such as electricity, natural gas, and oil products. This is because improvements in efficiency more than offset rising underlying demand for cooking as presented in Figure 4. More than offsetting the 180%, 0.7 EJ, rise in energy demand for other household uses (appliances, cooling, and lighting), the energy demand for cooking decreases by 66%, or 7.5 exajoules (EJ). Cooking energy supply are reduced by a factor of two when moving to upgraded biomass cookstoves, and by a factor of half to over 90% when transiting to the modern solid biomass stoves-type, biogas-type, bioliquids-type, and LPG, which depends on the original cooking-ware used and the current remedy implemented. The most effective cooking appliances are electric stoves, which are becoming more and more significant in the mix of cooking energy (Sovacool and Monyei, 2021; IRENA, 2022). From Figure 5, As nearly one billion people move from traditional use of biomass to more efficient cooking fuels by 2030, traditional usage of bioenergy has the greatest fall in use in Africa. In South Africa, as the use of coal as a fuel for family cooking is phased out and the use of natural gas in the industry increases, demand for coal is falling in end-use industries. The highest gains in demand for electricity, oil products, and modern bioenergy are expected between now and 2030, but these are more than compensated by a decline in the use of solid biomass in conventional methods (Sovacool and Monyei, 2021; IRENA, 2022).
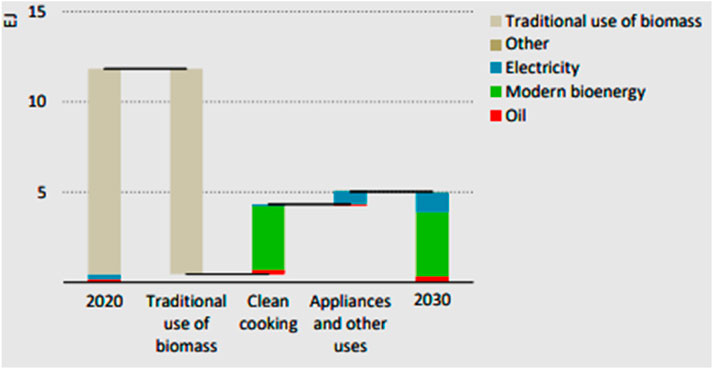
FIGURE 4. Residential energy demand fluctuation by fuel in sub-Saharan Africa in the SAS, 2020–2030 (Sovacool and Monyei, 2021; IRENA, 2022).
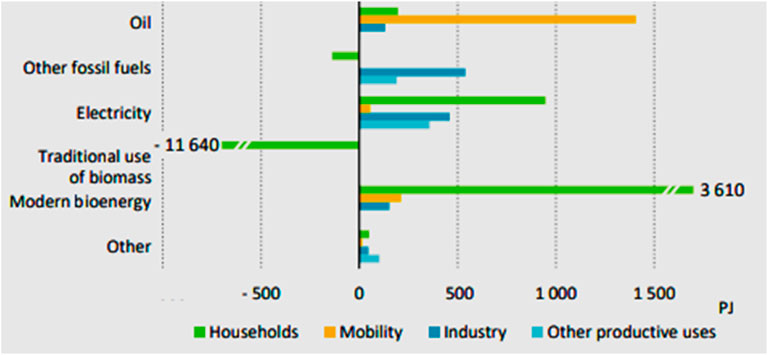
FIGURE 5. Changes in fuel and sector-specific final energy usage in the SAS from 2020 to 2030 (Sovacool and Monyei, 2021; IRENA, 2022).
2 Overview of biomass as a sustainable energy source
The utilization of biological products (also known as biomass) specifically for energy purposes is referred to as “bioenergy.” Energy utilization includes the generation of heat and power from biomass and the secondary processing of biomass into biofuels for the transportation industry. The energy present in biofuels is regarded as primary energy. The same is valid for charcoal, biogas, pellets, etc. The term “bioenergy” refers to all energy produced by biofuels, substances with a biological origin known as biomass (Li and Hu, 2017). The most significant global source of renewable energy was presented by Haberl (IEA, 2012). Using Figures 6, 7, the primary energy supply from biomass as a whole fell by 0.9 EJ in 2017 to reach 55.6 EJ, practically matching the previous year’s level. The biomass supply decreased for the first time since 2010 during this time. Despite reducing the supply, biomass continues to play a noteworthy part in providing energy from sustainable sources, concerning 70% of the overall renewable stream. The biomass contribution has been steadily declining since 2000. It is vital to remember that historically, biomass used for cooking and heating in underdeveloped countries accounts for the majority of the worldwide supply of biomass. Present-day bioenergy sources will perform a noteworthy measure in the subsequent mix of sustainable energy sources as their utilization as wood pellets, biogas, and liquid biofuels increases.
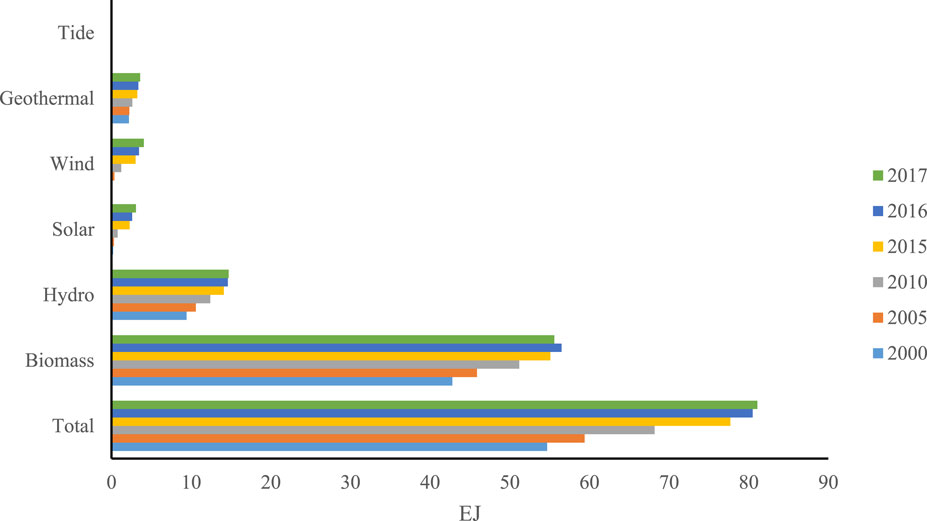
FIGURE 6. Total primary energy supply of renewables (IEA, 2012; Tesfamichael et al., 2021).
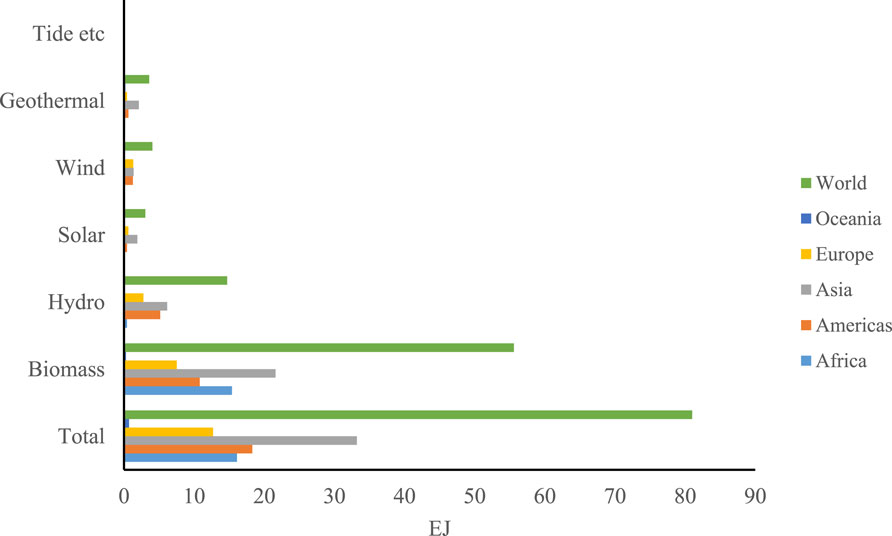
FIGURE 7. Total primary energy supply of renewables in continents in 2017 (IEA, 2012; Tesfamichael et al., 2021).
Because biomass accounts for 96% of all sustainable energy sources in Africa, its significance in some regions’ energy mix is self-evident. It also has much higher shares in Europe (59%) and the Americas (59%), as well as Asia (65%). The accessibility of a range of feedstock (agricultural, forestry) to create useable final products (biodiesel, bioethanol, biogas, wood chips, and pellets) aimed at power, heat, and transport usage promotes the growth of the bioenergy industry globally.
2.1 Feedstock for bioenergy
Local wood resources are used to produce the majority of bioenergy. According to FAO statistics, four billion plus hectares (ha), or about 30% of the globe’s land surface, are covered in forests (Tesfamichael et al., 2021). The United States (305 million ha), Canada (311 million ha), the Brazilian Republic (521 million hectares), Russia (810 million ha), and China are the five forest-rich countries with the most significant overall land area (207 million ha). India generated the most wood fuel in 2011, followed by China, Brazil, Ethiopia, and Nigeria.
Using agricultural leftovers as a source of bioenergy is one of the most potential areas for expansion. The sector’s share of global bioenergy output is less than 3%. According to data, the globe could produce between 4.2 billion tonnes (a low estimation) and 9.3 billion tonnes (a high estimation) of energy yearly by using the residues from all main crops. Using standard energy conversion, the speculative energy potential from leftovers can range from 17.7 to 82.4 EJ. Cereals, mainly maize, rice, and wheat, would make up most of the contribution. About 3%–14% of the globe’s energy necessities might be met by energy production from agricultural wastes (Shahid et al., 2021).
Globally, the forestry industry produces the most bioenergy sources. More than 85% of the biomass utilized for energy generation comes from forestry products such as wood chips, charcoal, fuelwood, and pellets. One of the primary forest products used in bioenergy production is wood fuel. In underdeveloped nations in Asia and Africa, wood fuel is primarily utilized for conventional cooking and heating (FAOStat, 2019). The world’s energy consumption of wood fuel was estimated to be 1.9 billion m3. (World Bioenergy Association, 2018). Urban and industrial waste used for energy, primarily in metropolitan areas, is the third and last category for bioenergy provision. Domestic waste to bioenergy supply in 2017 was 2.51 EJ, of which 58% was municipal trash, and the residual was energy from industrial waste (Pellegrini and Fernández, 2018).
2.2 Biomass supply
The most significant renewable energy source, making up more than two-thirds of the source of green energy, is bioenergy. Organic material, sometimes referred to as biomass, which contains carbon that plants consume during photosynthesis, is used to create bioenergy. When this biomass is burned to provide energy, the carbon is freed during combustion and is released back into the atmosphere. Modern bioenergy is an almost emission-free fuel because an equal quantity of carbon is absorbed as more biomass is produced. Providing about 6% of the world’s energy supply and 55% of all renewable energy, it is the greatest source of renewable energy in the world (Chong et al., 2021). Waste from the public and private sectors and primary solid, biogas, and liquid biofuels are specific categories for biomass sources (Wu et al., 2021).
From Figure 8, 355.6 EJ of biomass was utilized as fuel in 2017, with 86% of the consumption going to main solid biofuels such as wood chips, wood pellets, and fuelwood for heating and cooking. 7% of the biomass was converted into liquid biofuels. Nearly equal shares of biogas, municipal garbage, and industrial waste were between 2% and 3% (Statistics and WBA Global Bioenergy, 2020).
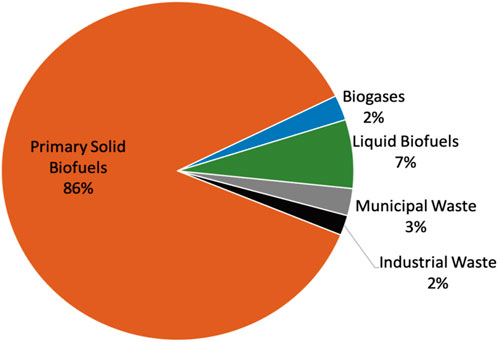
FIGURE 8. Domestic biomass supplies in 2017 (Statistics and WBA Global Bioenergy, 2020).
Figure 9 shows that Asia, Africa, and the Americas have the most extensive domestic biomass supply of all the continents. Asia and Africa make up a significant amount of the world’s biomass due to the widespread use of fuelwood and charcoal for heating and cooking (Olabi et al., 2022). The Americas (the United States and Brazil) produced a substantial number of liquid biofuels due to the manufacture of bioethanol from corn and sugarcane, respectively. More than 70% of the biofuels produced worldwide are produced in this region collectively. Europe is a leader in the sector, providing more than 50% of the biogas used worldwide. Due to the substantial use of garbage to produce bioenergy, with two-thirds of the world’s supply, Europe also has the highest supply of municipal waste.
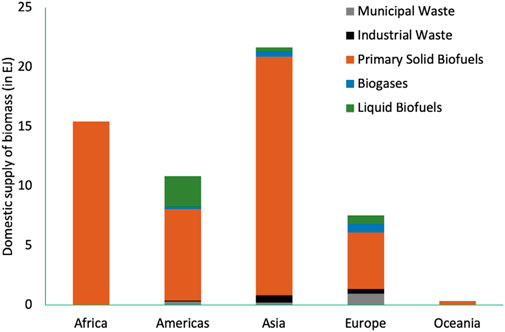
FIGURE 9. Domestic supply of biomass (Olabi et al., 2022).
In Africa, biomass energy accounts for over 30% of all energy consumption, with over 80% occurring in the Sub-Saharan region.
Renewable energy is progressively spreading over the continent with an annual growth rate of 21% between 2010 and 2020, a total capacity of more than 58 GW (of which hydropower contributes 63%), and a total dimension of 12% of the total energy consumption. Hydropower is the main source of renewable electricity in Africa, with more than 37 GW of installed capacity. Africa’s overall capacity for renewable energy as of 2022 was close to 59 GW. In compared to the prior year, this represented an increase of over 5%, maintaining the rising trend (Olabi et al., 2022). Globally speaking, less than 2% of the total capacity was made up of renewable energy in Africa.
2.3 Benefits of bioenergy
The fact that bioenergy is accessible everywhere is one of its main advantages. Municipal waste is found in every city, whereas biomass—which includes forestry products, crops, and leftovers—is found in almost every nation worldwide. Utilizing locally accessible resources to meet heating needs (such as hot water, space heating, etc.) offers substitutes for imported crude oil. A large portion of the biomass is produced globally in Europe and utilized for power plant heating. Considering the prevalence of district heating systems and the governmental emphasis on converting to renewable forms of heating, the majority of biomass used for sustainable heating originates in Europe, at 87%. A small amount of renewable heating from biomass-based sources is also used throughout Asia and the Americas. Europe has a high solar and geothermal energy concentration among other sustainable heating sources (Elavarasan et al., 2020; Simionescu et al., 2020; Chabhadiya et al., 2021).
2.3.1 Bioenergy contribution to world total energy supply
Around 10% of the world’s principal energy sources are bioenergy (47.2 EJ of bioenergy out of 479 EJ in total in 2005, or 9.85 percent) (Martins et al., 2018). Most of these were used in the residential market (heating and cooking). Bioenergy accounted for 78% of all renewable energy generated in 2005. Solid biomass makes up about 97 percent of all biofuels, with the residential sector using 71 percent of them (Abas et al., 2017; Narayan and Doytch, 2017; Reuter et al., 2017).
The gross final energy consumption in 2017 increased by 2% over the previous year to 370 EJ. Oil and petroleum products account for 40% of all energy utilized globally, while coal and gas each make up 20%. In 2017, the everyday use of crude oil made up 80 percent of the energy used worldwide. The proportion of renewable energy in the world’s gross final energy consumption fell to 17.7% in 2017 from 18.0% in 2016 (Abas et al., 2017; Narayan and Doytch, 2017; Reuter et al., 2017). Bioenergy, or energy generated from bio-based sources, is the most widely used type of renewable energy. Seventy percent of the renewable energy use in 2017 was bioenergy. The utilization of traditional biomass sources has been declining, which has contributed to a few percentage points (about 0.5%–1%) annual declines in the input of bioenergy. Most energy is consumed as heat, which is needed for industrial activities and space heating in homes and businesses. Biomass, which accounts for 96% of the market for renewable heat globally, is one of the most often used renewable energy sources for generating heating. One of the finest fossil fuel substitutes is fuels made from biomass in the transportation sector (bioethanol, biodiesel, etc.). In 2017, biofuels contributed 3.5 EJ to the transportation industry or around 3% of the total.
Direct heating’s end-use segment is dominated by biomass. About 94% of the renewable resources in these regions in 2017 came from the 40 EJ of biomass. The energy was consumed for heating and cooking reasons in end-use sectors such as household, business, agricultural, etc. 55.5 EJ of biomass was used for energy in 2017, with fuelwood, wood chips, and wood pellets accounting for 85% of the total consumption. Liquid biofuels were produced from 7% of the biomass. Nearly equal shares of biogas, municipal garbage, and industrial waste were between 2% and 3%. Solid fuels account for over 85% of biomass energy consumption, often inefficiently, for lighting, heating, and cooking. In emergent nations, where biomass accounts for up to 95% of total national energy consumption, traditional bioenergy (fuelwood and charcoal, which can only produce heat) predominates. Modern biomass is a low-carbon, distributed, renewable element of the national energy matrix that is becoming more and more significant to nations. In OECD nations, modern bioenergy use is expanding. Particularly co-firing biomass materials in coal-fired boilers have grown during the past several years, and some gasification technologies are almost ready for market (Abas et al., 2017; Narayan and Doytch, 2017; Reuter et al., 2017).
2.3.2 Potentials of bioenergy production
Due to the abundance of land on the continent, its choice due to the expensive and limited fossil fuel supply, the large variety of energy crops that may be cultivated there, and the resource’s inherent greenness, biomass has a great potential in Africa (IEA, 2019).
The use of agricultural leftovers as a source of bioenergy is one of the most potential areas for expansion as shown in Table 1. Currently, the sector’s share of global bioenergy output is less than 3%. Agriculture leftovers, such as straw and husk, can, nevertheless, make up a significant portion of the production of bioenergy due to the growing demand to replace fossil fuels in power plants that generate heat and electricity with sustainable, renewable, and dispatchable energy sources. Agriculture residues not only replace fossil fuels and cut emissions, but they also address the environmental problem brought on by the annual burning of harvest remains in developed nations like China and India. Considering that 50% of the residues must be left on the field for soil quality reasons, there is a tremendous opportunity for using agricultural residues. Figures 10–12 show that the world could generate between 4.3 billion tonnes (the low estimate) and 9.4 billion tonnes (the high estimate) of energy each year by using the leftovers from all significant crops, according to data. Using conservative moisture content and fuel energy contents as well as standard energy conversion coefficients for residues, the theoretical energy potential can range from 17.8 EJ to 82.3 EJ. From Table 1, cereals, mainly maize, rice, and wheat, would make up most of the contribution (Wu et al., 2021). In contrast, the domestic biomass energy supply in 2017 was roughly 55.6 EJ. About 585 EJ worth of energy was available from all energy sources combined, including fossil fuels. In other words, energy production from agricultural waste might offer 3%–14% of the world’s energy needs. It is significant to emphasize that the sustainable potential would be far lower than the theoretical potential when considering economic, social, and environmental concerns. Agriculture leftovers have a considerable potential to be a part of the future energy mix, even considering all sustainable variables (Statistics and WBA Global Bioenergy, 2020; Olabi et al., 2022).
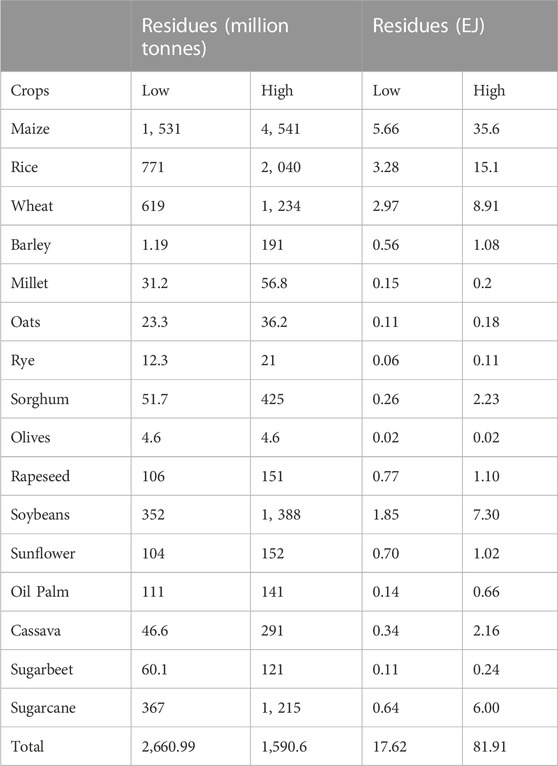
TABLE 1. Theoretical potential of agricultural residues globally (Pellegrini and Fernández, 2018; World Bioenergy Association, 2018; Statistics and WBA Global Bioenergy, 2020).
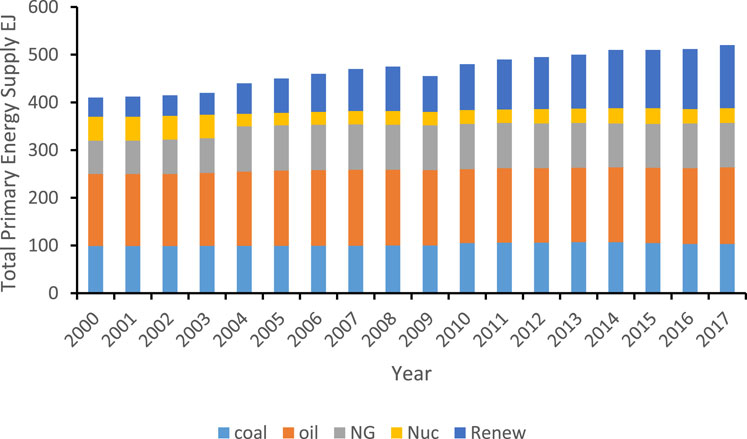
FIGURE 10. Total primary energy supply globally (Statistics and WBA Global Bioenergy, 2020; Olabi et al., 2022).
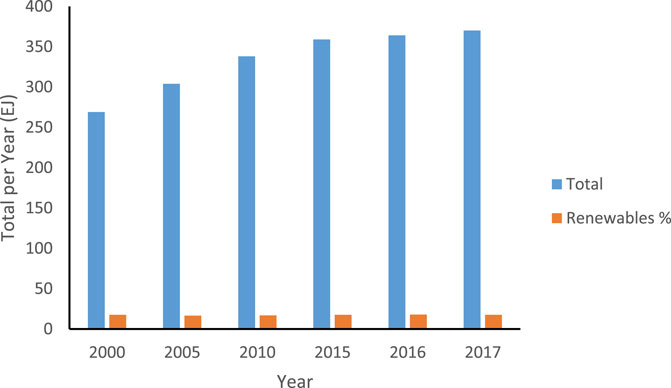
FIGURE 11. Percentage of global renewable energy (Statistics and WBA Global Bioenergy, 2020; Olabi et al., 2022).
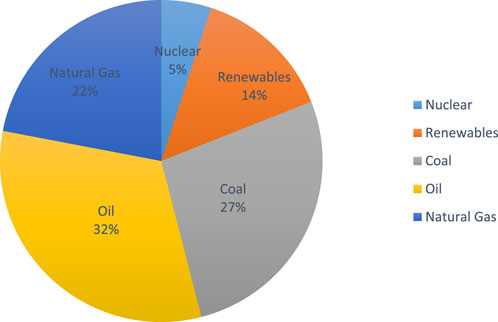
FIGURE 12. Total primary energy supply globally (Statistics and WBA Global Bioenergy, 2020; Olabi et al., 2022).
From Figure 13 it can be seen that Asia had the most considerable primary energy supply of fossil fuels (284 EJ) in 2017, accounting for 50% of the world’s total supply. The continent of Africa only comprised 6% of the total, with 47% going to Europe and North and South America. Because so much biomass is used for cooking and heating, 47% of the energy used on the African continent comes from non-fossil sources (EIA, 2007; Arto et al., 2016; Keho, 2016).
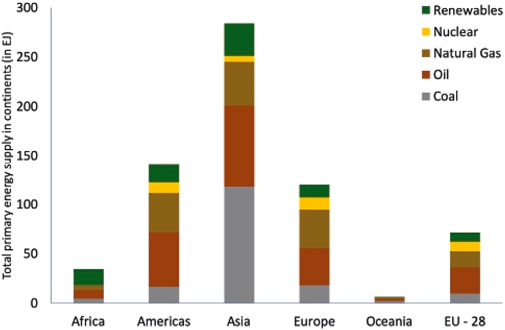
FIGURE 13. Primary energy sources’ overall output in 2017 by continents (EIA, 2007; Arto et al., 2016; Keho, 2016).
From Figure 14, energy sources used 370 EJ of gross final energy in 2017, a 2% increase over 2016. Oil and petroleum products account for 40% of all energy utilized globally, while coal and gas each make up 20%. The world’s energy consumption in 2017 (EIA, 2007; Arto et al., 2016; Keho, 2016) was 80% fueled by fossil fuels. In 2017, renewable energy accounted for 17.7% of the world’s total final energy consumption, a 0.2% decline from 2016 (Grübler et al., 1996; Panos et al., 2015).
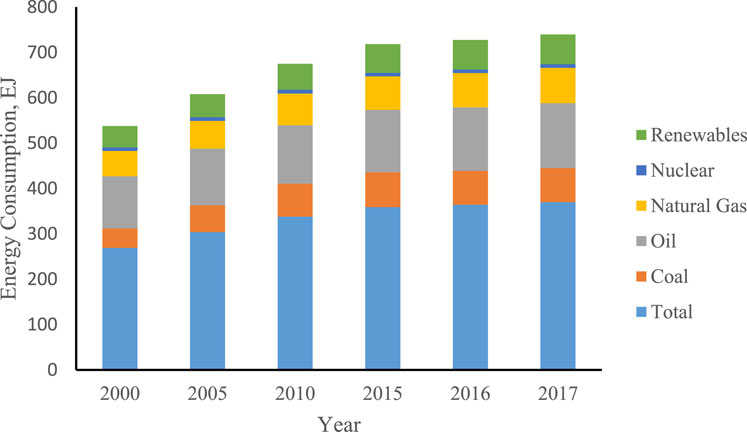
FIGURE 14. Gross final energy consumption globally (World Energy Council, 2016; Mahmoodi, 2017; Lobanova et al., 2018).
Global energy demand from Figure 15, has shown promise to be met by renewable energy sources, and some technologies’ exponential growth is encouraging. Since 2000, the share of solar energy has increased by 11 times, while the share of wind energy has increased by 35. To considerably reduce the use of fossil fuels, it is imperative to maintain the rapid development of renewable energy technology while focusing on reducing our global energy consumption through energy efficiency measures (World Energy Council, 2016; Mahmoodi, 2017; Lobanova et al., 2018).
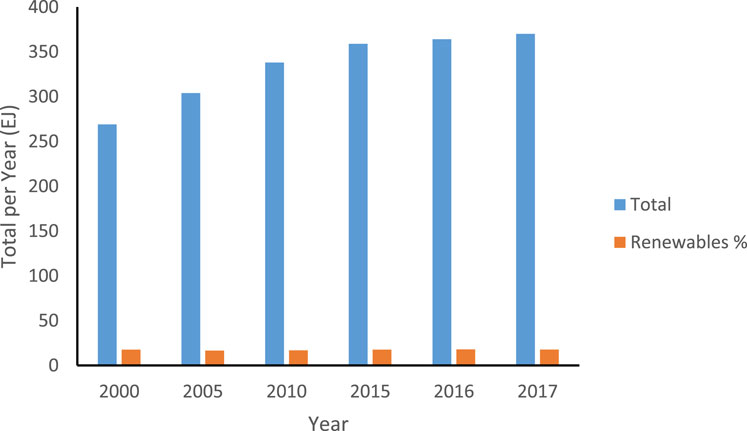
FIGURE 15. Gross final energy consumption globally (World Energy Council, 2016; Mahmoodi, 2017; Lobanova et al., 2018).
The most accessible renewable energy is bioenergy or energy produced from biological sources. Bioenergy accounted for 70% of the total renewable energy usage in 2017. As shown in Figure 16 by the fact that the contribution of bioenergy has been falling by a few percentage points (about 0.5%–1%) annually, it is likely that less traditional biomass sources are being used (Ardakani and Ardehali, 2014; Shyu, 2014; Cao et al., 2016; Ou et al., 2019).
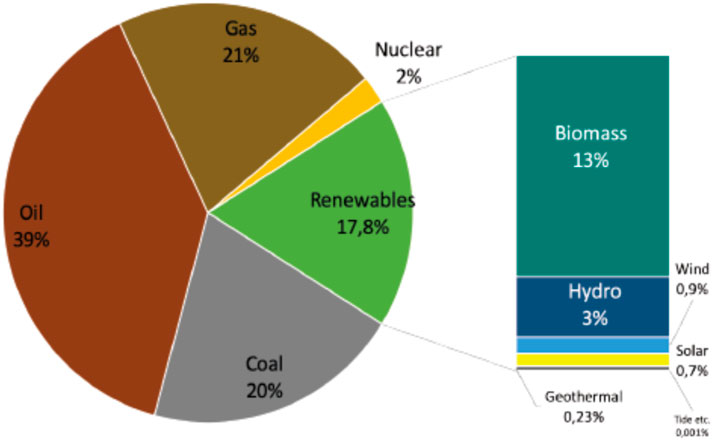
FIGURE 16. Gross final energy consumption in 2017 (Ou et al., 2019; He et al., 2020).
As observed in Figure 17, Asia is one of the continents with the highest fossil fuel consumption. The area uses over 80% of the coal produced worldwide. The region consumes 40% of global oil and oil-related product production, followed by North and South Americas at 30% and Europe at 21%. Europe, the Americas, and Asia each utilize around one-third of the world’s natural gas, according to the global distribution of consumption. Asia is the world leader in all energy sources, even renewable ones. The continent’s most enormous renewable energy consumption, including biomass, hydropower, wind, solar, geothermal, tide, etc (Ou et al., 2019; He et al., 2020).
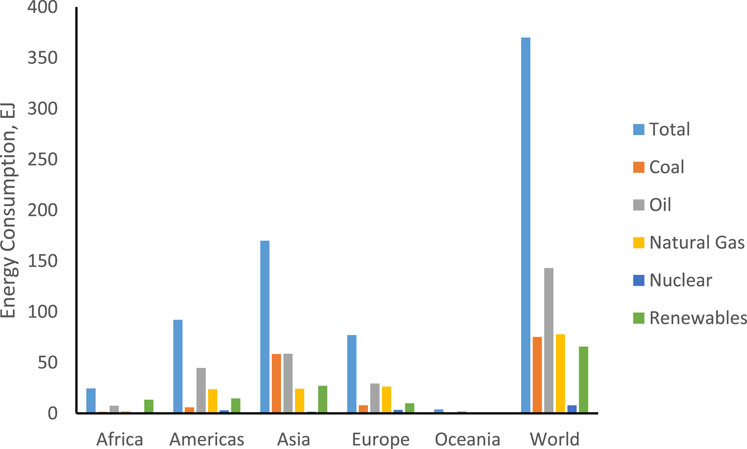
FIGURE 17. Gross final energy consumption in continents in 2017 (Ou et al., 2019; He et al., 2020).
2.3.3 Drivers behind development and promoting bioenergy utilization
The global energy system must shift to a low-carbon one to minimize the greenhouse gases we emit globally by exploring renewable technologies and nuclear energy, excluding carbon capture and storage (CCS) technology. Both options offer exceptionally low CO2 emissions per unit of energy compared to fossil fuels. A significant increase in interest in bioenergy has been observed during the past five to 10 years, coupled with the progressive advancement of increasingly advanced and effective bioenergy production methods. Several factors have contributed to this rebirth, including rising oil prices, unrest in oil-producing areas, shifting financial investments toward commodities and oil in 2007–2008, extreme weather events, and growing energy demand from developing countries. Domestic agricultural support programs, the need for energy self-sufficiency, the reduction of climate change, and the notion that biofuels are less expensive than fossil fuels are further factors driving the production of biofuels (World Energy Council, 2007; Yang and Bandivadekar, 2017; Benoit, 2019).
Interest in bioenergy is primarily being sparked by four factors: growing fossil fuel prices, particularly those of oil; energy infrastructure protection; global warming; and countryside growth. Since the production of biofuels in most nations is not cost-competitive with fossil fuels, bioenergy markets are primarily dependent on policy in much of the world. According to nearly all nations, energy security and climate change are the two most significant motivations for national bioenergy development initiatives (UNDP, 1997; Ailleret, 2000; Schiffer, 2008).
Most of the time, the G8 and +5 countries’ policy objectives are comparable. The +5 Countries’ emphasis on the development of bioenergy places a greater emphasis on rural development, which frequently aligns with reducing poverty (UNDP, 1997; Ailleret, 2000; Schiffer, 2008).
2.3.4 Assessment of bioenergy for sustainable development
Bioenergy development offers both potential and challenges for the environment and the economy. Using wood as a source of energy generation will likely have a considerable influence on the forest industry directly and indirectly due to land use. The potential for increased access to improved types of bioenergy by the poor, the effects on energy and food prices, and the opportunities for agricultural development, including the creation of income and employment, will all play a role in how much of an impact bioenergy has on reducing poverty in developing countries (Food and Agricultural Organization FAO, 2004; Haberl et al., 2013).
Bioenergy can enhance the entrance to better forms of energy for the poor and generate chances for income and employment. However, there are still a lot of questions about how it will affect the environment, agriculture, food security, and sustainable forest management. Also, how it will affect people, especially the poor in developing nations, who the changes will impact it will bring about to land use, land tenure, and land rights (FAO, 2013; Gupta and Verma, 2015).
2.4 Bioethanol production and alternative biofuel
Since its potential has not yet been completely realized, biomass fuel is considered a fuel for the future. Over 76% of the population in developing nations, mostly in Africa, depends on bioenergy such as ethanol-based types as their primary source of fuel. This fuel is mostly used in rural areas where most households rely on fuelwood, wood waste like sawdust, and charcoal for everyday cooking (Ludwig et al., 2003).
The first nation in the world to adopt a comprehensive program for creating and advancing ethanol-based biofuels was the emerging country Brazil. This initiative was known as PROALCOOL (Programa Nacional do lcool); to address the issues posed by the crisis in crude oil that resulted from the Middle East. The crisis caused a significant increase in crude oil prices, which posed a severe challenge to the Brazilian economy. To increase the commercial use of biofuels, the program was introduced. It was a combination of research and development that supported biofuels, the creation of ethanol-powered vehicles, the expansion of sugarcane distilleries through subsidies, the installation of ethanol pumps, and price controls (Ludwig et al., 2003). In the beginning, the programme was quite adequate, and between 1975 and 1984, Brazil’s production of biofuels from sugarcane expanded quickly from less than one billion to over 12 billion tons (Bennamoun, 2022). In all expanses of the globe, the price of petroleum products fell dramatically and steadily toward the end of the 1980s. Still, the price of sugar rose, which resulted in a decrease in the production of sugarcane-based biofuel since sugarcane was mostly used for the production of sugar. The upshot of this is that ethanol, a biofuel made from sugarcane, became comparatively expensive compared to gasoline, which also caused the government to lose interest in ethanol production from sugarcane (Yadav et al., 2021). China has started creating biofuel regulations to reduce its reliance on crude oil and greenhouse gas (GHG) emissions (Singal and Singh, 2007). China started producing biofuels when worries about the safety of its domestic energy supply were growing (Shahid et al., 2021). Just behind the United States of America, China is currently the world’s second-largest importer of crude oil (Dafnomilis et al., 2017), and by 2030, it is predicted that imports will supply 75% of China’s energy requirements (Demirbaş, 2006). The economy of China also has a severe issue with environmental pollution and deterioration. These environmental pollution and degradation issues have gotten out of hand, and the international community is putting more and more pressure on China to reduce its carbon emissions (Matsuo et al., 2013). China began its biofuel program in early 2000, intending to supply 10% of its total energy demand through biofuel by 2010, and by 2020, biofuel must have contributed 15% of China’s overall energy requirement (Shahsavari and Akbari, 2018). China’s biofuel initiative started with five large-scale plants that can produce 1.87 billion tons of ethanol (Shahsavari and Akbari, 2018). China is currently the third-largest manufacturer in the world of biofuel (Matsuo et al., 2013; Shahsavari and Akbari, 2018), but comparable to Brazil, there is growing competition between bioethanol production and food security.
2.5 Environmental impacts
Because of their abundance and renewability, bioenergy has clear advantages over conventional fossil fuels and is essential to maintaining global energy security. When implementing bioenergy production, it is critical to consider resource costs and environmental effects (Khatib, 2012; Salgado et al., 2021). Although the manufacture of bioenergy can harm the environment, including soil erosion, greenhouse gas emissions, and biodiversity the adverse impact on the environment varies greatly subject to the type of plant, the source of the land, and the organization procedures used. Finding the ideal cultivation sites, bioenergy crop varieties, and management techniques can help the environment and the production of bioenergy (Ang et al., 2015; Jonsson et al., 2015). Although China has a significant potential for producing bioenergy, its bioenergy output has lagged and is not keeping up with the country’s expanding energy needs. Future studies should study the leading nations in this area, learn more, and develop the best decision support systems to direct the growth of bioenergy in China and other emerging nations (Bauen, 2006).
2.6 Obstacles to effective utilization of bioenergy in developing countries
Maltsglou et al. (Goldemberg, 2000) acknowledged that in comparison to countries like the US, Brazil, Sweden, and certain European Countries, understanding bioenergy and its production in Africa is still in its infancy. There are not many policies in place in developing countries such as Africa to accept this alternative energy source, which contributes to its unpopularity. Utilizing food as a source of raw materials for biofuel presents a hurdle since it competes with the food chain and puts a cap on its further development. In contrast to diesel and gasoline, other nations that support its production do not subsidize the cost of biofuel manufacturing. General difficulties include inadequate domestic raw material availability and a mismatch between feedstock supply and biofuel processing capacity. Bioenergy policy should be founded on thoroughly comprehending the country context, food security context, and sustainable bioenergy potential to ensure that bioenergy can be generated sustainably. The market obstacles for three bioenergy products, including bioethanol, biodiesel, and wood pellets, were found by Junginger et al. (Bauen, 2006). Junginger et al. (Bauen, 2006) found that whereas logistics are primarily perceived as a barrier for wood pellets, import taxes and the adoption of mechanisms for sustainability certification are considered significant obstacles to the trading of biodiesel and bioethanol. Biofuel’s import taxes and duties may be lessened or eliminated concerning multilateral harmonization of trade agreements which may deliver the needed prerequisites for continued, steady expansion of global commerce in bioenergy (Bauen, 2006). Suzuki (Goldemberg, 2000) identifies several challenges that are frequently encountered in developing countries, including a restricted capability to evaluate, embrace, adjust, and engage technology alternatives, a lack of proficiency in managing and operating technology, an absence of trained employees or training resources, an inability to obtain financing, maybe not being commercially viable, banking institutions are lacking to support renewable energy know-how, inadequate equipment, and unclear governmental policies. The difficulty of attracting investment has been cited by Bakhtiar (Kaygusuz, 2012) as an obstacle to the commercialization of biomass-utilizing energy in underdeveloped nations. The biggest obstacle to the success of BET investments is their high reliance on public and governmental financial resources. According to Ravindranath (Michoud and Hafner, 2021), the buildout of bioenergy technology in emerging nations has been relatively gradual because of institutional, technical, informational, commercial, and financial barriers (Owen et al., 2013; Michoud and Hafner, 2021).
3 Sustainability issues of bioenergy production in developing countries
Sustainable human development can be attained through the effective utilization of energy. Although it is an essential component of economic activities, how we generate, deliver, and use energy needs to change significantly (Biermann et al., 2012). Fossil fuels, which severely affect air quality and significantly increase carbon emissions, comprise a massive percentage of the existing energy system (Glemarec, 2012; Chirambo, 2018). The global energy demand is significantly increasing due to population and fiscal expansion, mainly in developing market countries, which will be responsible for 90% of the rise in energy consumption through 2035. There is currently a window of opportunity to undertake a transformative change in the energy supply industry to satisfy economic and environmental goals, given the need to replace outdated plants and add new capacity, particularly in emerging nations, to meet the expanding electricity demand (Berndes et al., 2003; Rose et al., 2014; Lynd et al., 2015; Souza et al., 2017).
When assessing energy alternatives, it is vital to consider three main factors: economic competitiveness, energy security, and environmental effects. Regrettably, economic competitiveness frequently takes precedence since many sustainable energy options are expensive, especially in places with limited resources. We must, however, come up with a solution for a future with sustainable energy in both developing and developed nations since climate change affects everyone. Then, how can we afford low- and middle-income countries with the tools they need to switch to a future run-on sustainable energy? Financial strategies and other technology developments will be required to make renewable energy systems that can compete economically with older fossil fuel-burning technologies. Continuous technological advancement can lead to applications tailored to local conditions and enable the founding of novel “clean industries,” which could contribute to the economic growth of these emerging nations. Still, it can lower a few trade-offs between new, renewable energy technologies and their market by lowering financiers’ risk and improbability when competing with conventional energy sources (Fischer and Schrattenholzer, 2001; Balat and Ayar, 2005; Reid et al., 2020).
3.1 Policy on land use strategy for bioenergy production
Due to a potential change in land use and crop planting patterns, policymakers must carefully consider the economic and environmental trade-offs associated with the production of food, feed, and fuel, as well as the impact on food security. Another crucial factor that national policymakers should consider is the processing and conversion technological part of the development and manufacturing of biofuels. Instead of using food crops, future bioenergy technologies might rely on waste agricultural and forestry products and specialized energy crops. Energy security and climate change mitigation may benefit more from second-generation bioenergy production and processing technology (World Bioenergy Association, 2018; Statistics and WBA Global Bioenergy, 2020).
As nations use more biofuels to strengthen their energy security, they should work to ensure that poor people and small farmers are involved in creating a more sustainable energy system taking a cue from Figure 18. With the right technology and trade policies, bioenergy in developing countries can produce win-win outcomes that are good for the impoverished and for energy efficiency. A greater number of times should be spent utilizing cutting-edge economic tools like the Clean Development Mechanism (CDM) and carbon market trading. In order to meet the requirements of the Kyoto Protocol and reduce greenhouse gas emissions that contribute to climate change, additional private and governmental investment must be mobilized (Pellegrini and Fernández, 2018).
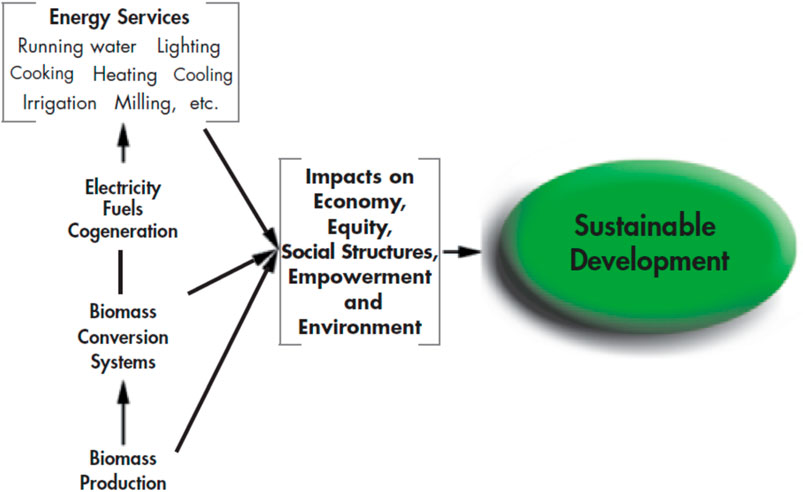
FIGURE 18. Conceptual representation of biomass energy systems and linkages to sustainable human development (Pellegrini and Fernández, 2018; World Bioenergy Association, 2018; Statistics and WBA Global Bioenergy, 2020).
3.2 Policy options and recommendations for promoting utilization of bioenergy for sustainable development
Around the world, over 600 million people live in places without electricity, and in developing nations, 2.06 billion people utilize solid fuels for heating and cooking. For many regions of the world to have universal entrée to energy, efficient policies, simplified institutional frameworks, and creative finance will be necessary (Adams et al., 2011; Kang et al., 2015; Guo and Song, 2019; Pulighe et al., 2019; Kardung et al., 2021).
Since energy access is a development issue, a sector-wide strategy is required. This strategy depends on several enabling elements, including business financing, technology ownership and availability, productive activities, and future growth prospects. Additionally, long-term technological adaptation and community growth must be taken into account by policymakers. Planning, coordinating, and implementing energy access policies and programs, fostering policy dialogue, exchanging knowledge, enlisting key partners, and raising funds require suitable institutional arrangements. It is necessary to have a capable and adaptable national institution responsible for delivering dependable, high-quality, and timely outcomes. To strengthen institutional capacity, it is necessary to examine the mandates of pertinent government sectors, such as
• identify their limitations, as well as their technical and financial capabilities;
• streamline those sectors to encourage cooperation and coordination;
• encourage cooperation between governments in planning and implementing;
• ensure that the legislative and regulatory frameworks are appropriate;
• establish an appropriate regulatory framework;
• develop a legal and institutional framework; (Naqvi et al., 2018; Blair et al., 2021).
To satisfy the growing demand for essentials from rural and urban residents, emerging nations like Latin America, India, Nigeria, etc., have recognized the necessity for alternate forms of energy and have thus given substantial support for it (Agbedahin, 2019; Zaharia et al., 2019; Ohunakin et al., 2023). The list of developing nations is presented in Table 2.
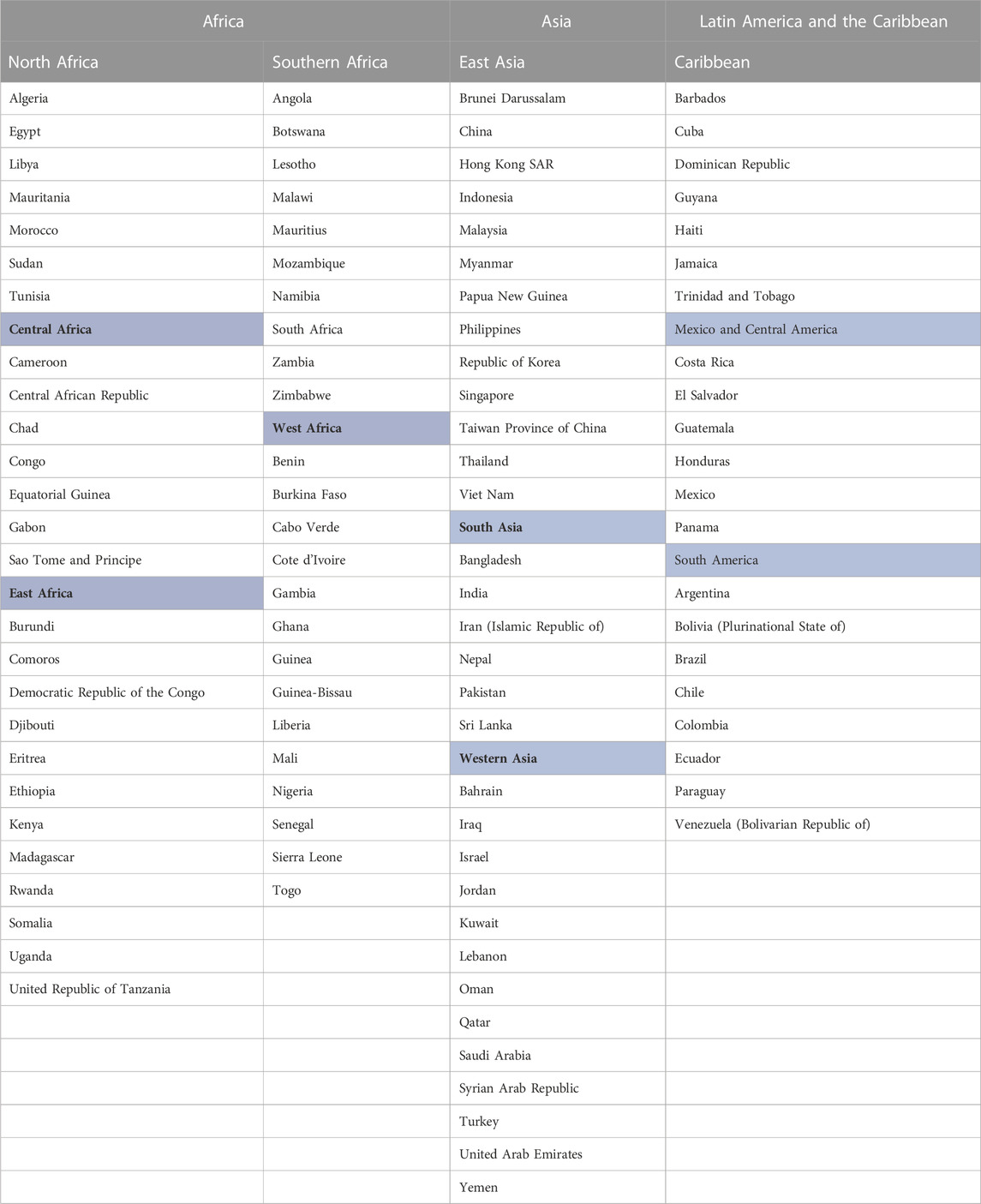
TABLE 2. Developing countries by region (Ailawadi and Bhattacharyya, 2006; Obaideen et al., 2021).
Biogas is a gas created by the anaerobic digestion or fermentation of organic waste under anaerobic conditions and is another type of bioenergy (Ailawadi and Bhattacharyya, 2006; Obaideen et al., 2021).
The degree of bioenergy deployment is not at the level required to meet the medium- and long-term objectives. This is due to several obstacles, including supply chain issues (such as unstable feedstock supplies, a lack of qualified workers, and sustainability risks), financial and economic issues (such as fossil fuel subsidies, high costs, and access to affordable financing), technical and infrastructure issues (such as low technology readiness and reliability), and informational issues (IRENA, 2022).
Sustainability in bioenergy is a complicated issue. The usage of bioenergy has several potential advantages. Substituting fossil fuels for them in the production of electricity, heating, transportation, and manufacturing, for instance, can reduce GHG emissions. Additionally, it may have positive effects on the environment and society. In the meanwhile, if bioenergy production is not properly controlled, it could have negative effects on the environment, society, or the economy outside the energy sector. These effects result from the close connections that bioenergy has with several crucial industries, including waste management, forestry, agriculture, and rural development. The traditional usage of biomass should gradually be phased out, and the loss of carbon stocks, or emissions through indirect land-use change and the supply chain, should be taken into account while decarbonizing through bioenergy (IRENA, 2022).
In one place but not in another, a particular bioenergy production approach can be seen as sustainable. For instance, this can be a result of climatic and biophysical variations. The availability of bioenergy at a location is also influenced by socio-economic aspects like culture, politics (especially when it comes to land ownership), and population. A comprehensive framework that includes setting sustainability goals and long-term planning, coordinating bioenergy across sectors, supporting sustainability governance with rules and certificate programs, and integrating bioenergy policy with the SDGs could facilitate decision-making and sustainability about the development of bioenergy; see Figure 19.
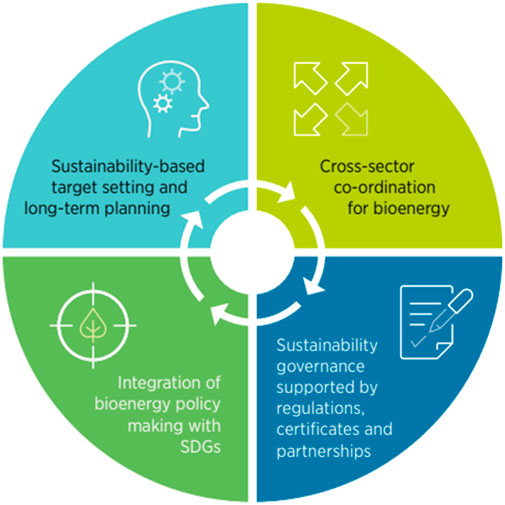
FIGURE 19. An institutional structure for the development of sustainable bioenergy (IRENA, 2022).
A long-term national bioenergy development strategy and plan can offer a dependable and long-term policy signal to inspire trust in investors project developers and direct policymakers. Long-term plans with numerous objectives will enable higher investments in innovations and speed up commercialization and scale-up (IRENA, 2022). With the help of practical strategies and incentives, clear medium- and long-term bioenergy targets can increase the certainty of policy. The amount of feedstock that can be obtained sustainably should, nevertheless, be the basis for bioenergy targets. This would stop further detrimental effects on the environment and society. Long-term bioenergy growth necessitates coordinated and integrated policies and action plans across several industries, including energy, forestry, agriculture, and the environment. Cross-sector coordination and integration can promote interdepartmental synergy, assure policy coherence, and head off possible sustainability problems (IRENA, 2022).
Mandates and obligations may boost interest in and demand for bioenergy. These include mandates for the use of renewable energy sources in heating or responsibilities for the transportation sector to combine biofuels. They might also include regulations governing the production or use of bioenergy, such as licenses, permits, and quotas. The growth of the bioenergy sector may also be aided by pledges and policies that forbid the use of fossil fuels in particular end-use industries. In this context, nations must quickly phase off subsidies for the production and use of fossil fuels, notably the G20 nations who are responsible for the majority of the subsidies (IRENA, 2022). Furthermore, carbon pricing policies are required to level the playing field for renewable energy sources such as bioenergy. Through the reduction of taxes and fees, such as the value-added tax on bioenergy producers and import tariffs on liquid biofuels, bioenergy products could become more cost-competitive than their fossil fuel counterparts. Supporting bioenergy and other renewable technologies frequently takes the form of capital grants and subsidies. They lower the initial outlay and ongoing expenses.
Supporting innovation through technical research, development, and demonstration can improve technology readiness and hasten the commercialization of new biofuels for usage in industries, such as aviation biofuels. National and regional governments can promote technology adoption by raising understanding of the advantages and difficulties connected with bioenergy (IRENA, 2022).
3.3 Important metrics for the supply and use of bioenergy
To meet the 1.5°C climate goal, bioenergy is essential (IRENA, 2022). It is crucial for the transition to an energy mix based on renewables because of its potential to replace fossil fuel in all energy sectors, including the production of electricity, end uses in industry, buildings, and transportation, as well as a chemical feedstock. Bioenergy made approximately 9.5% of the total primary energy supply in 2020. Modern uses of solid biomass accounted for 43% of the total bioenergy supply, followed by biomass used for developing nations’ traditional cooking and heating (39%) and biogas and biofuel feedstocks (18%) (IRENA, 2022).
From Figure 20, the biomass supply would need to increase from the 2020 level (56 EJ) to 86 EJ by 2030 and 135 EJ by 2050 under the 1.5°C Scenario. Reducing the wasteful use of conventional biomass, which is now utilized as fuel for heating and cooking, is one aspect of this. By 2030, modern bioenergy will need to expand by a factor of roughly 2.5 from 33 EJ (current levels), and by 2050, it will need to increase by four times. Although the estimate of 135 EJ of biomass supply for 2050, as predicted by IRENA (IRENA, 2022) and other institutions, is on the higher end of what might be sustainably produced, such a level can in theory be supplied sustainably without adverse changes in land use. Scaling up biomass production to those levels, though, will be extremely difficult to do without having negative effects on the environment or society.
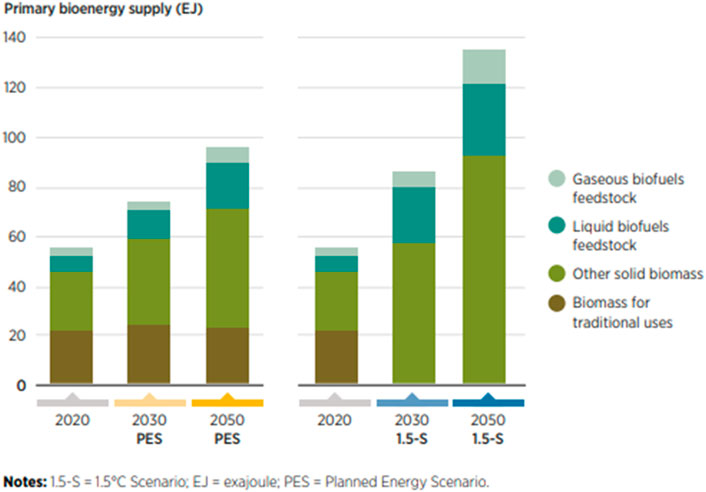
FIGURE 20. Primary bioenergy supply by carrier in 2020, 2030, and 2050 under the 1.5°C Scenario and the Planned Energy Scenario (IRENA, 2022).
Under the 1.5°C Scenario, bioenergy would account for roughly 4.5% of the generation, or 6.5 EJ in 2030 and 14.9 EJ in 2050. This represents an increase over current levels of more than three and seven times, respectively. To reach the net-zero objective, the use of biomass in conjunction with CCS in the electricity sector and some industrial sectors would be crucial. By 2050, processes that use biomass and to which CCS could theoretically be applied might perhaps capture 10 GtCO2 equivalent year (Lyons et al., 2021). Traditional biomass use accounted for 51% of its consumption, with modern uses in industry (22%), buildings (17%), transportation (9%), and miscellaneous use (1%) rounding out the breakdown as shown in Figure 21. The most significant source of energy in several developing nations is biomass, but only under specific circumstances is it sustainable. Only when realistically replaced is it renewable. Deforestation is caused by the widespread use of biomass in emerging nations, whether it be on a small or large scale for industrial uses. Additionally, the use of biomass for heating and cooking in underdeveloped nations is a significant contributor to acute indoor pollution, especially for women, young children, and the elderly (IRENA, 2022). Biomass is typically used in open flames and basic, inefficient stoves. This kind of usage has detrimental effects on one’s health by causing indoor air pollution (Goldemberg and Coelho, 2004). Additionally, it causes the destruction of forests, which raises greenhouse gas emissions and reduces biodiversity. As stated in UN Sustainable Development Goal (SDG) 7 (IEA et al., 2021: 7), a global effort is required to ensure that everyone has access to clean cooking fuel. The main actions necessary to achieve the 1.5°C Scenario are a significant decrease in the use of inefficient (traditional) biomass by 2030, increased use of modern biomass for building energy until 2030, stabilizing growth until 2050, steep increases in bioenergy in industry until 2050, and increased use of biofuels for shipping, aviation, and land transportation (IRENA, 2022).
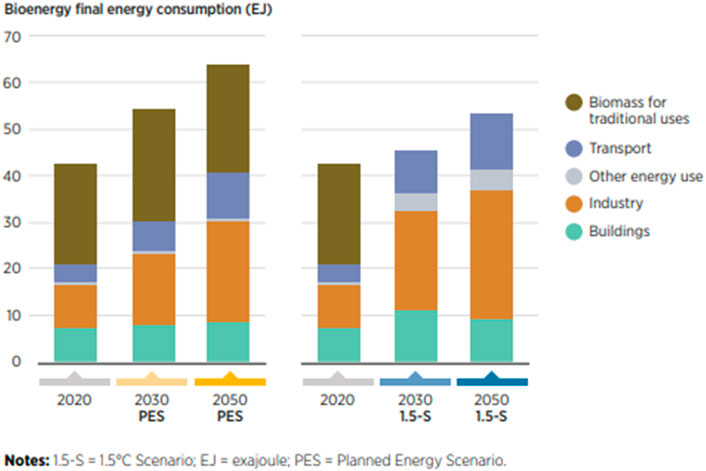
FIGURE 21. Sector-specific final energy consumption of bioenergy in 2020, 2030, and 2050 under the 1.5°C Scenario and the Planned Energy Scenario (IRENA, 2022).
The 1.5°C Scenario anticipates significant reductions in the sector of buildings’ inefficient use of biomass for heating and cooking by 2030. By 2030, it will be necessary to deploy clean technologies such as upgraded cookstoves that are efficient and run on sustainably produced bioenergy or electric stoves to ensure that everyone has access to clean cooking technology and fuels. By 2050, 9.4 EJ of modern bioenergy will be used in buildings, up from 7.3 EJ in 2020, 11.3 EJ in 2030, and 7.3 EJ in 2020. In industrial applications for medium- and high-temperature heat as well as chemical feedstock, bioenergy will be crucial. Global bioenergy demand in the industrial sector would be 21 EJ by 2030 and 27.6 EJ by 2050 under the 1.5°C Scenario. Global chemical and petrochemical sector production of bio-based plastic would increase from a little over 2 Mt currently to 73 Mt annually. This would account for about 20% of the overall production of plastic. By 2050, the production of other synthetic organic compounds will increase to 51 Mt. Currently, the manufacture of chemicals uses a small number of bio-based feedstocks. About 1% of plastics and fewer than 1% of chemicals are made using bio-based feedstocks. In 2030 and 2050, respectively, the 1.5°C Scenario sees 4.1 EJ and 10.9 EJ of bioenergy feedstocks used for chemical manufacturing (IRENA, 2022).
Transport decarbonization is aided by bioenergy. According to the 1.5°C Scenario, bioenergy use in transportation would increase from 3.7 EJ in 2020 to 9.1 EJ in 2030 and 12 EJ in 2050. Improvements in fuel economy and an increase in the number of electric vehicles on the road would be complemented by this growth. Up until worldwide fleets are electrified, increasing biofuel blending ratios, especially in this decade, may be essential to reducing emissions from road transport. Biojet fuels are much more prevalent in aviation. They provide chances to decarbonize the industry when used in conjunction with synthetic fuels. The annual production of biojet fuels would be over 110 billion liters under the 1.5°C Scenario. By 2050, the output of liquid biofuels, which would nearly double, would be able to fulfill 24% of the transportation sector’s total energy requirement. In the maritime industry, biofuel would have a smaller impact, making up only 10% of the energy mix by 2050 under the 1.5°C Scenario. Under the 1.5°C Scenario, Figure 22 lists important statistics for bioenergy supply and consumption in 2030 and 2050 (IRENA, 2022).
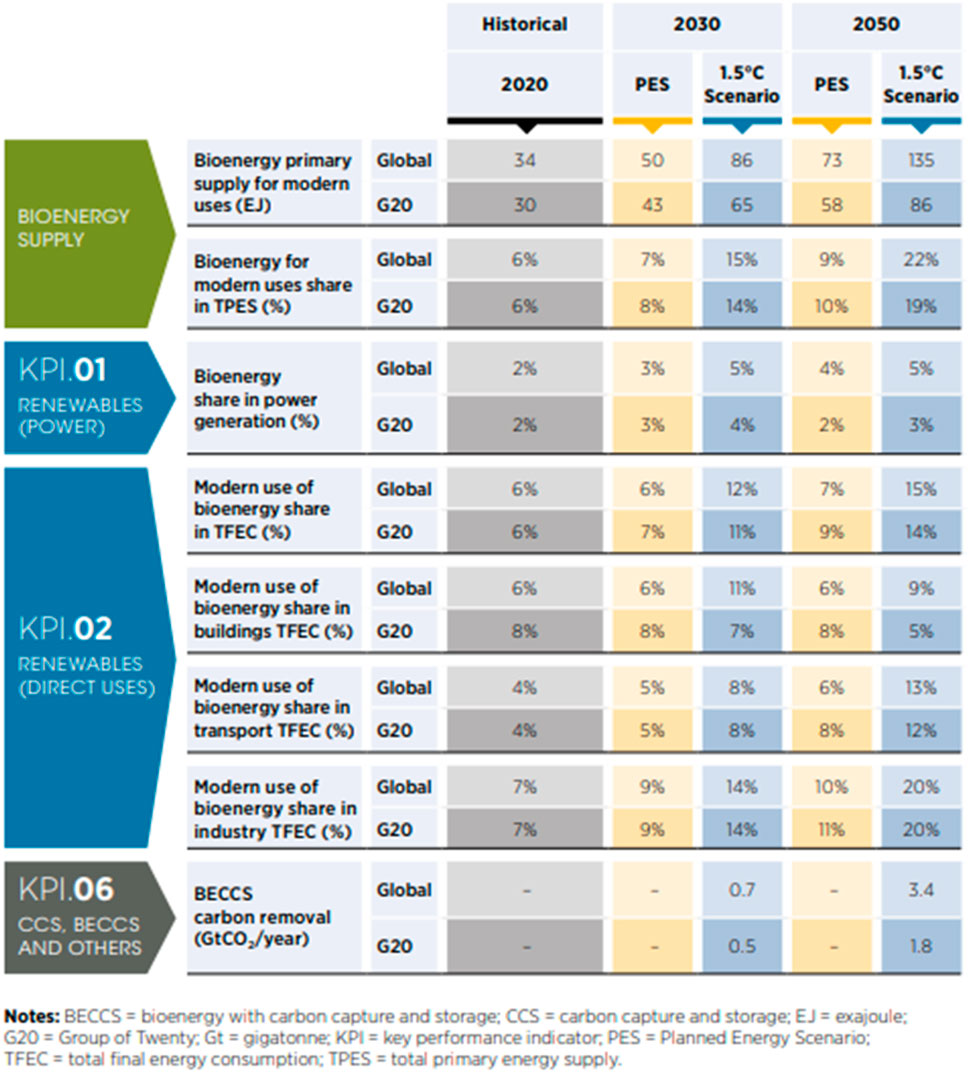
FIGURE 22. Key performance metrics for bioenergy supply and consumption: 1.5°C Scenario in 2030 and 2050 and Planned Energy Scenario (IRENA, 2022).
4 Modern bioenergy and renewables
The use of bioenergy must be doubled by 2060 to slow global warming (IRENA, 2022). The International Energy Agency (IEA) recommends increasing the use of advanced biofuels in transportation as one important strategy for accomplishing this goal. While the use of solar and wind energy for power generation is expanding, according to the IEA, bioenergy is presently the only renewable energy source that can be used to directly generate electricity, heat, and transportation fuels.
One way to reduce carbon dioxide emissions while still meeting the country’s fast-rising energy needs is to replace modern bioenergy with fossil fuels, provided that it is generated sustainably. Heat, electricity, gaseous biofuels (such as biogas and hydrogen), liquid biofuels (such as biodiesel, bioethanol, methanol, and Fischer-Tropsch (FT) synfuels), and other energy carriers/services can all be produced from bioenergy. Therefore, each form of fossil fuel might potentially be replaced by contemporary bioenergy. Additionally capable of supplying base loads, bioelectricity is essential for balancing the grid and offering storage possibilities (IRENA, 2022).
4.1 Modern bioenergy in Africa
Modern bioenergy sources include biofuels, which are mostly used in transportation, biogas, which is used to cook cleanly, biomethane, which is used to produce electricity, and solid biomass, such as wood pellets, which are used to heat homes. Depending on the kind of feedstock and the method of production, biogas can have a variety of different compositions. Even though production is now very low, Africa has enough sustainable feedstock to meet all of its energy needs for clean cooking. We calculate that the continent has the potential to create close to 2 EJ of locally produced low-carbon biogas, which may double to 4 EJ by 2040 (IEA, 2020). This estimate is based on a bottom-up analysis (Sovacool and Monyei, 2021; IRENA, 2022).
In Africa nowadays, most biogas is used in nations with dedicated support initiatives. While many initiatives supported by non-governmental organizations offer useful know-how and subsidies to lower the net investment cost, certain governments, including those in Benin, Burkina Faso, and Ethiopia, offer subsidies that can cover up to half of the investment (SNV, 2019). Additionally, a few nations have established financial facilities, like as Kenya, where a lease-to-own agreement financed roughly half of the anaerobic digester installations in 2018. By 2030, the SAS projects that 66 PJ of biogas will be used for cooking in Africa, significantly increasing access to clean cooking. This is being driven by significant subsidies to reduce the digester’s relatively high start-up cost. A typical size family unit with a technological lifespan of more than 20 years can cost somewhere between USD 500 and USD 800 in Africa. Other clean cooking systems have significantly less expensive installation expenses (Sovacool and Monyei, 2021; IRENA, 2022).
However, since fuel expenses are low or non-existent after 2 years of continuous operation, digesters may be more affordable in terms of total cost of ownership. As a by-product, they can also create biofertilizers. Senegal’s National Domestic Biogas Programme implemented a strategy to reduce the cost of biodigesters by creating a market for bio-fertilizers. Currently, just a small portion of Africa’s total final consumption is made up of biofuels for transportation, but this segment has enormous growth potential (Sovacool and Monyei, 2021; IRENA, 2022).
4.2 Sustainable energy development Indices
The economy’s development, people’s wellbeing, and poverty’s reduction depend on having access to energy. Global development faces the constant and urgent problem of ensuring everyone has enough access (Ailawadi and Bhattacharyya, 2006; Agbedahin, 2019; Zaharia et al., 2019). Energy systems do, however, also have significant environmental effects (United Nations Conference on Environment and Development UNCED, 1992; Michaelowa et al., 2019; UNEP, 2023). The present-day energy production and consumption patterns, which have historically influenced the course of development, are not sustainable. Providing energy services that enable all people to reach a decent quality of life in line with sustainable human growth is the current energy challenge that nations worldwide must address. This continuing connection between energy and development is a crucial aspect of development policy (United Nations Conference on Environment and Development UNCED, 1992; Michaelowa et al., 2019; UNEP, 2023).
According to the World Energy Council (United Nations Conference on Environment and Development UNCED, 1992; Michaelowa et al., 2019; UNEP, 2023), tackling the three objectives of energy accessibility, availability, and acceptability is essential to promoting new energy business strategies for the twenty-first century, promoting global political stability, and creating a sustainable future. As a result of the work done to create Agenda 21, UNDP has also determined (Yuwan and Xuemei, 2017; Chen et al., 2019) the need for a focused analysis of the role of energy in achieving sustainable socio-economic development and has identified some steps that must be taken to increase the adoption of sustainable energy options.
It is widely known that energy use needs to be decreased to lessen the continual climatic changes that result from it. The United Nations has created a set of 17 sustainable development goals through their 2015 sustainable development plan known as the 2030 Agenda. Goal number seven in this set, “low-cost, sustainable energy,” explicitly mentions the energy industry (Attabo et al., 2023). This objective has two components: improving access to electricity for underserved or remote populations and boosting the number of renewable energy sources in overall energy use may even totally replace traditional energy generation in some cases (Tracking Clean Energy Progress, 2023).
5 Concept of clean development mechanism (CDM)
An “Annex B Party” (a country that has agreed to restrict or reduce its emissions following the Kyoto Protocol) may carry out an emission-reduction project in developing countries per the Clean Development Mechanism (CDM), which is outlined in Article 12 of the Protocol. The CDM is an intriguing tool for encouraging fresh initiatives in renewable and energy-efficient projects. The Millennium Development Goals (MDGs) must be achieved to provide contemporary energy services that are clean, dependable, and inexpensive (Tolliver et al., 2019). The Clean Development Mechanism is the only one that includes developing nations out of the three flexibility mechanisms in the Kyoto Protocol designed to include the market in reaching the obligations of the industrialized countries (Babatunde et al., 2020). The CDM seeks to encourage sustainable development in developing nations by channeling private sector funding into projects that reduce emissions in these nations (Hultman et al., 2020; Oyedepo and Fakeye, 2023).
The CDM is exceptional because the product it delivers—reductions in greenhouse gas emissions—can be sold on international markets without any physical infrastructure. Therefore, if transaction costs and other barriers can be lowered, even small-scale development initiatives might theoretically boost the amount of money that flows to developing countries through the budding market for greenhouse gas reductions. Future energy policies will be greatly impacted by efforts to mitigate the effects of global climate change (Nyika et al., 2021). CDM can contribute significantly to sustainable development through the increase in energy efficiency and conservation, employment generation, a more sustainable energy generation, and technology transfer between developed and developing countries (Bio energy, 2023).
5.1 Energy gaps in Africa
There are still enormous gaps in accurate data and statistics, and millions of people are still suffering from energy poverty and the negative effects of climate change. The COVID-19 pandemic has caused financial turmoil in more than 20 African nations and halted efforts to increase access to energy. These issues are made worse by the abrupt spikes in energy and food costs brought on by Russia’s invasion of Ukraine, which are impacting many consumers and businesses worldwide, most acutely in underdeveloped countries where people have the least access to these resources (Senyagwa, 2022; Zakeri et al., 2022). Fossil fuel are still much utilized as fuel source in Africa and contribute to emissions to the environment which can only be minimized when the government invests in renewable energies.
6 Future consideration and conclusion
Africa has the fastest rates of population increase, the largest frequency of food insecurity, and the lowest crop yields, but it also has the highest proportion of arable land and, in contrast to energy demand, the biggest amount of land resources (Senyagwa, 2022). Modern bioenergy development, agricultural development, and food security may all work in unison rather than counter to one another. They can be accomplished by clear leadership, effective administration, and the adaptation of business models, expertise, and technologies to the various local conditions. Modern bioenergy has the potential to alter Africa, with societal advantages spreading across many other fields and going far beyond just energy production (Löhr et al., 2022).
Political pressure to implement plans for utilizing renewable energy is constantly emergent due to Europe’s significant import need for fuel and its pledges to lessen CO2 emissions under the Kyoto Protocol. It is essential to reach the 1.5°C objective that the electricity industry is decarbonized by 2050 (IRENA, 2022) As a result, Europe hopes to use a lot more biofuels than it presently generates (Löhr et al., 2022).
Europe and Africa have a lot of room to grow its bioenergy program without endangering its ability to produce food. In France, Germany, and Spain, this potential is most significant but in contrast to Brazil, Europe produces biofuels at a comparatively high cost. Despite the substantial social and environmental advantages of the current initiatives, these may be exceeded by their financial costs as compared to other strategies for assisting rural communities and lowering greenhouse gas emissions. Any solution will be hampered by the vested interests that have already been established in the domestic business—finding the ideal balance between supporting a domestic biofuel program and adopting more economically effective techniques. Africa explore biofuel production by setting aside land for its purpose and by developing technologies that make biomass crops more productive economically and energetically. The following recommendations can be adopted:
• Large-scale awareness campaigns about the use of biogas should be launched on television, radio, and in newspapers.
• Governmental organizations should improve and increase credits and financial incentives to promote the usage of biogas.
• Farmers planting energy-generating crops like rapeseed, sugar cane, and jatropha should also receive incentives.
• The home and industrial growth of various biogas digesters using various feedstocks should be taken into account.
• Laws that forbid the indiscriminate felling of trees for wood fuel should be passed and bravely put into effect; this could encourage the use of alternate energy sources, particularly for household purposes.
• Suppose government efforts to achieve reliable, economical, clean, and steady power generation through Bioenergy Technologies (BETs) are increased. In that case, well-designed programs and the implementation of policies must be done with zeal and perseverance. People should be given a chance and encouraged to operate small to medium-sized agricultural businesses to produce cow dung, poultry droppings, and other agricultural waste.
• Government funding should be allocated for technical assistance, capacity-building, and research and development in the biofuel industry.
• Farmers, scholars, institutions from the public and private sectors, investors, and non-state actors should all be encouraged to participate in the growth of the biofuels business through establishing institutional and regulatory frameworks by the government
Promoting the use of renewable energy sources (such as bioenergy) in developing countries can increase access for rural families, reduce energy costs for households and businesses as they enter the middle class, and cut greenhouse gas emissions.
Decision-makers should take advantage of new opportunities in sectors like critical minerals, modern bioenergy and green hydrogen to shift attention from over depending on fossil fuels.
In addition to making deployment easier, comprehensive strategies are required to guarantee that the shift will have significant socioeconomic advantages. Legislation must include net-zero promises and translate them into implementation plans with sufficient funding. Climate announcements would remain aspirational and the required advancement would be impossible without this important step.
Given the longevity of the energy sector’s infrastructure, long-term planning should be done before investing in fixed infrastructure. Demand will be altered by the electrification of end users. Modernizing the current infrastructure and expanding and reinforcing the grid on land and at sea are prerequisites for the use of renewable energy. Other than the current oil and gas fields, green hydrogen will also be produced in these other places. The environmental and social implications should be adequately addressed from the outset, as well as the technical difficulties and financial costs of revamping infrastructure. Hundreds of millions of people’s qualities of life will be improved by a just and inclusive energy transition, which will aid in bridging wide inequities. Policies for the energy transition must be in sync with broader systemic changes intended to protect human wellbeing, enhance international equality, and bring the global economy into compliance with resource, environmental, and climate limits. Supporting developing nations to quicken the energy transition could increase energy security while halting the expansion of the global decarbonization gap. A varied energy market would promote local value creation for commodity producers, lower supply chain risks, and enhance energy security. To the fullest extent possible, countries’ contributions to the global energy transition must have access to technology, training, capacity building, and reasonable financing, especially for those with a wealth of renewable energy sources and allied resources. The shift to a cleaner energy source must prioritize the security and wellbeing of people. Sustainable development must be a top priority in bioenergy policy. The fundamental pillars for developing Africa’s new energy economy are energy efficiency and renewables, particularly solar and contemporary bioenergy.
All Africans need to have access to modern electricity, which will require annual investments of USD 25 billion. International assistance is needed to encourage the necessary investment, and stronger national institutions on the ground are also needed to lay out clear strategies.
Author contributions
JD Updated the research, analysis of data, Wrote the paper, Edit the paper. ES Wrote the abstract, the analysis, and the interpretation of data. IE Wrote the paper, the analysis, and interpretation of data. NU Wrote the paper, the analysis, and interpretation of data. OF Wrote the paper, the analysis, and interpretation of data. SO Conceived the research, Wrote the paper, the analysis, and interpretation of data. OA the analysis and interpretation of data. SK Interpretation of data.
Funding
The author(s) declare(s) that no financial support was received for the research, authorship, and/or publication of this article.
Acknowledgments
The authors appreciate the continuous support for their publications from Covenant University Management.
Conflict of interest
The authors declare that the research was conducted in the absence of any commercial or financial relationships that could be construed as a potential conflict of interest.
The author SO declared that they were an editorial board member of Frontiers, at the time of submission. This had no impact on the peer review process and the final decision.
Publisher’s note
All claims expressed in this article are solely those of the authors and do not necessarily represent those of their affiliated organizations, or those of the publisher, the editors and the reviewers. Any product that may be evaluated in this article, or claim that may be made by its manufacturer, is not guaranteed or endorsed by the publisher.
Abbreviations
BETs, Bioenergy Technologies; BECCS, bioenergy with carbon capture and storage; CCS, carbon capture and storage; CDM, clean development mechanism; EJ, Exajoule; EU, European Union; FAO, Food and Agriculture Organization; GHG, greenhouse gas; GTOE, giga-tonnes of oil equivalent; GW, Giga Watt; IEA, International Energy Agency; IPCC, Intergovernmental Panel on Climate Change; IRENA, International Renewable Energy Agency; LPG, Liquefied petroleum gas; MDGs, Millennium Development Goals; NZE, Net Zero Emissions; OECD, Organisation for Economic Co-operation and Development; PROALCOOL, Programa Nacional do lcool; SDG, Sustainable Development Goal (SDG); SAS, Sustainable Africa Scenario; TFEC, total final energy consumption; TPES, Total primary energy supply.
References
Abas, N., Kalair, A., Khan, N., and Kalair, A. R. (2017). Review of GHG emissions in Pakistan compared to SAARC countries. Renew. Sustain. Energy Rev. 80, 990–1016. doi:10.1016/j.rser.2017.04.022
Adams, P. W., Hammond, G. P., McManus, M. C., and Mezzullo, W. G. (2011). Barriers to and drivers for UK bioenergy development. Renew. Sustain. Energy Rev. 15 (2), 1217–1227. doi:10.1016/j.rser.2010.09.039
Agbedahin, A. V. (2019). Sustainable development, education for sustainable development, and the 2030 agenda for sustainable development: emergence, efficacy, eminence, and future. Sustain. Dev. 27 (4), 669–680. doi:10.1002/sd.1931
Ailawadi, V. S., and Bhattacharyya, S. C. (2006). “Access to energy services by the poor in India: current situation and need for alternative strategies,” in Natural resources forum (vol. 30, No. 1, pp. 2-14) (Oxford, UK: Blackwell Publishing Ltd).
Ailleret, F. (2000). The energy challenges in the 21. century according to the World Energy Council (WEC). Liaison Energ. Francoph., 16–18.
Ang, B. W., Choong, W. L., and Ng, T. S. (2015). Energy security: definitions, dimensions and indexes. Renew. Sustain. energy Rev. 42, 1077–1093. doi:10.1016/j.rser.2014.10.064
Ardakani, F. J., and Ardehali, M. M. (2014). Long-term electrical energy consumption forecasting for developing and developed economies based on different optimized models and historical data types. Energy 65, 452–461. doi:10.1016/j.energy.2013.12.031
Arto, I., Capellán-Pérez, I., Lago, R., Bueno, G., and Bermejo, R. (2016). The energy requirements of a developed world. Energy Sustain. Dev. 33, 1–13. doi:10.1016/j.esd.2016.04.001
Attabo, A. A., Ajayi, O. O., Oyedepo, S. O., and Afolalu, S. A. (2023). Assessment of the wind energy potential and economic viability of selected sites along Nigeria's coastal and offshore locations. Front. Energy Res. 11, 1186095. doi:10.3389/fenrg.2023.1186095
Babatunde, O. M., Ayegbusi, C. O., Babatunde, D. E., Oluseyi, P. O., and Somefun, T. E. (2020). Electricity supply in Nigeria: cost comparison between grid power tariff and fossil-powered generator. Int. J. Energy Econ. Policy 10 (2), 160–164. doi:10.32479/ijeep.8590
Bakhtiar, A., Aslani, A., and Hosseini, S. M. (2020). Challenges of diffusion and commercialization of bioenergy in developing countries. Renew. energy 145, 1780–1798. doi:10.1016/j.renene.2019.06.126
Balat, M., and Ayar, G. (2005). Biomass energy in the world, use of biomass and potential trends. Energy sources. 27 (10), 931–940. doi:10.1080/00908310490449045
Balzani, V., and Armaroli, N. (2010). Energy for a sustainable world: from the oil age to a sun-powered future. New Jersey, United States: John Wiley & Sons.
Bauen, A. (2006). Future energy sources and systems—acting on climate change and energy security. J. Power Sources 157 (2), 893–901. doi:10.1016/j.jpowsour.2006.03.034
Bennamoun, L. (2022). Bioresource technology for bioenergy: development and trends. Energies 15 (5), 1717. doi:10.3390/en15051717
Benoit, P. (2019). Energy and development in a changing world: a framework for the 21st century. Amsterdam Avenue New York: Center on Global Energy Policy at Columbia University SIPA, 1–14.
Berndes, G., Hoogwijk, M., and Van den Broek, R. (2003). The contribution of biomass in the future global energy supply: a review of 17 studies. Biomass bioenergy 25 (1), 1–28. doi:10.1016/s0961-9534(02)00185-x
Bharathiraja, B., Sudharsana, T., Jayamuthunagai, J., Praveenkumar, R., Chozhavendhan, S., and Iyyappan, J. (2018). RETRACTED: biogas production–A review on composition, fuel properties, feed stock and principles of anaerobic digestion. Renew. Sustain. Energy Rev. 90, 570–582. April. doi:10.1016/j.rser.2018.03.093
Biermann, F., Abbott, K., Andresen, S., Bäckstrand, K., Bernstein, S., Betsill, M. M., et al. (2012). Transforming governance and institutions for global sustainability: key insights from the Earth System Governance Project. Curr. Opin. Environ. Sustain. 4 (1), 51–60. doi:10.1016/j.cosust.2012.01.014
Bio energy (2023). Tracking clean energy progress 2023. https://www.iea.org/energy-system/renewables/bioenergy#tracking.
Blair, M. J., Gagnon, B., Klain, A., and Kulišić, B. (2021). Contribution of biomass supply chains for bioenergy to sustainable development goals. Land 10 (2), 181. doi:10.3390/land10020181
Bößner, S., Devisscher, T., Suljada, T., Ismail, C. J., Sari, A., and Mondamina, N. W. (2019). Barriers and opportunities to bioenergy transitions: an integrated, multi-level perspective analysis of biogas uptake in Bali. Biomass bioenergy 122, 457–465. doi:10.1016/j.biombioe.2019.01.002
Buraimoh, O. M., Olusola, O. I., Humphrey, I., Raheem, I. A., Onyekachi, U. K., and Ogundipe, O. T. (2020). Bioconversion of organic wastes for sustainable biogas production using locally sourced materials. Int. J. Energy a Clean Environ. 21 (3), 201–219. doi:10.1615/interjenercleanenv.2020033074
Cao, X., Dai, X., and Liu, J. (2016). Building energy-consumption status worldwide and the state-of-the-art technologies for zero-energy buildings during the past decade. Energy Build. 128, 198–213. doi:10.1016/j.enbuild.2016.06.089
Chabhadiya, K., Srivastava, R. R., and Pathak, P. (2021). Growth projections against set-target of renewable energy and resultant impact on emissions reduction in India. Environ. Eng. Res. 26 (2), 1–11. doi:10.4491/eer.2020.083
Chavez, A. Y., Morales, R., Gonzalez, C., and Moya, F. V. (2020). Production of ethanol from two varieties of potato peel waste through cellulolytic and amylolytic enzymes. Int. J. Energy a Clean Environ. 21 (1), 41–58. doi:10.1615/interjenercleanenv.2020032719
Chen, C., Xue, B., Cai, G., Thomas, H., and Stückrad, S. (2019). Comparing the energy transitions in Germany and China: synergies and recommendations. Energy Rep. 5, 1249–1260. doi:10.1016/j.egyr.2019.08.087
Chirambo, D. (2018). Towards the achievement of SDG 7 in sub-Saharan Africa: creating synergies between Power Africa, Sustainable Energy for All and climate finance in-order to achieve universal energy access before 2030. Renew. Sustain. Energy Rev. 94, 600–608. doi:10.1016/j.rser.2018.06.025
Chong, C. T., Loe, T. Y., Wong, K. Y., Ashokkumar, V., Lam, S. S., Chong, W. T., et al. (2021). Biodiesel sustainability: the global impact of potential biodiesel production on the energy–water–food (EWF) nexus. Environ. Technol. Innovation 22, 101408. doi:10.1016/j.eti.2021.101408
Dafnomilis, I., Hoefnagels, R., Pratama, Y. W., Schott, D. L., Lodewijks, G., and Junginger, M. (2017). Review of solid and liquid biofuel demand and supply in Northwest Europe towards 2030–A comparison of national and regional projections. Renew. Sustain. Energy Rev. 78, 31–45. doi:10.1016/j.rser.2017.04.108
Dahunsi, S. O., Fagbiele, O. O., and Yusuf, E. O. (2020). Bioenergy technologies adoption in Africa: a review of past and current status. J. Clean. Prod. 264, 121683. doi:10.1016/j.jclepro.2020.121683
Demirbaş, A. (2006). Global renewable energy resources. Energy sources. 28 (8), 779–792. doi:10.1080/00908310600718742
Ebhota, W. S., and Jen, T. C. (2020). Fossil fuels environmental challenges and the role of solar photovoltaic technology advances in fast tracking hybrid renewable energy system. Int. J. Precis. Eng. Manufacturing-Green Technol. 7 (1), 97–117. doi:10.1007/s40684-019-00101-9
EIA (2007). Annual energy outlook 2007. Washington, DC: Energy Information Administration, DOE/ EIA-0383. February, web site Available at: http://www.eia.doe.gov/oiaf/aeo.
Elavarasan, R. M., Afridhis, S., Vijayaraghavan, R. R., Subramaniam, U., and Nurunnabi, M. (2020). SWOT analysis: a framework for comprehensive evaluation of drivers and barriers for renewable energy development in significant countries. Energy Rep. 6, 1838–1864. doi:10.1016/j.egyr.2020.07.007
FAO (2013). FAO statistical yearbook: world food and agriculture. Rome: Food and Agriculture Organization of the United Nations. Available at: http://www.fao.org/docrep/018/i3107e/i3107e00.htm.
Faostat, F. A. O. (2019). Food and agriculture data. Crop Statistics. Available online: http://www.fao.org/faostat (Accessed on August 14, 2020).
Fischer, G., and Schrattenholzer, L. (2001). Global bioenergy potentials through 2050. Biomass bioenergy 20 (3), 151–159. doi:10.1016/s0961-9534(00)00074-x
Food and Agricultural Organization (FAO) (2004). Monitoring progress towards the world food summit and millennium development goals. The state of food insecurity report. Rome: Food and Agriculture Organization of the United Nations, Vialedelle Terme di Caracalla.
Gielen, D., Boshell, F., Saygin, D., Bazilian, M. D., Wagner, N., and Gorini, R. (2019). The role of renewable energy in the global energy transformation. Energy Strategy Rev. 24, 38–50. doi:10.1016/j.esr.2019.01.006
Glemarec, Y. (2012). Financing off-grid sustainable energy access for the poor. Energy policy 47, 87–93. doi:10.1016/j.enpol.2012.03.032
Goldemberg, J., and Coelho, S. T. (2004). Renewable energy—traditional biomass vs. modern biomass. Energy Policy 32 (6), 711–714. doi:10.1016/s0301-4215(02)00340-3
Grübler, A., Jefferson, M., and Nakićenović, N. (1996). Global energy perspectives: a summary of the joint study by the international Institute for applied systems analysis and world energy Council. Technol. Forecast. Soc. Change 51 (3), 237–264. doi:10.1016/0040-1625(95)00251-0
Guo, M., and Song, W. (2019). The growing US bioeconomy: drivers, development and constraints. New Biotechnol. 49, 48–57. doi:10.1016/j.nbt.2018.08.005
Gupta, A., and Verma, J. P. (2015). Sustainable bio-ethanol production from agro-residues: a review. Renew. Sustain. energy Rev. 41, 550–567. doi:10.1016/j.rser.2014.08.032
Haberl, H., Erb, K. H., Krausmann, F., Running, S., Searchinger, T. D., and Smith, W. K. (2013). Bioenergy: how much can we expect for 2050? Environ. Res. Lett. 8 (3), 031004. doi:10.1088/1748-9326/8/3/031004
He, X., Ou, S., Gan, Y., Lu, Z., Przesmitzki, S. V., Bouchard, J. L., et al. (2020). Greenhouse gas consequences of the China dual credit policy. Nat. Commun. 11 (1), 1–10. doi:10.1038/s41467-020-19036-w
HLPE (2014). Biofuels and food security: a report by the high-level panel of experts on food security on food security and nutrition. Rome: Committee of the World Food Security.
Hultman, N., Lou, J., and Hutton, S. (2020). A review of community co-benefits of the clean development mechanism (CDM). Environ. Res. Lett. 15 (5), 053002. doi:10.1088/1748-9326/ab6396
Johansson, T. B., Nakicenovic, N., Patwardhan, A., and Gomez-Echeverri, L. (2012). Global energy assessment toward a sustainable future. Cambridge, New York: Cambridge University Press, 13–113.
Jonsson, D. K., Johansson, B., Månsson, A., Nilsson, L. J., Nilsson, M., and Sonnsjö, H. (2015). Energy security matters in the EU energy roadmap. Energy Strategy Rev. 6, 48–56. doi:10.1016/j.esr.2015.03.002
Junginger, M., Van Dam, J., Zarrilli, S., Mohamed, F. A., Marchal, D., and Faaij, A. (2011). Opportunities and barriers for international bioenergy trade. Energy Policy 39 (4), 2028–2042. doi:10.1016/j.enpol.2011.01.040
Kang, S., Selosse, S., and Maïzi, N. (2015). Strategy of bioenergy development in the largest energy consumers of Asia (China, India, Japan and South Korea). Energy Strategy Rev. 8, 56–65. doi:10.1016/j.esr.2015.09.003
Kardung, M., Cingiz, K., Costenoble, O., Delahaye, R., Heijman, W., Lovrić, M., et al. (2021). Development of the circular bioeconomy: drivers and indicators. Sustainability 13 (1), 413. doi:10.3390/su13010413
Kartha, S., and Larson, E. D. (2000). Bioenergy primer: modernised biomass energy for sustainable development. New York, NY 10017, USA: UNDP, 10–24.
Kaygusuz, K. (2012). Energy for sustainable development: a case of developing countries. Renew. Sustain. Energy Rev. 16 (2), 1116–1126. doi:10.1016/j.rser.2011.11.013
Keho, Y. (2016). What drives energy consumption in developing countries? The experience of selected African countries. Energy Policy 91, 233–246. doi:10.1016/j.enpol.2016.01.010
Khatib, H. (2012). IEA world energy outlook 2011—a comment. Energy policy 48, 737–743. doi:10.1016/j.enpol.2012.06.007
Kumar, A., and Samadder, S. R. (2017). A review on technological options of waste to energy for effective management of municipal solid waste. Waste Manag. 69, 407–422. doi:10.1016/j.wasman.2017.08.046
Lebre La Rovere, E., Pereira, A. S., and Simões, A. F. (2011). Biofuels and sustainable energy development in Brazil. Development 39 (6), 1026–1036. doi:10.1016/j.worlddev.2010.01.004
Li, J., and Hu, S. (2017). History and future of the coal and coal chemical industry in China. Resour. Conservation Recycl. 124, 13–24. doi:10.1016/j.resconrec.2017.03.006
Lobanova, J. Z., Kračun, D., and Kavkler, A. (2018). Effects of cross-border mergers and acquisitions on GDP per capita and domestic investment in transition countries. J. Bus. Econ. Manag. 19 (1), 124–137. doi:10.3846/16111699.2017.1408677
Löhr, K., Matavel, C. E., Tadesse, S., Yazdanpanah, M., Sieber, S., and Komendantova, N. (2022). Just energy transition: learning from the past for a more just and sustainable hydrogen transition in west Africa. Land 11 (12), 2193. doi:10.3390/land11122193
Ludwig, J., Marufu, L. T., Huber, B., Andreae, M. O., and Helas, G. (2003). Domestic combustion of biomass fuels in developing countries: a major source of atmospheric pollutants. J. Atmos. Chem. 44 (1), 23–37. doi:10.1023/a:1022159910667
Lynd, L. R., Sow, M., Chimphango, A. F., Cortez, L. A., Brito Cruz, C. H., Elmissiry, M., et al. (2015). Bioenergy and african transformation. Biotechnol. biofuels 8 (1), 18. doi:10.1186/s13068-014-0188-5
Mahmoodi, M. (2017). The relationship between economic growth, renewable energy, and CO2 emissions: evidence from panel data approach. Int. J. Energy Econ. Policy 7 (6), 96.
Maltsoglou, I., Koizumi, T., and Felix, E. (2013). The status of bioenergy development in developing countries. Glob. Food Secur. 2 (2), 104–109. doi:10.1016/j.gfs.2013.04.002
Martins, F., Felgueiras, C., and Smitková, M. (2018). Fossil fuel energy consumption in European countries. Energy Procedia 153, 107–111. doi:10.1016/j.egypro.2018.10.050
Matsuo, Y., Yanagisawa, A., and Yamashita, Y. (2013). A global energy outlook to 2035 with strategic considerations for Asia and Middle East energy supply and demand interdependencies. Energy Strategy Rev. 2 (1), 79–91. doi:10.1016/j.esr.2013.04.002
Michaelowa, A., Shishlov, I., and Brescia, D. (2019). Evolution of international carbon markets: lessons for the Paris Agreement. Wiley Interdiscip. Rev. Clim. Change 10 (6), e613. doi:10.1002/wcc.613
Michoud, B., and Hafner, M. (2021). Financing clean energy access in sub-saharan Africa: risk mitigation strategies and innovative financing structures. Springer Nature, 197.
Mohammed, Y. S., Mustafa, M. W., Bashir, N., Ogundola, M. A., and Umar, U. (2014). Sustainable potential of bioenergy resources for distributed power generation development in Nigeria. Renew. Sustain. Energy Rev. 34, 361–370. doi:10.1016/j.rser.2014.03.018
Naqvi, S. R., Jamshaid, S., Naqvi, M., Farooq, W., Niazi, M. B. K., Aman, Z., et al. (2018). Potential of biomass for bioenergy in Pakistan based on present case and future perspectives. Renew. Sustain. Energy Rev. 81, 1247–1258. doi:10.1016/j.rser.2017.08.012
Narayan, S., and Doytch, N. (2017). An investigation of renewable and non-renewable energy consumption and economic growth nexus using industrial and residential energy consumption. Energy Econ. 68, 160–176. doi:10.1016/j.eneco.2017.09.005
Nejat, P., Jomehzadeh, F., Taheri, M. M., Gohari, M., and Majid, M. Z. A. (2015). A global review of energy consumption, CO2 emissions and policy in the residential sector (with an overview of the top ten CO2 emitting countries). Renew. Sustain. energy Rev. 43, 843–862. doi:10.1016/j.rser.2014.11.066
Nyika, J., Adesoji Adediran, A., Olayanju, A., Seun Adesina, O., and Odikpo Edoziuno, F. (2021). The potential of biomass in Africa and the debate on its carbon neutrality. London, United Kingdom: IntechOpen. doi:10.5772/intechopen.93615
Obaideen, K., AlMallahi, M. N., Alami, A. H., Ramadan, M., Abdelkareem, M. A., Shehata, N., et al. (2021). On the contribution of solar energy to sustainable developments goals: case study on Mohammed bin Rashid Al Maktoum Solar Park. Int. J. Thermofluids 12, 100123. doi:10.1016/j.ijft.2021.100123
Ohunakin, O. S., Matthew, O. J., Adaramola, M. S., Atiba, O. E., Adelekan, D. S., Aluko, O. O., et al. (2023). Techno-economic assessment of offshore wind energy potential at selected sites in the Gulf of Guinea. Energy Convers. Manag. 288, 117110. doi:10.1016/j.enconman.2023.117110
Olabi, A. G., Obaideen, K., Elsaid, K., Wilberforce, T., Sayed, E. T., Maghrabie, H. M., et al. (2022). Assessment of the pre-combustion carbon capture contribution into sustainable development goals SDGs using novel indicators. Renew. Sustain. Energy Rev. 153, 111710. doi:10.1016/j.rser.2021.111710
Østergaard, P. A., Duic, N., Noorollahi, Y., Mikulcic, H., and Kalogirou, S. (2020). Sustainable development using renewable energy technology. Renew. Energy 146, 2430–2437. doi:10.1016/j.renene.2019.08.094
Ou, S., Hao, X., Lin, Z., Wang, H., Bouchard, J., He, X., et al. (2019). Light-duty plug-in electric vehicles in China: an overview on the market and its comparisons to the United States. Renew. Sustain. Energy Rev. 112, 747–761. doi:10.1016/j.rser.2019.06.021
Owen, M., van der Plas, R., and Sepp, S. (2013). Can there be energy policy in Sub-Saharan Africa without biomass? Energy Sustain. Dev. 17 (2), 146–152. doi:10.1016/j.esd.2012.10.005
Oyedepo, S. O. (2012). On energy for sustainable development in Nigeria. Renew. Sustain. energy Rev. 16 (5), 2583–2598. doi:10.1016/j.rser.2012.02.010
Oyedepo, S. O., Dunmade, I. S., Adekeye, T., Attabo, A. A., Olawole, O. C., Babalola, P. O., et al. (2019). Bioenergy technology development in Nigeria - pathway to sustainable energy development. Int. J. Environ. Sustain. Dev. 18 (2), 175–205. doi:10.1504/ijesd.2019.10020922
Oyedepo, S. O., and Fakeye, A. B. (2023). “Waste heat recovery technologies for sustainability and economic growth in developing countries,” in Enabling methodologies for renewable and sustainable energy (Howick Place, London: CRC Press), 213–229.
Panos, E., Turton, H., Densing, M., and Volkart, K. (2015). Powering the growth of sub-saharan Africa: the jazz and symphony scenarios of world energy Council. Energy Sustain. Dev. 26, 14–33. doi:10.1016/j.esd.2015.01.004
Pellegrini, P., and Fernández, R. J. (2018). Crop intensification, land use, and on-farm energy-use efficiency during the worldwide spread of the green revolution. Proc. Natl. Acad. Sci. 115 (10), 2335–2340. doi:10.1073/pnas.1717072115
Petinrin, J. O., and Shaaban, M. (2015). Renewable energy for continuous energy sustainability in Malaysia. Renew. Sustain. Energy Rev. 50, 967–981. doi:10.1016/j.rser.2015.04.146
Pousa, G. P. A. G., Santos, A. L. F., and Suarez, P. A. Z. (2007). Viewpoint: history and policy of biodiesel in Brazil. Energy Policy 35, 5393–5398. doi:10.1016/j.enpol.2007.05.010
Pulighe, G., Bonati, G., Colangeli, M., Morese, M. M., Traverso, L., Lupia, F., et al. (2019). Ongoing and emerging issues for sustainable bioenergy production on marginal lands in the Mediterranean regions. Renew. Sustain. Energy Rev. 103, 58–70. doi:10.1016/j.rser.2018.12.043
Rather, R. A., Wani, A. W., Mumtaz, S., Padder, S. A., Khan, A. H., Almohana, A. I., et al. (2022). Bioenergy: a foundation to environmental sustainability in a changing global climate scenario. J. King Saud University-Science 34 (1), 101734. doi:10.1016/j.jksus.2021.101734
Ravindranath, D., and Rao, S. S. N. (2011). “Bioenergy in India: barriers and policy opfions,” in Diffusion of renewable energy technologies (Vol. 129), New Delhi, India.
Reid, W. V., Ali, M. K., and Field, C. B. (2020). The future of bioenergy. Glob. change Biol. 26 (1), 274–286. doi:10.1111/gcb.14883
Reuter, M., Patel, M. K., and Eichhammer, W. (2017). Applying ex-post index decomposition analysis to primary energy consumption for evaluating progress towards European energy efficiency targets. Energy Effic. 10 (6), 1381–1400. doi:10.1007/s12053-017-9527-2
Rose, S. K., Kriegler, E., Bibas, R., Calvin, K., Popp, A., van Vuuren, D. P., et al. (2014). Bioenergy in energy transformation and climate management. Clim. change 123 (3), 477–493. doi:10.1007/s10584-013-0965-3
Salgado, R. M., Danzi, F., Oliveira, J. E., El-Azab, A., Camanho, P. P., and Braga, M. H. (2021). The latest trends in electric vehicles batteries. Molecules 26 (11), 3188. doi:10.3390/molecules26113188
Schiffer, H. W. (2008). WEC energy policy scenarios to 2050. Energy policy 36 (7), 2464–2470. doi:10.1016/j.enpol.2008.02.045
Shahare, V. V., Kumar, B., and Singh, P. (2017). “Biofuels for sustainable development: a global perspective,” in Green technologies and environmental sustainability. Editors R. Singh, and S. Kumar (Switzerland: Springer International Publishing AG), 67–90. ISBN 978-3-319-50654-8.
Shahid, M. K., Batool, A., Kashif, A., Nawaz, M. H., Aslam, M., Iqbal, N., et al. (2021). Biofuels and biorefineries: development, application and future perspectives emphasizing the environmental and economic aspects. J. Environ. Manag. 297, 113268. doi:10.1016/j.jenvman.2021.113268
Shahsavari, A., and Akbari, M. (2018). Potential of solar energy in developing countries for reducing energy-related emissions. Renew. Sustain. Energy Rev. 90, 275–291. doi:10.1016/j.rser.2018.03.065
Shyu, C. W. (2014). Ensuring access to electricity and minimum basic electricity needs as a goal for the post-MDG development agenda after 2015. Energy Sustain. Dev. 19, 29–38. doi:10.1016/j.esd.2013.11.005
Simionescu, M., Strielkowski, W., and Tvaronavičienė, M. (2020). Renewable energy in final energy consumption and income in the EU-28 countries. Energies 13 (9), 2280. doi:10.3390/en13092280
Singal, S. K., and Singh, R. P. (2007). Rural electrification of a remote island by renewable energy sources. Renew. energy 32 (15), 2491–2501. doi:10.1016/j.renene.2006.12.013
Souza, G. M., Ballester, M. V. R., de Brito Cruz, C. H., Chum, H., Dale, B., Dale, V. H., et al. (2017). The role of bioenergy in a climate-changing world. Environ. Dev. 23, 57–64. doi:10.1016/j.envdev.2017.02.008
Sovacool, B. K., and Monyei, C. G. (2021). Positive externalities of decarbonization: quantifying the full potential of avoided deaths and displaced carbon emissions from renewable energy and nuclear power. Environ. Sci. Technol. 55 (8), 5258–5271. doi:10.1021/acs.est.1c00140
Suzuki, M. (2015). Identifying roles of international institutions in clean energy technology innovation and diffusion in the developing countries: matching barriers with roles of the institutions. J. Clean. Prod. 98, 229–240. doi:10.1016/j.jclepro.2014.08.070
Tesfamichael, B., Montastruc, L., Negny, S., and Yimam, A. (2021). Designing and planning of Ethiopia's biomass-to-biofuel supply chain through integrated strategic-tactical optimization model considering economic dimension. Comput. Chem. Eng. 153, 107425. doi:10.1016/j.compchemeng.2021.107425
Tolliver, C., Keeley, A. R., and Managi, S. (2019). Green bonds for the Paris agreement and sustainable development goals. Environ. Res. Lett. 14 (6), 064009. doi:10.1088/1748-9326/ab1118
Tracking Clean Energy Progress (2023). Tracking clean energy progress 2023. Available at: https://www.iea.org/energy-system/renewables/bioenergy#tracking.
UNEP (2023). UNEP collaborating centre on energy and environment, riso national laboratory roskilde, Denmark. Available at: https://unfccc.int/files/cooperation_and_support/capacity_building/application/pdf/unepcdmintro.pdf (Accessed February 27, 2023).
United Nations Conference on Environment and Development (UNCED) (1992). Earth summit, took place in Rio de Janeiro, Brazil, from 3 to 14 june 1992.
Wilkinson, J., and Herrera, S. (2010). Biofuels in Brazil: debates and impacts. J. Peasant Stud. 37 (4), 749–768. doi:10.1080/03066150.2010.512457
World Energy Council (2007). Deciding the future: energy policy scenarios to 2050. London: World Energy Council.
Wu, W., Kanamori, Y., Zhang, R., Zhou, Q., Takahashi, K., and Masui, T. (2021). Implications of declining household economies of scale on electricity consumption and sustainability in China. Ecol. Econ. 184, 106981. doi:10.1016/j.ecolecon.2021.106981
Yadav, K. K., Krishnan, S., Gupta, N., Prasad, S., Amin, M. A., Cabral-Pinto, M. M., et al. (2021). Review on evaluation of renewable bioenergy potential for sustainable development: bright future in energy practice in India. ACS Sustain. Chem. Eng. 9 (48), 16007–16030. doi:10.1021/acssuschemeng.1c03114
Yang, Z., and Bandivadekar, A. (2017). Light-duty vehicle greenhouse gas and fuel economy standards. Washington: The international Council on clean transportation.
Yang, Z., Wu, Y., Zhang, Z., Li, H., Li, X., Egorov, R. I., et al. (2019). Recent advances in co-thermochemical conversions of biomass with fossil fuels focusing on the synergistic effects. Renew. Sustain. Energy Rev. 103, 384–398. doi:10.1016/j.rser.2018.12.047
Yuwan, D., and Xuemei, J. (2017). Temporal change of China's pollution terms of trade and its determinants. Ecol. Econ. 132, 31–44. doi:10.1016/j.ecolecon.2016.10.001
Zaharia, A., Diaconeasa, M. C., Brad, L., Lădaru, G.-R., and Ioanăș, C. (2019). Factors influencing energy consumption in the context of sustainable development. Sustainability 11 (15: 4147), 1–28. doi:10.3390/su1115414
Keywords: bioenergy, clean development mechanism, climate change, energy consumption, sustainable development goal
Citation: Dirisu JO, Salawu EY, Ekpe IC, Udoye NE, Falodun OE, Oyedepo SO, Ajayi OO and Kale SA (2024) Promoting the use of bioenergy in developing nations: a CDM route to sustainable development. Front. Energy Res. 11:1184348. doi: 10.3389/fenrg.2023.1184348
Received: 11 March 2023; Accepted: 28 November 2023;
Published: 08 January 2024.
Edited by:
Anna Stoppato, University of Padua, ItalyReviewed by:
Xin He, Sun Yat-sen University, ChinaAnthony Onokwai, Bells University of Technology, Nigeria
Copyright © 2024 Dirisu, Salawu, Ekpe, Udoye, Falodun, Oyedepo, Ajayi and Kale. This is an open-access article distributed under the terms of the Creative Commons Attribution License (CC BY). The use, distribution or reproduction in other forums is permitted, provided the original author(s) and the copyright owner(s) are credited and that the original publication in this journal is cited, in accordance with accepted academic practice. No use, distribution or reproduction is permitted which does not comply with these terms.
*Correspondence: Sunday O. Oyedepo, c3VuZGF5Lm95ZWRlcG9AY292ZW5hbnR1bml2ZXJzaXR5LmVkdS5uZw==; Joseph O. Dirisu, am9zZXBoLmRpcmlzdUBjb3ZlbmFudHVuaXZlcnNpdHkuZWR1Lm5n