- 1Research, Technology and Development Department, Independent Power Transmission Operator (IPTO) S.A., Athens, Greece
- 2Applied Electronics Laboratory, Department of Electrical and Computer Engineering, University of Patras, Patras, Greece
- 3ETRA Investigacion y Desarrollo (ETRA I+D) S.A., Valencia, Spain
The scalability and replicability analysis (SRA) is usually performed for Smart Grid Architecture Model (SGAM) projects to assess the applicability of proposed solutions in various regions, conditions, densities, and sizes. In this framework, the replication and scaling-up roadmap of the Horizon 2020 (H2020) CROSSBOW project encompasses a variety of interesting aspects. This study evaluates CROSSBOW results and their impact under various technical, regulatory, market, and social factors. The relevant methodology follows the literature and is properly adjusted to investigate the level at which the CROSSBOW concepts can be escalated and transferred to different scenarios and regions in an efficient and cost-effective manner. Limitations and challenges regarding scalability and replicability are also illustrated. The results depict the total impact of specific factors on scalability and replicability while useful conclusions are drawn. Lessons learnt from the CROSSBOW are very insightful for the scalability and replicability of other upcoming projects.
1 Introduction
CROSS BOrder management of variable renewable energy and storage units enabling a transnational Wholesale market (CROSSBOW) is a transmission system operator (TSO)–driven project that has recently been completed under the umbrella of Horizon 2020 (H2020) CROSSBOW (2017a). The core objective of CROSSBOW is to deploy technological solutions in the market, which enable the increasing shared use of resources by fostering transmission networks and cross-border management of variable renewable energy assets and storage units. This enables higher penetration of clean energy while reducing network operational costs and improving the economic benefits of renewable energy sources (RES) and storage units. The project demonstrates a number of different technologies offering TSO's increased grid flexibility and robustness through: 1) better control of cross-border balancing energy at interconnection points; 2) new storage solutions—distributed and centralized—offering ancillary services to operate virtual storage plants (VSPs); 3) better information communication technology (ICT), such as better network observability, enabling flexible generation, and demand response schemas; 4) the definition of a transnational wholesale market, proposing fair and sustainable remuneration for clean energies through the definition of new business models supporting the participation of new players, i.e., aggregators, and the reduction of costs (Andriopoulos et al., 2022; CROSSBOW, 2017a; Crossbow, 2017b). Towards this end, CROSSBOW proposed, realized, and tested nine complete solutions—products, while a completed scalability and replicability analysis (SRA) was conducted for these nine products.
Scalability is defined as the ability of a system to adjust its scale to respond to augmented volumes of demand. The idea connotes the ability of a system to accommodate an increasing number of elements or objects, manage growing volumes of work gracefully, and/or be susceptible to being enlarged. When procuring or designing a system, it is often required that it be scalable. However, the ability of a system to scale does not certainly mean that the scaled-up system operates well. In a more restrictive definition, scalability is considered the ability of a system to maintain its performance and operation and keep all its desired properties when its scale is increased without having a relative increase in the system’s complexity (Naber et al., 2017). On the other hand, replicability denotes the property of a system that allows it to be duplicated at another location or time. In that sense, it is important for a product and/or any scenario to be replicable at each operational level in order to be a product with added value (Sigrist et al. 2016; van Summeren et al., 2022).
Scalability and replicability analysis is the preliminary requirement to perform a scaling-up and replication successfully; therefore, scalability and replicability foster or at least decrease barriers towards the growth, communication, and reuse of the results of research and development (R&D) projects (ENTSO-E, 2020). This is of great significance for companies and stakeholders given that scaling-up and replication offer major advantages, for example, by reusing proven solutions in a cost-effective way and/or for a different or larger group of customers. Therefore, an SRA is usually a prerequisite for the successful completion of an R&D project (Menci et al., 2021b; Menci et al., 2021a; Potenciano Menci et al., 2020).
According to the BRIDGE initiative—coordinating energy research and innovation projects across the EU—the steps to conduct an SRA of a Smart Grid Architecture Model (SGAM) project can be separated into four stages, with each of them involving several steps (Andriopoulos et al., 2022; Gottschalk et al., 2017; Crossbow, 2017b). The most complex stages correspond to the definition of the SRA methodology and to actually carrying out the SRA. The four stages are
1. Definition of the scope of the SRA: firstly, the SGAM layers and, within each SGAM layer, the SRA dimension or dimensions that will be assessed must be selected.
2. Definition methodology for each SRA dimension selected: the second stage requires defining the methodological approach specifically for each previously selected dimension.
3. Performing the SRA for each dimension selected: once the methodology has been defined, SRA developers have to collect the required input data and perform the corresponding qualitative/quantitative analyses for the scenarios previously defined.
4. Drawing conclusions and delivering the SRA rules/roadmap: the last stage includes the analysis of the results derived from the SRA, firstly per each dimension separately and then trying to relate among them the results for the different dimensions when relevant. This analysis should allow inferring a set of SRA rules, which may be defined as conclusions on the most significant aspects affecting the scalability and replicability of the technological solutions examined.
Towards implementing the BRIDGE methodology, a simplified SRA is adopted without the loss of generality. A scalable project is considered a project that favourably augments its size under the same boundary conditions. By contrast, a replicable project is a project that successfully performs under various boundary conditions. For this purpose, the first question is “what makes a particular project scalable and replicable?” (Sigrist et al., 2016; van Summeren et al., 2022).
Factors that influence and condition CROSSBOW’s scalability and replicability are gathered and identified, according to the relevant literature (Sigrist et al., 2016; May et al., 2015). These factors include technical, economic, regulatory, and stakeholder acceptance aspects. Under this perspective, smart grid projects address a wide area of research and innovation challenges, and the factors have to be adequately general and cannot be too application specific. In this work, we identify the main categories of factors as follows (Sigrist et al., 2016):
• Technical factors establish if the solution of a particular project is inherently scalable and/or replicable, i.e., whether it is possible to scale up and/or replicate.
• Economic factors demonstrate if it is viable to pursue scaling up or replication. This critical point—checking the validity of the investment analysis (e.g., internal rate of return, net present value, etc.) and the business model’s hold on a bigger scale or in a different framework—is often omitted and becomes a major barrier.
• Factors linked to regulation and acceptance of stakeholders such as end users, regulators, and authorities that determine the extent to which the current regulatory and social framework is ready to receive and accept a scaled-up version of the project or if a new environment is appropriate for the project.
In this study, a complete SRA for the CROSSBOW project is presented as a case study (Gottschalk et al., 2017; Aberdeen 2013). The significance of the project lies in the necessity of regional coordination in terms of energy scheduling. In this context, CROSSBOW has addressed several challenging problems aiming at efficient cross-border coordination. The project has developed an arsenal of tools and tested them under real-world conditions to verify the efficiency of the solutions proposed. Moving a step forward, CROSSBOW has adopted a framework to assess the scalability and replicability of its solutions. This step is of paramount importance, especially under the current energy crisis in Europe since the tools can be easily adapted to address the risk of high energy prices and, eventually, the energy supply. The relevant SRA methodology, which is retrieved by literature review for SGAM projects and adjusted in a suitable manner, is presented in detail and is suitable for large-scale smart grid projects (Sigrist et al., 2016; Rodriguez-Calvo et al., 2018; Menci et al., 2021b). In particular, a variety of factors concerning the scalability and replicability of the CROSSBOW products are recorded. To facilitate the reader, we define several diverse factors that cover all the aspects of a product’s applicability, such as economic, technological, and regulatory Various aspects are covered by extrapolating the project results and evaluating the impact under different conditions. The outcome of the analysis is that CROSSBOW products are highly scalable and replicable and can address the emerging challenges of the energy sector. Apparently, there are factors that show a lower score, especially in the regulatory domain.
The rest of the article is organized as follows: in Section 2, we give the general concept behind CROSSBOW solutions and a short description of every CROSSBOW product. In Section 3, the methodology for scalability and replicability factors’ identification and quantification is illustrated in detail. In Section 4, the SRA is conducted per CROSSBOW product, and in Section 5, the limitations and challenges regarding the scalability and replicability factors are discussed, and some of the lessons learnt are presented. Finally, some useful conclusions are drawn in Section 6.
2 CROSSBOW general concept: Short description of products
CROSSBOW has developed nine products, namely, Regional Operation Centre-Balancing Cockpit (ROC-BC), RES Regional Coordination Centre (RES-CC), RES Regional Dispatchable Unit (RES-DU), Regional Storage Coordination Centre (STO-CC), virtual storage plant (VSP), demand side management integration platform (DSM-IP), Wide Area Monitoring and Awareness System (WAMAS), Cooperative Ownership of Flexibility Assets Platform (CFP), and Wholesale and Ancillary Market toolset (AM) (Crossbow, 2017b). In Figure 1, the CROSSBOW products’ integration and interoperability within the project’s ecosystem are depicted in blue. As is evident, many of the CROSSBOW products are strictly related to each other, while WAMAS acts as the interface between ROC-BC and other products regarding regional management and cooperation. In addition, the link between the CROSSBOW ecosystem and external assets such as RES plants, storage assets, supervisory control, and data acquisition (SCADA) systems is apparent in the same figure, depicted in green (Crossbow, 2017b).
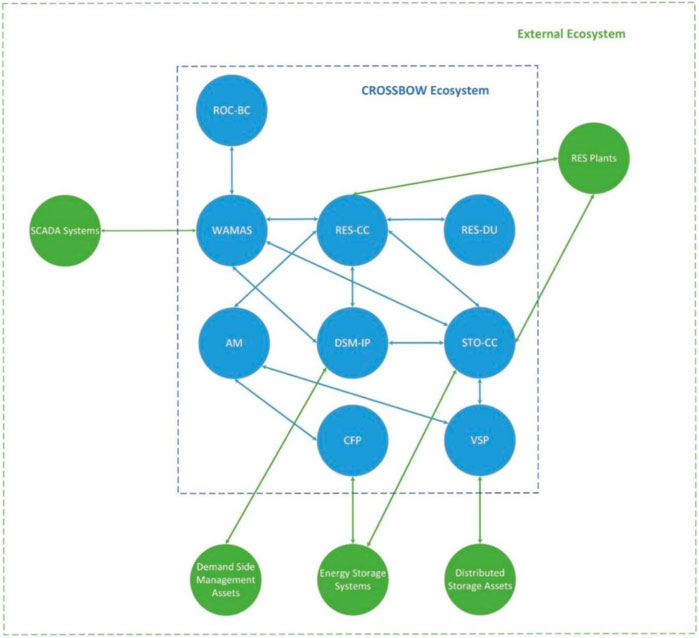
FIGURE 1. Diagram on internal CROSSBOW products integration and interoperability and external ones with other systems.
2.1 CROSSBOW Regional Operation Centre Balancing Cockpit
The ROC-BC was designed for coordinating the cost-efficient and secure operation of the South Eastern European (SEE) transmission network and enabling the shared use and cross-border management of various resources, such as renewable generators, centralized/distributed storage, and demand response resources. The services of the ROC-BC involve the ones already provided by established Regional Security Coordinators (RSCs), such as the Security Coordination Centre (SCC) and the newly established Southeast Electricity Network Coordination Centre (SELENE) in the SEE region, which includes the improved Individual Grid Model (IGM)/Common Grid Model (CGM) delivery, Short Term Regional Adequacy Assessment (RAA) forecasts, coordinated security analysis, coordinated capacity calculation, and outage planning coordination, but with improved supporting calculation and optimization methodologies. Furthermore, it also involves new services associated with short-term operation tasks, such as imbalance management, congestion management, and probabilistic sizing of reserves, through the enhanced exchange of data and information between the national control centres close to real-time.
2.2 CROSSBOW RES Regional Coordination Centre
RES-CC is a holistic solution for the centralized management of renewable energy generation plants spread across a wide region. It offers new services to the market, providing new automated RES control capabilities (together with smart management procedures to TSOs, supporting them in the management of large portfolios of RES while keeping the electrical system’s security) and new services to generators/RES managers/RES producers to optimize the use of assets and, at the same time, automatically to compute what units among their pool of generation resources will be subjected to run countermeasures for generation-related requests sent by the TSOs. In particular, this product enables RES operators to cut their operational costs through the joint management of renewable energy generation plants, reduce the time and resources required to maintain and operate these facilities, and improve the overall productivity of the regional electricity network due to continuous monitoring. For the system operators, RES-CC offers a novel tool for advanced decision-making, monitoring, and maintenance.
2.3 CROSSBOW RES Regional Dispatchable Units
RES-DU product offers new services to the market, providing improved power plant management options to the generator facilities and new functionalities to the system operators, among other power system actors. These new services are based on weather and market data analytics, considering the system operator requirements and allowing the management and design of future hybrid power plants (HPPs, which are RES and storage units connected to a common coupling point) according to the optimal criteria set by RES-DU product. The main functionalities are ancillary services provision from the HPPs, increased revenues for the HPPs by adapting the energy/power supply to the best market prices of electricity sale when possible and applying different sale energy strategies like energy time shifting, provision of services from the storage assets to ensure continuous power supply from the HPPs and design new HPPs according to the requirements of each system operator and the corresponding national regulatory framework.
2.4 CROSSBOW Regional Storage Coordination Centre
The CROSSBOW STO-CC coordinates storage units at the national and regional level in order to improve the stability of the system, enabling the provision of multiple services, such as efficient RES integration, frequency restoration, voltage regulation, and cross-border power transfer.
2.5 CROSSBOW virtual storage plants
The VSP aggregates small-capacity storage units and lowers the market barriers for storage owners since small-scale assets are not eligible to participate in the ancillary service markets. The VSP aggregates the storage units of different capacities and different types, which can be operated equivalently as a large, centralized storage plant. The considered services include frequency regulation, voltage regulation, and congestion management.
2.6 CROSSBOW wide area monitoring and awareness system
The WAMAS provides real-time data exchange between TSOs, distribution system operators (DSOs), RESs, and storage devices and provides information about storage availability, congestion, and warnings. It can also perform control actions to maintain the stable operation of the power system. The goal is to ensure stable power system operation with integration or RES and storage in dynamic market conditions. The WAMAS addresses the impact of market actions and RES on the power system stability and dynamics.
2.7 CROSSBOW regional DSM integration platform
The DSM-IP product offers a solution for the centralized and efficient transnational use of demand-side management (DSM). This is achieved by communication interfaces that monitor and control dispatchable (flexible) loads, advanced algorithms that integrate DSM, interfaces with other Regional Control Centres (RES-CC, STO-CC, and ROC), interfaces with the TSO-DSO coordination platform, and applications for business and market actors. DSM-IP allows the cost-effective coordination of multiple DSM assets, continuous monitoring and management of load, voltage, and frequency profiles, and enhances decision-making support through data analytics and monitoring.
2.8 CROSSBOW wholesale and ancillary market toolset
The AM product facilitates the participation of variable renewable energy sources, storage, and new actors in electricity markets, in order to adequately remunerate new services and reduce the overall operation cost. New services and approaches are based on the harmonized set of rules that enable market participants from countries in a region to participate in common wholesale and ancillary services cross-border market platforms. The AM product offers new services to the market, providing participation to balance service providers (BSPs) and TSOs in the regional balancing market. In this context, cross-border trading is conducted on two independent markets, namely, manual frequency restoration reserves (mFRR) and intraday energy market platform.
2.9 Cooperative ownership of flexibility assets platform
The CFP is an ICT tool designed for flexibility providers like RES, prosumers, consumers, and energy communities to organize themselves into energy communities or cooperatives. The CFP is an extension tool, complementing existing flexibility aggregation technologies (e.g., VPP and DSM), which provide monitoring, characterization, aggregation, activation, and monetization of different flexibilities (loads, generation, and storage). It comprises processes for the establishment and management of energy communities, such as opening user accounts, defining business objectives, rules and bylaws, membership management with shares and profit sharing, democratic governance with voting procedures, and accounting of flexibility monetization.
3 Description of methodology
3.1 Identification and classification of factors
The scalability and replicability factors are classified into four main categories and subcategories, which are presented in Table 1 and explained in more detail in the next subsections (Sigrist et al., 2016; May et al., 2015).
3.1.1 Identification of scalability factors
With regard to the technical area, five factors have been identified. Especially in technology, the modularity is the basic prerequisite for scaling up. It refers to whether a solution can be separated into interdependent modules/elements. This factor then investigates to what extent a solution is modular (e.g., how easy it is to append new elements or if there are limitations on appending components). In addition, technology evolution examines and demonstrates to what extent technological aspects allow enlarging the solution in size. Regarding the control and communications interface, the interface design copes with a number of interactions between the solution elements. The interface design then studies to what extent the interactions among the components are controlled in a centralized or decentralized way. Besides the complexity of the solution itself, the software tools utilized for the deployment (e.g., simulation models, databases, etc.) have to address the augmented size. This factor investigates and answers to what extent the performance of the software tools is influenced if the size of the solution increases. In terms of infrastructure, compatibility analysis refers to the compatibility of the technical framework where the solution will be established and the interactions between the elements of the solution and the outside of the project ecosystem. Regarding the economic field, two factors are identified. The economies of scale explore and determine to what extent the cost increases when expanding a solution. In addition, profitability defines to what extent profits grow when extending a solution. Regarding the regulatory and stakeholder, two factors are added. Regulation poses the framework for the generation, transmission, distribution, and supply procedures, defining how the different participants work and interact among themselves. Referring to scalability, regulation is interpreted via its impact on the size and scope of the project. Acceptance refers to the extent to which stakeholders like regulators, policymakers, and end users are ready to embrace and accept an augmented project.
3.1.2 Identification of replicability factors
Regarding the technical area, two factors are identified. Especially in the technology, and control and communications interface, the standardization factor examines to what extent the solution is standard compliant and/or whether the solution can be easily made standard compliant. The factor interoperability studies and demonstrates to what extent solutions and their elements/functions are interoperable or even plug-and-play. Network configuration explores to what extent the solution relies on given resources and infrastructures. In terms of the economic field, three factors are added. The factor business model examines and answers to what extent the viability of the solution has been analysed and/or if the solution is viable under various conditions [e.g., another European Union (EU) member state]. In addition, profitability analysis determines to what extent the solution relies on given macroeconomic factors (e.g., discount rate, inflation rate, etc.). In addition, market design asks and answers to what extent the solution relies on a given market design. Regarding the regulatory and stakeholder, two factors are identified. Regulation examines to what extent the solution relies on current national or local regulation in order to be possible and viable and/or if relevant limitations and barriers emerge. The acceptance factor refers to the acceptance of the solution by the main stakeholders, asking and examining to what extent acceptance issues are anticipated in the case of exporting the solutions to other countries.
3.2 Quantification of scalability and replicability factors per CROSSBOW product
To quantify the impact of the scalability and replicability factors, we introduce a scale ranging from 0 to 3 to better understand how scalable or replicable each solution is. If the factor is not useful or is irrelevant to the product, then it is graded 0, meaning that this factor has no impact on this product’s scalability/replicability. If the scalability or/and replicability of a specific product is based on future technological advancements or knowledge, then the factor is 1. Furthermore, if the product shows low scalability/replicability due to this factor, then it is graded 1; otherwise, if the product has moderate scalability/replicability due to this factor, then it is graded 2, and finally, the grade is 3 if the product is of great scalability/replicability due to this factor. To illustrate the scalability and applicability of each product, we created spider diagrams using input from the relevant product leaders. By utilizing this diagrammatic approach, we provide the reader with a comprehensive and detailed understanding of each product’s scalability and replicability, highlighting all the critical features that shape these characteristics. The use of spider diagrams facilitates the reader’s comprehension and offers a clear and concise view of each product’s unique qualities.
4 Scalability and applicability analysis per CROSSBOW product
The quantification values per scalability and replicability factors and per CROSSBOW product are illustrated in Figures 2–10, as they are assessed by each CROSSBOW product leader, while a qualitative analysis per product is also given in the next subsections (Crossbow, 2017b).
4.1 Scalability and replicability assessments for ROC-BC
In the scalability assessment of the ROC-BC product, the highest score refers to modularity since ROC-BC has been shaped to offer a wide spectrum of diverse functionalities, inherently entailing modularity in order to be easily scalable. Profitability and regulatory issues show the lowest score since the budget of the RSCs is regulated by their shareholders (TSOs) according to the offered services to the latter or bilateral agreements with other TSOs, while a variety of regulatory gaps may hinder the quick adoption in power systems of a wider scale. The highest scoring replicability factor is achieved by the interoperability of the control and communication infrastructure and channels of ROC-BC. The latter is performed through common protocols used in the industrial sector. The lowest score factor refers to the macroeconomic factors and the business model. That is because heterogeneous market frameworks possess the biggest barrier when targeting to replicate solutions in regions with different market rules, while business models for RSCs are subject to the special needs and contracts among the different shareholders (TSOs) participating in the RSCs. By rating the several factors, the overall graphs are formed, as depicted in Figure 2.
4.2 Scalability and replicability assessments for RES-CC
Starting with scalability and replicability, specifically with the technical factors, modularity is a very important factor for RES-CC since it is engineered in a way in which each different functionality/module is performed by different modules, enabling an extension of the functionalities and interaction. Moving to the economic factors, regarding economies of scale, RES-CC provides additional services to SEE region’s TSOs, such as cross-border power exchanges and regional ancillary services. A wide deployment across the countries will allow higher benefits (as more clients will pay for the tool) with proportionally lower costs of deployment and operation. Regarding regulatory issues, for the scalability of RES-CC to a unified system, cross-border regulations should be compatible with enhanced profitability for them. In terms of acceptance, an effort has been made to make the product attractive and easy to use for the RES owners such that their adoption is not only acceptable but also attractive. For the replicability analysis, we begin with the technical factors, in particular with the interoperability one. The compatibility with other tools or assets for information exchange is based on real-time data, using common, standardized, and open models and protocols. In terms of standardization, RES-CC works with network standards that enable quick replicability of the solution. The RES-CC business model, targeting system operators, is developed considering a regional market in the SEE region. The bidirectional impact of macroeconomic factors (such as GDP, welfare, employment, or trade) on RES large-scale deployment and vice versa is the key for the profitability of RES-CC. Finally, in terms of the regulatory issues, the adaptation to one or the other grid code is not a blocking issue in the replication of the solution. Regarding product acceptance, products’ goals are in accordance with societal objectives since RES-CC focuses on maximizing the penetration of renewable sources while ensuring their efficient integration in the power mix. The scalability and replicability assessments form the overall graphs as depicted in Figure 3.
4.3 Scalability and replicability assessments for RES-DU
In terms of scalability assessment, RES-DU comprises several modules. It can be configured according to the HPP requirements and design, hence modularity is a very important factor. Technology evolution plays a significant role since all involved technologies have a wide range of parameters that can be modified according to the technology evolution. The interface design and software tools can be easily adapted to new HPPs. Regarding economic factors, economies of scale have a low impact on the product. Moreover, in terms of profitability, HPPs with higher capacity can obtain higher benefits and, consequently, pay higher prices for this product. Regarding regulations, specific permits may be required depending on the country where the HPPs would be deployed. However, the tool shows high acceptance since it is focused on maximizing renewable penetration and its maximization in the power mix. To proceed with the replicability assessment, both in terms of standardization and interoperability at the technology level, the high standardization level facilitates the replicability of the product. The business model is very replicable regardless of the HPP configuration or the country where the product is deployed. Regarding the macroeconomic factors, the product is replicable, but wholesale energy prices are different depending on the country under study. Furthermore, there is a high dependence on both the market design and regulatory issues, which are based on the existing conditions in each country. Finally, the acceptance rate is high since the tool is focused on maximizing renewable penetration and its efficient integration in the power mix. By rating the several factors, the overall graphs are formed, as depicted in Figure 4 for the scalability and replicability assessments.
4.4 Scalability and replicability assessments for STO-CC
The STO-CC’s scalability modularity and software integration tools are of a high level. STO-CC deployment is based on software tools such as MATLAB and PowerFactory DIgSILENT, therefore it can be modelled with good precision. Regarding the compatibility factor, STO-CC requires communication with other assets, especially WAMAS for data sharing and exchange. Moving to economic factors, scaling the exact rate of benefits is not easy at this stage due to some marketing barriers relating to energy trading and required variables which should be used in the product. In terms of regulation, the product requires complementary technical and non-technical regulations to provide the highest benefits. Finally, the acceptance rate is high since STO-CC increases renewables’ penetration with respect to energy availability of RES. Therefore, it can provide benefits for RES owners and TSOs. To proceed with the replicability assessment, the product can be considered replicable once additional technical and non-technical regulations are issued in the Pan-European transmission network. In terms of control and communication, STO-CC can be developed in any power network collaborating with different assets, and therefore it shows high interoperability. For the economic factors, although complicated, the business model, macro-economic factors, and market design of STO-CC might possibly be interoperable. In terms of regulation, STO-CC is not replicable at this stage. There is a lack of a unified legal framework, a fact that delays investments in storage systems. Regarding acceptance, STO-CC increases the penetration of renewables with respect to the energy availability of RES, and users are most likely to accept the product. By rating the several factors, the overall graphs are formed, as depicted in Figure 5 for the scalability and replicability assessments.
4.5 Scalability and replicability assessments for VSP
In terms of scalability, modularity and compatibility are of a very high level since VSP is developed mainly by widely used software tools such as MATLAB and PowerFactory DIgSILENT. For the interface design, the VSP requires communication and interactions between the participant agents. Hence, the product has a solid interface design. Regarding the economies of scale, since the VSP uses a distributed control framework, the cost of adding new components to the system is low. In terms of profitability, the VSP gets contractual rewards from the TSOs or DSOs. Moving to the regulatory issues, the current regulatory framework is rather limited for storage action in the energy market, especially for the aggregated small units. Finally, a unified acceptance of them is important to perform a fully distributed control, but the trend of enabling a decentralized power system providing ancillary services to the grid is clear. Regarding the replicability assessment, the product is highly interoperable since it is portable when compared to the current practice of using centralized control architecture. The VSP applies distributed control algorithms, enabling each agent to be independent of the central controller. Hence, it can be viewed as a plug and play. In terms of the business model, VSP is used for aggregation and optimal coordination of the storage units. Moreover, macroeconomic factors have an impact on the VSP. The cost of a VSP includes control centres, communication infrastructure, etc. From the regulatory aspect, regulatory issues have a considerable impact on the VSP. A slight modification is possible due to the regulations in different areas. In the framework of acceptance, the VSP could provide ancillary services to TSOs and DSOs. Additionally, the owners of the storage units could receive increased monetary benefits. By rating the several factors, the overall graphs are formed, as depicted in Figure 6, for the scalability and replicability assessments.
4.6 Scalability and replicability assessments for DSM-IP
From the scalability aspect, the DSM-IP product architecture is modular enough to be highly scalable, as most of the applications and services have been generated or installed on docker containers. Moreover, the existing SCADA systems can be used, and the product is designed considering the existing infrastructure and state-of-the-art technologies, therefore no compatibility issues are expected during the actual deployment. Regarding profitability, a higher number of DSM assets will increase the size of the market, and hence, more benefits are expected for the different actors. However, the product itself does not require a large number of assets to work or bring benefits. On the other hand, there is a lot of potential for improved regulations for the market DSM services, which are currently mostly lacking in Europe. Due to the lack of established regulatory conditions for DSM market services in general, acceptance by users, local authorities, and the public is still not measurable, although it is highly influential. Regarding the replicability assessment, interactions among components would not increase the complexity of the software that provides the corresponding services since interfaces are defined in a highly standardized manner for this purpose. From an economic aspect, the proposed business models can be applied to any region/country, while different conditions, markets, and regulations will have to be studied in order to assess profitability with acceptable accuracy. Finally, regulation and acceptance are highly influential for replicability purposes. However, the product itself, from the technical point of view only, is not affected much by this factor. Figure 7 presents the scalability and replicability assessments regarding the DSM product.
4.7 Scalability and replicability assessments for WAMAS
Regarding scalability factors, WAMAS is highly modular since it is a building block that provides services to complement and assist the rest of the products in CROSSBOW. Considering technology evolution, WAMAS is still ahead of the commercial competitors in the market due to better observability of the network with low-resolution time data. In the field of interface design, interoperability also plays a major role, as WAMAS interfaces are implemented in different products using the OPC-UA and FTP protocols for communications. The software tools in WAMAS provide integration of its functionalities within other systems. In terms of regulation, the initial legal and regulatory framework concerning the operation and responsibilities of the TSOs has been improved over time. In the acceptance field, end users are involved in the definition of requirements, enhancing the acceptance of the tool. Regarding replicability assessment, WAMAS is designed to be highly interoperable, acting as a link between ROC-BC and the rest of the products in CROSSBOW (see Figure 1). Considering standardization on control and communications interface, WAMAS uses widespread standards in order to ease the deployment and implementation in replication sites. Referring to the business model factor, WAMAS addresses system operators in Europe (42 TSOs from 35 countries), thus the replicability of the solution in terms of market demand is ensured. In terms of regulation, the initial legal and regulatory framework concerning the structure, functions, and responsibilities of the TSOs has been improved. Regarding the acceptance framework, involving the end users in the definition of the system functionalities leads to a wider adoption of WAMAS from TSOs. The results of the scalability and replicability analysis for the product are depicted in Figure 8.
4.8 Scalability and replicability assessments for CFP
Regarding scalability, the CFP consists of high modularity. It is developed with a microservice architecture, meaning the platform is broken down into smaller modules, allowing scalability when the platform grows. Considering technology evolution, CFP technologies enable the automatically scalable solution when a flexible system is required. Regarding the economies of scale, when the number of energy assets connected to the CFP increases, the HW and SW should increase as well, while in terms of profitability, the profits are becoming greater since more flexibility is sold on the market. As in previous products, regulation is one of the key issues of the CFP. CFP’s integration and rules can be different for each country, thus it is hard to achieve greater scalability. In the field of acceptance, the stakeholders are already familiar with the issues of regulatory obstacles and aim to harmonize the flexibility markets for the entire EU. Regarding replicability assessment, the CFP is of a moderate level of standardization, interoperability, and network configuration since the interaction with other systems uses the standard APIs and communication protocols. Considering the macroeconomic factors, the CFP is flexible enough to connect with any type of energy asset. On the other hand, the business models should be re-evaluated if the flexibility prices are reduced drastically. In terms of regulations and acceptance, the CFP’s integration and rules can be different for every country, thus it is harder to achieve greater replicability and acceptance. The outcome of the several factors forming the graphs for the scalability and replicability assessments is depicted in Figure 9.
4.9 Scalability and replicability assessments for AM
Regarding ancillary market (AM) scalability and specifically modularity, the tool is designed in a modular way that enables extension and modification of its functionalities if necessary. Considering the interface design, AM trading platform consists of a graphical user interface that allows users to participate in the market. The economy of scale applies to this product, as adding additional market participants to the platform presents deployment costs much lower than the profit gained by new members. In terms of profitability, the revenue from the AM trading platform tool mostly results from the trading fees paid by market participants. Considering the regulatory issues, a drastic change in the current regulatory framework legislation could impact the usability and profitability of the developed tool significantly. Acceptance on a wider scale is desired for the transnational market for most of the stakeholders. Regarding replicability assessment, interoperability (on control and communications) is at a high level because the exchange of information among networks, systems, and applications is one of the core functionalities of the AM trading platform tool. Regarding the economic factors, the viability of an alternative business model is not tested. As the AM business model is also replicable in other neighbouring EU and non-EU countries, there is a potential room for wider integration. In the regulatory field, the AM trading platform tool is in line with the EU legislation, but the application of such tools is highly reliant on the adaptation of the legislation in each specific country. Considering the acceptance factor, acceptance of the solution is not included in the study. By rating the several factors, the overall graphs are formed, as depicted in Figure 10.
5 Limitation and challenges with regard to scalability and replicability factors
5.1 Identification of critical parameters affecting scalability/replicability
After the detailed SRA, some critical aspects of CROSSBOW scalability and replicability factors are given in brief. Therefore, key metrics are employed. These metrics are presented per the main category of factors: technical, economic, regulatory and acceptance, and are quantified specifically per the CROSSBOW product. In Figure 11 and Figure 12, the impact per area of the factors on the scalability and replicability of CROSSBOW products is illustrated. The impact of every factor is obtained considering the scaling methodology described in Section 3. By using this scale, we draw solid conclusions on how scalable or replicable is each solution with regard to a specific area of factors.
Figure 11 depicts the influence of technical factors on products’ scalability. As can be observed, the technical factors are of great scalability for all CROSSBOW products. The score recorded ranges in very high levels, between 10 and 15 points. The VSP and DSM seem to be the most scalable products regarding technical parameters, while ROC-BC is the least scalable one. However, the difference observed among all products is quite small since all products are designed to be highly scalable from a technical perspective. Figure 11 shows the influence of economic factors on scalability. Here the calculated score varies for the several products. Obviously, the economic factors do not apply to VSP and WAMAS scalability. Regarding WAMAS, the product itself can provide benefits when considering implementing WAMAS in power networks. Scaling the benefit is not clear for now. The VSP uses distributed control framework. The cost is low when new components are introduced into the system. The VSP will get a contractual reward from the TSOs or DSOs. The revenue will be distributed to the storage units integrated into the framework. DSM-IP is the most scalable, with a maximum score of 6 points, while ROC-BC and STO-CC are the least scalable with a score of 3 points, regarding the economic framework. Regarding the regulatory framework and relevant stakeholder’s acceptance, Figure 11 illustrates the relevant metrics. The score obtained is moderate to high for almost all the products and ranges from 2 to 5 points. Apparently, WAMAS is majorly scalable in terms of regulatory issues and acceptance, while DSM-IP is of low scalability in this area of factors.
Figure 12 shows the influence of technical factors on replicability. The technical factors are also of great replicability for all CROSSBOW products. The score recorded ranges at very high levels between 10 and 12 points. RES-CC, DSM, and WAMAS seem to be the most replicable products regarding technical parameters, while AM is the less replicable one. However, all products are designed to be highly replicable from a technical point of view. Figure 12 shows the influence of economic factors on replicability. The calculated score varies in a wider range for the various products. RES-DU is the most replicable product with the maximum score of 7 points, while some products (RES-CC, VSP, WAMAS, and AM) are of moderate replicability with a score between 5 and 6 points, and the rest (ROC-BC, STO-CC, DSM, and CFP) are of low replicability with a score of 3 points, regarding the economic framework. Regarding the regulatory framework and relevant stakeholder’s acceptance, Figure 12 depicts the relevant metrics. RES-CC, RES-DU, VSP, and WAMAS are substantially replicable in terms of regulatory issues and acceptance, while some products (ROC-BC and STO-CC) are of moderate replicability, and the rest (DSM, CFP, and AM) are of low replicability on this area of factors. The score obtained is moderate for almost all the products and ranges from 2 to 5 points.
5.2 Lessons learnt from CROSSBOW scalability and replicability analysis
In general, all CROSSBOW products have a great prospect for scale-up and replication according to the SRA that has been conducted. The total scores recorded via SRA are satisfactory for all the products, giving a positive perspective for the solutions proposed and demonstrated under the CROSSBOW umbrella. Each product shows good scalability and replicability prospect in the technical area of research. Especially, the modularity is of excellent scalability and replicability for every product, and technical evolution will not be a problem in the future. In addition, there is a high level of interoperability on each product, and the integration of software tools is possible. Table 2 and Table 3 record the average score of each scalability and replicability factor. The average score is derived from the SRA on all CROSSBOW products and the respective quantification results (see Section 4).
The main barrier is the lack of regulatory framework both at the national and regional levels, as it seems that this issue affects all products. In a way, it does not seem like an important problem as the regulations are evolving rapidly at the regional level but not so at the national level. The lack of regulation also affects some other factors such as the market design and acceptance of stakeholders, which are dependent on a complete market framework, and this is a basic requirement that aims to push stakeholders to accept and invest in these products.
In addition, the macroeconomic factors are vastly diverse and not easy to prognosticate across different regions, under the major economic disturbances in the power sector, especially during the current COVID-19 pandemic and energy crises. The conclusion of the analysis for the CROSSBOW products is positive. More specifically, the technical evaluation shows that the products are scalable and replicable. Nevertheless, the regulatory and economic areas of research have to be investigated more in the next projects to identify more precisely the barriers and recommendations.
6 Conclusion
In this study, the SRA methodology for smart grid projects was retrieved from the literature. The H2020 CROSSBOW project was investigated as a case study. Hence, its final products were assessed regarding replication and scaling-up aspects under different technical, regulation, market, and social factors. A completed SRA is conducted on the CROSSBOW project. As a result, technical factors are of high scalability and replicability for all CROSSBOW products. Regarding the economic framework, some CROSSBOW products are moderately scalable and replicable, while the rest are of low-level scalability and replicability. On the other hand, the regulatory and acceptance factors are of low scalability and replicability up to now. It should be noticed that the differences observed in the scores recorded on all areas of factors are perfectly normal both on the scalability and replicability properties. Even though in a smart grid project, such as the CROSSBOW, the solutions provided are of high technology readiness level (TRL), some concerns about acceptance from the relevant stakeholders arise from the uncertainty of the regulatory framework that is not stable and unified across the SEE region and all over Europe. Such an immature regulatory landscape leads to some solutions/product uncertainty from an economic point of view, too. In general terms, we conclude that the SRA has a positive outcome for all CROSSBOW products with expected variations in the four areas of the relevant factors.
Data availability statement
The data sets presented in this article are not readily available because of a non-disclosure agreement among project members. Requests to access the data sets should be directed to d.makrygiorgou@admie.gr.
Author contributions
All authors listed have made a substantial, direct, and intellectual contribution to the work and approved it for publication.
Funding
This research was supported by the EU H2020 project CROSSBOW (Grant Agreement no. 773430).
Acknowledgments
The authors would like to thank all the CROSSBOW product leaders, namely, ETRA Investigacion y Desarrollo S.A. (ETRA), Institute of Communication and Computer Systems (ICCS), the University of Manchester (UNIMAN), University of Ljubljana (UL), ELPROS electronski in programski sistemi (ELPROS), COBRA Instalaciones y Servicios S.A. (COBRA), and CyberGRID GmbH (CGRID), for providing all the necessary inputs to conduct the abovementioned SRA analysis per CROSSBOW product.
Conflict of interest
Authors EC and MM were employed by the company ETRA Investigacion y Desarrollo (ETRA I+D) S.A.
The remaining authors declare that the research was conducted in the absence of any commercial or financial relationships that could be construed as a potential conflict of interest.
Publisher’s note
All claims expressed in this article are solely those of the authors and do not necessarily represent those of their affiliated organizations, or those of the publisher, editors, and reviewers. Any product that may be evaluated in this article, or claim that may be made by its manufacturer, is not guaranteed or endorsed by the publisher.
References
Aberdeen, T. (2013). Yin, R. K. (2009). Case study research: Design and methods (4th ed.). Thousand oaks, CA: Sage. Can. J. Action Res. 14, 69–71. doi:10.33524/cjar.v14i1.73
Andriopoulos, N., Georgantas, I., Makrygiorgou, D. I., Skipis, D., Dikaiakos, C., Moraitis, I., et al. (2022). Coordinating capacity calculation via electricity market coupling: Insights from the h2020 crossbow project. Electricity 3, 182–201. doi:10.3390/electricity3020011
CROSSBOW (2017a). Crossbow – CROSS BOrder management of variable renewable energies and storage units enabling a transnational wholesale market accessed: 2022-12-30.
Gottschalk, M., Uslar, M., Delfs, C., Gottschalk, M., Uslar, M., and Delfs, C. (2017). The smart grid architecture model–sgam. The use case and smart grid architecture model approach: The IEC 62559-2 use case template and the SGAM applied in various domains, 41–61.
May, K., Vingerhoets, P., and Sigrist, L. (2015). “Barriers regarding scalability and replicability of smart grid projects,” in 2015 12th international conference on the European energy market (EEM) (IEEE), 1–5.
Menci, S. P., Herndler, B., Kupzog, F., Zweistra, M., Steegh, R., and Willems, M. (2021a). “Scalability and replicability analysis of grid management services in low voltage networks in local flexibility markets: An interflex analysis,” in 2021 IEEE madrid PowerTech (IEEE), 1–6.
Menci, S. P., Schwalbe, R., Corner, C., Herndler, B., Kahtan, J., Gutschi, C., et al. (2021b). Functional scalability and replicability analysis framework for distribution grids.
Naber, R., Raven, R., Kouw, M., and Dassen, T. (2017). Scaling up sustainable energy innovations. Energy Policy 110, 342–354. doi:10.1016/j.enpol.2017.07.056
Potenciano Menci, S., Le Baut, J., Matanza Domingo, J., López López, G., Cossent Arín, R., and Pio Silva, M. (2020). A novel methodology for the scalability analysis of ict systems for smart grids based on sgam: The integrid project approach. Energies 13, 3818. doi:10.3390/en13153818
Rodriguez-Calvo, A., Cossent, R., and Frías, P. (2018). Scalability and replicability analysis of large-scale smart grid implementations: Approaches and proposals in Europe. Renew. Sustain. Energy Rev. 93, 1–15. doi:10.1016/j.rser.2018.03.041
Sigrist, L., May, K., Morch, A., Verboven, P., Vingerhoets, P., and Rouco, L. (2016). On scalability and replicability of smart grid projects—A case study. Energies 9, 195. doi:10.3390/en9030195
Keywords: smart grid, replicability, scalability, H2020, CROSSBOW
Citation: Makrygiorgou DI, Andriopoulos N, Georgantas I, Rong J, Moraitis I, Calatayud EP and Matoses MS (2023) Scalability and replicability analysis of smart grid projects: Insights from the H2020 CROSSBOW project. Front. Energy Res. 11:1167517. doi: 10.3389/fenrg.2023.1167517
Received: 16 February 2023; Accepted: 03 April 2023;
Published: 20 April 2023.
Edited by:
Constantinos S. Psomopoulos, University of West Attica, GreeceReviewed by:
Giuditta Pisano, University of Cagliari, ItalyJay Zarnikau, The University of Texas at Austin, United States
Copyright © 2023 Makrygiorgou, Andriopoulos, Georgantas, Rong, Moraitis, Calatayud and Matoses. This is an open-access article distributed under the terms of the Creative Commons Attribution License (CC BY). The use, distribution or reproduction in other forums is permitted, provided the original author(s) and the copyright owner(s) are credited and that the original publication in this journal is cited, in accordance with accepted academic practice. No use, distribution or reproduction is permitted which does not comply with these terms.
*Correspondence: Despoina I. Makrygiorgou, d.makrygiogou@admie.gr