- Norwegian Institute for Nature Research (NINA), Trondheim, Norway
With the rapid acceleration of wind energy development there is a growing need to meet the consequences this has for the natural environment. Failing to mitigate environmental impacts is an important cause of conflict in wind energy projects, leading to costly delays in planned wind energy development. It is therefore of the utmost importance to identify effective solutions and measures to reduce such impacts. This requires that the joint responsibility for mitigation across stakeholders is recognized and acted upon. This is exemplified with the black-blade concept that has shown to reduce bird collision rates at the Smøla wind-power plant in Norway by 70%. While presented as a “golden bullet” solution in the media, there remain unanswered challenges that need to be addressed. However, instead of disagreeing on the uncertain efficacy elsewhere, I pose that collaborative and transdisciplinary action is needed to jointly resolve remaining challenges and actively seek for solutions to support the sound implementation of promising wind-turbine collision-reducing solutions.
Introduction
The current energy crisis coupled with the IPCC targets to reduce our dependency on fossil fuels is expected to lead to a rapid acceleration of wind energy development, particularly offshore. To reach a low-emission and sustainable society by 2050, wind energy worldwide will have to increase seven- and thirty-fold for onshore and offshore wind, respectively, relative to installed capacity in 2020 (IRENA, 2019). Assuming a direct and indirect area requirement of 0.7 ha/MW (Denholm et al., 2009) would render a total footprint of over 35,000 km2 and 7 000 km2 for onshore and offshore wind, respectively in 2050 (Figure 1). These footprints are however likely an underestimate as they are based on onshore turbines from a study from 2009, and neither include lost airspace nor species-specific sensitivities. With the increase of the total wind energy footprint, also impacts on the natural environment will increase as the developed areas often replace habitats for species (Diffendorfer et al., 2019). While wind energy contributes to the reduction of greenhouse gas emissions, it can also affect biodiversity negatively, and thus necessitates balancing trade-offs between climate change mitigation and environmental protection (IPCC, 2011; Köppel et al., 2014; May et al., 2017; Kati et al., 2021). As wind energy deployment increases and larger wind-power plants are considered, existing concerns become more acute and new concerns may arise (May et al., 2021; Green et al., 2022; Maxwell et al., 2022). One of the major environmental concerns are birds colliding with the wind turbines. To avoid costly delays of planned wind energy projects, it is therefore of the utmost importance to identify effective solutions and measures to reduce such impacts (Marques et al., 2014; May et al., 2015; Gartman et al., 2016).
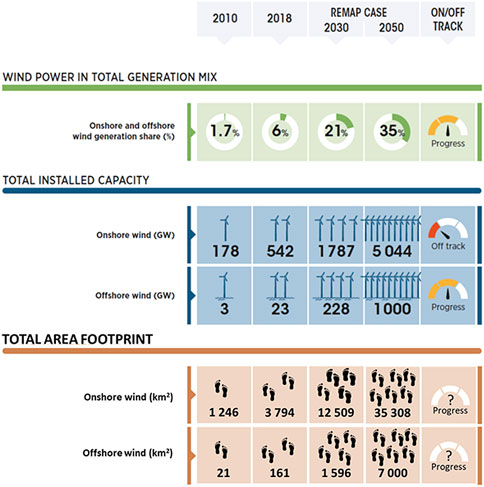
FIGURE 1. Wind roadmap to 2050: tracking progress of wind energy to achieve the global energy transformation (source: IRENA 2019 (top two panels)). Area footprints (lower panel) are calculated from the installed capacity, assuming an area requirement of 0.7 ha/MW.
Mitigation responsibility
Regulatory consenting practice are usually based on impact assessments, however, often lack requirements for mitigating allowed impacts (Tallis et al., 2015; Copping et al., 2020). Failing to fully address environmental impacts is an important cause of conflict in wind energy proposals (Tinker et al., 2005; Inderberg et al., 2020; Vuichard et al., 2022). This often leads to significant and costly delays or refusal of consent (Hastik et al., 2015; May et al., 2017), with subjective assessments of environmental risks, driven largely by budget caps rather than a sound knowledge base (Stoutenborough and Vedlitz, 2016; Inderberg et al., 2019; Pedersen et al., 2019). Evidence-based knowledge on the efficacy of mitigation measures can therefore foster sound decision-making processes, particularly when based on “best available science” efforts (Stoutenborough and Vedlitz, 2016; Weber et al., 2019). As wildlife impacts are not intended, these often become public costs external to the private costs of developing wind power (Cole, 2011). Also, such impacts are also often considered to be “local costs” (Inderberg et al., 2020). To avert this, potential impacts should be considered by developers by evoking the polluter-pays and precautionary principles to prevent harm to the environment (Stabell and Steel, 2018), and encompassed into consenting practice (Tinker et al., 2005; May 2017; Copping et al., 2020). Still, mitigating wildlife impacts is a shared responsibility, since society desires more renewable energy (“beneficiary of development”) as well as a resilient natural environment (“beneficiary of mitigation”) (May 2019). This means that all stakeholders related to wind energy development have a responsibility to ensure that proper measures are implemented to reduce for instance collision risks to birds.
Paint it black
To exemplify this, I would like to address the consequences a recently published in-situ experiment have had in practice. This experiment tested the efficacy of painting one rotor blade black in reducing collision rates in birds (May et al., 2020; Figure 2). The rationale behind this measure is that by painting one rotor blade black will reduce so-called motion smear (i.e., blurring of the moving blades) to increase their visibility to enable birds to take evasive action in due time. May et al. (2020) found a staggering 70% reduction in bird collision rates in a Before-After-Control-Impact (BACI) study performed over an 11-year period at the Smøla wind-power plant in Norway. Upon its publication, this was quickly picked by the media worldwide (35 million hits in the Google search engine for the search terms “bird black blade wind,” d.d. 10.10.2022) as being a simple and cost-effective solution to significantly reduce bird collisions. Even though May et al. (2020) stressed the importance of replicating their study to verify whether similar results are obtained elsewhere to determine to which extent the effect is generalizable across sites and species compositions, it was readily picked up as a “golden bullet” solution. Since then, initiatives have been employed elsewhere to do just that, including Westereems wind farm (171 MW, RWE), the Netherlands; Cavar and Zorreras wind farms (111 MW and 20 MW, Iberdrola), Spain; Glenrock Rolling Hills wind energy project (281 MW, PacifiCorp), Wyoming (United States). In the coming years more insight into this can be expected.
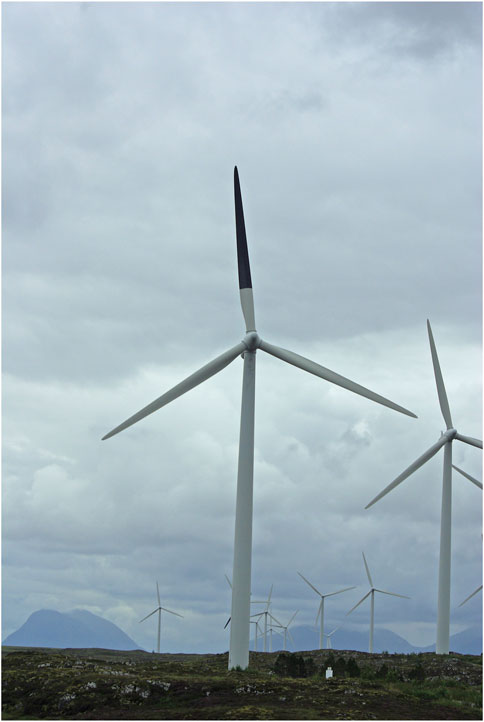
FIGURE 2. A wind turbine in the Smøla wind-power plant with one rotor blade painted black. © Roel May.
Transdisciplinary challenges
Although there has been much interest to implement this mitigation measure, there is also scepticism both due to uncertainty related to its efficacy elsewhere–which is being addressed through the above-mentioned replication studies–but also other challenges that need to be resolved prior to implementation. Besides the need for replications, May et al. (2020) also discussed other aspects that need to be addressed, these being.
• efficacy of other colour regimes,
• issues related to aviation regulations,
• visual effects on humans, and
• mechanical damages to the rotor blades.
To start with the first aspect of colouring, this will require more in-situ tests either through fatality monitoring within existing wind farms or with dedicated research-based experiments. For instance, the laboratory studies investigating effect of colour in reducing “motion smear” (Hodos, 2003) showed that also green, red and yellow performed well (besides black). As wind turbines in various countries must be fitted with red blade tips to forewarn civil aviation, this may well have similar mitigating effects on birds. This remains to be tested during operation.
This brings me to the aviation regulations that require that rotor blades are to be off-white. Although the Smøla study received exemption from these regulations, this may not be the same for other situations. The purpose of painting one blade black, however, is to increase its visibility. Would this also hold for pilots? This merits further investigations (e.g., 3D terrain modelling, virtual reality, flight simulators) as that may lead to a more ready acceptance to provide exemptions from aviation regulations. This could also include studying visibility effects for people living in the vicinity to the wind turbines, and their attitudes towards black blades to reduce bird collisions. Here a trade-off exists between protecting landscape scenery and avifauna (Alphan, 2021). Both aspects receive more influence regarding the licensing of wind farms in most countries (Suškevičs et al., 2019; Diógenes et al., 2020; Vuichard et al., 2022), and are interlinked. There exist multiple and continually refined virtual methods for testing visual perceptions of wind turbines, however often based on worst-case (full-frontal) conditions (Bishop, 2019). Simulation of ‘typical’ conditions that take into account visual magnitude and colour difference may in addition provide greater insight into modification of aesthetic features of individual turbines (Bishop, 2019). Evaluation of human perceptions is of special relevance for the black blade concept as a higher visibility might be accepted more readily when people know its purpose is to reduce bird collisions. Whether or not environmental concerns “colour” human perceptions of turbine visibility in the landscape and enhance socio-technical acceptance merits further research.
Finally, black blades may have effects on the long-term performance of the rotor blades. Due to differential heating of black blades relative to off-white blades, this may lead to structural damages to the blade paints or imbalances in the blades during operation. Such effect may be site-specific, whereby heating may either pose positive effects (e.g., reduced icing of blades) in more Northern latitudes or more negative effects in warmer regions. Black carbon-based paints might also enhance lightning likelihood. Blade and turbine manufacturers and wind farm operators should address such issues both during production and after construction.
Discussion
The consequence of the uncertainty related to such issues has led to opposing views in practice. While some wish to implement the black blade concept without further delay, others remain hesitant and point to the limitations of the published study at hand. However, uncertainty will always remain to some degree, and the only way to reduce uncertainty will be to implement and test the aspects mentioned above. Decisions regarding the necessity of mitigation is in the end based on normative ethical stances (May 2019). Mitigation has–potentially conflicting–economic, technological, social and environmental dimensions that decision-makers have to reconcile. This means that making the right decisions regarding the implementation of mitigation actions, requires insight into the ethical principles guiding decision-making (i.e., energy justice; Jenkins et al., 2016). May (2019) here warns that a pure utilitarian stance focusing solely on the cost-effectiveness of mitigation measures may, especially when uncertain aspects still need to be resolved, lead to a situation where none will take responsibility for action. However, all stakeholders have a certain level of responsibility for mitigation; either as the beneficiary of development or beneficiary of mitigation (May 2019). With all this in mind, I hereby call for collaborative and transdisciplinary action of manufacturers, developers, operators, consenting and environmental agencies as well as the ecological, sociological and engineering research and consulting communities and environmental and societal NGO’s. It will be our joint responsibility and in everyone’s interest to overcome practical hindrances to implementation to ensure a sustainable development of wind energy. Jointly, addressing the above-mentioned challenges and actively seeking for solutions will support the sound implementation of the black blade concept and other wind-turbine collision-reducing solutions in the years to come. Also, results should be made readily available to all to ensure the immediate uptake of effective solutions. Given the fast pace of development in the decades to come, having collision-reducing mitigation solutions that are proven effective and ready to implement is of the utmost importance to tackle the climate-energy-nature nexus.
Data availability statement
The original contributions presented in the study are included in the article/supplementary material, further inquiries can be directed to the corresponding author.
Author contributions
The author confirms being the sole contributor of this work and has approved it for publication.
Conflict of interest
The author declares that the research was conducted in the absence of any commercial or financial relationships that could be construed as a potential conflict of interest.
Publisher’s note
All claims expressed in this article are solely those of the authors and do not necessarily represent those of their affiliated organizations, or those of the publisher, the editors and the reviewers. Any product that may be evaluated in this article, or claim that may be made by its manufacturer, is not guaranteed or endorsed by the publisher.
References
Alphan, H. (2021). Modelling potential visibility of wind turbines: A geospatial approach for planning and impact mitigation. Renew. Sustain. Energy Rev. 152, 111675. doi:10.1016/j.rser.2021.111675
Bishop, I. D. (2019). The implications for visual simulation and analysis of temporal variation in the visibility of wind turbines. Landsc. Urban Plan. 184, 59–68. doi:10.1016/j.landurbplan.2018.12.004
Cole, S. G. (2011). Wind power compensation is not for the birds: An opinion from an environmental economist. Restor. Ecol. 19 (2), 147–153. doi:10.1111/j.1526-100X.2010.00771.x
Copping, A. E., Gorton, A. M., May, R., Bennet, F., DeGeorge, E., Repas Goncalves, M., et al. (2020). Enabling renewable energy while protecting wildlife: An ecological risk-based approach to wind energy development using ecosystem-based management values. Sustainability 12 (22), 9352. doi:10.3390/su12229352
Denholm, P., Hand, M., Jackson, M., and Ong, S. (2009). Land-use requirements of modern wind power plants in the United States. Golden, CO, USA: National Renewable Energy Laboratory.
Diffendorfer, J. E., Dorning, M. A., Keen, J. R., Kramer, L. A., and Taylor, R. V. (2019). Geographic context affects the landscape change and fragmentation caused by wind energy facilities. PeerJ 7, e7129. doi:10.7717/peerj.7129
Diógenes, J. R. F., Claro, J., Rodrigues, J. C., and Loureiro, M. V. (2020). Barriers to onshore wind energy implementation: A systematic review. Energy Res. Soc. Sci. 60, 101337. doi:10.1016/j.erss.2019.101337
Gartman, V., Bulling, L., Dahmen, M., Geißler, G., and Köppel, J. (2016). Mitigation measures for wildlife in wind energy development, consolidating the state of knowledge — Part 2: Operation, decommissioning. J. Environ. Assess. Policy Manag. 18 (3), 1650014. doi:10.1142/s1464333216500149
Green, R. E., Gill, E., Hein, C., Couturier, L., Mascarenhas, M., May, R., et al. (2022). International assessment of priority environmental issues for land-based and offshore wind energy development. Glob. Sustain. 5, e17–e21. doi:10.1017/sus.2022.14
Hastik, R., Basso, S., Geitner, C., Haida, C., Poljanec, A., Portaccio, A., et al. (2015). Renewable energies and ecosystem service impacts. Renew. Sustain. Energy Rev. 48, 608–623. doi:10.1016/j.rser.2015.04.004
Hodos, W. (2003). Minimization of motion smear: Reducing avian collisions with wind turbines. Golden, CO, USA: National Renewable Energy Laboratory.
Inderberg, T. H. J., Rognstad, H., Saglie, I.-L., and Gulbrandsen, L. H. (2019). Who influences windpower licensing decisions in Norway? Formal requirements and informal practices. Energy Res. Soc. Sci. 52, 181–191. doi:10.1016/j.erss.2019.02.004
Inderberg, T. H. J., Theisen, O. M., and Flåm, K. H. (2020). What influences windpower decisions? A statistical analysis of licensing in Norway. J. Clean. Prod. 273, 122860. doi:10.1016/j.jclepro.2020.122860
Ipcc, (2011). Intergovernmental panel on climate change special report on renewable energy sources and climate change mitigation. Cambridge, UK: Cambridge University Press.
IRENA, (2019). Future of wind: Deployment, investment, technology, grid integration and socio-economic aspects (A Global Energy Transformation paper). Abu Dhabi, UAE: International Renewable Energy Agency.
Jenkins, K., McCauley, D., Heffron, R., Stephan, H., and Rehner, R. (2016). Energy justice: A conceptual review. Energy Res. Soc. Sci. 11, 174–182. doi:10.1016/j.erss.2015.10.004
Kati, V., Kassara, C., Vrontisi, Z., and Moustakas, A. (2021). The biodiversity-wind energy-land use nexus in a global biodiversity hotspot. Sci. Total Environ. 768, 144471. doi:10.1016/j.scitotenv.2020.144471
Köppel, J., Dahmen, M., Helfrich, J., Schuster, E., and Bulling, L. (2014). Cautious but committed: Moving toward adaptive planning and operation strategies for renewable energy's wildlife implications. Environ. Manage 54 (4), 744–755. doi:10.1007/s00267-014-0333-8
Marques, A. T., Batalha, H., Rodrigues, S., Costa, H., Pereira, M. J. R., Fonseca, C., et al. (2014). Understanding bird collisions at wind farms: An updated review on the causes and possible mitigation strategies. Biol. Conserv. 179, 40–52. doi:10.1016/j.biocon.2014.08.017
Maxwell, S. M., Kershaw, F., Locke, C. C., Conners, M. G., Dawson, C., Aylesworth, S., et al. (2022). Potential impacts of floating wind turbine technology for marine species and habitats. J. Environ. Manag. 307, 114577. doi:10.1016/j.jenvman.2022.114577
May, R., Gill, A. B., Köppel, J., Langston, R. H. W., Reichenbach, M., Scheidat, M., et al. (2017). “Future research directions to reconcile wind turbine–wildlife interactions,” in Wind energy and wildlife interactions: Presentations from the CWW2015 conference. Editor J. Köppel (Cham: Springer International Publishing), 255–276.
May, R., Jackson, C. R., Middel, H., Stokke, B. G., and Verones, F. (2021). Life-cycle impacts of wind energy development on bird diversity in Norway. Environ. Impact Assess. Rev. 90, 106635. doi:10.1016/j.eiar.2021.106635
May, R., Nygård, T., Falkdalen, U., Åström, J., Hamre, Ø., and Stokke, B. G. (2020). Paint it black: Efficacy of increased wind-turbine rotor blade visibility to reduce avian fatalities. Ecol. Evol. 10 (16), 8927–8935. doi:10.1002/ece3.6592
May, R., and Perrow, M. (2017). “Mitigation options for birds,” in Wildlife and windfarms: Conflicts and solutions (Exeter, United Kingdom: Pelagic Publishing), 124–145.
May, R., Reitan, O., Bevanger, K., Lorentsen, S. H., and Nygard, T. (2015). Mitigating wind-turbine induced avian mortality: Sensory, aerodynamic and cognitive constraints and options. Renew. Sustain. Energy Rev. 42, 170–181. doi:10.1016/j.rser.2014.10.002
May, R. (2019). “The mitigation of impact and the impact of mitigation: An ethical perspective,” in Wind energy and wildlife impacts. Balancing energy sustainability with wildlife conservation. Editors R. Bispo, J. Bernardino, H. Coelho, and J. Lino Costa (Cham, Switzerland: Springer), 93–113.
Pedersen, S., Handberg, Ø. N., and Løset, F. (2019). Kvalitet på konsekvensutredninger av klima-og miljøtemaer i kommuneplanens arealdel. Oslo, Norway: Menon Economics/SWECO.
Stabell, E. D., and Steel, D. (2018). Precaution and fairness: A framework for distributing costs of protection from environmental risks. J. Agric. Environ. Ethics 31, 55–71. doi:10.1007/s10806-018-9709-8
Stoutenborough, J. W., and Vedlitz, A. (2016). The role of scientific knowledge in the public's perceptions of energy technology risks. Energy Policy 96, 206–216. doi:10.1016/j.enpol.2016.05.031
Suškevičs, M., Eiter, S., Martinat, S., Stober, D., Vollmer, E., de Boer, C. L., et al. (2019). Regional variation in public acceptance of wind energy development in Europe: What are the roles of planning procedures and participation? Land Use Policy 81, 311–323. doi:10.1016/j.landusepol.2018.10.032
Tallis, H., Kennedy, C. M., Ruckelshaus, M., Goldstein, J., and Kiesecker, J. M. (2015). Mitigation for one and all: An integrated framework for mitigation of development impacts on biodiversity and ecosystem services. Environ. Impact Assess. Rev. 55, 21–34. doi:10.1016/j.eiar.2015.06.005
Tinker, L., Cobb, D., Bond, A., and Cashmore, M. (2005). Impact mitigation in environmental impact assessment: Paper promises or the basis of consent conditions? Impact Assess. Proj. Apprais. 23 (4), 265–280. doi:10.3152/147154605781765463
Vuichard, P., Broughel, A., Wüstenhagen, R., Tabi, A., and Knauf, J. (2022). Keep it local and bird-friendly: Exploring the social acceptance of wind energy in Switzerland, Estonia, and Ukraine. Energy Res. Soc. Sci. 88, 102508. doi:10.1016/j.erss.2022.102508
Weber, J., Biehl, J., and Köppel, J. (2019). “Lost in bias? Multifaceted discourses framing the communication of wind and wildlife research results: The PROGRESS case,” in Wind energy and wildlife impacts. Balancing energy sustainability with wildlife conservation. Editors R. Bispo, J. Bernardino, H. Coelho, and J. L. Costa (Cham, Switzerland: Springer Nature), 179–204.
Keywords: wind energy (WE), environmental impact, mitigation measure, sustainable development, bird collision risk
Citation: May R (2023) Joint responsibility in the development of effective wind-turbine collision-reducing solutions. Front. Energy Res. 11:1146324. doi: 10.3389/fenrg.2023.1146324
Received: 17 January 2023; Accepted: 14 April 2023;
Published: 21 April 2023.
Edited by:
David Howe Wood, University of Calgary, CanadaReviewed by:
Johann Koeppel, Technical University of Berlin, GermanyCopyright © 2023 May. This is an open-access article distributed under the terms of the Creative Commons Attribution License (CC BY). The use, distribution or reproduction in other forums is permitted, provided the original author(s) and the copyright owner(s) are credited and that the original publication in this journal is cited, in accordance with accepted academic practice. No use, distribution or reproduction is permitted which does not comply with these terms.
*Correspondence: Roel May, roel.may@nina.no