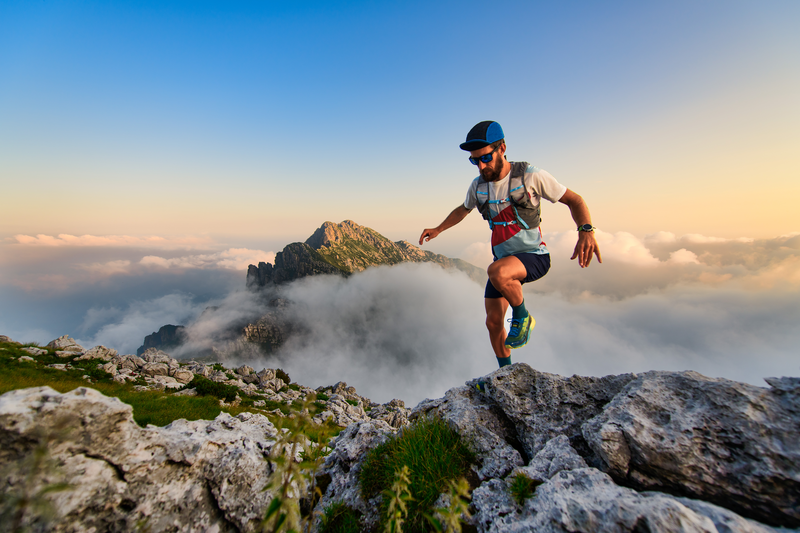
95% of researchers rate our articles as excellent or good
Learn more about the work of our research integrity team to safeguard the quality of each article we publish.
Find out more
ORIGINAL RESEARCH article
Front. Energy Res. , 14 June 2023
Sec. Solar Energy
Volume 11 - 2023 | https://doi.org/10.3389/fenrg.2023.1164892
This article is part of the Research Topic Advances in Modeling and Simulation of Wind and Solar Energy Systems for the Built Environment View all 8 articles
This study examines the problems caused by intermittent renewable energy sources, especially wind farms, and suggests a different solar energy penetration strategy to improve their loading capacity. The study uses real-time data from a wind farm in Jhampir, Pakistan, to analyse and assess various aspects of grid stations connected to wind farms. Electrical Transient Analyzer Program is used to validate the results by linking these with actual grid system. The article focuses on creating a model for a grid connected to a wind farm and the simulation of outcomes following capacity expansion, with the installation of an autotransformer. The original capacity of the wind farm was 750 MW, which was increased to 1,250 MW, i.e., 1.66 times the actual capability. Furthermore, this capacity was further enhanced to 1,540 MW, which becomes 1.23 times the previous capacity by the penetration of a photovoltaic power plant.
Electricity generation has produced significant greenhouse gas emissions since the Industrial Revolution. Recently, there has been a global push to convert to sustainable and ecologically friendly energy technologies. For the energy industry to have a sustainable future, it is essential to integrate renewable energy sources into organised environments, such as solar systems. As a result, photovoltaic systems have seen a sharp surge in their use recently (Lampropoulos et al., 2020; Laowanitwattana and Uatrongjit, 2020; Lin, 2022; Stecanella et al., 2020). Global energy demand is expected to rise by more than 30% by 2040, according to the International Energy Agency (Vainio et al., 2020). Emerging nations are now adopting integrated resource planning to increase renewable energy generation. Consequently, the need for the installation of distributed generation (DG) systems to the network is anticipated to proliferate (Elavarasan, 2019; Kumar et al., 2020; Raju et al., 2020; Noorollahi et al., 2021). The reliance on the distribution, as mentioned previously, infrastructure cost, yearly energy losses, and carbon emissions are all decreased by distributed photovoltaic power systems (Kumar et al., 2020).
The power supply system (EDS) can manage and meet the growing demand for load by integrating distributed power generation. Distributed output sources like a photovoltaic system and electric charges are combined with EDS’s energy-saving system (ESS) (Jinfeng et al., 2016; Elavarasan et al., 2019; Elavarasan et al., 2020; Madurai Elavarasan et al., 2020). There are extensive research studies on sustainable energy production and management. National state policies on energy, renewable energy sources (linked to the grid or individual), and the development of renewable energy production systems, such as small hydropower, photovoltaic energy, wind power, geothermal energy, and wave energy, are implemented in different countries (Tamoor et al., 2020; Schöniger et al., 2021). Individual microgrids should be optimised to minimise energy production costs and the environmental impact (Ostovar et al., 2021). An economical and clean method to electrify remote areas is covered by analysing and comparing extending primary grid and distribution generation.
One such option is solar radiation which is easily accessible and does not suffer from intermittent supply or availability. The other option would be an efficient energy management algorithm to ensure minimal energy usage whilst satisfying a load demand. However, with fewer resources available, renewable energy will be more expensive (Louy et al., 2017; Hamoud et al., 2021; Hoang et al., 2022; Le et al., 2022; Miao et al., 2022).
To increase efficiency, intermittent renewable energy sources can be combined. A hybrid system will use one or a combination of renewable technologies to generate and store electricity. The benefit of this system is that autonomy is guaranteed with a multi-source approach to generating electricity. Multiple research papers have documented different systems which combine various sources of solar generators, wind generators, and storage systems to create a hybrid standby system. We can apply a technique to make the most out of limited resources. One crucial aspect is ensuring that energy is used wisely and intelligent decisions are made (Louy et al., 2017; Li et al., 2022; Wang et al., 2022; Shang et al., 2023; Wang et al., 2023).
This study aims to scrutinise the penetration of solar energy in wind farms. PV penetration can considerably reduce reliance on finite and environmentally destructive fossil fuels. This integration can also assist in reducing carbon emissions, a significant source of climate change. Furthermore, combining solar and wind energy helps compensate for the intermittent nature of wind farms, as the two renewable energy sources complement each other. Finally, this integration can increase the system’s power capacity, improving efficiency and cost savings in the long run. Overall, solar energy penetration in wind farms and the national grid has the potential to revolutionise the energy landscape and pave the way for a more sustainable future.
As the increase in the load with the growth and dependency on the power in increasing the loading of the equipment such as transformers, buses, and other protective devices is raised from the permissible level, to continue the safe operation of the associated equipment and meet the future expansion and need of power, the load flow is needed. In this paper, we have added more energy from renewable sources to cater the power issues as well as maintain a good and healthy environment in developing countries like Pakistan, where economic problems are involved. Figure 1 shows the abstractable representation of this paper.
The remainder of the paper is organized as follows: Section 2 gives the literature review, including research methodology. In Section 3, the simulation models and the proposed block diagrams are discussed. Conclusion is reported in Section 4.
In the new energy power system, two technical revolutions co-occur. The optimal operating method of interaction between supply and demand groups was established for power system planning, decision-making, and safe operation (Nwaigwe et al., 2019). Efficiency, safety, economy, and environmental benefits can be maximised by a harmonious interplay between supply and demand groups, which are interwoven (Hamoud et al., 2021). A two-layer optimum scheduling model was established, so that the intelligent industrial park could participate in system operation. Scheduling approaches for source-charge coordination were examined, and the active participation of the load was implemented for the intelligent industrial park (Luty et al., 2023). Researchers studied wind farm power systems with fuzzy opportunity scheduling models to see if they could save energy, reduce pollutants, and improve overall system safety. The reliable and safe operation of a wind-integrated electrical grid is conducted using an HVDC system (Miao et al., 2022).
Due to the development in demand flexibility, the interplay between demand and the grid has been dramatically strengthened, but so has the risk during power system operations (Rokni Nakhi and Ahmadi Kamarposhti, 2020). Using probabilistic currents as a starting point for overcoming the shortcomings of the electrical grid is essential because of this unpredictability. The total electricity generation cost will be reduced by approximately a little less than one-third of the total cost of generation (Mirbarati et al., 2022). Grid performance is enhanced by the involvement of distributed generation in the national grid as shown in a case study of Saudi Arabia (Alqahtani and Patino-Echeverri, 2023). For power flow calculations, the authors combined semi-invariant and Gram–Charlier series expansions (Fu et al., 2020). This has been extensively studied (Nwaigwe et al., 2019). A stochastic configuration point technique for a probabilistic power flow algorithm was developed using uncertainty quantisation. The Monte Carlo approach was used as a reference value, and the analysis object was a wind and PV power generation system. A faulty condition related to the induction generator of a wind turbine was discussed to protect the plant (Ansari and Dyanamina, 2022). Several different potentials were tested to see how well the lower-invariant approach was computed accurately (Rebollal et al., 2021).
The bus in a power system is accompanied by the most significant phase angle of voltage, real, and reactive power. Three classes of buses are classified confidentially based on four dimensions, two of which are quantified and the remaining two can be derived from the comparison’s outcome (Al-Shetwi et al., 2020).
However, the phase angle and bus voltage magnitude can be identified, and the active and reactive power of the load bus can be defined. Only the active and reactive components of a load bus that can be allowed to fluctuate the voltage by a permissible value, such as 5%, would need to be specified, as the voltage phase angle is insignificant.
The bus’s rated actual power and rated voltage are specified. The voltage phase angle and the reactive power cohort of the bus are two critical metrics. At power generation stations, the generator bus is taken into consideration.
Identifying the voltage’s magnitude and phase angle in this bus is possible. However, the load flow resolution strongly influences the actual and reactive power. To compensate for transmission losses, the generator bus in this category underwrites the additional real and reactive power supply. For this bus is acknowledged as a swing bus (Soomar et al., 2021).
Load flow is considered obligatory for planning, operating, and developing a system that exchanges power between utility divisions, as well as economic accelerations. Load flow investigation is used in stability under transients, optimal power flow, continuity or urgency studies, and load flow analysis. As a result of this increasing demand, not only will we need to build more generating stations, but we will also need to redesign and restructure the current power grids using advanced techniques, as well as the data on analysis of load flow, which are helpful to oversee degree and angle in phase between voltages at every bus with the reactive and real power consumptions in separate transmission lines through the implementation of electrical transient software (Mirbarati et al., 2022). The power flow was analysed, and the equation was derived using a rudimentary power system. The authors used iterative techniques like Newton–Raphson, Gauss–Seidel, and fast decoupled methods to solve these equations. The fast decoupled approach is a quicker and simplified form of the Newton–Raphson method. Solving overflow equations is much more reliable and faster than the Newton–Raphson method (Li et al., 2022).
The contribution of the life cycle of hybrid renewable energy sources energised by solar and wind power is assessed in the sustainable development background for the energy systems (Piotrowska et al., 2022). It was determined that the 138/69 kV grid station’s power flow analysis and the load flow analysis results could improve a variety of performances by applying various approaches to power factor rectification that result in several technical and economic benefits. Improved voltage stability and loss of power, with minimal equipment loading and reorganisation of heavy costs, can be achieved using these strategies. They used the Electrical Transient Analysis Program to create a one-line diagram of the substation. They then analysed various aspects of the substation, including the loss of generators, a transmission line, a transformer, and a load. The optimal size and area of a capacitor bank were determined to overcome the problem of under-voltage (Wang et al., 2022). The autoregulated model of the 220/132 kV grid was developed in the study. Load flow analysis for the future expansion of the grid was successfully carried out in the paper. The simulation for various cases, such as the optimum load capacity with maximum generation, allows us to see the behaviour of the total capacity without any faults, and the system will remain intact. The loading of other generators was discussed under the total capacity. For future load addition and expansion of the grid, adding new circuits to extend its power included one transformer into the grid to increase its capacity from 750 to 1,250 MW. We suggest system reliability and save the operation and future expansion, so that more power is delivered to the user end and the cheapest power from renewable sources can be injected into this grid. Simulations confirm the effectiveness of the proposed work.
Numerous other advantages of solar generation are available besides the enormous environmental benefits. The extensive climate change impact was studied in Togo the using solar-energy-powered smart grid (Amega et al., 2022). Furthermore, carbon emission is also reduced by using the photovoltaic generation method, along with a decrement in the cost of power generation (Xu et al., 2022). However, it comes with its own set of problems regarding grid integration. In light of the benefits and constraints, utilities must incorporate solar generation into their long-term planning processes and handle the grid integration challenges through appropriate means (Ansari and Dyanamina, 2022).
• Diversification of fuels and energy sustainability.
• Cost stability due to no fuel costs: long-term price saving.
• Benefits and geographical distribution models.
• Partial correlations with reaching peak demand.
• Uncertain output that fluctuates, especially during different weather conditions.
• Partial unpredictability.
• Unable to share solar energy with networks, such as frequency support.
• It supports reactive power and system inertia.
• Obstacles crossing the sides.
• Possibility of disabling it, as it is not subjected to the law ordering program (Mahmoud et al., 2021).
The balance between demand and supply is critical to a secure and reliable grid operation in the electricity system. A detailed advancement of computing techniques is covered while integrating intelligent renewable energy systems into the smart grid (Al-Shafei et al., 2022). There is a frequency deviation if there is a mismatch. Additionally, voltage fluctuations can be caused by reactive power imbalances. Load following is required to keep the system frequency within target bands to keep the power system operation safe. Reactive power management is also critical to ensuring safe grid voltages and, thus, a reliable grid operation. Supplying imbalances in the system to keep the frequency and voltage under defined targets is often carried out by conventional plants referred to as dispatchable units. Demand-side management (DSM) may also address these imbalances. A characteristic of renewable energy sources (such as wind and solar) is that they are known as variable generators (VGs) (Rebollal et al., 2021).
Because VGs can only sometimes be generated on demand, they need to be dispatchable by design. It was previously thought that they would only participate in grid support or leave a minor influence on the grid through non-compliance because of technological limitations. However, if renewable energy sources become more prevalent, VGs, such as solar generators, will be expected to carry out more than offering grid support. In that case, they will also be expected to overcome their limits, such as compliance with fault ride-through, which could negatively affect them. Advanced inverter systems, which have recently made significant technological advances due to their advanced design, can already provide grid support capabilities, such as active power reduction, fault ride-through (FRT), and voltage support; however, these capabilities currently need to be improved. Expanded inverters are not a new form of inverters but instead require only minor software upgrades or operational parameters to enable them to advanced stages (Allouhi et al., 2019; Al-Shetwi et al., 2020). Inverters’ ability to offer uninterruptible service during disruptions is demonstrated by fault ride-through on low/high-voltage ride-through compliances. Excessive DC-link voltages, excessive AC, and loss synchronisation are common causes of inverter disconnection in the aforementioned situations. If an inverter’s solid-state device (IGBT/GTO) current rating exceeds the grid’s maximum AC, the inverter is unplugged from the grid. A voltage sag in the grid causes a rise in the current because the voltage drops on the grid side (Louy et al., 2017; Liu et al., 2020; Mahmoud et al., 2021; Reddy et al., 2021).
An over-current protection mechanism is activated, removing the inverter from the grid. The DC-link capacitor voltage increases when the power generated in the grid-connected PV plant’s DC side exceeds the capacity fuelled into the grid. As previously stated, the inverter must resist such a rise in the DC-link voltage. The plant must, therefore, be safeguarded under fault conditions because the generated power cannot be reduced.
The standard phase-locked loop (PLL) arrangement fails miserably in asymmetrical fault situations, and the inverter is unplugged from the grid. Because of their rising penetration and equal treatment with conventional generation, solid-state and photovoltaic generators have evolved with four operational grids. Various grid codes for a solar generator can be analysed in light of the global standards. Few global standards have been found to address the PV grid connectivity requirements at EHV for such inverter-based solar generation systems, as the majority of the global solar generation capacity is connected at the MV/LV (distribution) level as opposed to the EHV level (Amega et al., 2022; Xu et al., 2022).
A detailed study was conducted on the ETAP platform. A comprehensive case study was performed on the 220/132 kV Jhampir grid by developing a one-line diagram. Power flow analysis was conducted by analysing the flow of losses and the load on individual buses. Relevant data were collected from the 220/132 kV Jhampir grid. First, the addition of an autotransformer was considered to observe the feasibility. Finally, the grid was penetrated with PV power, and the results were obtained after analysing these conditions on the ETAP platform.
In this paper, we discuss three cases for the load flow analysis, which are provided in the following sections.
An optimum condition was considered to observe the Jhampir power station. Each plant operated at its highest possible capacity under normal and steady-state conditions, as depicted in Table 1. The power flow of 132 kV/220 kV is displayed in Table 2 based on the requirements shown in Table 1, while the power flow from generation to the consumer’s side is shown in Table 3.
The potential of power generation of various power plants in the wind farm of Jhampir is described in Figure 2. As shown in the figure, multiple wind power plants were installed at the wind farm of Jhampir, Pakistan. Most plant generation was rated at 50 MW, while UEP showed the highest generation in the wind farm.
In this case, adding one autotransformer was considered to enhance the grid’s capacity. The grid enhancement was supposed to deliver additional power to a small city Tando Muhammad Khan. This city is almost three-fourth of 100 km from the existing grid station and comprises two circuits. Figure 3 displays the Jhampir power station’s one-line diagram after the autotransformer’s addition. This one-line diagram consists of the entire setup of the feeder, along with its protection schemes. This single-line diagram comprises all of the plants in Figure 2.
The results showed that each power plant in the Jhampir wind farm operates at its maximum capability. It showed that additional power is drawn from the TMK circuit (depicted as TMK City I and TMK City II). The details of the power flow are shown in Table 4. The power contribution of the individual plant is displayed in Table 5. Table 6 shows the transformer loading capability, and bus loading capability is shown in Table 6.
In this case, the penetration of PV power in existing wind farms is discussed. The proposed single-line diagram is displayed inFigures 4A, B. Figure 4A contains the layout of the extension of the existing power capacity of the wind farm in Jhampir. This figure was generated using ETAP software to analyse and simulate PV penetration in the wind farm. PV power enhances the reliability of the existing Jhampir wind power station. Figure 4B provides a more precise concept of the proposed scheme, highlighting the location of PV penetration in the wind farm. The renewable mix was integrated at 132 kV and transmitted into two primary circuits rated at 220 kV.
Table 7 represents the details after adding the PV panel power station. In contrast, Table 8 describes the data of the reserves of the transformer, and the bus power flow is shown in Tables 7, 8. Figure 5 comprehensively illustrates the generation capacity of PV-penetrated mixed farms. The figure describes the detailed MW power generated as a graphical representation by the individual plant listed in Figures 4A, B. Table 9 shows the power flow at the bus after the integration of the solar in the Grid.
In this study, the potential is explored by penetrating the PV power in the existing wind farm of Jhampir, Pakistan. Conclusively, case 1 details the installed power capacity of the wind farm. In case 2, adding an autotransformer raises the installed capacity of the wind farm to 1,250 MW (1.66 times the original total). In case 3, PV penetration enhances the wind farm supply system, increasing the installed capacity to 1,540 MW (1.23 times the previous value). The results describe the power flow analysis and explore the feasibility of PV penetration of the Jhampir power station. Conclusively, fossil fuel dependency is reduced as a renewable energy mix is utilised instead. This is highly desirable for developing countries like Pakistan, as it is struggling from extreme power, economic crisis, and fuel crisis.
The original contributions presented in the study are included in the article/Supplementary Material; further inquiries can be directed to the corresponding author.
All authors listed have made a substantial, direct, and intellectual contribution to the work and approved it for publication.
All authors have helped with the completion of this paper, and we would like to express our gratitude to Power China Huadong Engineering Co., Ltd., Hangzhou, China, for their assistance in data collection, and last but not least, thanks to the Faculty of Electrical and Control Engineering, Gdańsk University of Technology, Poland, for providing online library resources.
LG was employed by Power China Huadong Engineering Co., Ltd.
The remaining authors declare that the research was conducted in the absence of any commercial or financial relationships that could be construed as a potential conflict of interest.
All claims expressed in this article are solely those of the authors and do not necessarily represent those of their affiliated organizations, or those of the publisher, the editors, and the reviewers. Any product that may be evaluated in this article, or claim that may be made by its manufacturer, is not guaranteed or endorsed by the publisher.
Al-Shafei, A., Zareipour, H., and Cao, Y. (2022). High-performance and parallel computing techniques review: Applications, challenges and potentials to support net-zero transition of future grids. Energies (Basel). 15 (22), 8668. doi:10.3390/en15228668
Al-Shetwi, A. Q., Hannan, M. A., Jern, K. P., Mansur, M., and Mahlia, T. M. I. (2020). Grid-connected renewable energy sources: Review of the recent integration requirements and control methods. J. Clean. Prod. 253, 119831. doi:10.1016/j.jclepro.2019.119831
Allouhi, A., Saadani, R., Buker, M. S., Kousksou, T., Jamil, A., and Rahmoune, M. (2019). Energetic, economic and environmental (3E) analyses and LCOE estimation of three technologies of PV grid-connected systems under different climates. Sol. Energy 178, 25–36. doi:10.1016/j.solener.2018.11.060
Alqahtani, B. J., and Patino-Echeverri, D. (2023). Identifying economic and clean strategies to provide electricity in remote rural areas: Main-grid extension vs. Distributed electricity generation. Energies (Basel) 16 (2), 958. doi:10.3390/en16020958
Amega, K., Laré, Y., Bhandari, R., Moumouni, Y., Egbendewe, A., Sawadogo, W., et al. (2022). Solar energy powered decentralized smart-grid for sustainable energy supply in low-income countries: Analysis considering climate change influences in Togo. Energies (Basel) 15 (24), 9532. doi:10.3390/en15249532
Ansari, A. A., and Dyanamina, G. (2022). Fault Ride-Through operation analysis of doubly fed induction generator-based wind energy conversion systems: A comparative review. Energies (Basel) 15 (21), 8026. doi:10.3390/en15218026
Elavarasan, R., Shafiullah, G., Manoj Kumar, N., and Padmanaban, S. (2019). A state-of-the-art review on the drive of renewables in Gujarat, state of India: Present situation, barriers and future initiatives. Energies (Basel) 13 (1), 40. doi:10.3390/en13010040
Elavarasan, R. M., Shafiullah, G. M., Padmanaban, S., Kumar, N. M., Annam, A., Vetrichelvan, A. M., et al. (2020). A comprehensive review on renewable energy development, challenges, and policies of leading Indian states with an international perspective. IEEE Access 8, 74432–74457. doi:10.1109/access.2020.2988011
Elavarasan, R. M. (2019). The motivation for renewable energy and its comparison with other energy sources: A review. Eur. J. Sustain. Dev. Res. 3 (1). doi:10.20897/ejosdr/4005
Fu, X., Guo, Q., and Sun, H. (2020). Statistical machine learning model for stochastic optimal planning of distribution networks considering a dynamic correlation and dimension reduction. IEEE Trans. Smart Grid 11 (4), 2904–2917. doi:10.1109/tsg.2020.2974021
Hamoud, G., el Nahas, I., and Faried, S. O. (2021). Reliability comparison of two deterministic criteria for planning high voltage auto-transformer stations. IEEE Trans. Power Deliv. 36 (4), 2056–2065. doi:10.1109/tpwrd.2020.3018938
Hoang, T. T., Tuan Tran, Q., Le, H. S., Nhan Nguyen, H., and Duong, M. Q. (2022). “Impacts of high solar inverter integration on performance of FLIRS function: Case study for Danang distribution network,” in Proceedings of the 2022 11th International Conference on Control, Automation and Information Sciences (ICCAIS), Hanoi, Vietnam, November 2022 (IEEE), 327–332.
Jinfeng, W., Chunxiu, Y., Fang, L., Xufei, M., Jiangbo, W., and Junhui, L. (2016). “The development and utilisation of new clean energy,” in Proceedings of the 2016 IEEE International Conference on Power and Renewable Energy (ICPRE), Shanghai, China, October 2016 (IEEE), 639–643.
Kumar, N. M., Chopra, S. S., Chand, A. A., Elavarasan, R. M., and Shafiullah, G. M. (2020). Hybrid renewable energy microgrid for a residential community: A techno-economic and environmental perspective in the context of the SDG7. Sustainability 12 (10), 3944. doi:10.3390/su12103944
Lampropoulos, I., Alskaif, T., Schram, W., Bontekoe, E., Coccato, S., and van Sark, W. (2020). Review of energy in the built environment. Smart Cities 3 (2), 248–288. doi:10.3390/smartcities3020015
Laowanitwattana, J., and Uatrongjit, S. (2020). Probabilistic power flow analysis based on arbitrary polynomial chaos expansion of bus voltage phasor. Int. Trans. Electr. Energy Syst. 30 (5). doi:10.1002/2050-7038.12315
Le, X. C., Duong, M. Q., and Le, K. H. (2022). Review of the modern maximum power tracking algorithms for permanent magnet synchronous generator of wind power conversion systems. Energies (Basel) 16 (1), 402. doi:10.3390/en16010402
Li, M., Yang, M., Yu, Y., and Lee, W. J. (2022). A wind speed correction method based on modified hidden markov model for enhancing wind power forecast. IEEE Trans. Ind. Appl. 58 (1), 656–666. doi:10.1109/tia.2021.3127145
Lin, C. H. (2022). The impact of integration of the VSC-HVDC connected offshore wind farm on torsional vibrations of steam turbine generators. Sustainability 15 (1), 197. doi:10.3390/su15010197
Liu, Z., Wang, D., Wang, J., Wang, X., and Li, H. (2020). A blockchain-enabled secure power trading mechanism for smart grid employing wireless networks. IEEE Access 8, 177745–177756. doi:10.1109/access.2020.3027192
Louy, M., Tareq, Q., Al-Jufout, S., Alsafasfeh, Q., and Wang, C. (2017). “Effect of dust on the 1-MW photovoltaic power plant at Tafila Technical University,” in Proceedings of the 2017 8th International Renewable Energy Congress (IREC), Amman, Jordan, March 2017 (IEEE), 1–4.
Luty, L., Zioło, M., Knapik, W., Bąk, I., and Kukuła, K. (2023). Energy security in light of sustainable development goals. Energies (Basel) 16 (3), 1390. doi:10.3390/en16031390
Madurai Elavarasan, R., Selvamanohar, L., Raju, K., Rajan Vijayaraghavan, R., Subburaj, R., Nurunnabi, M., et al. (2020). A holistic review of the present and future drivers of the renewable energy mix in Maharashtra, state of India. Sustainability 12 (16), 6596. doi:10.3390/su12166596
Mahmoud, A. M., Ezzat, M., Abdelaziz, A. Y., and Aleem, S. H. E. A. (2021). “A cost-benefit analysis of optimal active and reactive power compensators and voltage conditioners allocation in a real Egyptian distribution system,” in Proceedings of the 2021 22nd International Middle East Power Systems Conference (MEPCON), Assiut, Egypt, December 2021 (IEEE), 116–123.
Miao, Z., Meng, X., and Liu, L. (2022). Analyzing and optimizing the power generation performance of thermoelectric generators based on an industrial environment. J. Power Sources 541, 231699. doi:10.1016/j.jpowsour.2022.231699
Mirbarati, S. H., Heidari, N., Nikoofard, A., Danish, M. S. S., and Khosravy, M. (2022). Techno-economic-environmental energy management of a micro-grid: A mixed-integer linear programming approach. Sustainability 14 (22), 15036. doi:10.3390/su142215036
Noorollahi, Y., Kalantari, A. S., Saifoddin, A., and Yousefi, H. (2021). Distributed wind and solar power for grid sustainability and emission reduction. Environ. Prog. Sustain Energy 40 (6), 40. doi:10.1002/ep.13686
Nwaigwe, K. N., Mutabilwa, P., and Dintwa, E. (2019). An overview of solar power (PV systems) integration into electricity grids. Mater Sci. Energy Technol. 2 (3), 629–633. doi:10.1016/j.mset.2019.07.002
Ostovar, S., Esmaeili-Nezhad, A., Moeini-Aghtaie, M., and Fotuhi-Firuzabad, M. (2021). Reliability assessment of distribution system with the integration of photovoltaic and energy storage systems. Sustain. Energy, Grids Netw. 28, 100554. doi:10.1016/j.segan.2021.100554
Piotrowska, K., Piasecka, I., Kłos, Z., Marczuk, A., and Kasner, R. (2022). Assessment of the life cycle of a wind and photovoltaic power plant in the context of sustainable development of energy systems. Materials 15 (21), 7778. doi:10.3390/ma15217778
Raju, K., Madurai Elavarasan, R., and Mihet-Popa, L. (2020). An assessment of onshore and offshore wind energy potential in India using moth flame optimization. Energies (Basel) 13 (12), 3063. doi:10.3390/en13123063
Rebollal, D., Carpintero-Rentería, M., Santos-Martín, D., and Chinchilla, M. (2021). Microgrid and distributed energy resources standards and guidelines review: Grid connection and operation technical requirements. Energies (Basel) 14 (3), 523. doi:10.3390/en14030523
Reddy, G. R., Rayaguru, N. K., Karthikumar, K., Chandrasekar, P., and Murthy, P. (2021). “Enhancement of power quality with fuzzy based UPQC in grid integrated and battery assisted PV system,” in Proceedings of the 2021 2nd Global Conference for Advancement in Technology (GCAT), Bangalore, India, October 2021 (IEEE), 1–8.
Rokni Nakhi, P., and Ahmadi Kamarposhti, M. (2020). Multi objective design of type II fuzzy based power system stabilizer for power system with wind farm turbine considering uncertainty. Int. Trans. Electr. Energy Syst. 30 (4), 30. doi:10.1002/2050-7038.12285
Schöniger, F., Thonig, R., Resch, G., and Lilliestam, J. (2021). Making the sun shine at night: Comparing the cost of dispatchable concentrating solar power and photovoltaics with storage. Energy Sources, Part B Econ. Plan. Policy 16 (1), 55–74. doi:10.1080/15567249.2020.1843565
Shang, Y., Zhu, L., Qian, F., and Xie, Y. (2023). Role of green finance in renewable energy development in the tourism sector. Renew. Energy 206, 890–896. doi:10.1016/j.renene.2023.02.124
Soomar, A. M., Shaikh, A. H., and Lashari, M. H. (2021). Power flow analysis of doubly fed induction generator based 220/132kv power grid. Int. J. Electr. Eng. Technol. 12 (9). doi:10.34218/ijeet.12.9.2021.010
Stecanella, P. A. J., Vieira, D., Vasconcelos, M. V. L., and Ferreira Filho, A. D. L. (2020). Statistical analysis of photovoltaic distributed generation penetration impacts on a utility containing hundreds of feeders. IEEE Access 8, 175009–175019. doi:10.1109/access.2020.3024115
Tamoor, M., Tahir, M. S., Sagir, M., Tahir, M. B., Iqbal, S., and Nawaz, T. (2020). Design of 3 kW integrated power generation system from solar and biogas. Int. J. Hydrogen Energy 45 (23), 12711–12720. doi:10.1016/j.ijhydene.2020.02.207
Vainio, A., Pulkka, A., Paloniemi, R., Varho, V., and Tapio, P. (2020). Citizens’ sustainable, future-oriented energy behaviours in energy transition. J. Clean. Prod. 245, 118801. doi:10.1016/j.jclepro.2019.118801
Wang, P., Yu, P., Huang, L., and Zhang, Y. (2022). An integrated technical, economic, and environmental framework for evaluating the rooftop photovoltaic potential of old residential buildings. J. Environ. Manage 317, 115296. doi:10.1016/j.jenvman.2022.115296
Wang, S., Wang, B., He, S., Wang, Y., Cheng, J., and Li, Y. (2023). Enhancing the photovoltaic performance of planar heterojunction perovskite solar cells via introducing binary-mixed organic electron transport layers. New J. Chem. 47 (10), 5048–5055. doi:10.1039/d2nj05127c
Keywords: photovoltaic penetration, photovoltaic, load flow study, wind farm, renewable energy, clean energy, solar
Citation: Soomar AM, Guanghua L, Shaikh S, Shah SHH and Musznicki P (2023) Scrutiny of power grids by penetrating PV energy in wind farms: a case study of the wind corridor of Jhampir, Pakistan. Front. Energy Res. 11:1164892. doi: 10.3389/fenrg.2023.1164892
Received: 13 February 2023; Accepted: 22 May 2023;
Published: 14 June 2023.
Edited by:
Michael Folsom Toney, University of Colorado Boulder, United StatesReviewed by:
Mohamed Salem, University of Science Malaysia (USM), MalaysiaCopyright © 2023 Soomar, Guanghua, Shaikh, Shah and Musznicki. This is an open-access article distributed under the terms of the Creative Commons Attribution License (CC BY). The use, distribution or reproduction in other forums is permitted, provided the original author(s) and the copyright owner(s) are credited and that the original publication in this journal is cited, in accordance with accepted academic practice. No use, distribution or reproduction is permitted which does not comply with these terms.
*Correspondence: Arsalan Muhammad Soomar, YXJzYWxhbi5tdWhhbW1hZC5zb29tYXJAcGcuZWR1LnBs
Disclaimer: All claims expressed in this article are solely those of the authors and do not necessarily represent those of their affiliated organizations, or those of the publisher, the editors and the reviewers. Any product that may be evaluated in this article or claim that may be made by its manufacturer is not guaranteed or endorsed by the publisher.
Research integrity at Frontiers
Learn more about the work of our research integrity team to safeguard the quality of each article we publish.