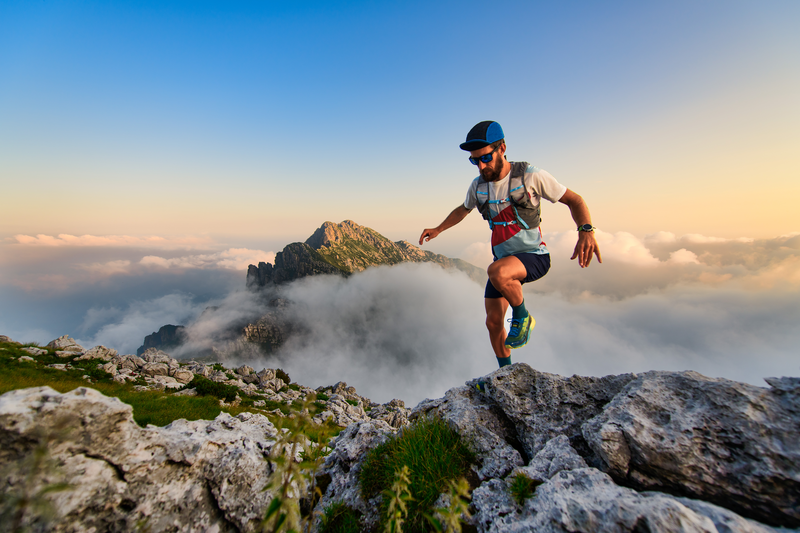
94% of researchers rate our articles as excellent or good
Learn more about the work of our research integrity team to safeguard the quality of each article we publish.
Find out more
ORIGINAL RESEARCH article
Front. Energy Res. , 15 September 2022
Sec. Sustainable Energy Systems
Volume 10 - 2022 | https://doi.org/10.3389/fenrg.2022.992617
This article is part of the Research Topic Critical Energy Minerals: A Material Enabler for Carbon Neutrality View all 17 articles
With the rapid development of China’s new energy vehicle industry, the supply security of lithium resources is crucial. To ensure the healthy development of the new energy vehicle industry and the security of supply of key mineral resources, it is necessary to accurately determine the supply and demand pressure of lithium resources and their sources. This study analyzes the lithium stock and flow at the end of the new energy vehicle chain by constructing a material flow analysis framework for the new energy vehicle industry and compiling a lithium resource flow table for the new energy vehicle industry, and the results show that 1) the supply and demand pressure on lithium resources in China is increasing year by year, and the external dependence of lithium resources has reached 75% in 2019; 2) China’s domestic lithium battery production and consumption accounted for nearly 70% of the lithium consumption in various industries, of which 60% of the lithium batteries were assembled on new energy vehicles, and the net outflow of lithium exceeded more than the lithium consumption of new energy vehicles in 2019. The lithium consumption of new energy vehicles was more than five times that of the previous year. Insufficient supply of domestic lithium ore, lithium inventory, and import and export are the key reasons for the pressure on lithium supply and demand in the new energy vehicle industry; 3) By the end of 2019, the cumulative scrap lithium batteries in new energy vehicles contain about 10,000 tons. The lithium accumulated in new energy vehicles has 26,500 tons. These results provide a theoretical basis for policy recommendations to ensure the healthy development of the new energy vehicle industry and to promote international cooperation in the development, utilization, and recycling of lithium resources.
As one of the most important strategic emerging minerals, lithium is widely used in battery energy storage, glass ceramics, grease, air treatment, metallurgy, medicine, and other fields. It is a key industrial raw material for the current and future (Xing et al., 2015). Due to the rapid development of the new energy vehicle industry, the high dependence of lithium-ion batteries on lithium resources has further accelerated the development of lithium resources. According to the statistics of the Lithium Industry Branch of the China Nonferrous Metals Association and related research institutions, the global lithium demand from 2015 to 2019 increased from 162 thousand tons to 297 thousand tons, with an average annual growth rate of 16.4%. However, the global lithium resources are relatively limited. As of 2020, the global lithium ore reserves are estimated to be 128.28 million tons (lithium carbonate equivalent), which are mainly distributed in Argentina, Bolivia, Chile, Australia, China, and other countries. China’s lithium ore reserves are 8.1 million tons (lithium carbonate equivalent), accounting for 6.31% of the world (Wang et al., 2021).
China is the world’s largest producer and seller of new energy vehicles and a consumer of lithium resources. In 2020, sales of new energy vehicles will reach 1.367 million units, accounting for 42% of global sales. In 2019, China’s lithium resource consumption was 186 thousand tons (LCE) (Dai et al., 2019), which accounted for 60% of global lithium resource consumption. Although China’s lithium resources are relatively abundant, there are problems such as poor resource quality and difficult development. The current development scale of lithium resources is not large and relies heavily on imports (Ju and Zhao, 2018). According to the “New Energy Vehicle Industry Development Plan (2021–2035),” the sales of new energy vehicles in China will account for about 20% of the total sales of new vehicles by 2025. With the continuous improvement of global status, the demand for lithium resources will increase sharply in the future development of the new energy vehicle industry. In the post-epidemic era, global uncertainty and instability have further increased, and China’s lithium resource supply may face greater uncertainty in the future. In order to actively respond to the supply and demand risks of lithium resources, it is currently necessary to accurately grasp the sources and causes of lithium supply and demand pressures in the new energy vehicle industry.
In recent years, material flow analysis methods have been applied in the fields of emerging mineral resources such as lithium, cobalt, nickel, indium, rare Earth elements, etc.(Liu et al., 2021). This method has played an important role in the analysis of international trade, consumption space, waste recycling, and environmental impact of emerging minerals (Shinkuma and Nguyen Thi Minh, 2009). Saskia Ziemann and others established the first global lithium flow model including production, manufacturing, and use, which laid the foundation for later lithium flow analysis (Ziemann et al., 2012; Ziemann et al., 2018a; Ziemann et al., 2018b). At present, the research on lithium material flow has covered the world, various regions, and countries, and the research methods include static material flow, dynamic material flow, element material flow, mixed model including material flow analysis, etc. However, most studies are still based on the traditional bulk mineral material flow analysis framework to examine the material flow of emerging minerals. In contrast, there will be a certain amount of loss in the process of lithium resources from mining, processing into raw materials, and then entering commodities. The material flow analysis results based on bulk minerals cannot accurately describe the real lithium flow in the new energy vehicle industry. In addition, due to the high price, small volume, speculative, and other characteristics of lithium, the lithium resources and lithium raw materials that are mined and refined at a certain time cannot be completely consumed at that time. But most of the current research did not take into account the analysis of lithium inventories and lithium losses. At present, new energy vehicles have gradually grown into the largest application field of lithium batteries, and new energy vehicles have also become key areas of lithium material flow research. Rapid development and its high dependence on lithium batteries will accelerate the consumption of lithium resources (Hao et al., 2017), and the spatial distribution of lithium resources and international trade will lead to lithium supply and demand risks (Hu et al., 2021). However, in these studies new energy vehicles are only one part of the lithium material flow (Richa et al., 2014), and they do not consider the lithium flow in the whole industry chain of new energy vehicles (Univ et al., 2016; Shafique et al., 2022), nor can they describe the changes and risks (Chang et al., 2009; Gaines, 2014; Song et al., 2019) of lithium material flow caused by changes in new energy vehicles. Although dynamic material flow analysis can analyze the material flow in the system at different times, and can also realize the connection of material flow within the system at the same time, it is still difficult to solve the connection and comparison of material flow between time and systems. Therefore, from the perspective of three-dimensional chain, time, and space, this paper constructs the lithium flow analysis framework of the new energy automobile industry, compiles the dynamic lithium flow table of the new energy automobile industry, and sorts out the stock and flow of lithium resources at each chain end of the new energy automobile industry, analyzes the key sources of lithium supply and demand pressure in the new energy vehicle industry, and provides reference for the compilation of other key mineral resource flow tables.
Material Flow Analysis (MFA) follows the law of conservation of mass, analyzes the flow of materials by linking resources, paths, intermediate processes, and the final destination of materials, and traces the source and path of material flow in a specific system and sinks (Islam and Huda, 2019). As an important tool for material management analysis (Brunner and Rechberger, 2016), MFA has been widely used in the fields of resource management, waste management, environmental management, and sustainable development (Kiddee et al., 2013). Material flow analysis methods can be divided into two categories: material flow analysis of economic system and element flow analysis. Material flow analysis of economic system is the study of the total amount and structure of matter in a system within a certain time and space range, regardless of the internal material flow and element flow in the system. The research object of the analysis is a specific element, monomer or compound. Based on the law of conservation of mass, it tracks and investigates the material flow trajectory in a certain space-time system, and then obtains data on the circulation and waste discharge of each link during the flow of the material throughout its life cycle. A large number of studies have successfully examined the material flows of bulk minerals such as steel (Davis et al., 2007; Park et al., 2011; Pauliuk et al., 2012), copper (Gloser et al., 2013; Wang et al., 2018), aluminum (Chen et al., 2008; McMillan et al., 2010; Liu and Muller, 2013) and zinc (Meylan and Reck, 2017) in various countries around the world using material flow analysis methods (Sun et al., 2019).
New energy vehicles are powered by unconventional energy. Lithium batteries are divided into two categories: primary lithium batteries and secondary lithium batteries. Secondary lithium batteries include lithium-ion batteries and all-solid-state lithium batteries. Lithium-ion batteries are composed of positive electrode materials, negative electrode materials, electrolytes, diaphragm, and auxiliary materials such as ultra-thin copper foil and aluminum foil. The positive electrode materials mainly include lithium cobaltate, lithium manganate, lithium nickelate, ternary materials (lithium nickel manganese cobalt) and lithium iron phosphate, the negative electrode materials are mainly graphite, graphitic fiber materials, etc., and the electrolyte is composed of solvent, lithium salt (LiFL6), and additives. The new energy vehicles mentioned in this study are electric vehicles equipped with lithium-ion batteries, and three types of new energy vehicles are selected as pure electric vehicles, hybrid electric vehicles, and fuel cell electric vehicles. The spatial boundaries of the system are defined as China and outside China. Although new energy vehicles have been promoted and used in China since this century, it was not until 2012 that China’s new energy vehicle production and sales exceeded 10,000 units, and only after 2014 did they begin to be developed on a large scale. Due to the limitation of data availability, the time boundary was chosen to be 2014–2019. In this paper, new energy vehicles are composed of plug-in hybrid electric passenger cars, plug-in hybrid electric special vehicles, pure electric passenger cars, pure electric special vehicles, and fuel cell vehicles. The battery is composed of lithium iron phosphate lithium battery, lithium manganate lithium battery, lithium cobalt oxide lithium battery, and ternary material lithium battery.
In this study, the lithium material flow in the new energy industry chain was analyzed based on the element flow analysis method. The new energy vehicle industry is a collection of economic activities carried out around the design, research and development, production, sales, maintenance and repair, scrap and dismantling of new energy vehicles. The focus of the research is to analyze the production (consumption) links directly related to lithium resources in the new energy vehicle industry chain. The new energy vehicle industry chain is centered on the manufacture of new energy vehicles, and the upper end includes lithium battery production, lithium raw material mining and extraction, lithium battery material production, and other links, the lower end of the new energy vehicle use, scrap, recycling, and other links. According to the flow process and important links of lithium element in the new energy vehicle industry chain, the new energy vehicle industry chain is divided as follows (see Figure 1 for details).
The lithium material flow of the new energy vehicle industry has three attributes of chain, time, and space. “Lithium” can flow along the three dimensions of chain, time, and space. From the perspective of the entire life cycle of the industry, “lithium” flows in sequence following the industrial chain, and the flow of lithium is one-way and cyclic. From the perspective of time, the new energy vehicle industry chain needs to span different periods. Generally speaking, lithium mineral mining, lithium raw material production, lithium battery material production, lithium battery production, and even new energy vehicle manufacturing can be completed within the same period. The consumption of energy vehicles and the reuse of retired lithium batteries take a very long time, and the time required for the recycling, dismantling, and recycling of lithium resources of scrapped lithium batteries is also relatively long. From a spatial point of view, the lithium material flow in the new energy vehicle industry involves the country (region) and other countries (regions), and can be divided into two categories: domestic and foreign. The inflow of lithium from the foreign new energy vehicle industry to China is lithium import. On the contrary, it is lithium export. Lithium-containing brine in salt lakes is bulky and difficult to transport. Brine cannot flow between countries. Scrap lithium batteries have potential environmental risks, and scrap lithium batteries should not flow between countries. The “lithium” (materials, products) in the production raw materials of a certain link in the domestic new energy automobile industry chain added the “lithium inventory” and “lithium import” that flowed in the previous cycle minus the “lithium export”, are the “lithium” stock on the link in this country.
In a certain period (usually 1 year), the lithium stock at the end of a certain industrial chain of new energy vehicles in the country may not all enter the next production stage, and the difference is the inventory, which will flow into the industrial chain of the next period and even other periods. Although lithium has inter-period lithium flow (stock) in various industries of new energy vehicles, generally speaking, the lithium stock of new energy vehicle manufacturing and its previous industrial chains in a certain period is not much different, and the lithium stock at each chain end is also not much. In comparison, there are more lithium stocks at the consumption end of new energy vehicles involved in a certain period (including the retirement and reuse of assembled lithium batteries), and the lithium stocks at this time include the lithium stocks on all new energy vehicles that are still in consumption and use. The same is true for the lithium stock at the scrap and recycling end of lithium batteries, which involves the lithium on all lithium batteries scrapped from new energy vehicles. To sum up, the lithium flow of the new energy vehicle industry in a certain period not only includes the sequential flow of the lithium stock in the production link of the new energy vehicle in the current period, but also includes the lithium stock of all the new energy vehicles in use during this period, that is, the lithium stock in different periods intertemporal flow. (See Figure 2).
According to the above division of the new energy vehicle industry chain, the lithium material flow analysis framework of the new energy vehicle industry, and based on the lithium existence form and flow process at each industrial chain end, determine the nodes and flow directions of each industrial chain end, and compile the new energy vehicle industry of lithium flow meter standard template. The flow meter can measure the flow and stock of lithium resources in the new energy vehicle industry, and also include the flow direction and flow information of lithium resources.
Considering uncontrollable factors, the following assumptions are made: 1) The lithium battery and the vehicle produced in the year were consumed and put into use; 2) The demand and consumption of lithium batteries in new energy vehicles, 3C, and energy storage industries are consistent. The lithium consumption of battery products in China from 2014 to 2019 is consistent with the total demand for lithium-ion batteries in the corresponding industries, and the relevant data are consistent. 3) Domestic and foreign new energy vehicles, lithium battery production technology level, all kinds of lithium battery unit storage lithium consumption intensity are consistent; 4) The performance of new energy vehicle industry is consistent with that of lithium batteries applied in 3C and energy storage fields, and the lithium consumption intensity of unit power storage is the same.
The production information data of new energy vehicles and the production information data of assembled lithium batteries are from the “Energy Saving and New Energy Vehicle Yearbook,” refer to the “Vehicle Purchase Tax Exemption” and “Enjoy Vehicle and Vessel Tax Reduction and Exemption” jointly issued by the Ministry of Industry and Information Technology and the State Administration of Taxation to supplement the missing data of new energy vehicle model information. The historical lithium consumption data of China’s lithium batteries, pharmaceuticals, glass ceramics, lubricants, and other products; China’s demand for lithium batteries for new energy vehicles, 3C products, and energy storage comes from Shanghai Nonferrous Metals Network (SMM).
Lithium mining enterprises excavate lithium ore and lithium-containing brine (lithium-rich minerals) from mines and salt lakes (seawater). The calculation formula of lithium flow during the extraction stage of lithium ore is as follows,
Eq. 1,
Part of the lithium raw materials have entered the field of lithium battery production, and other parts have entered the production fields of glass ceramics, grease, polymers, metallurgy, and other industries. According to the proportion of the domestic lithium battery industry production demand for lithium to the production demand for lithium in all industries, the demand for lithium in domestic production can be calculated. The calculation formula of lithium flow at the production end of lithium raw materials can be as follows,
In Eq. 2,
First, according to the vehicle type of the new energy vehicle, the type of lithium battery assembled and its power storage information, the unit vehicle storage power of each type of new energy vehicle is calculated.
In Eq. 3,
In Eq. 4,
Secondly, according to the output of new energy vehicles and the power storage per unit vehicle, the total power storage of new energy vehicles is calculated.
In Eq. 5,
In Eq. 6,
Finally, according to the total storage capacity of lithium batteries assembled in new energy vehicles and the total consumption of lithium in the new energy vehicle industry, the lithium consumption intensity of lithium batteries is calculated.
In Eq. 7,
Combined with the actual production technology of lithium batteries in China, the structural decomposition method is used to set the lithium consumption intensity of lithium iron phosphate, lithium manganate, lithium cobaltate, and ternary material lithium batteries in China from 2014 to 2019 (See Table 1).
The calculation formula of lithium consumption of various types of new energy vehicles is as follows,
In Eq. 8,
In Eq. 9,
The calculation formula of lithium flow at the production end of lithium battery is as follows,
In Eq. 10,
According to the import and export of lithium primary batteries, the total number of lithium batteries accounted for the proportion of the total number of lithium batteries assembled in new energy vehicles, and the above measurement of lithium consumption of lithium batteries assembled in new energy vehicles, we can measure the import and export of lithium primary batteries and lithium ion storage batteries in lithium content.
The lithium consumption of the lithium battery production of new energy vehicles calculated above is the lithium flow rate of the lithium battery production side, that is,
According to the ratio of the import and export of new energy vehicles to domestic production, the import and export flow of lithium in the industry and the net inflow of lithium are calculated.
In Eq. 12,
The lithium stock of the new energy vehicle consumer side in that year is the lithium stock of the new energy vehicle in the current state of use minus the lithium recovery potential formed by the retired lithium battery that year.
In Eq. 13,
When the power storage performance of the lithium battery assembled in the new energy vehicle drops below 80%, the lithium battery will no longer be able to be used in the new energy vehicle and it needs to be retired (scrapped).
Calculation method for retired lithium battery in new energy vehicle assembly. The two-parameter Weibull distribution model was used to estimate the amount of lithium resource decommissioning of new energy vehicles in each year. Comprehensively consider the cycle times and charging times of lithium batteries, refer to existing research results (Zheng et al., 2022), China’s mandatory automobile scrapping indicators, technical standards and maximum mileage, and other factors, as well as new energy passenger cars, passenger buses, and special vehicle power batteries equipped with different lithium batteries. The maximum retirement age shall be treated as 1.3 times the average retirement age. According to the maximum years of lithium batteries installed in different new energy vehicles, the corresponding retirement time probability distribution of lithium batteries is calculated as Table 2 shows.
Lithium recycling mainly comes from lithium batteries that are directly scrapped and lithium batteries that are scrapped after secondary use. Considering the actual situation in China, only a small part of lithium batteries retired before 2018 have been used in cascade, and only a small part of lithium batteries have been used in cascade after 2018. This paper will no longer measure the lithium recovery potential of this part. According to the lithium battery retirement probability of new energy vehicles and the calculated lithium content of new energy vehicles, the lithium recovery potential of retired lithium batteries of new energy vehicles is calculated.
In Eq. 14,
The flow of lithium in the new energy vehicle industry chain is affected by factors such as production technology and technical level. Lithium loss occurs in some production links. Lithium loss mainly occurs in the production process of lithium ore raw materials and cathode materials. At present, the relevant technical parameters show that China’s lithium minerals are mainly lithium ore (Tian et al., 2020; Zhang et al., 2020), the lithium recovery rate in the production of lithium carbonate and lithium hydroxide is 90%, and the lithium recovery rate in the production of cathode materials is 98%, that is, there is a 12% loss of lithium in the whole production process of lithium raw materials. Theoretically, the lithium element will be lost in the production of lithium batteries, assembly and even the assembly of new energy vehicles. The lithium in the scrap lithium battery can not be completely recycled, but the loss is small, this paper is no longer considered (Kamran et al., 2021).
China’s external dependence on lithium mines is high and continues to increase. The mining of concentrate and brine has been increasing year by year, and the extraction of domestic lithium resources has increased from 4.014 thousand tons in 2014 to 12.631 thousand tons in 2019. Among them, the lithium extracted from brine has increased from 1.989 thousand tons in 2014 to 6.692 thousand tons in 2019. However, this still cannot meet the domestic demand for lithium resources. The import of lithium raw materials continues to increase, and the external dependence of lithium salt production raw materials has rapidly increased from 67% in 2014 to 75% in 2019. (See Supplementary Table S1).
China is a net outflow country of lithium raw materials and lithium battery materials, and has made positive contributions to the global supply of lithium raw materials and lithium battery materials. In 2019, the net outflow of lithium from the lithium raw material end in China was 4.679 thousand tons, of which 8.062 thousand tons was net through lithium hydroxide, and lithium carbonate, lithium fluoride, and lithium iron phosphate were the net inflow raw materials. China is a net inflow country in terms of lithium battery materials, and in 2019, a net inflow of 1.004 thousand tons of lithium through lithium battery materials. Overall, in 2019, China had a net outflow of 3.675 thousand tons of lithium through lithium raw materials and lithium battery materials, while before 2018, on the contrary, China was a net inflow country in this regard. (See Supplementary Table S2).
The consumption of lithium in various industries in China has grown rapidly year by year, from 9.137 thousand tons in 2014 to 21.938 thousand tons in 2019. The average annual growth rate of lithium consumption has reached 19%. Among them, the proportion of lithium consumption in lithium battery industry has increased from 57% in 2014 to 69% in 2019 (see Supplementary Table S3).
The new energy vehicle industry has gradually grown into the industry with the largest demand for lithium batteries. In 2019, the lithium content of lithium batteries in China’s new energy vehicles was 9.06 thousand tons, which accounted for 60% of the total domestic lithium battery consumption. In 2014, this proportion was only 13%. The lithium batteries assembled in new energy vehicles are mainly ternary material batteries and lithium iron phosphate batteries. In 2019, the lithium content of these two types of lithium batteries was 6.348 thousand tons and 2.548 thousand tons respectively. (See Supplementary Table S4, S5).
The number of lithium batteries China provides to the world continues to grow rapidly. In 2019, China had a net outflow of 7.9 thousand tons of lithium through the import and export of lithium batteries. In 2018, the net outflow of lithium was only 3.271 thousand tons. In 2014, the net outflow of lithium was 11 tons. Most of the net outflow of lithium was achieved through lithium battery imports and exports, and the import and export of lithium batteries alone was very small. (See Supplementary Table S5).
The consumption of lithium in the production stage of new energy vehicles continues to grow rapidly. The consumption of lithium in domestic new energy vehicle production increased rapidly from 655 tons in 2014 to 90.6 million tons in 2019, with an average annual growth rate of 83%. Among them, the main force of lithium consumption is the production of pure electric passenger cars and pure electric buses. In 2019, the lithium required for domestic production of pure electric passenger cars and pure electric buses accounted for 67% and 20% of the total, respectively. However, the net inflow of lithium through imported new energy vehicles is less than 100 tons, and the lithium stock on the consumption side of new energy vehicles is roughly equal to the lithium stock on the domestic production side. (See Supplementary Table S6).
Passenger cars have the longest retirement (scrap) years. In 2020, the retired (scrapped) lithium batteries of plug-in hybrid electric vehicles and pure electric passenger vehicles will contain about 346 tons of lithium; passenger cars and special vehicles have a shorter service life. In the early stage, the amount of scrapped is relatively large. In 2020, the retired (scrapped) lithium battery of pure electric buses will contain about 2.571 thousand tons of lithium; At present, most new energy vehicles are directly scrapped after the lithium batteries are scrapped. In the future, the amount of lithium battery replacement generated by the retirement (scrapped) of lithium batteries in new energy vehicles will increase rapidly, which will increase the demand for lithium resources. China’s new energy vehicles retired lithium batteries have less echelon utilization. In 2020, the retired lithium batteries will be used in other industries and the lithium batteries will contain less than 800 tons of lithium (See Supplementary Table S7, S8).
According to the existing status and stock of lithium in the new energy vehicle industry chain from 2014 to 2019, the flow table and flow chart of lithium resources in China’s new energy vehicle industry can be compiled, as shown in Figures 3-6.
Insufficient supply of domestic lithium resources is a key bottleneck for the pressure of lithium supply and demand in China’s new energy vehicle industry. According to the British Geological Survey report, China’s lithium reserves account for about 8% of the world’s reserves (BGS, 2016), which is relatively abundant, but 78% of China’s lithium reserves are distributed in the Qinghai-Tibet region, which exists in the form of salt lake resources. The development of lithium resources is difficult and costly, especially the challenges such as salt lake mining are difficult to overcome. The extraction of spodumene and lepidolite resources also faces the same problem. At the same time, the development of lithium resources is subject to environmental restrictions such as energy consumption standards, fluorine emissions, and solid waste emissions, further limiting its mining capacity. In 2019, the total domestic production of lithium resources in China was only 12.631 thousand tons. Although it has increased significantly compared with 4.014 thousand tons in 2014, China’s lithium consumption of 9.137 thousand tons in that year is still significantly insufficient. The domestic supply capacity of lithium resources is limited. Under the “dual carbon” goal, China’s future demand for lithium in the new energy vehicle industry will continue to maintain rapid growth, and the pressure on supply and demand of lithium resources will continue to increase in the future.
The import and export of related products is also an important source of lithium supply and demand pressure in the new energy industry. China’s new energy vehicle industry is actively integrating into the global industrial chain and value chain. China has exported a large amount of lithium hydroxide, lithium hexafluorophosphate, lithium metal and other lithium raw materials and lithium battery materials to foreign countries. In 2019, China passed lithium raw materials, lithium battery materials, lithium batteries, and the total net outflow of lithium from new energy vehicles is about 11.669 thousand tons, while the domestic consumption of lithium produced by new energy vehicles in 2019 is only 9.06 thousand tons. With the advancement of China’s lithium battery and new energy vehicle production technology, China will contribute more lithium battery raw materials, materials, lithium batteries, and new energy vehicles to the world in the future, which will further increase the supply and demand pressure of lithium resources in the new energy industry.
The global lithium ore market has a strong monopoly, and the price of lithium ore fluctuates greatly. The lithium inventory brought by these factors is the main source of lithium supply and demand pressure in China’s new energy vehicle industry. At present, the global concentration of lithium ore resources is relatively high, and lithium ore resources are mainly in the hands of a few global mining groups. According to data provided by Roskill, in 2017, 80% of global lithium production capacity and 85% of lithium production came from Talison Lithium, SQM, Reed Ind. Mins 11 other manufacturers. The overly concentrated market structure is likely to cause collective market conspiracy and abnormal fluctuations in lithium ore prices. Since 2016, the global “lithium” price began to grow rapidly. Although it began to drop sharply after 2018 (Wang, 2020), the global “lithium” price will increase rapidly in 2021. The drastic changes in the price of lithium raw materials have not only caused price pressure on the downstream manufacturers of new energy vehicles, but also caused huge pressure on the raw material supply of various manufacturers in the industrial chain. Inventory has become a common choice for many raw material manufacturers to avoid risks.
In order to ensure the safe supply of lithium resources required by the new energy vehicle industry in China and even in the world, the following main suggestions are put forward around the key links, main pressures and future development trends of lithium material flow in the new energy vehicle industry.
First, in order to guide the healthy development of the lithium resource market, industry organizations such as professional key mineral industry associations and lithium mining industry alliances can be established, which can help further develop and publish information to strengthen industry standards, industry information, and development plans. At the same time, this can also effectively inhibit vicious inventory and vicious market competition behavior. In addition, it is necessary to strengthen the market information monitoring and statistics of lithium resources, lithium raw materials, and their related products. It is also important to strengthen the data monitoring, data analysis, and risk control of key aspects of lithium material flow for new energy vehicles. When formulating competition norms for the key mineral industry, it is necessary to center on lithium and strictly control the disorderly development of the industry.
Second, international cooperation in lithium resource development is also important. For China, we need to promote cooperation with South America, Africa, the Belt and Road, and other regions on the development of lithium mineral resources. At the same time, international cooperation in the whole industrial chain of lithium, lithium raw materials, lithium materials, lithium batteries, and new energy vehicles is also essential. Only in this way can we improve China's voice and influence in global lithium resource development and market operation, and further participate in global lithium mineral resource exploration and development research.
Third, we need to pay attention to technological innovation. Innovative research on key technologies for lithium battery production, lithium extraction from salt lakes, lithium extraction from ores, and lithium battery resourcing is very important. The joint efforts of global scholars can help break through the technical bottleneck of lithium extraction from lithium ore more quickly. In addition, solid fuel cells and hydrogen energy development and application are equally important. Reducing the dependence of new energy vehicles on lithium resources is a key measure to ease the pressure of lithium supply and demand. In order to give full play to the full life value of lithium, more efforts are needed in research to extend the life length of various lithium products. For example, retired lithium batteries from new energy vehicles can be encouraged for safe stepwise utilization in other industries (wind power, photovoltaic energy storage).
Finally, we need to pay attention to the construction of lithium resource recovery system. With the increasing amount of lithium resources at end-of-life, in order to improve the efficiency of lithium resources recycling, we can encourage the lithium resources recycling system to change from government-driven to market-led, so that enterprises engage in lithium battery recycling, storage, and disposal. The problems faced by lithium battery resources, such as economy of scale and transportation cost, also need to be taken seriously. We need to combine the spatial layout of the lithium battery material industry with the scientific and reasonable layout of the lithium battery resource industry. We should implement different lithium battery recycling policies and give financial support to small and medium-sized cities, remote areas, and ecological environment fragile areas.
In this article, the flow and stock of lithium resources in China’s new energy vehicle industry were analyzed using the material flow analysis method, and a new energy industry lithium material flow table for this industry in 2019 and a lithium material flow diagram for this industry from 2014 to 2019 were completed. In summary, the following main conclusions can be obtained.
The technical performance and structural changes of lithium batteries assembled in new energy vehicles will affect their consumption of lithium. Although the lithium consumption intensity of lithium batteries produced in China continues to decline, the lithium battery storage required for new energy vehicles to assemble lithium batteries continues to increase, and the structure of lithium batteries for new energy vehicles has gradually changed from lithium iron phosphate batteries to ternary batteries. Material battery transformation, these changes affect the lithium consumption of the new energy vehicle industry.
The lithium battery and new energy vehicle industries have gradually become the main force of lithium resource consumption. In 2019, China’s domestic lithium battery production and consumption consumed 15.04 thousand tons of lithium, accounting for 29% of the total lithium output at the lithium mineral end and 69% of the total domestic lithium consumption of lithium raw materials. Domestic new energy vehicle production consumes 9.06 thousand tons of lithium through lithium batteries, which accounts for 18% of the total lithium output at the lithium mineral end, and 60% of the lithium consumption required for domestic production of lithium batteries.
The supply and demand pressure of lithium resources in China’s new energy vehicle industry mainly comes from lithium batteries, new energy vehicles, import and export, and inventory. In 2019, China’s net outflow of lithium through the import and export of lithium batteries, lithium battery raw materials, materials and new energy vehicles accounted for 23% of the domestic lithium raw material consumption. The cumulative domestic lithium inventory is more than twice the lithium consumption of various industries in that year, and more than five times the lithium consumption of new energy vehicles.
New energy vehicles are an important lithium mineral that cannot be ignored. As of 2019, the lithium stock accumulated in new energy vehicles is about 26.484 thousand tons. More and more lithium batteries will be retired and scrapped from new energy vehicles in the future. From 2014 to 2019, the accumulated lithium content of retired (scrapped) lithium batteries is about 6.92 thousand tons. In 2020, the accumulated lithium content of retired (scrapped) lithium batteries will reach 10.721 thousand tons, which is the threshold value for enterprises to recycle and reuse lithium batteries. It is also an important node for China to formulate lithium battery recycling policies.
The original contributions presented in the study are included in the article/Supplementary Material, further inquiries can be directed to the corresponding authors.
LZ: data curation, writing original draft, writing review, and editing. GC: resources, writing original draft, writing review, and editing. LL: methodology, resources, writing original draft. YH: methodology, resources, writing original draft. All authors contributed to the article and approved the submitted version.
We acknowledge the data and valuable suggestions from Global Mineral Resources Strategy Research Center of China Geological Survey. We are thankful to the financial supports from the National Social Science Foundation of China (No. 20ATJ004); Geological Survey project of China Geological Survey (No. DD20221975).
The authors declare that the research was conducted in the absence of any commercial or financial relationships that could be construed as a potential conflict of interest.
All claims expressed in this article are solely those of the authors and do not necessarily represent those of their affiliated organizations, or those of the publisher, the editors and the reviewers. Any product that may be evaluated in this article, or claim that may be made by its manufacturer, is not guaranteed or endorsed by the publisher.
The Supplementary Material for this article can be found online at: https://www.frontiersin.org/articles/10.3389/fenrg.2022.992617/full#supplementary-material
BGS (2016). British geography Survey natural environment council. Lithium [Online]. Available at: https://www2.bgs.ac.uk/mineralsuk/download/mineralProfiles/lithium_profile.pdf.
Brunner, P. H., and Rechberger, H. (2016). Handbook of material flow analysis : For environmental, resource, and waste engineers. Boca Raton: Place of publication not identified: CRC Press. doi:10.1201/9781315313450 |
Chang, T. C., You, S. J., Yu, B. S., and Yao, K. F. (2009). A material flow of lithium batteries in Taiwan. J. Hazard. Mat. 163 (2-3), 910–915. doi:10.1016/j.jhazmat.2008.07.043 | |
Chen, W., Qian, Y., and Shi, L. (2008). Aluminium substance flow analysis for mainland China in 2005. Resour. Sci. 30 (9), 1320–1326.
Dai, T., Wang, G., Chen, Y., and Wen, B. (2019). China’s demand for energy and mineral resources by 2035. Chin. J. Eng. Sci. 21 (1), 68. doi:10.15302/j-sscae-2019.01.010 |
Davis, J., Geyer, R., Ley, J., He, J., Clift, R., Kwan, A., et al. (2007). Time-dependent material flow analysis of iron and steel in the UK. Resour. Conservation Recycl. 51 (1), 118–140. doi:10.1016/j.resconrec.2006.08.007 |
Gaines, L. (2014). The future of automotive lithium-ion battery recycling: charting a sustainable course. Sustain. Mater. Technol. 1-2, 2–7. doi:10.1016/j.susmat.2014.10.001 |
Gloser, S., Soulier, M., and Tercero Espinoza, L. A. (2013). Dynamic analysis of global copper flows. global stocks, postconsumer material flows, recycling indicators, and uncertainty evaluation. Environ. Sci. Technol. 47 (12), 6564–6572. doi:10.1021/es400069b | |
Hao, H., Liu, Z., Zhao, F., Geng, Y., and Sarkis, J. (2017). Material flow analysis of lithium in China. Resour. Policy 51, 100–106. doi:10.1016/j.resourpol.2016.12.005 |
Hu, X., Wang, C., Zhu, X., Yao, C., and Ghadimi, P. (2021). Trade structure and risk transmission in the international automotive li-ion batteries trade. Resour. Conservation Recycl. 170, 105591. doi:10.1016/j.resconrec.2021.105591 |
Islam, M. T., and Huda, N. (2019). Material flow analysis (MFA) as a strategic tool in e-waste management: applications, trends and future directions. J. Environ. Manage. 244, 344–361. doi:10.1016/j.jenvman.2019.05.062 | |
Ju, G., and Zhao, X. (2018). Special topic of non-ferrous metals-the pattern of lithium resources in salt lakes in China. [Online]. Available at: http://www.767stock.com/2018/07/25/37207.html.
Kamran, M., Raugei, M., and Hutchinson, A. (2021). A dynamic material flow analysis of lithium-ion battery metals for electric vehicles and grid storage in the UK: Assessing the impact of shared mobility and end-of-life strategies. Resour. Conservation Recycl. 167, 105412. doi:10.1016/j.resconrec.2021.105412 |
Kiddee, P., Naidu, R., and Wong, M. H. (2013). Electronic waste management approaches: an overview. Waste Manag. 33 (5), 1237–1250. doi:10.1016/j.wasman.2013.01.006 | |
Liu, G., and Muller, D. B. (2013). Centennial evolution of aluminum in-use stocks on our aluminized planet. Environ. Sci. Technol. 47 (9), 4882–4888. doi:10.1021/es305108p | |
Liu, W., Liu, W., Li, X., Liu, Y., Ogunmoroti, A. E., Li, M., et al. (2021). Dynamic material flow analysis of critical metals for lithium-ion battery system in China from 2000–2018. Resour. Conservation Recycl. 164, 105122. doi:10.1016/j.resconrec.2020.105122 |
McMillan, C. A., Moore, M. R., Keoleian, G. A., and Bulkley, J. W. (2010). Quantifying U.S. aluminum in-use stocks and their relationship with economic output. Ecol. Econ. 69 (12), 2606–2613. doi:10.1016/j.ecolecon.2010.08.005 |
Meylan, G., and Reck, B. K. (2017). The anthropogenic cycle of zinc: Status quo and perspectives. Resour. Conservation Recycl. 123, 1–10. doi:10.1016/j.resconrec.2016.01.006 |
Park, J.-a., Hong, S.-j., Kim, I., Lee, J.-y., and Hur, T. (2011). Dynamic material flow analysis of steel resources in Korea. Resour. Conservation Recycl. 55 (4), 456–462. doi:10.1016/j.resconrec.2010.12.007 |
Pauliuk, S., Wang, T., and Muller, D. B. (2012). Moving toward the circular economy: the role of stocks in the Chinese steel cycle. Environ. Sci. Technol. 46 (1), 148–154. doi:10.1021/es201904c | |
Richa, K., Babbitt, C. W., Gaustad, G., and Wang, X. (2014). A future perspective on lithium-ion battery waste flows from electric vehicles. Resour. Conservation Recycl. 83, 63–76. doi:10.1016/j.resconrec.2013.11.008 |
Shafique, M., Rafiq, M., Azam, A., and Luo, X. (2022). Material flow analysis for end-of-life lithium-ion batteries from battery electric vehicles in the USA and China. Resour. Conservation Recycl. 178, 106061. doi:10.1016/j.resconrec.2021.106061 |
Shinkuma, T., and Nguyen Thi Minh, H. (2009). The flow of E-waste material in the Asian region and a reconsideration of international trade policies on E-waste. Environ. Impact Assess. Rev. 29 (1), 25–31. doi:10.1016/j.eiar.2008.04.004 |
Song, J., Yan, W., Cao, H., Song, Q., Ding, H., Lv, Z., et al. (2019). Material flow analysis on critical raw materials of lithium-ion batteries in China. J. Clean. Prod. 215, 570–581. doi:10.1016/j.jclepro.2019.01.081 |
Sun, X., Hao, H., Liu, Z., Zhao, F., and Song, J. (2019). Tracing global cobalt flow: 1995–2015. Resour. Conservation Recycl. 149, 45–55. doi:10.1016/j.resconrec.2019.05.009 |
Tian, J., Li, T., Wang, M., Zhao, H., and Shi, J. (2020). Research progress on extraction process of lithium from lepidolite. doi:10.3969/j.issn.1000-2375.2020.01.009 |
Univ, K., Xu, G., and Yano, J. (2016). Material flow analysis of lithium-ion batteries from electric vehicle considering reuse scheme. [Online]. Available at: https://www.researchgate.net/publication/316523784_Material_Flow_Analysis_of_Lithium-ion_Batteries_from_Electric_Vehicle_Considering_Reuse_Scheme.
Wang, M., Liang, Y., Yuan, M., Cui, X., Yang, Y., and Li, X. (2018). Dynamic analysis of copper consumption, in-use stocks and scrap generation in different sectors in the U.S. 1900–2016. Resour. Conservation Recycl. 139, 140–149. doi:10.1016/j.resconrec.2018.07.022 |
Wang, S., Liu, S., Wang, T., and Zhao, T. (2021). Assessment report for lithium, cobalt, nickel, tin, and potash reserves in the world.
Wang, Z. (2020). Analysis of lithium supply and demand and price fiuctuation. doi:10.12075/j.issn.1004-4051.2020.S1.097 |
Xing, J., Peng, H., Zhang, Y., and Chen, Q. (2015). Global lithium demand and supply. Resour. Sci. 37 (5), 988–997.
Zhang, X., Tan, X., Liu, W., Wang, W., and Zhang, L. (2020). Current status and research progress of Lithium extraction technology from ore. doi:10.13779/j.cnki.issn1001-0076.2020.05.003 |
Zheng, L., Zhang, Y., Li, Z., and Zhao, Y. (2022). Lithium recovery potential of new energy vehicles in China under different consumption scenarios. Resour. Sci. 44 (1), 97–113. doi:10.18402/resci.2022.01.08 |
Ziemann, S., Müller, D. B., Schebek, L., and Weil, M. (2018a). Modeling the potential impact of lithium recycling from ev batteries on lithium demand: A dynamic MFA approach. Resour. Conservation Recycl. 133, 76–85. doi:10.1016/j.resconrec.2018.01.031 |
Ziemann, S., Rat-Fischer, C., Müller, D. B., Schebek, L., Peters, J., and Weil, M. (2018b). A critical analysis of material demand and recycling options of electric vehicles in sustainable cities. Matériaux Tech. 105 (5-6), 515. doi:10.1051/mattech/2018028 |
Keywords: new energy vehicles, lithium battery, the chart of lithium flow, material flow, lithium supply and demand bottleneck
Citation: Zheng L, Chen G, Liu L and Hu Y (2022) Tracing of lithium supply and demand bottleneck in China’s new energy vehicle industry—Based on the chart of lithium flow. Front. Energy Res. 10:992617. doi: 10.3389/fenrg.2022.992617
Received: 12 July 2022; Accepted: 02 August 2022;
Published: 15 September 2022.
Edited by:
Weiqiong Zhong, Chinese Academy of Geological Sciences, ChinaReviewed by:
Qiangfeng Li, University of Southern Denmark, DenmarkCopyright © 2022 Zheng, Chen, Liu and Hu. This is an open-access article distributed under the terms of the Creative Commons Attribution License (CC BY). The use, distribution or reproduction in other forums is permitted, provided the original author(s) and the copyright owner(s) are credited and that the original publication in this journal is cited, in accordance with accepted academic practice. No use, distribution or reproduction is permitted which does not comply with these terms.
*Correspondence: Litao Liu, bGl1bHRAaWdzbnJyLmFjLmNu; Yuqi Hu, eXVxaWh1QHN0dW1haWwuaGJ1LmVkdS5jbg==
Disclaimer: All claims expressed in this article are solely those of the authors and do not necessarily represent those of their affiliated organizations, or those of the publisher, the editors and the reviewers. Any product that may be evaluated in this article or claim that may be made by its manufacturer is not guaranteed or endorsed by the publisher.
Research integrity at Frontiers
Learn more about the work of our research integrity team to safeguard the quality of each article we publish.