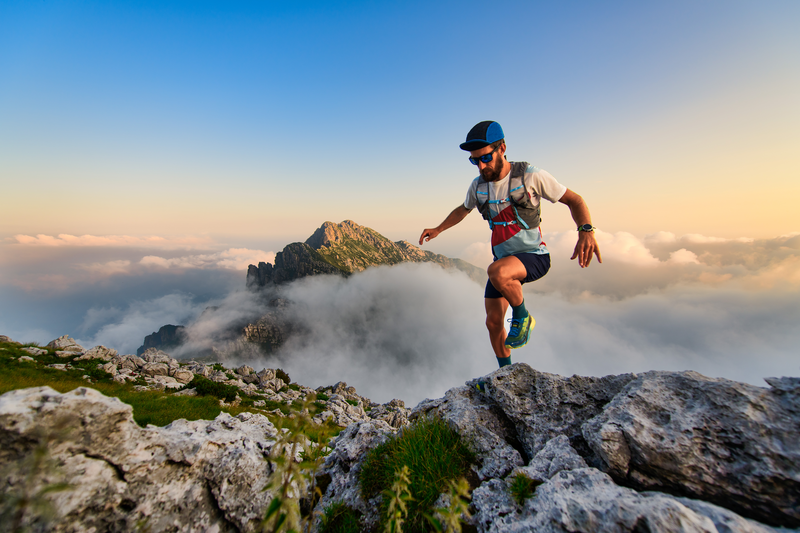
95% of researchers rate our articles as excellent or good
Learn more about the work of our research integrity team to safeguard the quality of each article we publish.
Find out more
ORIGINAL RESEARCH article
Front. Energy Res. , 15 June 2023
Sec. Solar Energy
Volume 11 - 2023 | https://doi.org/10.3389/fenrg.2023.1137941
This article is part of the Research Topic Solar Desalination for Small-scale Decentralized Applications in Remote Areas View all 5 articles
Water scarcity is widely known as one of the major current issues. As of now, many solar distillers are using the same concept of evaporation and condensation of saline water to produce distillates. The main problem with such solar distillers is their low productivity, as the latent heat produced is lost to the surroundings. A multi-stage solar distiller can solve the issue of productivity by utilizing the latent heat released. This design consists of multiple solar distillers stacked one on top of the another, producing distillates in each stage while having the same amount of energy intake. Malaysia falls within the tropical belt where solar radiation appears to be diffused, resulting in low-quality heat energy that can be absorbed. To solve this problem, concentrated solar power (CSP) technology has been introduced. However, CSP technology can be very expensive, thus using a Fresnel lens as a direct refraction device will be a cheaper option. In this study, a four-stage solar distiller system integrated with the Fresnel lens is introduced. It was found that the productivity and efficiency of the multi-stage system were higher, with a productivity of 0.164 g/kJ and efficiency of 39.5% when compared with the single-stage solar distiller with a productivity of 0.104 g/kJ and an efficiency of 23.5%.
• A multi-stage solar distiller has been tested in Malaysian weather.
• A Fresnel lens has been implemented into the first stage as a solar concentrator.
• Multi-stage solar distiller has a higher productivity and efficiency.
• Efficiency and yield dropped significantly from stage 3 onwards.
• Unit cost of water is lower in the multi-stage system than in the single-stage solar distiller.
Water is one of the essential natural elements for every life form on earth. There is approximately 71% of the earth’s stratum that is covered by water (Amiri, 2022). Even though seas and oceans cover up to 97.5% of global water, merely 0.5% of the water found fresh in rivers and lakes, and as underground water, is usable (Kumar et al., 2013). This is because most of the water obtained has some harmful organisms present and contains a large number of salts, which make the water unusable. Water scarcity has been widely known to be a substantial current issue, as the demand continues to increase due to the rapid growth in population and industry (Shah et al., 2022). Sharshir et al. (2022) reported that approximately two-thirds of the total population suffer from water shortage each year, with half of this population coming from China and India. The use of global freshwater has increased by six times over the past 100 years (Ahmed et al., 2022). According to Shahzad et al. (2017), the global water demand is expected to increase by 55% in 2050. This is mainly because of a higher GDP growth rate, which increases the water demand for manufacturing, power generation, and domestic sector use by 400%, 140%, and 130%, respectively. Currently, there are 1.2 billion people who live in water scarcity areas, with less than 1,000 m3 of freshwater available per capita per year (Shahzad et al., 2017).
To obtain more freshwater, saline water from the oceans can be processed using desalination methods. According to Amiri (2022), the main desalination technology is reverse osmosis, which accounts for 60% of the global capacity. This is followed by multi-stage flash (MSF) methods with 26.8% share. However, these two methods are expensive and require a huge amount of energy (Khanmohammadi et al., 2022). Multi-effect distillation (MED) is similar to the MSF system, as both methods are operating with thermal energy in multiple stages to undergo evaporation and condensation which converts brackish water into freshwater. Even so, MED operates with low-pressure steam, while MSF requires higher energy and pressure steam. As for the stages of the MED system, vapors produced are condensed in successive stages, which recover the heat of condensation, thus improving energy utilization (Shahzad et al., 2015). Shahzad et al. (2015) have provided an innovative design that integrates the MED system with a heat-driven adsorption desalination (AD) cycle. This hybrid system is also known as MEDAD, which utilizes low-temperature energy sources. The added AD cycle can recover additional heat from any remaining vapor by further cooling and dehumidification with an adsorbent material like silica and zeolite (Shahzad et al., 2019).
Due to the rise in environmental pollutions, using renewable energies has become more vital in water desalination processes. Another advantage of renewable energies is that it is applicable in remote and underdeveloped regions, where there is no supply of conventional energy (Chen, and Xie, 2022). Solar energy is the best alternative source for heating in distillation, mainly because of its availability and low consumption of energy resources (Hameed, 2022). It uses the principle of the hydrologic cycle, where evaporated water rises and cools down by the wind to its dew point to produce freshwater as rain (Lal et al., 2017). Even in the mediaeval ages, people have been using solar distillers, and this ancient technology has become more popular during the past 50 decades (Demeke Agonafer, 2020). A solar distiller will consist of a basin filled with saline/brackish water covered by an inclined glass/transparent lid through which the solar heat enters and evaporate the contaminated water. The generated vapor is condensed on the inclined glass/transparent lid’s inner side before being collected as the distillate. Although the produced water quality is high, the process is limited to small quantity production because of scattered solar irradiation and the unavailability of solar heat due to intermittent cloudy weather. In order to improve the efficiency of solar distillers, ideas surrounding the capability of effectively capturing solar energy are considered. Multi-staging of solar distillers is a suitable solution since it allows multiple practical uses of the input energy.
Malaysia falls within the tropical belt, where the incoming solar irradiation appears to be diffused due to the humid environment. It means that even though this region receives substantial solar heat, most of the heat obtained is not considered high-quality energy. One way to utilize the available energy is using concentrated solar power (CSP) technology. However, CSP technology can be quite expensive; instead, using a Fresnel lens as a direct refraction device will be cheaper. Besides, the Fresnel lens has a wider aperture but a shorter focal length and can be made from acrylic material. Therefore, in this project, a solar distiller is planned to be designed and developed using multiple stages and is associated with a Fresnel lens. This study aims to design and develop a multi-stage solar distiller associated with a Fresnel lens and then evaluate the performance of such a setup before comparing it with other existing fabricated units in terms of economic feasibility.
Based on many researchers like Reddy et al. (2012), Abed (2018), and Younas et al. (2016), a similar conclusion can be obtained on the effects of solar irradiance, number of stages, the surface area of a solar concentrator, the distance of the gap between the stages, and evaporation–condensation temperature difference, on the yield produced. First, the effect of solar irradiance is not surprising since a higher intensity can cause more heat to be absorbed into the system, thus increasing the evaporation rate in the distiller. As for the number of stages, most researchers have confirmed that the optimum number of stages is four. However, the overall distillate produced will still increase as the number of stages passes beyond the fourth stage, just that it is not great to implement more stages since the increase in yield after the fourth stage is too low to be considered adequate. Next, the surface area of the thermal concentrator plays a significant part in affecting the yield obtained. Because a larger surface area will concentrate a higher amount of solar irradiance, resulting in more heat that will be absorbed by the thermal concentrator, which will further increase the temperature of the water basin in the distiller. The distance between each tray can affect the yield obtained as well. It is because as the gap increases, the vapor produced will take longer to reach the condensing plate from the next stage, thus reducing the overall processing time of condensation. Last, the temperature difference between the water in each tray or basin with the condensing plate can affect the amount of distillate produced. An increase in temperature difference, whether to provide more external heating or cooling on the condensing plate, will increase the rate of evaporation or condensation, producing more yield over time.
There are several different multi-stage solar distiller designs as of now. The work presented by Ahmed et al. (2009) comprises three cylindrical stages stacked one on top of the other and is intended to see if there are any improvements for such a system to perform under vacuum conditions. The maximum yield was recorded to be 14.2 kg/m2/day at a vacuum pressure of 0.5 bar, which has an increase of 45% from the productivity of the solar distiller at atmospheric pressure. The authors have also concluded that these proposed three stages of solar desalination will have a unit cost of $25.44/1,000 gallons of distilled water. The design proposed by Abdessemed et al. (2019) is distinct, as it comes with two different designs of the evaporation chamber in conjunction with a parabolic trough solar collector. This is because the authors wanted to find out whether a “V”-shaped tray produces more yield than a “ʌ”-shaped tray and found out later on that the “V”-shaped tray was twice as efficient when compared to the “ʌ”-shaped tray. Abed (2018) used three focal concentric collectors that could track the Sun’s movement to ensure that the solar radiation absorbed was always vertical. The prototype was fabricated to have a total of three stages. Heat absorbed by the concentric collector causes the heat transfer fluid to gain temperature, which in turn causes an increase in temperature for the water in the lower basin after the heat exchange. With the obtained results, the authors mentioned that the maximum yield obtained was 22 l/m2/day with a minimum yield of 5 l/m2/day.
With all the different designs reviewed, the productivity of the multi-stage solar distiller was higher than the modified single-slope solar distiller with a corrugated tray proposed by Abdullah et al. (2021). This is mainly due to the utilization of latent heat of vaporization that was released from the condensing plate, which can be used up to heat any water available in the next stage. However, studies regarding the multi-stage solar distiller are commonly seen nowadays, especially in the Middle Eastern countries where the solar irradiance is enormous during the day. On the other hand, the multi-stage solar distiller is uncommon in Malaysia, and is even rarer in research that consists of actual outdoor experiments. This is because most of the local researchers perform only theoretical results, which can be too ideal for a country like Malaysia that has inconsistent solar irradiance at different times of the day. One local research conducted by Ahmed et al. (2009) uses a flat plate collector for solar water heating, which will then transfer the heat to the water in the solar distiller via a heat exchanger. A series of photovoltaic thermal collectors were installed to power up the solar vacuum pump, making the system very costly. When compared to other designs proposed by researchers from Middle Eastern countries, the design can be quite simple with an acceptable range of productivity. A Fresnel lens can be an excellent solution to the costly solar water-heating system. At the moment, there are only two designs that use a Fresnel lens as the thermal concentrator. The first design was proposed by Wu et al. (2017), using the humidification–dehumidification technique. This is different from any conventional solar distiller that uses the evaporation–condensation process. Younas et al. (2016) proposed the other designs that use a Fresnel lens. For this design, the author used the Fresnel lens as the only thermal concentrator to raise the temperature of the water basin in the multi-stage solar distiller. However, this research was carried out in Abu Dhabi, where the amount of solar irradiance is much higher than in Malaysia. Due to this, it is unlikely that this design is feasible to be implemented in Malaysia due to the lower amount of solar heat absorbed. Furthermore, most of the proposed designs used a closed-loop system, where heat is transferred to the water inside the basin via a heat exchanger. This will increase the cost as the heat exchanger is costly, especially those made of copper tubes. Currently, only one study by Reddy et al. (2012) has proposed an open-loop system that excludes the requirement for a heat exchanger, as saline water is fed directly to the solar distiller. However, the research was concluded solely based on theoretical calculations, as no actual experiment has been conducted. Therefore, the effectiveness of the open-loop system for a solar desalination process is yet to be explored. In a recently conducted study in Malaysia, it was found that using two Fresnel lens instead of a single large one increased the production per unit of solar irradiation by 39% for a single-sloped single-stage solar distiller (Choong et al., 2020).
At the moment, there are very few research studies that have been conducted in Malaysia. Considering the unsuitable climate in Malaysia where solar irradiation appears to be diffused due to the humid environment and implementing the use of the Fresnel lens in the solar distiller to amplify solar heating is even less seen. Moreover, the design of this multi-stage system is unlike any conventional multi-stage solar distiller design available at the moment. The double-slope single-stage solar distiller with a Fresnel lens attached, was coupled directly to a three-stage solar distiller through a solar powered pump, making it an open-loop system. For a conventional multi-stage system, the closed-loop system is a more common design that is used where hot water from a thermal concentrator enters a heat exchanger in the lowest stage of a multi-stage solar distiller. This is because the closed-loop system can maintain the temperature for a long duration, whereas an open-loop system only holds the desired temperature for a short period of time (Mallouh et al., 2022). However, the main drawback of a closed-loop system is its high cost, which is unsuitable to be implemented in economically developing countries, let alone remote areas in such countries.
In this study, a portable multi-stage solar distiller has been fabricated and tested. The productivity and efficiency have been evaluated, and it has been found that the multi-stage solar distiller associated with the Fresnel lens can be a viable device to improve the efficiency of solar desalination systems in the tropical weather.
The basic principle of a single-stage solar distiller is replicating the hydrologic cycle, where evaporated water rises and cools down by the wind to its dew point to produce freshwater as rain. Applying the same concept to the solar distiller, when the water vapor rises and meets with the lower temperature glass, it will condense on the glass surface and form freshwater. The water will fall alongside the slanted glass until it reaches the collector in order to be collected. The water produced this way will not have any impurities, such as salts and heavy metals, and will be considered fresh since it is no longer saline.
The multi-stage solar distiller has a very similar concept, except that the same process is repeated depending on the number of stages. The water basin located at the bottom of the system will be treated as the first stage of the system, where vapor will be produced when heat has been supplied. An external element, such as a heater or flat plate collector normally achieves this. Vapor is produced, then rises and meets with the surface from the next stage, substituting a tray containing saline water. The forming of freshwater from this stage will also release the latent heat of vaporization, which can heat up the water content from the tray used by the next stage. The subsequent stage follows the same process until it reaches the last stage, where a glass or transparent lid will be used as the cover again.
As for the design used by this research, a double-slope single-stage solar distiller with two Fresnel lenses placed on top of each glass sheet will be the main heating source for the multi-stage system, which is proposed to be a three-stage system. Adding both the systems together results in achieving the total of a four-stage solar distiller system. The single-stage solar distiller is first allowed to be exposed to solar radiation such that the water inside the basin reaches a higher temperature before being pumped into the multi-stage system. This is to ensure that the rate of evaporation can be higher for the multi-stage system due to the greater temperature difference between the water temperature and condensing surface temperature. The proposed design of the four-stage solar distiller system is shown in Figure 1.
The single-stage solar distiller is built from a wooden base with a dimension of 0.6 m × 0.6 m × 0.4 m for its length, width, and height, respectively. The support surrounding this base is made of an aluminum bar, and each side is covered with a 3-mm-thick plexiglass. A square stainless steel tray, painted black, is used as the water basin. The dimensions for both the length and width are 0.5 m, and it has a total height of 0.1 m. Two sets of the glass panel with a thickness of 3 mm are used, each with a length and width of 0.55 m and 0.25 m, respectively. The glass panel is placed onto the support that is leaning at an angle of 30°. Each glass panel has two sets of Fresnel lens attached above. These Fresnel lens are made of optical PMMA plastics that have a focal length of 0.33 m and an overall transmittance of 92%. The dimensions of each Fresnel lens are measured to be 0.3 m on both sides, with a thickness of 2 mm each.
Each support is made from a slotted iron steel bar for the multi-stage system. The distance between each stage is set to be 0.1 m. Three sets of non-stick rectangular baking trays are used as the water basin for each stage. These trays have a dimension of 0.435 m × 0.295 m × 0.015 m as their length, width, and height, respectively. Each tray is inclined at an angle of 10° with a total collection area of 0.08 m2. The top of the multi-stage system is covered with a 2-mm-thick polycarbonate sheet. The four outer sides are all covered with a 3-mm-thick acrylic sheet for better insulation of heat. As for the distillate collector, it is built from modifying the PVC water pipes, which act as a storage to collect freshwater, before transferring them to the measuring cylinder through a PVC clear hose. Since the system runs continuously, excessive water supplied to the bottommost stage in the multi-stage system is transferred back into the first stage of the solar distiller to prevent it from exceeding the threshold set for water in that stage. Both systems are further sealed with silicone to minimize heat loss and prevent leakage through the tiny holes between the cover and supports.
To supply water from the first stage of the solar distiller to the multi-stage system, a 5 V DC-powered submersible water pump is used in conjunction with a 5 V, 300 mAh solar panel. Hot water from the first stage of the solar distiller is supplied to the third and fourth stages of the system when it has depleted significantly in these trays. This is because hot water is always supplied to the bottommost stage of the multi-stage system, which is the second stage in this case. The excessive amount of water supplied to this stage will be transferred back to the first stage of the solar distiller to be reheated again. The single-stage solar distiller, multi-stage system, and the overall four-stage system are shown in Figures 2A, B and Figure 2C, respectively. Table 1 shows the materials and also the dimensions for each system.
FIGURE 2. (A) Double-slope single-stage solar distiller. (B) Three stages solar distiller. (C) Combined four-stage solar distiller system.
The experiment setup was performed to evaluate the performance of the proposed multi-stage solar distiller system in terms of economic feasibility and productivity. The setup of this multi-stage system was placed on the rooftop of the KB building of Universiti Tunku Abdul Rahman–Sungai Long Campus, Kajang, Malaysia, with the coordinates 2.9935° N and 101.7874° E. The experiments were carried out twice every week between June and August 2022, with the readings being taken down at 1-h intervals from 9:00 am to 7:00 pm. The amount of freshwater produced was measured with a 25 ml measuring cylinder for each stage, which was attached to a pipe connecting to the distillate collector. These measuring cylinders were emptied when fully filled so that they could be used for measuring again. UNI-T UT320D “K”-type thermocouple was used to measure the temperature of the water inside each basin and their respective condensing surface temperature to calculate the rate of evaporation and the productivity of their respective systems. Solar radiation was also measured at hourly intervals using an SM206 model solar power meter.
The mathematical model can be separated between the first stage of the solar distiller and the multi-stage system. Since the first stage of the solar distiller has a conventional design that is commonly used, many researchers have come up with several equations for calculating the convective heat transfer and evaporative heat transfer. In the literature provided by Kabeel et al. (2019) and Abujazar et al. (2018b), both have assumed that the system is fully sealed with no heat loss considered. The equations are given in Equation 1 and Equation 2.
However, the saturation pressure must be known to determine these two variables. Water saturation pressure above the water surface and underneath the glass lid has also been mentioned in the literature by Alduchov and Eskridge (1996) and is given in Equation 3 and Equation 4, respectively.
With the saturation pressure being calculated and by obtaining the temperature of the condensing surface and water during data collection, convective and evaporative heat transfer can be determined. The evaporative heat transfer is used to calculate the mass of the yield obtained. However, the latent heat of vaporization of saline water has to be determined first. The mass of the yield can be calculated by applying Equation 5 (Badusha and Arjunan, 2013; Abujazar et al., 2018a), while the latent heat of vaporization of saline water is given in Equation 6 and Equation 7(Henderson-Sellers, 1984; Sharqawy et al., 2010).
The value for
Theoretical yield for both systems can be calculated and compared with the actual yield collected when the system is exposed to outdoor conditions. To calculate the productivity, the total amount of energy absorbed by the first stage of the solar distiller must be calculated, which is given in Equation 8. The productivity and efficiency of this first stage of the solar distiller is also given in Equations 9, 10, respectively.
Equations 6–10 are applied for the multi-stage systems in order to calculate the productivity and efficiency of the system. The heat input to the lowest stages of the multi-stage solar distiller is based on the hot water output from stage 1. This can be determined by simply applying Equation 11. The amount of water carried by the basin is measured to be 1.5 kg. Since the temperature of water inside this basin is measured every hour, the change in temperature is known. Using Equation 11, the amount of heat input into the system in a day is known.
The variable
The measurements recorded by each apparatus or device are subjected to certain uncertainty. These measurements are distributed uniformly, making it a type B uncertainty, where the accuracy has to be divided by the square root of three. Equation 12 is applied to calculate the uncertainty for the mass of yield, energy, and average latent heat. Meanwhile, Equation 13 shows the generalized equation for calculating the efficiency of both systems.
The optimum result was obtained on 22 August 2022. The measurements recorded were applied on the theoretical calculations to determine the productivity, efficiency, and cost per unit liter for each system.
Solar irradiance and ambient temperature have been measured every hour between 9:00 am and 7:00 pm and recorded as shown in Figure 3A.
FIGURE 3. (A) Ambient temperature and solar irradiance throughout the day. (B) Temperature of water from each stage’s basin throughout the day. (C) Temperature of the condensing surface from each stage throughout the day. (D) Cumulative yield obtained for each stage throughout the day. (E) Efficiency and productivity of a multi-stage system.
The optimum solar irradiance was seen to have occurred at 14:00 h. It could reach as high as 952.8 W/m2, which was measured using the SM206 solar power meter. Solar irradiance did not have any significant change between 13:00 and 15:00 when compared with that at 15:00 onwards. This was also the period where the amount of freshwater collected by the solar distiller was at its highest. The ambient temperature, however, was maintained above 30°C for most of the time. The water inside each basin was exposed to solar radiation under these conditions.
The temperature of water for each basin and their corresponding condensing surfaces was measured and recorded as shown in Figure 3B and Figure 3C, respectively. According to these two figures, it is clear that the water temperature from each stage reached its peak value at the same time, which is at 2:00 pm. The temperature of water in stage 1 reached up to 68.1°C, while the temperature of its condensing surface had a peak value of 57.1°C. The overall trend for both graphs that represent the temperature is also identical to the solar irradiance pattern, where there is a significant increase in temperature during the 12:00 h–15:00 h before it declines rapidly. The temperature of water in the second stage is only slightly lower than that in the first stage. This is mainly due to the fact that water from the first stage is directly fed into the second stage with a small amount of heat loss. However, the temperature from the third and fourth stages is significantly lower than that from the first two stages. This is because the increase in water temperature during these two stages is only caused by the latent heat of vaporization released in the previous stage of the system. As for the temperature on the condensing surface for each stage, there are signs of fluctuations in the results. Unlike Figure 3B, which has a smooth curve throughout the multi-stage, the overall trend for the first and fourth stages deviates from the expected trend. This can be explained by the additional cooling factor caused by the wind. The condensing surface of the single-stage and multi-stage solar distiller is exposed to the wind, which are the first and fourth stages in this case. The second and third stages, however, are properly sealed. Therefore, the cooling factors from the wind are only significant on the condensing surface of the first and fourth stages. To simplify, the amount of solar irradiance greatly affects the temperature of water in every stage, as any increase in solar irradiance causes more heat to be absorbed by water inside the basin. Not to mention, the improvement on the rate of evaporation due to higher temperature differences causes an increase in the amount of latent heat of vaporization produced inside the multi-stage system, resulting in a higher temperature in the subsequent stage.
The total amount of freshwater collected in the first stage of the solar distiller was 209 g. However, only 91 g of water was collected by the multi-stage solar distiller. Using Equation 5 on both systems, the total theoretical yield for the single-stage and multi-stage solar distillers can be calculated as 230 g and 98 g, respectively. The percentage error for the yield was calculated as 9.1% and 7.2% for the first-stage and multi-stage systems, respectively.
The cumulative production of freshwater for every stage was calculated in kg/m2/day and is shown in Figure 3D. From the very same figure, there is a noticeable difference in the amount of freshwater collected in the first stage when compared to the subsequent stages. The main reason behind this is due to the larger water basin and condensing surface area in the first stage, storing approximately six times more than the single multi-stage tray. This causes freshwater to form on the larger surface area of the condensing surface. Not to mention, the first stage of the solar distiller acts as the main source of heat supplied to the multi-stage system. Heat received by the multi-stage system is expected to be less than the amount of solar heat absorbed by the single-stage solar distiller alone. Despite this, the total amount of yield obtained in kg/m2/day for the multi-stage system is still slightly higher than that of the single-stage solar distiller at 1.1 kg/m2/day when compared to 0.8 kg/m2/day.
Using Equations 9, 10, the efficiency of the first stage solar distiller and multi-stage solar distiller is calculated to be 23.5%, with a productivity of 0.1036 g/kJ and 39.5% with 0.1638 g/kJ, respectively. The breakdown of the efficiency and productivity for the multi-stage system are 28.8% and 0.12 g/kJ for stage 2, 8.3% and 0.03 g/kJ for stage 3, and 2.4% and 0.01 g/kJ for stage 4. An illustration of this breakdown is shown in Figure 3E. From the very same figure, it can be seen that the efficiency and productivity show a tremendous decrease in stage 3 and stage 4. This is mainly because hot water is continuously fed in stage 2 only, while stages 3 and 4 have to rely on the latent heat of vaporization released from its previous stages to heat the water up inside their respective basins.
When considering the whole system, the average efficiency and productivity of the combined system drops to 26.8% and 0.12 g/kJ, respectively. Stage 1 remains to be the most efficient, covering up to 69% of the heat energy used. It is then followed by stage 2 at 22%, stage 3 at 7%, and then stage 4 at only 2% of the total energy. This is because only stage 1 was exposed to the most solar irradiance. Not to mention, the solar heating was amplified with the installation of the Fresnel lens on stage 1, which resulted in a huge energy intake difference between the single-stage and multi-stage systems, with a total of 2,221 kJ in stage 1 and only 596 kJ in stage 2 onwards.
All the measured quantities were distributed uniformly, making the uncertainty a type B in this case. The accuracy for each measuring apparatus was divided by a square root of three. The SM206 model solar power meter was stated to have an accuracy of ±10 W/m2, while the accuracy for the UNI-T UT320D “K”-type thermocouple was stated to be ±0.2°C. The uncertainty for the mass of freshwater collected had an accuracy of ±1 g. All the dimensions were measured using the same meter rule, which had an accuracy of ±1 mm.
Using the abovementioned accuracies on the different variables mentioned previously, the uncertainty for the total energy can be simply known by applying Equation 12, differentiating energy with respect to solar irradiance. Since the measurements taken were from 9:00 h to 19:00 h, the uncertainty has to be calculated every hour. The sum of the uncertainty for the total energy for the single-stage solar distiller and multi-stage system was calculated to be ±15.1 kJ and ±66.7 kJ, respectively.
To calculate the uncertainty for the efficiency of each system, the uncertainty of the average latent heat of vaporization of saline water must be first known, by differentiating Equation 7 with respect to temperature and applying Equation 12 in order to find this uncertainty. However, this uncertainty is only applicable for one particular hour. Therefore, the average uncertainty for the latent heat of vaporization of saline water has to be calculated to apply Equation 13. This can be achieved by differentiating Equation 10 with respect to latent heat of vaporization of saline water and applying Equation 12. The average uncertainty obtained for the single-stage solar distiller and multi-stage solar distiller is ±0.052 kJ and ±0.105 kJ, respectively. Applying Equation 13, the uncertainty for the efficiency of the single-stage solar distiller and the multi-stage system was found to be ±0.00181 and ±0.00233, respectively, which is also equivalent to ±0.77% and ±0.59%.
Unit cost can be one way of evaluating the economic feasibility of a system. The total amount spent on fabricating each system is recorded as the cost principle, with 10% of the total cost being considered its salvage value. Assuming the life span of both systems to be 15 years and neglecting the interest rate for the proposed system, the capital recovery factor (CRF) and sink fund factor (SFF) will have a constant number of 0.117 and 0.017, respectively. The annual first cost can be calculated by multiplying the CRF with the cost principle, while the annual salvage value is calculated by multiplying the SFF with its salvage value. Simply multiplying the annual first cost by 0.15 will obtain the amount of annual maintenance cost. The annual cost can then be calculated by adding the annual first cost and annual maintenance cost and then subtracting with the annual salvage value. The formula for calculating the cost per unit liter is simply by dividing the annual cost to the annual yield obtained by the solar distiller. The unit cost of the first stage is calculated to be approximately $0.255/L which is also equivalent to $0.0638/kg/m2. The multi-stage system will have a unit cost of $0.227/L or $0.0182/kg/m2. The results of the unit cost are shown in Table 2.
The unit cost obtained can be compared with those from other designs. A solar distillation design that applies a thermoelectric cooling system on a double-glazed solar distiller was reported to have a cost per unit liter per square meter of $0.103/kg/m2 and with modifications, the cost could be lowered to $0.0972/kg/m2 (Khanmohammadi et al., 2022). The multi-stage solar distiller with an expansion nozzle designed by Jubran et al. (2000) has the lowest unit cost of only $0.007/L. However, the cost was adjusted in large-scale implementations, as the collector area used in the calculation was 720 m2. This does in fact lower the cost per unit liter since the calculation does not consider the area of the collector but the average daily yield of a system, which skews the unit cost to become this low. A better comparison would be using the cost per kilogram per square meter.
A new multi-stage system that combines a single-stage solar distiller to a three-stage distiller has been built. This new system also integrates the technology of the Fresnel lens as a cheaper alternative to that of the CSP technology that has been designed and tested under the Malaysian weather. Throughout the study, experiments were conducted, and the results were recorded separately between the single-stage solar distiller and multi-stage system. The study revealed that the mass of yield obtained per day in Malaysia can be lower than that obtained per day in other countries, especially from the Middle East. However, the efficiency of both systems remains acceptable since the total amount of heat supplied is also lower. When considering the cost per unit liter, the proposed multi-stage system has a lower unit cost when compared to other designs proposed by previous researchers. This is mainly due to the inexpensive materials used when designing the multi-stage system. In addition to this, the usage of the Fresnel lens as an alternative to the expensive CSP technology greatly reduced the total cost. The findings can be summarized as follows:
• The total amount of freshwater produced in the first stage of the solar distiller is calculated to be 209 mL/day or 0.836 kg/m2 day, which is 9.1% lesser than the theoretical yield calculated.
• As for the multi-stage system, the distribution of yield obtained can be seen as 56.0%, 29.7%, and 14.3% for stage 2, stage 3, and stage 4, respectively, resulting in a total of 91 mL/day or 1.138 kg/m2 day and is 7.2% lesser than the theoretical yield.
• The efficiency of the first-stage and multi-stage solar distiller is also calculated to be 23.5% with a productivity of 0.1036 g/kJ and 39.5% with 0.164 g/kJ, respectively.
• The uncertainty for the efficiency of the single-stage distiller and multi-stage solar distiller is calculated to be ±0.00181 and ±0.00233, respectively, which is also equivalent to ±0.77% and ±0.59%.
• Considering the economic aspects of the combined system, the unit costs for both systems have been calculated, which is $0.255/L or $0.0638/kg/m2 for the first-stage and $0.227/L or $0.0182/kg/m2 for the multi-stage system.
The following are recommendations for future work to address the current limitation of this research.
• Considering other inclination angles, depths, stages, and heights to find out the optimum design that is suitable for Malaysian weather.
• Perform the testing of a prototype during months that are hotter with ample solar irradiation.
• Usage of a phase change material can be explored especially on the multi-stage solar distiller.
The original contributions presented in the study are included in the article/Supplementary Material; further inquiries can be directed to the corresponding author.
JY: conceptualization, methodology, formal analysis, validation, and data curation. RB: formal analysis, validation, funding resources, editing, and supervision. CK: review analysis, editing, and co-supervision. SL: review analysis and editing. All authors contributed to the article and approved the submitted version.
The study has been funded by Ministry of Higher Education (MOHE), Malaysia through Fundamental Research Grant Scheme Grant (FRGS/1/2021/TK0/UTAR/02/13) and UTAR internal grant UTARRF (IPSR/RMC/UTARRF/2021-C1/R02).
The authors would like to thank the Universiti Tunku Abdul Rahman for funding this project and providing facilities and support. The authors would also like to thank the Department of Safety and Security from UTAR for providing access to the rooftop of the UTAR KB building during the testing of the prototype. The authors are greatly indebted to Lee Kong Chian Faculty of Engineering and Science, and the Center for Sustainable Mobility Technologies (CSMT), University Tunku Abdul Rahman, Sungai Long Campus.
The authors declare that the research was conducted in the absence of any commercial or financial relationships that could be construed as a potential conflict of interest.
All claims expressed in this article are solely those of the authors and do not necessarily represent those of their affiliated organizations, or those of the publisher, editors, and reviewers. Any product that may be evaluated in this article, or claim that may be made by its manufacturer, is not guaranteed or endorsed by the publisher.
Abdessemed, A., Bougriou, C., Guerraiche, D., and Abachi, R. (2019). Effects of tray shape of a multi-stage solar still coupled to a parabolic concentrating solar collector in Algeria. Renew. Energy 132, 1134–1140. doi:10.1016/j.renene.2018.08.074
Abdullah, A. S., Omara, Z., Essa, F., Younes, M., Shanmugan, S., Abdelgaied, M., et al. (2021). Improving the performance of trays solar still using wick corrugated absorber, nano-enhanced phase change material and photovoltaics-powered heaters. J. Energy Storage 40, 102782. doi:10.1016/j.est.2021.102782
Abed, F. M. (2018). Design and fabrication of a multi-stage solar still with three focal concentric collectors. J. Sol. Energy Eng. Trans. ASME 140 (4), 1–9. doi:10.1115/1.4039351
Abujazar, M. S. S., Fatihah, S., Ibrahim, I. A., Kabeel, A., and Sharil, S. (2018b). Productivity modelling of a developed inclined stepped solar still system based on actual performance and using a cascaded forward neural network model. J. Clean. Prod. 170, 147–159. doi:10.1016/j.jclepro.2017.09.092
Abujazar, M. S. S., Fatihah, S., Lotfy, E., Kabeel, A., and Sharil, S. (2018a). Performance evaluation of inclined copper-stepped solar still in a wet tropical climate. Desalination 425, 94–103. doi:10.1016/j.desal.2017.10.022
Ahmed, H., Najib, A., Zaidi, A. A., Naseer, M. N., and Kim, B. (2022). Modeling, design optimization and field testing of a solar still with corrugated absorber plate and phase change material for Karachi weather conditions. Energy Rep. 8, 11530–11546. doi:10.1016/j.egyr.2022.08.276
Ahmed, M. I., Hrairi, M., and Ismail, A. F. (2009). On the characteristics of multi-stage evacuated solar distillation. Renew. Energy 34 (6), 1471–1478. doi:10.1016/j.renene.2008.10.029
Alduchov, O. A., and Eskridge, R. E. (1996). Improved magnus form approximation of saturation vapor pressure. J. Appl. Meteorol. Climatol., 601–609. doi:10.1175/1520-0450(1996)035<0601:IMFAOS>2.0.CO;2
Amiri, H. (2022). Enhancing the stepped solar still performance using a built-in passive condenser. Sol. Energy 248, 88–102. doi:10.1016/j.solener.2022.11.006
Badusha, R., and Arjunan, T. V. (2013). Performance analysis of single slope solar still performance analysis of single slope solar still. Int. J. Emerg. Technol. Adv. Eng. 3 (3), 66–72.
Chen, W., and Xie, G. (2022). Performance of multi-stage tubular solar still operating under vacuum. SSRN Electron. J. 201, 34–46. doi:10.2139/ssrn.4084397
Choong, W. S., Ho, Z. Y., and Bahar, R. (2020). Solar desalination using Fresnel lens as concentrated solar power device: An experimental study in tropical climate. Front. Energy Res. 8 (1-8). doi:10.3389/fenrg.2020.565542
Demeke Agonafer, T. (2020). Actual practice on solar water distillation technology and clean water access crisis in north shoa-Ethiopia: A detailed review. Int. J. Sci. Res. (IJSR) 9 (6), 1884–1892. doi:10.21275/sr20627194904
Hameed, H. G. (2022). Experimentally evaluating the performance of single slope solar still with glass cover cooling and square cross-section hollow fins. Case Stud. Therm. Eng. 40, 102547. doi:10.1016/j.csite.2022.102547
Henderson-Sellers, B. (1984). A new formula for latent heat of vaporization of water as a function of temperature. Q. J. R. Meteorological Soc. 110 (466), 1186–1190. doi:10.1002/qj.49711046626
Kabeel, A. E., Sathyamurthy, R., Sharshir, S. W., Muthumanokar, A., Panchal, H., Prakash, N., et al. (2019). Effect of water depth on a novel absorber plate of pyramid solar still coated with TiO 2 nano black paint. J. Clean. Prod. 213, 185–191. doi:10.1016/j.jclepro.2018.12.185
Khanmohammadi, S., Khanjani, S., and Musharavati, F. (2022). Experimental study and economic examination of double-glazed solar still desalination with a thermoelectric cooling system. Sustain. Energy Technol. Assessments 54, 102854. doi:10.1016/j.seta.2022.102854
Kumar, D., Himanshu, P., and Ahmad, Z. (2013). Performance analysis of single slope solar still performance analysis of single slope solar still. Int. J. Emerging Technol. Adv. Eng. 3 (3), 66–72.
Jubran, B. A., Ahmed, M. I., Ismail, A. F., and Abakar, Y. A. (2000). Numerical modelling of a multi-stage solar still. Energy Convers. Manag. 41 (11), 1107–1121. doi:10.1016/S0196-8904(99)00157-0
Lal, R. K., Yadav, S. V. P., Dwivedi, J., and Dwivedi, H. (2017). Comparative Performance evaluation of an active/passive solar Distillation system. Mater. Today Proc. 4, 2822–2831. doi:10.1016/j.matpr.2017.02.162
Mallouh, M. A., AbdelMeguid, H., and Salah, M. (2022). A comprehensive comparison and control for different solar water heating system configurations. Eng. Sci. Technol. Int. J. 35, 101210. doi:10.1016/j.jestch.2022.101210
Reddy, K. S., Kumar, K. R., O'Donovan, T. S., and Mallick, T. (2012). Performance analysis of an evacuated multi-stage solar water desalination system. Desalination 288, 80–92. doi:10.1016/j.desal.2011.12.016
Shah, R., Makwana, M., Makwana, N., and Desai, R. (2022). Perfromance analysis of black gravel solar still. Mater. Today Proc. 72, 1000–1006. doi:10.1016/j.matpr.2022.09.115
Shahzad, M. W., Burhan, M., Ang, L., and Ng, K. C. (2017). Energy-water-environment nexus underpinning future desalination sustainability. Desalination 413, 52–64. doi:10.1016/j.desal.2017.03.009
Shahzad, M. W., Burhan, M., and Ng, K. C. (2019). A standard primary energy approach for comparing desalination processes. npj Clean. Water 2 (1), 1–7. doi:10.1038/s41545-018-0028-4
Shahzad, M. W., Thu, K., Kim, Y. d., and Ng, K. C. (2015). An experimental investigation on MEDAD hybrid desalination cycle. Appl. Energy 148, 273–281. doi:10.1016/j.apenergy.2015.03.062
Sharqawy, M. H., Lienhard, J. H., and Zubair, S. M. (2010). Thermophysical properties of seawater: A review of existing correlations and data. Desalination Water Treat. 16 (1–3), 354–380. doi:10.5004/dwt.2010.1079
Sharshir, S. W., Rozza, M., Elsharkawy, M., Youns, M., Abou-Taleb, F., and Kabeel, A. (2022). Performance evaluation of a modified pyramid solar still employing wick, reflectors, glass cooling and TiO2 nanomaterial. Desalination 539, 115939. doi:10.1016/j.desal.2022.115939
Wu, G., Zheng, H., Ma, X., Kutlu, C., and Su, Y. (2017). Experimental investigation of a multi-stage humidification-dehumidification desalination system heated directly by a cylindrical Fresnel lens solar concentrator. Energy Convers. Manag. 143, 241–251. doi:10.1016/j.enconman.2017.04.011
Younas, O., Banat, F., and Islam, D. (2016). Seasonal behavior and techno economical analysis of a multi-stage solar still coupled with a point-focus Fresnel lens. Desalination Water Treat. 57 (11), 4796–4809. doi:10.1080/19443994.2014.1001443
Keywords: solar energy, solar desalination, multi-stage solar distiller, sunlight concentration, Fresnel lens, freshwater (health/environment), water treatment, energy efficiency
Citation: Yeang JYK, Bahar R, Koo CH and Lee SS (2023) Performance evaluation of a multi-stage solar distiller associated with Fresnel lens in Malaysian weather. Front. Energy Res. 11:1137941. doi: 10.3389/fenrg.2023.1137941
Received: 05 January 2023; Accepted: 30 May 2023;
Published: 15 June 2023.
Edited by:
Qian Chen, Tsinghua University, ChinaReviewed by:
Muhammad Wakil Shahzad, Northumbria University, United KingdomCopyright © 2023 Yeang, Bahar, Koo and Lee. This is an open-access article distributed under the terms of the Creative Commons Attribution License (CC BY). The use, distribution or reproduction in other forums is permitted, provided the original author(s) and the copyright owner(s) are credited and that the original publication in this journal is cited, in accordance with accepted academic practice. No use, distribution or reproduction is permitted which does not comply with these terms.
*Correspondence: Rubina Bahar, cnViaW5hQHV0YXIuZWR1Lm15
Disclaimer: All claims expressed in this article are solely those of the authors and do not necessarily represent those of their affiliated organizations, or those of the publisher, the editors and the reviewers. Any product that may be evaluated in this article or claim that may be made by its manufacturer is not guaranteed or endorsed by the publisher.
Research integrity at Frontiers
Learn more about the work of our research integrity team to safeguard the quality of each article we publish.