- 1School of Engineering and Technology, Central Queensland University, Rockhampton, QLD, Australia
- 2Centre for Technology in Water and Wastewater, School of Civil and Environmental Engineering, University of Technology Sydney, Ultimo, NSW, Australia
- 3Mechanical Engineering Department, Prince Mohammad Bin Fahd University, Al Khobar, Saudi Arabia
- 4Science and Math Program, Asian University for Women, Chattogram, Bangladesh
- 5Mechanical Engineering Department, College of Engineering, King Khalid University, Abha, Saudi Arabia
Microalgae are a vital resource for the coming years to address the concern of decrease in oil reserves and the negative impacts of fossil fuels on the environment. Their utilization is crucial for a wide range of industrial applications. Depending on the strain, microalgae contain a variety of chemical components and can be treated biochemically or thermochemically. This review thus focuses on the biochemical mechanisms that are used to convert algal biomass into sustainable fuel, including the challenges and potential of those processes. Microalgae have been shown to be a viable third-generation alternative to conventional biofuel feedstocks. The optimum production of biofuel depends on the proper selection of microalgae species based on their lipid, carbohydrate, and protein content in order to produce high-quality, sustainable biofuel. Nannochloropsis gaditana can contribute to a maximum biodiesel yield of 96.47%, whereas Nannochloropsis oculata can produce the least (25%) through the biochemical process of transesterification. Higher yields of microalgae-derived gaseous, solid and liquid fuels can be achieved by pre-treating microalgal biomass and then employing bioconversion processes such as photo-fermentation and hydrothermal carbonization.
1 Introduction
The pressing demand for energy, the diminishing sources of fossil fuels, and the mounting environmental concerns all call for a promising alternative fuel including biofuel (biodiesel, biomethane, bioethanol etc.) (Mofijur et al., 2013a; Chuah et al., 2016; Chuah et al., 2017). Microalgae, as a feedstock for biofuels have demonstrated a significant amount of potential. This is as a result of the fact that it matures with copious mass creation in a matter of days as opposed to terrestrial crops and plants, which can take anything from a few months to many years to reach this stage of development (Wang et al., 2007; Yu et al., 2017; Chia et al., 2018). The primary benefits of using biofuels from the microalgae are that they do not require the use of potable water, they can be grown on land that is not suitable for agriculture, they require only simple nutrients like CO2 and sunlight, they do not require the use of herbicides or pesticides during cultivation, they can use carbon-neutral feedstock, and they can produce high-quality pyrolytic materials (Singh et al., 2011; Lee and Ofori-Boateng, 2013; Debiagi et al., 2017; Chia et al., 2018; Gan et al., 2018). In addition, the high photosynthetic efficiency of microalgae, which ranges from 6% to 8%, when compared to the photosynthetic efficiency of terrestrial biomass, which ranges from 1.8% to 2.2%, as well as the high CO2 fixation by microalgae, makes them excellent biofuel feedstocks and natural environment remediators (Kumar et al., 2017; Yu et al., 2017; Yu et al., 2018; Brigljević et al., 2019). In addition to this, it has been observed that the bulk of microalgae is composed of more than 70% fat when measured based on their dry weight (Liang et al., 2010). Because of their high lipid content, microalgae are an important component in the manufacture of biofuels. Cultivating microalgae can, over the course of time, result in both the production of economically viable feedstock and the decrease of greenhouse gases.
The cultivation of microalgae for commercial purposes takes place in a particular sort of bioreactor that creates ideal growth conditions, resulting in high levels of output. At this time, open raceway ponds and closed photobioreactors are both utilised for the production of microalgae, and the selection of one or the other is determined by the particular requirements. Raceway ponds that are open to the environment are frequently used for the treatment of wastewater, whereas closed photobioreactors are typically utilised for the production of pharmaceutical and value-added goods. The quantity of biofuel produced and the quality of that biofuel are both highly dependent on a number of parameters, including the properties of the microalgae, the conditions of the production process, the catalyst type and dosage, and the configuration of the reactor (Azizi et al., 2018). The effect of these elements should be thoroughly investigated and modified in order to get the best output and quality of biofuels from microalgae. This will allow to reach goals. Few researchers already studied different microalgae conversion processes (Rowbotham et al., 2012; Marcilla et al., 2013; Chen et al., 2015a; Elliott, 2016). A large number of macromolecular components serve as substrate for the biochemical conversion process, which is one of these processes. As a result, the benefit of using microalgae in the biochemical conversion process is that it results in a reduced level of process complexity while simultaneously providing an energy form together with a mineralized digestate that is rich in beneficial nutrients. Thus, the purpose of this mini review is to highlight the utilisation of different biochemical processes and the challenges and future prospects of those processes to convert algal biomass into sustainable fuel.
2 Conversion technologies of microalgae into biofuels
Converting microalgae into biofuels can be accomplished through the use of a variety of different technologies. As shown in Figure 1, these conversion methods can be categorised into two primary categories: biochemical and thermochemical. Because certain biomass feedstocks include high levels of lipids, it is possible to extract those lipids using either physical or chemical separation methods. After that, the triglyceride portion of the extracted lipids can be converted into biodiesel by using the transesterification process. The biochemical conversion processes also result in the production of biogas or ethanol, and the conversion itself is a somewhat slow process, typically lasting hours, days, or weeks depending on the kind of feedstock (Bridgwater, 2010). Thermochemical conversion including pyrolysis and gasification process, on the other hand, results in the production of several products in a relatively short amount of time and the quality of the products that are intended for consumption may frequently be improved with the application of catalysts.
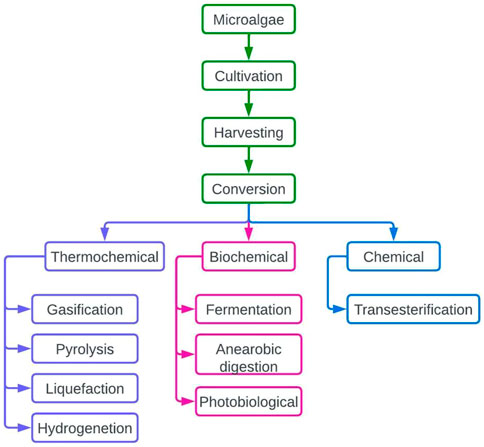
FIGURE 1. Various conversion technologies for biofuel production, modified from (Suali and Sarbatly, 2012).
One of the most important steps in ensuring that the production of biofuel is both economically viable and environmentally sustainable is making the choice of the appropriate conversion technology. At this point in time, there are no obvious advantages that can be demonstrated between the thermochemical and biochemical routes. However, according to a review that was done by Sims and colleagues (Sims et al., 2010), thermochemical conversion is regarded as being more favourable. The properties of the feedstock are one of the most significant challenges for biochemical conversion. Feedstock modifications, such as those involving pre-treatment processes, call for a significant investment of capital, which, in turn, drives up production costs. In addition, the efficiency with which biomass may be converted into biofuel by using biochemical processes is quite low. In addition, the biochemical pathway could only result in the production of a single type of end product for each application, in contrast to the thermochemical pathway, which may result in the production of multiple types of end products from a single operation. In the next subsections, a concise discussion of each kind of conversion technology is presented.
2.1 Chemical and biochemical conversion technologies of microalgae
2.1.1 Transesterification of microalgae
The typical process that is utilised to convert the triglyceride found in microalgal lipid into biodiesel is called transesterification (Sinha et al., 2008; Rajan and Senthilkumar, 2009). This is the reaction that occurs when TG is combined with an acyl-acceptor (Fan and Burton, 2009; Helwani et al., 2009). Acyl-acceptors might come in the form of carboxylic acids, alcohols, or even another ester. When alcohol is used as the acyl-acceptor in transesterification, glycerol is produced; however, triacylglycerol is produced when ester is utilised (Ma and Hanna, 1999; Dubé et al., 2007; Ranganathan et al., 2008; Sharma et al., 2008; Robles-Medina et al., 2009). According to the principles of stoichiometry, 3 mol of alcohol are needed for every mole of TG. In most cases, however, a greater molar ratio of alcohol is employed in order to accomplish the highest possible level of biodiesel generation. This molar ratio changes depending on the type of feedstock that is used, the type of catalyst that is used, the temperature, and the amount of time that the reaction takes. Methanol, ethanol, and propanol are the three types of alcohol that are utilised most frequently (Mofijur et al., 2013b; Mofijur et al., 2014; Rozina et al., 2022). In point of fact, the yield of biodiesel is not affected by the type of alcohol that is used; rather, the selection of alcohol is determined by cost (Al-Zuhair et al., 2007; Sinha et al., 2008).
The process of transesterification that makes use of a catalyst is referred to as a catalytic transesterification process, whereas the process that does not make use of a catalyst is referred to as a non-catalytic transesterification process (Gerpen, 2005; Meher et al., 2006; Marchetti et al., 2007). In addition, a catalytic process might be one of two categories: homogeneous or heterogenous, and this classification is determined by the catalyst that is being employed (Fjerbaek et al., 2009; Lin et al., 2009). It has been reported that the heating value of biodiesel made from microalgae is 41 MJ/kg (Xu et al., 2006) and this value is in accordance with the ASTM D6751 standards (Li et al., 2007). According to a number of studies, the enzyme that comes from lipase is fantastic for the process of converting a variety of vegetable oils to methyl ester. The transesterification process in generally take place at 35–50°C at atmospheric pressure and a molar ratio of 3:1–6:1 (Fjerbaek et al., 2009; Mata et al., 2010).
2.1.2 Fermentation
The production of ethanol from organic substrates like sucrose, bagasse, cellulose, or starch is the goal of the metabolic process known as fermentation (McKendry, 2002a; Lee and Lee, 2016). The fermentation of starch, sugar, and cellulose found in microalgal biomass is the primary route that leads to the synthesis of ethanol from microalgae (Kim et al., 2011), with starch making up the majority of the carbohydrate content and having a potential range of up to 60 dw% depending on the culture conditions (Nahak et al., 2011; (Brányiková et al., 2011). Controlling the amount of nitrogen or iron present during culture of microalgae can result in increased starch production (Liu et al., 2008; Dragone et al., 2011). In its most basic form, fermentation can be broken down into two categories: (Chuah et al., 2016) aerobic and (Chuah et al., 2017) anaerobic, with the distinction between the two based on whether or not the process requires oxygen.
Before microalgae biomass or starch can be fermented, it must first undergo pretreatment in the form of milling, which turns the biomass or starch into sugars. Next, the sugars are combined with Saccharomyces cerevisiae yeast and water in a fermenter for the fermentation process (Demirbaş, 2001). After fermentation, the diluted alcohol that was generated is put into a holding tank where it waits to be distilled. The ethanol concentration in the diluted alcohol product typically ranges from 10% to 15% (McKendry, 2002a). The product can be purified through the distillation process, which also results in the production of concentrated ethanol that has a purity level of 95% and is therefore appropriate for use as a supplement or substitute for transport fuel (McKendry, 2002b). This fermentation process uses less energy because the CO2 produced can be used to grow microalgae and the leftover material can be recycled as a medium for microalgae growth or used as animal feed (McKendry, 2002a; Milledge et al., 2014).
There are four primary processes that take place during the fermentation of ethanol. Ethanol has replaced gasoline in many vehicles over the last decade because to its ability to reduce emissions of CO2 and many harmful pollutants (CO, NO) during combustion (Demirbas, 2008). As a transportation fuel, bioethanol is widely available on the market and is utilised often (Walker, 2010). Nevertheless, bioethanol has a few downsides, including the fact that it is caustic, has a low energy value, is hazardous to the ecology, and has a low vapour pressure. However, in order to achieve long-term benefits and sustainability, additional efforts are required to upgrade bioethanol to a higher quality fuel that can stand alone and is compatible with fossil fuel. At the moment, bioethanol is ready to be integrated with the fuel that is already being used for transportation. C. vulgaris is a type of microalgae that is excellent for the production of bioethanol. This species has a high starch content (about 37%), which enables it to achieve up to 65% of ethanol conversion (Adeniyi et al., 2018). In addition to Chlamydomonas, Dunaliella, Scenedesmus, and Spirulina, which all contain more than fifty percent starch, there are also species that are thought to be promising candidates for the synthesis of bioethanol (Hu et al., 2012). Table 1 provides a summary of the findings of studies conducted on the production of biofuel using various biochemical processes.
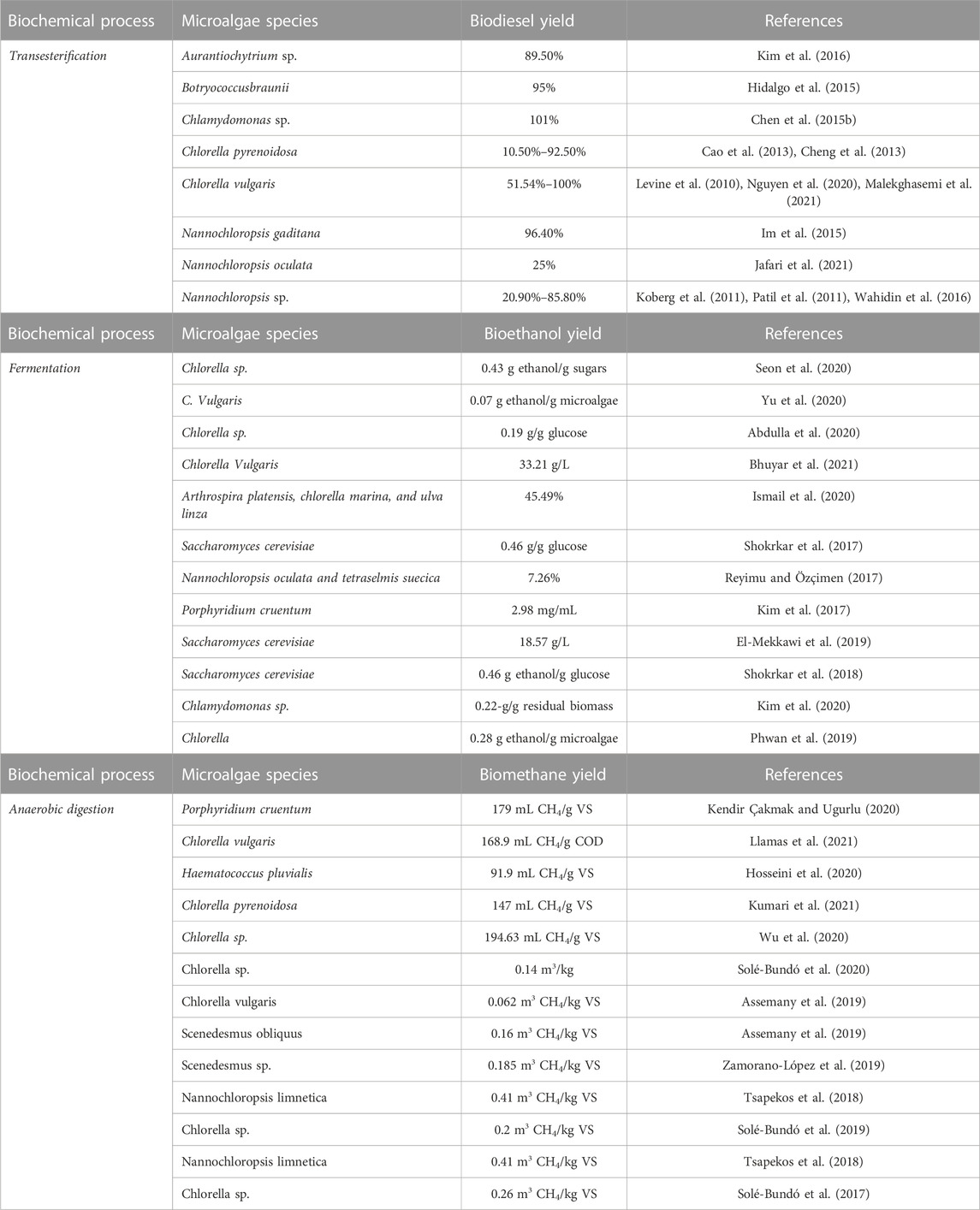
TABLE 1. Summary of the findings of studies conducted on the production of biofuel from microalgae using various biochemical processes.
2.2 Anaerobic digestion of microalgae
When the biomass to be converted has a high-water content, the biochemical process of anaerobic digestion becomes crucial. In this process, biogas, NH3, and CO2 are produced, along with other undesirable gases like H2S from the biomass’s organic content (Mahmudul et al., 2021). Microalgae biomass, both pre-treated and untreated, and lipid extraction leftovers can be employed to generate methane (Siddiki et al., 2021). Steps of hydrolysis, fermentation, and methanogenesis follow one another in the digestive process. First, the organic matter is broken down into soluble sugars, and then the bacteria ferment those sugars to produce methane and carbon dioxide. As a result of the digesting process, a gas is produced that contains 20%–40% of the lower heating value of the feed in terms of energy. To get the best possible end result from the anaerobic digestion process, it is necessary to tune the hydraulic retention, organic loading parameters, pH, and temperature (Ahmed et al., 2021). The majority of current biogas research is devoted to improving wastewater treatment and finding ways to integrate biogas generation with other algal-based manufacturing techniques. In addition to lowering odours, hazardous gas emissions, and oxygen demand in wastewater, anaerobic digestion of microalgae has a few other advantages.
3 Challenges of chemical/biochemical conversion of microalgae
Capital-intensive efforts to convert algae into biofuels have been advocated for on the basis of the plant’s rapid expansion, manageable size, and high oil content. There are a number of challenges that need to be addressed before algae can emerge as a financially feasible substrate to replace petroleum and, thus, decrease CO2 release. These challenges range from where and how to cultivate these algae to advancements in oil extraction and fuel processing (Hannon et al., 2010). Some of the challenges of converting microalgae into biofuel through chemical/biochemical conversion processes (Siddiki et al., 2022) are discussed as follows.
• The process of conversion is greatly governed by environmental factors.
• The products made through biochemical processes are more selective and can be processed at lower temperatures.
• Ideally suitable for processing algal biomass with a moisture content higher than 50%.
• The first step in this process of transformation is the pre-treatment of the feedstock.
• The slower reaction rate necessitates longer residence times for product formation.
• Biological conversion is a major bottleneck that reduces productivity. More money would need to be spent on infrastructure upgrades, like a larger reactor, to accommodate the increased production.
• To achieve any kind of feasible findings, the use of bacteria, enzymes, or chemicals is essential. Sludge, an undesirable waste product, is produced and needs to be treated in some way.
• Due to the biological nature of the conversion process, the amount of production is relatively low.
• In most cases, limited to a few products and additional microorganisms are required to produce additional products.
4 Future prospects of chemical/biochemical conversion of microalgae
There are challenges, but at the current rate of development, biofuels derived from microalgae may be capable of competing with existing energy sources within decades. Industrial-scale chemical/biochemical conversion of microalgae into biofuels will require concerted efforts to optimise strains and increase production volumes. As a result, on the basis of the most recent state of the art, the following are some proposals for potential future developments.
• The productivity of a specific strain of microalgae can be increased to some degree by updating the design of the photobioreactor, regulating the physical conditions, giving growth nutrients, and adding metal ions. Further progress can be made by improving strains utilising recombinant DNA technology, which could lead to enhanced metabolic pathways that concentrate more biofuel-grade compounds.
• In order to make the process of algal harvesting more efficient, it is possible to make use of cutting-edge methods like fungi-mediated harvesting and the application of tailored nanoparticles. This will make it easier to separate the farmed microalgae from the broth in which they were grown.
• The amount of biofuel that can be extracted from harvested microalgae depends on the pre-treatment processes that are employed. Additionally, the linking of more than one pre-treatment processes has emerged as a promising strategy; nevertheless, this technique requires additional study before it can be used at industrial scales.
• The application of physicochemical and physical pre-treatments in combination have shown the enhancement of carbohydrate release from algal biomass.
• The improvement of efficiency with which enzymes transform sugar into its constituent molecules.
• The enhancement of fermentation efficiency through nutritional supplementation of both liquid and gaseous media.
5 Environmental viability assessment of microalgal biofuels
Microalgae are able to withstand and absorb significantly higher quantities of CO2 than terrestrial plants. Because of this, microalgae are able to utilise CO2 released from petroleum-based power stations or other industrial sources, which can reduce the production of glasshouse gas (Nigam and Singh, 2011). It is possible to transform the entire algal biomass as well as extracts of algal oil into various forms of fuel. Because algae are able to extract nutrients like nitrogen and phosphorus from a wide range of wastewater sources, they are able to provide a sustained bioremediation of these wastewater sources, which has benefits for both the environment and the economy (Shilton et al., 2008). Carbon credits can be generated through the use of algal biofuels thanks to their ability to connect the generation of CO2-free fuel with the sequestration of CO2 from other power industries (Dismukes et al., 2008). When compared to terrestrial feedstock crops, algae have a number of advantages that make them more desirable. Despite the fact that their development requirements are comparable to those of terrestrial plants, they make very effective use of the resources available to them and as a result, they have great productivity despite using a relatively small amount of water (Brown and Zeiler, 1993; Chelf et al., 1993). The algae take in the additional CO2 that is present, storing it as biomass as a result of their accelerated rate of development. Microalgae offer other benefits in addition to their potential for capturing CO2 from fossil fuel power plants. This is because direct CO2 capture procedures are preferred to indirect CO2 capture processes. Passing flue gases directly through a media where microalgae can grow is an efficient technique to capture the CO2 in such streams (Benemann, 1997). While it’s likely to be extremely expensive to apply CO2 directly to terrestrial crops via enclosures, an indirect technique that uses flue gases to stimulate land species could be cost-effective while being much less direct and less efficient (Packer, 2009).
6 Conclusion
Increased interest in microalgae biofuel production has been driven by rising concerns about the use of fossil fuels, greenhouse emissions, and the conflict between food and fuel when it comes to conventional biofuel sources. This study reviewed the biochemical mechanisms used to convert algal biomass into sustainable fuel, as well as its challenges and potential. Microalgae were found to be the largest renewable, clean energy resource with the potential to replace fossil fuels. Cultured microalgae in a photobioreactor could meet the biomass requirements for continuous biofuel generation in addition to repairing CO2 emissions and treating wastewater. The best method for converting wet biomass into biofuel is the biochemical conversion process provided that the biomass’s moisture content is higher than 50%. Although microalgae hold great potential, neither upstream nor downstream methods now available can completely tap into this potential. Consecutive growth of microalgae requires extremely efficient and cost-effective processing in order to provide sustainable and high-quality biofuel production and reduce reliance on fossil fuels. However, there is still a significant knowledge gap in microalgal biofuel research since it is difficult to compare the chemical composition of different feedstocks to that of synthesized products. To bridge the gaps for future development, more comprehensive characterisation studies are needed.
Author contributions
ZR: Draft writing, MM: Draft writing, MH: Draft writing, SA: Review and editing, IB: Supervision and funding, TK: Review editing and funding.
Acknowledgments
The authors extend their appreciation to the Deanship of Scientific Research at King Khalid University, Saudi Arabia for funding this work through the large groups project under grant number RGP.2/ 235/43.
Conflict of interest
The authors declare that the research was conducted in the absence of any commercial or financial relationships that could be construed as a potential conflict of interest.
Publisher’s note
All claims expressed in this article are solely those of the authors and do not necessarily represent those of their affiliated organizations, or those of the publisher, the editors and the reviewers. Any product that may be evaluated in this article, or claim that may be made by its manufacturer, is not guaranteed or endorsed by the publisher.
References
Abdulla, R., King, T. K., Jambo, S. A., and Faik, A. A. (2020). “Microalgae chlorella as a sustainable feedstock for bioethanol production,” in Green engineering for campus sustainability. Editor A. Z. Yaser (Singapore: Springer Singapore), 81–103.
Adeniyi, O. M., Azimov, U., and Burluka, A. (2018). Algae biofuel: Current status and future applications. Renew. Sustain. energy Rev. 90, 316–335. doi:10.1016/j.rser.2018.03.067
Ahmed, S. F., Mofijur, M., Tarannum, K., Chowdhury, A. T., Rafa, N., Nuzhat, S., et al. (2021). Biogas upgrading, economy and utilization: A review. Environ. Chem. Lett. 19 (6), 4137–4164. doi:10.1007/s10311-021-01292-x
Al-Zuhair, S., Ling, F. W., and Jun, L. S. (2007). Proposed kinetic mechanism of the production of biodiesel from palm oil using lipase. Process Biochem. 42 (6), 951–960. doi:10.1016/j.procbio.2007.03.002
Assemany, P., de Paula Marques, I., Calijuri, M. L., and Reis, A. (2019). Complementarity of substrates in anaerobic digestion of wastewater grown algal biomass. Waste Biomass Valorization 11, 5759–5770. doi:10.1007/s12649-019-00875-8
Azizi, K., Moraveji, M. K., and Najafabadi, H. A. (2018). A review on bio-fuel production from microalgal biomass by using pyrolysis method. Renew. Sustain. Energy Rev. 82, 3046–3059. doi:10.1016/j.rser.2017.10.033
Benemann, J. R. (1997). CO2 mitigation with microalgae systems. Energy Convers. Manag. 38, S475–S479. doi:10.1016/s0196-8904(96)00313-5
Bhuyar, P., Trejo, M., Dussadee, N., Unpaprom, Y., Ramaraj, R., and Whangchai, K. (2021). Microalgae cultivation in wastewater effluent from tilapia culture pond for enhanced bioethanol production. Water Sci. Technol. 84, 2686–2694.
Brányiková, I., Maršálková, B., Doucha, J., Brányik, T., Bišová, K., Zachleder, V., et al. (2011). Microalgae—Novel highly efficient starch producers. Biotechnol. Bioeng. 108 (4), 766–776. doi:10.1002/bit.23016
Bridgwater, A. (2010). Fast pyrolysis of biomass for energy and fuels, 146–191. Thermochemical conversion of biomass to liquid fuels and chemicals.
Brigljević, B., Liu, J., and Lim, H. (2019). Green energy from Brown seaweed: Sustainable polygeneration industrial process via fast pyrolysis of S. Japonica combined with the brayton cycle. Energy Convers. Manag. 195, 1244–1254. doi:10.1016/j.enconman.2019.05.103
Brown, L. M., and Zeiler, K. G. (1993). Aquatic biomass and carbon dioxide trapping. Energy Convers. Manag. 34 (9), 1005–1013. doi:10.1016/0196-8904(93)90048-f
Cao, H., Zhang, Z., Wu, X., and Miao, X. (2013). Direct biodiesel production from wet microalgae biomass of chlorella pyrenoidosa through in situ transesterification. BioMed Res. Int. 2013, 1–6. doi:10.1155/2013/930686
Chelf, P., Brown, L. M., and Wyman, C. E. (1993). Aquatic biomass resources and carbon dioxide trapping. Biomass Bioenergy 4 (3), 175–183. doi:10.1016/0961-9534(93)90057-b
Chen, C-L., Huang, C-C., Ho, K-C., Hsiao, P-X., Wu, M-S., and Chang, J-S. (2015). Biodiesel production from wet microalgae feedstock using sequential wet extraction/transesterification and direct transesterification processes. Bioresour. Technol. 194, 179–186. doi:10.1016/j.biortech.2015.07.021
Chen, Y., Wu, Y., Hua, D., Li, C., Harold, M. P., Wang, J., et al. (2015). Thermochemical conversion of low-lipid microalgae for the production of liquid fuels: Challenges and opportunities. RSC Adv. 5 (24), 18673–18701. doi:10.1039/c4ra13359e
Cheng, J., Yu, T., Li, T., Zhou, J. H., and Cen, K. F. (2013). Using wet microalgae for direct biodiesel production via microwave irradiation. Bioresour. Technol. 131, 531–535. doi:10.1016/j.biortech.2013.01.045
Chia, S. R., Ong, H. C., Chew, K. W., Show, P. L., Phang, S-M., Ling, T. C., et al. (2018). Sustainable approaches for algae utilisation in bioenergy production. Renew. energy 129, 838–852. doi:10.1016/j.renene.2017.04.001
Chuah, L. F., Klemeš, J. J., Yusup, S., Bokhari, A., and Akbar, M. M. (2017). A review of cleaner intensification technologies in biodiesel production. J. Clean. Prod. 146, 181–193. doi:10.1016/j.jclepro.2016.05.017
Chuah, L. F., Yusup, S., Abd Aziz, A. R., Bokhari, A., and Abdullah, M. Z. (2016). Cleaner production of methyl ester using waste cooking oil derived from palm olein using a hydrodynamic cavitation reactor. J. Clean. Prod. 112, 4505–4514. doi:10.1016/j.jclepro.2015.06.112
Debiagi, P. E. A., Trinchera, M., Frassoldati, A., Faravelli, T., Vinu, R., and Ranzi, E. (2017). Algae characterization and multistep pyrolysis mechanism. J. Anal. Appl. Pyrolysis 128, 423–436. doi:10.1016/j.jaap.2017.08.007
Demirbas, A. (2008). Biofuels sources, biofuel policy, biofuel economy and global biofuel projections. Energy Convers. Manag. 49 (8), 2106–2116. doi:10.1016/j.enconman.2008.02.020
Demirbaş, A. (2001). Biomass resource facilities and biomass conversion processing for fuels and chemicals. Energy Convers. Manag. 42 (11), 1357–1378. doi:10.1016/s0196-8904(00)00137-0
Dismukes, G. C., Carrieri, D., Bennette, N., Ananyev, G. M., and Posewitz, M. C. (2008). Aquatic phototrophs: Efficient alternatives to land-based crops for biofuels. Curr. Opin. Biotechnol. 19 (3), 235–240. doi:10.1016/j.copbio.2008.05.007
Dragone, G., Fernandes, B. D., Abreu, A. P., Vicente, A. A., and Teixeira, J. A. (2011). Nutrient limitation as a strategy for increasing starch accumulation in microalgae. Appl. energy 88 (10), 3331–3335. doi:10.1016/j.apenergy.2011.03.012
Dubé, M. A., Tremblay, A. Y., and Liu, J. (2007). Biodiesel production using a membrane reactor. Bioresour. Technol. 98 (3), 639–647. doi:10.1016/j.biortech.2006.02.019
El-Mekkawi, S. A., Abdo, S. M., Samhan, F. A., and Ali, G. H. (2019). Optimization of some fermentation conditions for bioethanol production from microalgae using response surface method. Bull. Natl. Res. Centre 43 (1), 164. doi:10.1186/s42269-019-0205-8
Elliott, D. C. (2016). Review of recent reports on process technology for thermochemical conversion of whole algae to liquid fuels. Algal Res. 13, 255–263. doi:10.1016/j.algal.2015.12.002
Fan, X., and Burton, R. (2009). Recent development of biodiesel feedstocks and the applications of glycerol: A review. Open Fuels Energy Sci. J. 2 (1), 100–109. doi:10.2174/1876973x00902010100
Fjerbaek, L., Christensen, K. V., and Norddahl, B. (2009). A review of the current state of biodiesel production using enzymatic transesterification. Biotechnol. Bioeng. 102 (5), 1298–1315. doi:10.1002/bit.22256
Gan, Y. Y., Ong, H. C., Show, P. L., Ling, T. C., Chen, W-H., Yu, K. L., et al. (2018). Torrefaction of microalgal biochar as potential coal fuel and application as bio-adsorbent. Energy Convers. Manag. 165, 152–162. doi:10.1016/j.enconman.2018.03.046
Gerpen, J. V. (2005). Biodiesel processing and production. Fuel Process. Technol. 86 (10), 1097–1107. doi:10.1016/j.fuproc.2004.11.005
Hannon, M., Gimpel, J., Tran, M., Rasala, B., and Mayfield, S. (2010). Biofuels from algae: Challenges and potential. Biofuels 1 (5), 763–784. doi:10.4155/bfs.10.44
Helwani, Z., Othman, M. R., Aziz, N., Fernando, W. J. N., and Kim, J. (2009). Technologies for production of biodiesel focusing on green catalytic techniques: A review. Fuel Process. Technol. 90 (12), 1502–1514. doi:10.1016/j.fuproc.2009.07.016
Hidalgo, P., Ciudad, G., Schober, S., Mittelbach, M., and Navia, R. (2015). Biodiesel synthesis by direct transesterification of microalga Botryococcus braunii with continuous methanol reflux. Bioresour. Technol. 181, 32–39. doi:10.1016/j.biortech.2015.01.047
Hosseini, A., Jazini, M., Mahdieh, M., and Karimi, K. (2020). Efficient superantioxidant and biofuel production from microalga Haematococcus pluvialis via a biorefinery approach. Bioresour. Technol. 306, 123100. doi:10.1016/j.biortech.2020.123100
Hu, J., Yu, F., and Lu, Y. (2012). Application of Fischer–Tropsch synthesis in biomass to liquid conversion. Catalysts 2 (2), 303–326. doi:10.3390/catal2020303
Im, H., Kim, B., and Lee, J. W. (2015). Concurrent production of biodiesel and chemicals through wet in situ transesterification of microalgae. Bioresour. Technol. 193, 386–392. doi:10.1016/j.biortech.2015.06.122
Ismail, M. M., Ismail, G. A., and El-Sheekh, M. M. (2020). Potential assessment of some micro- and macroalgal species for bioethanol and biodiesel production. Energy Sources, Part A Recovery, Util. Environ. Eff., 1–17. doi:10.1080/15567036.2020.1758853
Jafari, A., Esmaeilzadeh, F., Mowla, D., Sadatshojaei, E., Heidari, S., and Wood, D. A. (2021). New insights to direct conversion of wet microalgae impregnated with ethanol to biodiesel exploiting extraction with supercritical carbon dioxide. Fuel 285, 119199. doi:10.1016/j.fuel.2020.119199
Kendir Çakmak, E., and Ugurlu, A. (2020). Enhanced biogas production of red microalgae via enzymatic pretreatment and preliminary economic assessment. Algal Res. 50, 101979. doi:10.1016/j.algal.2020.101979
Kim, E. J., Kim, S., Choi, H-G., and Han, S. J. (2020). Co-production of biodiesel and bioethanol using psychrophilic microalga Chlamydomonas sp. KNM0029C isolated from Arctic sea ice. Biotechnol. Biofuels 13 (1), 20. doi:10.1186/s13068-020-1660-z
Kim, H. M., Oh, C. H., and Bae, H-J. (2017). Comparison of red microalgae (Porphyridium cruentum) culture conditions for bioethanol production. Bioresour. Technol. 233, 44–50. doi:10.1016/j.biortech.2017.02.040
Kim, K. H., Lee, O. K., Kim, C. H., Seo, J-W., Oh, B-R., and Lee, E. Y. (2016). Lipase-catalyzed in-situ biosynthesis of glycerol-free biodiesel from heterotrophic microalgae, Aurantiochytrium sp. KRS101 biomass. Aurantiochytrium Sp. KRS101 biomass. Bioresour. Technol. 211, 472–477. doi:10.1016/j.biortech.2016.03.092
Kim, N-J., Li, H., Jung, K., Chang, H. N., and Lee, P. C. (2011). Ethanol production from marine algal hydrolysates using Escherichia coli KO11. Bioresour. Technol. 102 (16), 7466–7469. doi:10.1016/j.biortech.2011.04.071
Koberg, M., Cohen, M., Ben-Amotz, A., and Gedanken, A. (2011). Bio-diesel production directly from the microalgae biomass of Nannochloropsis by microwave and ultrasound radiation. Bioresour. Technol. 102 (5), 4265–4269. doi:10.1016/j.biortech.2010.12.004
Kumar, G., Shobana, S., Chen, W-H., Bach, Q-V., Kim, S-H., Atabani, A., et al. (2017). A review of thermochemical conversion of microalgal biomass for biofuels: Chemistry and processes. Green Chem. 19 (1), 44–67. doi:10.1039/c6gc01937d
Kumari, P., Varma, A. K., Shankar, R., Thakur, L. S., and Mondal, P. (2021). Phycoremediation of wastewater by Chlorella pyrenoidosa and utilization of its biomass for biogas production. J. Environ. Chem. Eng. 9 (1), 104974. doi:10.1016/j.jece.2020.104974
Lee, K. T., and Ofori-Boateng, C. (2013). Sustainability of biofuel production from oil palm biomass. Springer, 31–74.Biofuels: Production technologies, global profile, and market potentials
Lee, O. K., and Lee, E. Y. (2016). Sustainable production of bioethanol from renewable Brown algae biomass. Biomass Bioenergy 92, 70–75. doi:10.1016/j.biombioe.2016.03.038
Levine, R. B., Pinnarat, T., and Savage, P. E. (2010). Biodiesel production from wet algal biomass through in situ lipid hydrolysis and supercritical transesterification. Energy & Fuels 24, 5235–5243. doi:10.1021/ef1008314
Li, X., Xu, H., and Wu, Q. (2007). Large-scale biodiesel production from microalga Chlorella protothecoides through heterotrophic cultivation in bioreactors. Biotechnol. Bioeng. 98 (4), 764–771. doi:10.1002/bit.21489
Liang, Y., Sarkany, N., Cui, Y., Yesuf, J., Trushenski, J., and Blackburn, J. W. (2010). Use of sweet sorghum juice for lipid production by Schizochytrium limacinum SR21. Bioresour. Technol. 101 (10), 3623–3627. doi:10.1016/j.biortech.2009.12.087
Lin, L., Ying, D., Chaitep, S., and Vittayapadung, S. (2009). Biodiesel production from crude rice bran oil and properties as fuel. Appl. Energy 86 (5), 681–688. doi:10.1016/j.apenergy.2008.06.002
Liu, Z-Y., Wang, G-C., and Zhou, B-C. (2008). Effect of iron on growth and lipid accumulation in Chlorella vulgaris. Bioresour. Technol. 99 (11), 4717–4722. doi:10.1016/j.biortech.2007.09.073
Llamas, M., Greses, S., Tomás-Pejó, E., and González-Fernández, C. (2021). Tuning microbial community in non-conventional two-stage anaerobic bioprocess for microalgae biomass valorization into targeted bioproducts. Bioresour. Technol. 337, 125387. doi:10.1016/j.biortech.2021.125387
Ma, F., and Hanna, M. A. (1999). Biodiesel production: A review1Journal series #12109, agricultural research division, institute of agriculture and natural resources, university of Nebraska–Lincoln.1. Bioresour. Technol. 70 (1), 1–15. doi:10.1016/s0960-8524(99)00025-5
Mahmudul, H. M., Rasul, M. G., Akbar, D., Narayanan, R., and Mofijur, M. (2021). A comprehensive review of the recent development and challenges of a solar-assisted biodigester system. Sci. Total Environ. 753, 141920. doi:10.1016/j.scitotenv.2020.141920
Malekghasemi, S., Kariminia, H-R., Plechkova, N. K., and Ward, V. C. A. (2021). Direct transesterification of wet microalgae to biodiesel using phosphonium carboxylate ionic liquid catalysts. Biomass Bioenergy 150, 106126. doi:10.1016/j.biombioe.2021.106126
Marchetti, J. M., Miguel, V. U., and Errazu, A. F. (2007). Possible methods for biodiesel production. Renew. Sustain. Energy Rev. 11 (6), 1300–1311. doi:10.1016/j.rser.2005.08.006
Marcilla, A., Catalá, L., García-Quesada, J. C., Valdés, F., and Hernández, M. (2013). A review of thermochemical conversion of microalgae. Renew. Sustain. energy Rev. 27, 11–19. doi:10.1016/j.rser.2013.06.032
Mata, T. M., Martins, A. A., and Caetano, N. S. (2010). Microalgae for biodiesel production and other applications: A review. Renew. Sustain. Energy Rev. 14 (1), 217–232. doi:10.1016/j.rser.2009.07.020
McKendry, P. (2002). Energy production from biomass (part 1): Overview of biomass. Bioresour. Technol. 83 (1), 37–46. doi:10.1016/s0960-8524(01)00118-3
McKendry, P. (2002). Energy production from biomass (part 2): Conversion technologies. Bioresour. Technol. 83 (1), 47–54. doi:10.1016/s0960-8524(01)00119-5
Meher, L. C., Vidya Sagar, D., and Naik, S. N. (2006). Technical aspects of biodiesel production by transesterification—A review. Renew. Sustain. Energy Rev. 10 (3), 248–268. doi:10.1016/j.rser.2004.09.002
Milledge, J. J., Smith, B., Dyer, P. W., and Harvey, P. (2014). Macroalgae-derived biofuel: A review of methods of energy extraction from seaweed biomass. Energies 7 (11), 7194–7222. doi:10.3390/en7117194
Mofijur, M., Atabani, A. E., Masjuki, H. H., Kalam, M. A., and Masum, B. M. (2013). A study on the effects of promising edible and non-edible biodiesel feedstocks on engine performance and emissions production: A comparative evaluation. Renew. Sustain. Energy Rev. 23, 391–404. doi:10.1016/j.rser.2013.03.009
Mofijur, M., Masjuki, H. H., Kalam, M. A., and Atabani, A. E. (2013). Evaluation of biodiesel blending, engine performance and emissions characteristics of jatropha curcas methyl ester: Malaysian perspective. Energy 55, 879–887. doi:10.1016/j.energy.2013.02.059
Mofijur, M., Masjuki, H. H., Kalam, M. A., Atabani, A. E., Fattah, I. M. R., and Mobarak, H. M. (2014). Comparative evaluation of performance and emission characteristics of Moringa oleifera and Palm oil based biodiesel in a diesel engine. Industrial Crops Prod. 53, 78–84. doi:10.1016/j.indcrop.2013.12.011
Nahak, S., Nahak, G., Pradhan, I., and Sahu, R. (2011). Bioethanol from marine algae: A solution to global warming problem. J. Appl. Environ. Biol. Sci. 1 (4), 74–80.
Nguyen, T. T., Lam, M. K., Uemura, Y., Mansor, N., Lim, J. W., Show, P. L., et al. (2020). High biodiesel yield from wet microalgae paste via in-situ transesterification: Effect of reaction parameters towards the selectivity of fatty acid esters. Fuel 272, 117718. doi:10.1016/j.fuel.2020.117718
Nigam, P. S., and Singh, A. (2011). Production of liquid biofuels from renewable resources. Prog. Energy Combust. Sci. 37 (1), 52–68. doi:10.1016/j.pecs.2010.01.003
Packer, M. (2009). Algal capture of carbon dioxide; biomass generation as a tool for greenhouse gas mitigation with reference to New Zealand energy strategy and policy. Energy Policy 37 (9), 3428–3437. doi:10.1016/j.enpol.2008.12.025
Patil, P. D., Gude, V. G., Mannarswamy, A., Deng, S., Cooke, P., Munson-McGee, S., et al. (2011). Optimization of direct conversion of wet algae to biodiesel under supercritical methanol conditions. Bioresour. Technol. 102 (1), 118–122. doi:10.1016/j.biortech.2010.06.031
Phwan, C. K., Chew, K. W., Sebayang, A. H., Ong, H. C., Ling, T. C., Malek, M. A., et al. (2019). Effects of acids pre-treatment on the microbial fermentation process for bioethanol production from microalgae. Biotechnol. Biofuels 12 (1), 191. doi:10.1186/s13068-019-1533-5
Rajan, K., and Senthilkumar, K. (2009). Effect of exhaust gas recirculation (EGR) on the performance and emission characteristics of diesel engine with sunflower oil methyl ester. Jordan J. Mech. Industrial Eng. 3 (4), 306–311.
Ranganathan, S. V., Narasimhan, S. L., and Muthukumar, K. (2008). An overview of enzymatic production of biodiesel. Bioresour. Technol. 99 (10), 3975–3981. doi:10.1016/j.biortech.2007.04.060
Reyimu, Z., and Özçimen, D. (2017). Batch cultivation of marine microalgae Nannochloropsis oculata and Tetraselmis suecica in treated municipal wastewater toward bioethanol production. J. Clean. Prod. 150, 40–46. doi:10.1016/j.jclepro.2017.02.189
Robles-Medina, A., González-Moreno, P. A., Esteban-Cerdán, L., and Biocatalysis, Molina-Grima E. (2009). Biocatalysis: Towards ever greener biodiesel production. Biotechnol. Adv. 27 (4), 398–408. doi:10.1016/j.biotechadv.2008.10.008
Rowbotham, J., Dyer, P. W., Greenwell, H., and Theodorou, M. (2012). Thermochemical processing of macroalgae: A late bloomer in the development of third-generation biofuels? Biofuels 3 (4), 441–461. doi:10.4155/bfs.12.29
Rozina, , Chia, S. R., Ahmad, M., Sultana, S., Zafar, M., Asif, S., et al. (2022). Green synthesis of biodiesel from Citrus medica seed oil using green nanoparticles of copper oxide. Fuel 323, 124285. doi:10.1016/j.fuel.2022.124285
Seon, G., Kim, H. S., Cho, J. M., Kim, M., Park, W-K., and Chang, Y. K. (2020). Effect of post-treatment process of microalgal hydrolysate on bioethanol production. Sci. Rep. 10 (1), 16698. doi:10.1038/s41598-020-73816-4
Sharma, Y. C., Singh, B., and Upadhyay, S. N. (2008). Advancements in development and characterization of biodiesel: A review. Fuel 87 (12), 2355–2373. doi:10.1016/j.fuel.2008.01.014
Shilton, A., Mara, D., Craggs, R., and Powell, N. (2008). Solar-powered aeration and disinfection, anaerobic co-digestion, biological CO2 scrubbing and biofuel production: The energy and carbon management opportunities of waste stabilisation ponds. Water Sci. Technol. 58 (1), 253–258. doi:10.2166/wst.2008.666
Shokrkar, H., Ebrahimi, S., and Zamani, M. (2017). Bioethanol production from acidic and enzymatic hydrolysates of mixed microalgae culture. Fuel 200, 380–386. doi:10.1016/j.fuel.2017.03.090
Shokrkar, H., Ebrahimi, S., and Zamani, M. (2018). Enzymatic hydrolysis of microalgal cellulose for bioethanol production, modeling and sensitivity analysis. Fuel 228, 30–38. doi:10.1016/j.fuel.2018.04.143
Siddiki, S. Y. A., Mofijur, M., Kumar, P. S., Ahmed, S. F., Inayat, A., Kusumo, F., et al. (2022). Microalgae biomass as a sustainable source for biofuel, biochemical and biobased value-added products: An integrated biorefinery concept. Fuel 307, 121782. doi:10.1016/j.fuel.2021.121782
Siddiki, S. Y. A., Uddin, M. N., Mofijur, M., Fattah, I. M. R., Ong, H. C., Lam, S. S., et al. (2021). Theoretical calculation of biogas production and greenhouse gas emission reduction potential of livestock, poultry and slaughterhouse waste in Bangladesh. J. Environ. Chem. Eng. 9 (3), 105204. doi:10.1016/j.jece.2021.105204
Sims, R. E., Mabee, W., Saddler, J. N., and Taylor, M. (2010). An overview of second generation biofuel technologies. Bioresour. Technol. 101 (6), 1570–1580. doi:10.1016/j.biortech.2009.11.046
Singh, A., Nigam, P. S., and Murphy, J. D. (2011). Renewable fuels from algae: An answer to debatable land based fuels. Bioresour. Technol. 102 (1), 10–16. doi:10.1016/j.biortech.2010.06.032
Sinha, S., Agarwal, A. K., and Garg, S. (2008). Biodiesel development from rice bran oil: Transesterification process optimization and fuel characterization. Energy Convers. Manag. 49 (5), 1248–1257. doi:10.1016/j.enconman.2007.08.010
Solé-Bundó, M., Eskicioglu, C., Garfí, M., Carrère, H., and Ferrer, I. (2017). Anaerobic co-digestion of microalgal biomass and wheat straw with and without thermo-alkaline pretreatment. Bioresour. Technol. 237, 89–98. doi:10.1016/j.biortech.2017.03.151
Solé-Bundó, M., Garfí, M., and Ferrer, I. (2020). Pretreatment and co-digestion of microalgae, sludge and fat oil and grease (FOG) from microalgae-based wastewater treatment plants. Bioresour. Technol. 298, 122563. doi:10.1016/j.biortech.2019.122563
Solé-Bundó, M., Garfí, M., Matamoros, V., and Ferrer, I. (2019). Co-digestion of microalgae and primary sludge: Effect on biogas production and microcontaminants removal. Sci. total Environ. 660, 974–981. doi:10.1016/j.scitotenv.2019.01.011
Suali, E., and Sarbatly, R. (2012). Conversion of microalgae to biofuel. Renew. Sustain. Energy Rev. 16 (6), 4316–4342. doi:10.1016/j.rser.2012.03.047
Tsapekos, P., Kougias, P., Alvarado-Morales, M., Kovalovszki, A., Corbière, M., and Angelidaki, I. (2018). Energy recovery from wastewater microalgae through anaerobic digestion process: Methane potential, continuous reactor operation and modelling aspects. Biochem. Eng. J. 139, 1–7. doi:10.1016/j.bej.2018.08.004
Wahidin, S., Idris, A., and Shaleh, S. R. M. (2016). Ionic liquid as a promising biobased green solvent in combination with microwave irradiation for direct biodiesel production. Bioresour. Technol. 206, 150–154. doi:10.1016/j.biortech.2016.01.084
Walker, D. (2010). Biofuels–for better or worse? Ann. Appl. Biol. 156 (3), 319–327. doi:10.1111/j.1744-7348.2010.00404.x
Wang, S., Jiang, X., Wang, N., Yu, L., Li, Z., and He, P. (2007). Research on pyrolysis characteristics of seaweed. Energy & Fuels 21 (6), 3723–3729. doi:10.1021/ef700214w
Wu, H., Li, J., Wang, C., Liao, Q., Fu, Q., and Liu, Z. (2020). Sequent production of proteins and biogas from Chlorella sp. via CO2 assisted hydrothermal treatment and anaerobic digestion. J. Clean. Prod. 277, 123563. doi:10.1016/j.jclepro.2020.123563
Xu, H., Miao, X., and Wu, Q. (2006). High quality biodiesel production from a microalga Chlorella protothecoides by heterotrophic growth in fermenters. J. Biotechnol. 126 (4), 499–507. doi:10.1016/j.jbiotec.2006.05.002
Yu, K. L., Chen, W-H., Sheen, H-K., Chang, J-S., Lin, C-S., Ong, H. C., et al. (2020). Bioethanol production from acid pretreated microalgal hydrolysate using microwave-assisted heating wet torrefaction. Fuel 279, 118435. doi:10.1016/j.fuel.2020.118435
Yu, K. L., Show, P. L., Ong, H. C., Ling, T. C., Chen, W-H., and Salleh, M. A. M. (2018). Biochar production from microalgae cultivation through pyrolysis as a sustainable carbon sequestration and biorefinery approach. Clean Technol. Environ. Policy 20 (9), 2047–2055. doi:10.1007/s10098-018-1521-7
Yu, K. L., Show, P. L., Ong, H. C., Ling, T. C., Lan, J. C-W., Chen, W-H., et al. (2017). Microalgae from wastewater treatment to biochar–feedstock preparation and conversion technologies. Energy Convers. Manag. 150, 1–13. doi:10.1016/j.enconman.2017.07.060
Zamorano-López, N., Borrás, L., Giménez, J. B., Seco, A., and Aguado, D. (2019). Acclimatised rumen culture for raw microalgae conversion into biogas: Linking microbial community structure and operational parameters in anaerobic membrane bioreactors (AnMBR). Bioresour. Technol. 290, 121787. doi:10.1016/j.biortech.2019.121787
Keywords: microalgae, biochemical process, sustainable fuel, greenhouse gas emission, biomass
Citation: Rony ZI, Mofijur M, Hasan MM, Ahmed SF, Badruddin IA and Khan TMY (2023) Conversion of algal biomass into renewable fuel: A mini review of chemical and biochemical processes. Front. Energy Res. 11:1124302. doi: 10.3389/fenrg.2023.1124302
Received: 15 December 2022; Accepted: 23 January 2023;
Published: 09 February 2023.
Edited by:
Abdul-Sattar Nizami, Government College University, Lahore, PakistanReviewed by:
Awais Bokhari, Brno University of Technology, CzechiaMd. Nahid Pervez, University of Salerno, Italy
Nadeem Khan, Jamia Millia Islamia, India
Copyright © 2023 Rony, Mofijur, Hasan, Ahmed, Badruddin and Khan. This is an open-access article distributed under the terms of the Creative Commons Attribution License (CC BY). The use, distribution or reproduction in other forums is permitted, provided the original author(s) and the copyright owner(s) are credited and that the original publication in this journal is cited, in accordance with accepted academic practice. No use, distribution or reproduction is permitted which does not comply with these terms.
*Correspondence: M. Mofijur, mdmofijur.rahman@uts.edu.au