- 1School of Petroleum Engineering, China University of Petroleum, Qingdao, China
- 2Guizhou Panjiang Coalbed Methane Development and Utilization Company Limited, Guiyang, China
- 3Guizhou Research Center of Shale Gas and CBM Engineering Technology, Guiyang, China
- 4State Key Laboratory of Public Big Data, Guizhou University, Guiyang, China
- 5College of Resources and Environmental Engineering, Guizhou University, Guiyang, China
This article focused on the study of pore structure characteristics of Songhe and Linhua coal samples based on mercury injection experiments and high-pressure isothermal adsorption experiments. The results show that the pore structure of coal samples in the two mining areas is obviously different under the influence of metamorphic degree. The pores of Songhe mine coal samples, which are characteristic of middle-rank coal reserves, are predominantly open cylindrical pores with good connectivity to medium-to-large pores but poor absorption capacity. The tiny and medium pores of Linhua coal samples, which are characteristic of high-level coal reserves, have poor connectivity, dominated by conical pores with mostly closed and semi-closed pores. The pore size distribution of the middle-rank coal sample is multi-peaked, with better developed pore sizes, while the pore size distribution of the Linhua coal sample is bi-peaked, with micro and small pores developed. The pore fractal shape of high-rank coal reserves is higher than that of middle-rank coal reserves, and the complexity of permeable pore space and diffusive pore space of high-order coal reservoirs is higher than that of middle-order coal reservoirs.
Introduction
As an unconventional and clean energy source, coalbed methane (hereinafter referred to as CBM) bears great significance for safety, economy, and environmental protection, and its development is important for China’s economic development, energy upgrading, and environmental improvement. As an important coal and CBM resource area in southern China, the degree of coal maturity in coal reserves in the western part of the province is mainly of middle and high rank. The differences in pore distribution, pore morphology, and pore fractal shape of coal reserves profoundly affect the transport mode and production difficulty of CBM at microscopic scale, and the study of the micro-nano pore structure of coal reservoirs is the basis for revealing the enrichment-transport mechanism of CBM, which restricts the gas content and recoverability of coal reserves (Qi et al., 2012; Jiang et al., 2013). Studying the developmental characteristics of coal reservoirs in terms of pore size distribution, pore morphology, and connectivity can help deepen the understanding of the microscopic storage space of CBM, which is essential to the engineering implications for CBM development and resource evaluation (Li et al., 2017; Zhang and Li, 2019). The practice of CBM development shows that coal reserves in various mining areas exhibit significant physical differences in the gas content, permeability, and stress response, and scholars mainly study the effect of the pore structure characteristics of coal on the permeability of coal reservoirs at home and abroad (Gao and Yi, 2016; Deng et al., 2020). It is for this reason that coal samples from different mines were selected for industrial analysis, high-pressure mercury injection experiments, and low-temperature nitrogen adsorption experiments to analyze the pore structure characteristics and fractal characteristics of coal samples with different degrees of maturity and to explore the differences in the micro- and nano-scale pore structure of coal reservoirs in the two mines. The coal samples were taken from two typical mining areas, Linhua and Songhe, respectively. The coal samples taken reflect the pore development characteristics of coal samples with different degrees of metamorphism in Guizhou province, which all belong to the Longtan Formation, which is typically representative of the samples. The period of Longtan mainly consists of two major regressive and transgressive cycles, and the coal-forming sedimentary environment was predominantly the lagoon-tidal flat-delta system.
Experimental samples
The main sedimentary facies in the Songhe mining area are the delta plain in the Longtan Formation. All of the Songhe mining area is located between the fault zones (Luo et al., 2016; Wu et al., 2018; Yang et al., 2019). Affected by tectonism in the Songhe mining area in western Guizhou, the coal structure of the main coal seams in the mining area is mainly massive and fragmentary, with a small amount of fine-grained and silty particles. The color of the coal is mainly black-dark gray, with its luster being primarily glassy. The coal reserves are extremely non-homogeneous, as shown by the diversity of macroscopic coal rock types between different coal seams or vertically and laterally on the same coal seam, but semi-bright and semi-dark coals are predominant. The coal rank is mainly dominated by coking, lean, and fat coals with a medium degree of maturity and a strong gas generation capacity, and the organic microfraction is dominated by vitrinite (Hou, 2016; Jin, 2016; Jin et al., 2017).
The main sedimentary facies in the Linhua mining area are the lagoons of the Longtan Formation period and controlled by the Xinhua syncline (Xu et al., 2015). The coal structure of the Linhua mine, under the tectonic influence, is dominantly a primary and fractured structure, which is conducive to fracturing and production improvement, but the thicker coal seams with an individual thickness of over 3.0 m are prone to fractured coal and cinder coal. The types of coal rock are predominantly semi-bright and semi-dark, with the degree of maturity close to each coal seam, and the coal types are all anthracite (Long et al., 2011; Gao et al., 2017; Wang et al., 2017; Fang et al., 2021).
Methods of industrial analysis of coal samples from the two mines were carried out according to the standard industrial analysis method for coal (GB/ T212—2008), and the analysis results are shown in Table 1. As can be seen from Table 1, all of the coal samples from Songhe mine are medium-ash, medium-high volatile coking coals; the coal samples from the Linhua mine are characterized as medium-ash, low-volatile anthracite coals.
Experimental methods
The pore structure and types of coal samples are complex, with pore size distributions ranging from the nanometer to the millimeter scale (Figure 1). In order to fully characterize the pores of coal samples, the high-pressure mercury-compression method can be used to determine the full-scale pore (adsorption pore-percolation pore) distribution characteristics, but the accuracy of pore characterization below 50 nm is reduced due to pressure damage, while the low-temperature nitrogen adsorption test is more accurate for pores below 50 nm (adsorption pore) and can also reflect the pore structure morphology by hysteresis loop characteristics. Both the mercury injection method and the low-temperature nitrogen adsorption method are complementary in pore characterization.
High-pressure mercury injection experiment
Mercury injection experiments on coal samples were performed by means of a Micromeritics Autopore IV 9500 porosimeter. The coal samples were crushed and sieved into 60 meshes (250 μm) and dried in a vacuum at 90°C for 4 ∼ 5 h. The expansion joint was selected and cleaned with anhydrous ethanol and blow dried and weighed, and then put into the instrument for testing. The pore development of the coal samples was calculated from the Washburn equation.
Cryogenic liquid nitrogen adsorption experiment
Based on the supercritical nature of nitrogen at low temperature and pressure, the determination of the microscopic pore structure of the coal samples from both the mines was completed using an ASAP 2020M fully automated specific surface area and microporous physisorption analyzer. The coal sample was crushed and sieved into 100-mesh 0.35 g coal grain sample and dried under a vacuum at 150°C for 4–5 h. Then, after removing the water and volatile gas from the coal sample, it was put into the apparatus at −195.85°C low-temperature liquid nitrogen for the nitrogen desorption test. The specific surface area of the coal samples was obtained by BET analysis, and the pore volume and pore size distribution of the coal samples were obtained by the BJH method.
Experimental results and analysis
High-pressure mercury injection experiment
The advancing and retreating mercury curves of typical coal samples from the two mines can reflect the pore morphology and connectivity of the two coal samples. The micro- and nano-scale pore morphology of coal is divided into open pores, transitional pores, and closed pores. The three pore forms for CBM transport benefits are as follows: open > transitional > closed. Analysis of the advancing and retreating mercury curves of typical coal samples (Figure 2) shows that the advancing and retreating mercury curves of Songhe coal samples have obvious differences. The retreating mercury rates of SH-1 and SH-2 are 41.3 and 45.8%, respectively, with obvious hysteresis loops, indicating that the pores are mainly open pores, and the connectivity of medium and large pores is better. From the perspective of CBM extraction, the coal seam will exhibit a significant hysteresis loop under unloading conditions, and CBM extraction is easy. For the Songhe mine area, effective measures should be developed to eliminate protrusions, such as using top or bottom pumping lanes, hydraulic punching, and other measures to pump and release coal bed methane.
The difference between the advancing and retreating mercury curves of Linhua coal samples is small, and the retreating mercury rates of LH-1 and LH-2 are 21.5 and 14.1%, respectively, indicating that the pore connectivity between the mini and mesopores of coal samples is poor, and the pores are not uniformly distributed and are mostly closed and semi-closed pores. From the perspective of CBM extraction, the seam is not conducive to CBM extraction, and the pore connectivity should be modified. Corresponding measures to increase permeability should be taken for the Linhua mine, such as hydraulic fracturing and carbon dioxide repulsion, to improve permeability.
High-pressure mercury injection experiments have high accuracy for pores above 100 nm, focusing on research in percolation pores. When the mercury injection method is used to characterize the pore structure characteristics of coal samples, the pore diameter of coal samples can be divided into macropores (r > 103 nm), mesopores (102 nm < R < 103 nm), minipores (10 nm < R < 102 nm), and micropores (r < 10 nm), and in order to avoid the micro fracture error generated artificially in the sample preparation process, the pore diameter data greater than 104 nm are deleted to study the pore structure characteristics of coal samples in the two mining areas.
The pore diameter and pore volume distribution characteristics of coal samples are shown in Table 2. From Table 2, it can be seen that the pores of the Songhe coal sample are developed with the average value of total pore volume reaching 0.0314 ml/g, and the micropores and minipores are extremely developed, with the average values of relative pore volume reaching 23.3 and 30.24%, respectively. The average values of the relative pore volume of mesopores and macropores are relatively high, with the average values of relative pore volume of medium and large pores reaching 21.95 and 24.59%, respectively. It shows that the development degree of mesopores and macropores in the Songhe coal sample is dominant, thus presenting a greater contribution to the total pore volume. However, the pore development of the Linhua coal sample is relatively low compared to the Songhe coal sample, and the mean value of total pore volume is classified as 0.01745 ml/g, with an uneven and polarized pore size distribution. The pore size distribution of Linhua coal samples is concentrated in micropores and minipores, and the average relative pore volume is 40.89 and 41.67%, respectively. The development degree of mesopores and macropores is low, with the average relative pore volumes reaching 7.69 and 9.76%, respectively.
The mercury retrieval efficiency is controlled by the microstructure characteristics of the reservoir. The relatively developed nanopores of the test samples are the main factors affecting the mercury retreating efficiency, resulting in the failure of mercury to withdraw completely. The pore development degree of the Songhe coal sample is significantly higher than that of the Linhua mining area, and there is little difference between the two mining areas, but the development degree of minipores, mesopores, and macropores of the Songhe coal sample is higher than that of the Linhua mining area, which is the main factor leading to the differences in pore development between the two mining areas. The pore structure of the Linhua coal sample is conducive to the storage of CBM but not to the percolation of CBM in the reservoir, and the pore structure will lead to low mercury retreating efficiency in the coal sample of the Linhua mine, which requires permeability enhancement measures to improve gas permeability.
The relationship between stage mercury injection and pore size is shown in Figure 3. The stage mercury injection curve of the Songhe coal sample is divided into three stages: the first stage, i.e., in the range of R > 102 nm. When the external pressure is small, mercury is first pressed into the macropores and mesopores of the coal sample. The rise of the stage mercury injection curve is large, and there are multiple peaks, indicating that there are large quantities of macropores and mesopores with complex pore structures. In the second stage, i.e., in the range of 10 nm < R < 102 nm, when the external pressure increases gradually, mercury is pressed into minipores, and a peak appears in this stage. In the third stage, in the range of R < 10 nm, when the pressure continues to increase beyond a certain value, mercury is pressed into the micropores, and the stage mercury injection curve shows a surge phase and peaks. The stage mercury injection curve shows a multi-peak type, indicating that the pore structure of the Songhe coal sample is complex, and the pore structure of each pore size is mainly composed of minipores and micropores with a large proportion of mesopores and macropores.
The stage mercury injection curve of the Linhua coal sample is divided into three stages: in the first stage, i.e., in the range of R > 102 nm, mercury is pressed into the mesopores and macropores of coal rock, and the stage mercury injection curve remains in a flat state. This is also the stage that shows that the mercury injection rate maintains a balanced relationship with the pore diameter of the coal sample. In the second stage, i.e., in the range of 10 nm < R < 102 nm, when the pressure increases gradually, mercury is pressed into the minipores, and the mercury injection curve shows an upward stage and reaches the first peak. In the third stage, i.e.,, in the range of R < 10 nm, when the pressure continues to increase and breaks through a certain value, mercury will be pressed into the micropores and reach a peak. The stage mercury injection curve shows a bi-peaked type, indicating that the pore structure of the Linhua coal sample is mainly microporous and miniporous with a small portion of mesopores and macropores.
It can be concluded that the differences between typical coal samples in Songhe and Linhua mining areas lie in the first stage, i.e., the range of R > 102 nm. The mercury injection curve of Songhe coal samples for mesopores and macropores is significantly higher than that in the Linhua mining area, and the pore size distribution is complex. This is because the Linhua coal sample is anthracite, and its degree of maturity is significantly higher than that of the Songhe coal sample. The Linhua coal sample with high degrees of maturity is affected by stratum compaction, and the macropores and mesopores are compacted, resulting in the significant reduction of pores of these two types in quantity.
Low-temperature nitrogen adsorption experiment
High-pressure mercury injection experiments can destroy coal matrix pores and have nanoscale pore development in coal reservoirs, and nanoscale pore characterization is not accurate, whereas low-temperature nitrogen adsorption tests have no destructive effect on coal pores and characterize nanoscale pores with high accuracy. High-pressure mercury injection experiments and low-temperature nitrogen adsorption experiments have their own advantages in analyzing the coal pore structure and are also of good complementarity to each other (Hu et al., 2021).
Based on the experimental data, the BET equation is used to calculate the specific surface area of the test sample, and the BJH method is used to calculate the pore size distribution of the sample. The experimental results show that the BET surface area of the Songhe coal sample is 12.235–13.018 cm2/g, the pore volume is 0.00744–0.00835 cm3/g, and the average pore size is about 19.3099–22.6351 nm (Table 3). The BET surface area of the Linhua coal sample is 19.5566–26.9852 cm2/g, the pore volume is 0.01766–0.02559 cm3/g, and the average pore diameter is around 11.2018–19.3554 nm. The results show that the development degree of the adsorption pore (r < 50 nm) of the Linhua coal sample is higher than that of the Songhe coal sample, and the adsorption capacity of the Linhua coal sample is higher than that of the Songhe coal sample. The southern part of the Linhua mining area is controlled by the Xinhua syncline; this is because the Linhua coal sample is subjected to strong tectonic compaction, and the percolation pore (r > 50 nm) is compacted and transformed into an adsorption pore (r < 50 nm), resulting in a high degree of adsorption pore development (Figure 4). However, the Songhe coal mine area is located in the triangle formed by the Shizong-Guiyang fault, the Ziyun-Shuicheng fault, and the Panxian-Shuicheng fault. The northern part of the Tucheng syncline belongs to a stable structural zone (Dou et al., 2014; Xu et al., 2015; Luo et al., 2016; Wu et al., 2018). This may be the reason for the variation in the different pore types.
The essence of the liquid nitrogen adsorption and desorption processes of coal samples is the phenomenon that nitrogen condenses and evaporates in the coal pores. According to the classification standard of IUPAC for the types of hysteresis loops (Zhao et al., 2017), the pore morphology is divided into four categories: cylindrical, fractured, conical, and ink-bottle. The ink bottle-shaped pores can produce adsorption loops, but the desorption curve will have an inflection point of sharp decline (Figure 4). According to the types of hysteresis loop, the pore morphology of coal samples in the two mining areas is obviously different.
The adsorption curve of the Songhe coal sample shows that the isotherm of the coal sample is concave and presents extremely weak adsorption. When the relative pressure is less than 0.9, the increase in the adsorption capacity remains low. When the relative pressure is greater than 0.9, the increase in the adsorption capacity rises sharply. It can be seen that when the relative pressure is less than 0.9, what occurs predominantly is single-molecule adsorption. When the relative pressure is greater than 0.9, the multi-molecular layer adsorption increases, and capillary condensation occurs, resulting in a sharp increase in the adsorption capacity. The whole adsorption curve is obviously different from the desorption curve. The hysteresis loop type is type A, indicating that the pore shape is cylindrical.
The adsorption curve of the Linhua coal sample shows that the adsorption increase is small when the relative pressure is low, and when the relative pressure is high, the adsorption increase is large. In the early stage, it is mainly monolayer adsorption, and in the latter stage, it is mainly multilayer adsorption. The adsorption curve and desorption curve are obviously separated, forming a large hysteresis loop, which is similar to type C and has no inflection point, indicating that the pore shape is mostly conical (Figure 5).
Pore fractal characteristics
Fractal theory has been widely used to analyze the irregularity and complexity of the pore structure in porous media. According to the percolation theory, in the process of a high-pressure mercury injection experiment, mercury will enter the sample in two stages. 1) Low pressure stage: when the pressure does not reach the threshold value, mercury is first pressed into the open macropores and microfractures, and mercury mainly flows in the form of percolation. The fractal dimension of this stage is called the percolating fractal dimension Ds; 2) the fractal dimension of mercury diffusion along the pore throat: when the mercury pressure reaches the pore pressure threshold, the fractal dimension is called the percolating fractal dimension Dk. In these two stages, mercury will form two different fractal geometries, which are formed with different fluid flow modes, thus possessing different fractal characteristics (Jiang et al., 2013; Huang and Collier, 2021). Fu et al. (2005) classified coal pores naturally by taking the coal pore diameter as 65 nm. Coal pores are divided into percolation pores with a pore size greater than 65 nm and diffusion pores with a pore size less than 65 nm. According to the Washburn equation, the relationship between pore diameter and pressure is as follows:
The fractal characteristics of the pore structure of a coal reservoir have the following relationships when analyzing the high-pressure mercury injection curve:
Logarithm is taken on both sides to get the following:
where SHg is cumulative saturation, in %; Pc is the applied pressure, in MPa; Pmin is the initial pressure, in MPa; α is constant, and D is the fractal dimension. The results are counted with lg (1-SHg) and lg (Pc/Pmin) calculated. By fitting the scatter diagram to obtain the slope k, the fractal dimension of coal pore d = 3 + K can be obtained.
It can be seen from Figure 6 and Table 2 that lg (1-SHg) and lg (Pc/Pmin) show a good linear relationship, especially for the percolation pores (r > 65 nm), indicating that the coal reservoir has obvious fractal characteristics, and the curves of the Songhe coal sample and Linhua coal sample are obviously different. The percolation fractal dimension DS of the Songhe coal sample is 2.927 and 2.942 (Table 4), respectively, and the diffusion fractal dimension DK is 2.258 and 2.430, respectively. The percolation fractal dimension DS of the Linhua coal sample is 2.986 and 2.976, respectively, and the diffusion fractal dimension DK is 2.781 and 2.591, respectively. In conclusion, the pore fractal dimension of the Linhua coal sample is higher than that of the Songhe coal sample, that is, the complexity of the percolation pore and diffusion pore of the Linhua coal sample is higher than that of the Songhe coal sample.
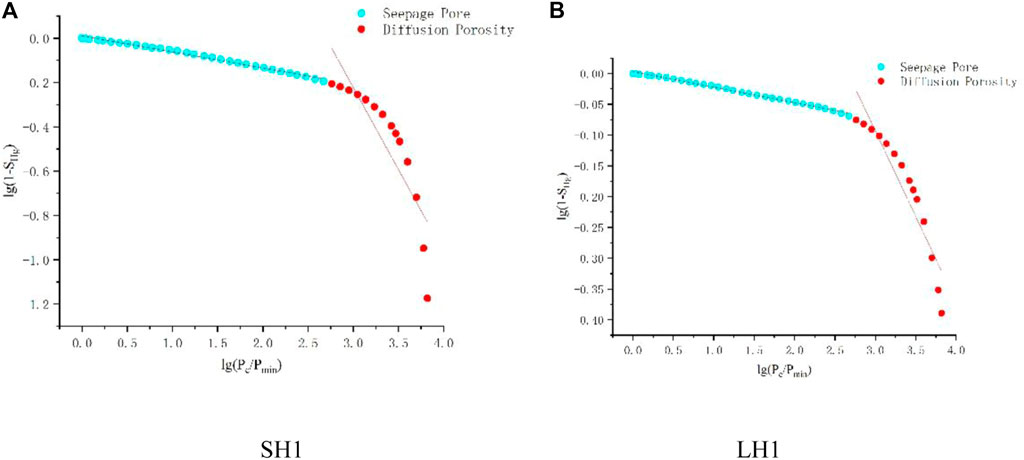
FIGURE 6. Characteristics of the fractal double logarithm curve of the coal sample. (A) SH1; (B) LH1.
Li et al. conducted effective pore correlation analysis on six kinds of coal samples with different metamorphic degrees. Zhang et al. used micro-CT technology to find that coal with a low metamorphic degree had a relatively large internal pore size, and the high metamorphic degree was mainly composed of micropores (Li et al., 2021; Zhang and Wang, 2021). The pore complexity is related to the maturity degree of coal samples. The Songhe coal sample belongs to coking coal with medium maturity, and the Linhua coal sample belongs to anthracite with high maturity. For Linhua coal samples with high maturity, due to intense tectonic compaction, the pore volume decreases and forms an irregular pore structure, which increases the complexity of pores. Other studies have shown that high-rank coal samples have relatively low pore development and an uneven pore size distribution. The high-rank Linhua coal samples have high percolation pores and diffusion pores, and thus, the results are consistent with those of previous studies.
Conclusion
1) Through the mercury injection experiment, it can be seen that there is an obvious difference in connectivity between Songhe and Linhua coal samples, which are characteristics of middle-rank coal reserves and high-rank coal reserves, respectively, and the advancing and retreating mercury curves of middle-rank coal samples have an obvious hysteresis loop, which indicates that the pores are mainly open-type pores, and the connectivity of mesopores and macropores is better. The difference between mercury advancing and retreating curves of high rank coal samples is small. The pore connectivity between minipores and mesopores of coal samples is poor, and the pore distribution is uneven, mostly closed pores and semi-closed pores.
2) The pores of middle-rank coal reserves are developed, in which micropores and minipores are extremely developed, and the development degree of mesopores and macropores is relatively high. The pore size distribution characteristics present a multi-peak type. Compared with middle-rank coal reserves, high-rank coal reserves have relatively low pore development and an uneven pore size distribution, which is realized as two-stage differentiation. The pores are concentrated in micropores and minipores, and the development degree of mesopores and macropores is low. The pore size distribution characteristics are of the bi-peaked type.
3) Nitrogen adsorption experiments show that there are obvious differences in pore morphology between middle- and high-rank reserves. The pore shape of middle-rank coal reserves is mainly cylindrical, and the pore morphology of high-rank coal reserves is mainly conical.
4) The pore volume fractal theory shows that the two pore fractal dimensions of middle-rank coal reserves are lower than those of high-rank coal reserves, and the permeable pores and diffusive pores of middle-rank coal reserves are more complex than those of high-rank coal reserves, which are closely related to the degree of coal sample maturity, and many irregular pores are formed in high-rank coal reserves with a high degree of maturity under strong tectonic compaction, and the complexity of permeable pores and diffusive pores increases.
Data availability statement
The original contributions presented in the study are included in the article/Supplementary Material; further inquiries can be directed to the corresponding author.
Author contributions
YL: data curation, formal analysis, and writing of the original draft. YS: conceptualization and supervision. WG: data curation and formal analysis. WW: supervision and writing—review and editing. WX: writing—review and editing. JC: writing—review and editing. PX: writing—review and editing.
Funding
This work was financially supported by the National Natural Science Fund of China (Grant No. 42002166) and the Guizhou Provincial Fund Project (Grant Nos. (2022)ZD001, (2020)2Y028, and ZK (2022)106).
Conflict of interest
Author YL was employed by Guizhou Panjiang Coalbed Methane Development and Utilization Company Limited.
The remaining authors declare that the research was conducted in the absence of any commercial or financial relationships that could be construed as a potential conflict of interest.
Publisher’s note
All claims expressed in this article are solely those of the authors and do not necessarily represent those of their affiliated organizations, or those of the publisher, the editors, and the reviewers. Any product that may be evaluated in this article, or claim that may be made by its manufacturer, is not guaranteed or endorsed by the publisher.
References
Deng, E., Jiang, B., and Gao, W. (2020). Study on pore structure and fractal characteristics of coal measure shale of Longtan Formation in western Guizhou [J]. Meitan Kexue Jishu(Coal Sci. Technol. 48 (08), 184–190. doi:10.13199/j.cnki.cst.2020.08.023
Dou, X., Jiang, B., and Zhang, W. (2014). Structural deformation Features and its CBM geological significance in western Guizhou[J]. Zhongguo Meitan Dizhi(Coal Geol. China) 26 (08), 54–59. doi:10.3969/j.issn.1674-1803.2014.08.12
Fang, Z., Xu, H., and Sang, S. (2021). Primary selection of target intervals for coal measure gas development in Linhua multi coal seam mine field in northern Guizhou [J]. Meikuang Anquan(Coal mine Saf. 52 (04), 174–181. doi:10.13347/j.cnki.mkaq.2021.04.032
Fu, X., Qin, Y., and Zhang, W. (2005). Study on fractal classification and natural classification of coal pores based on coalbed methane transportation. Kexue Tongbao Science Bull. (S1), 51–55.
Gao, W., Jin, J., and Yi, T. (2017). Characteristics and mining technology of high-rank coalbed methane reservoir in xiaolinhua mining area in northern Guizhou [J]. Yanxing Youqi Cang(Lithologic Reserv. 29 (05), 140–147. doi:10.3969/j.issn.1673-8926.2017.05.017
Gao, W., and Yi, T. (2016). Pore characteristics of coal reservoir and its influence on permeability in Songhe mine field, western Guizhou [J]. Meitan Kexue Jishu(Coal Sci. Technol. 44 (02), 55–61. doi:10.13199/j.cnki.cst.2016.02.010
Hu, T., Pang, X., Jiang, F., Wang, Q., Liu, X., Wang, Z., et al. (2021). Movable oil content evaluation of lacustrine organic-rich shales: Methodsand a novel quantitative evaluation model. Earth-Sci. Rev. 214, 103545. doi:10.1016/j.earscirev.2021.103545
Hou, D. (2016). Study on characteristics and recoverability of coalbed methane reservoir in Songhe mine field, Guizhou [D]. Xuzhou: China University of Mining and Technology.
Huang, Y., and Collier, R. (2021). Micro pore throat structure and fractal characteristics of tight sandstone reservoir -- Taking Upper montney member of block a in western Canada Basin as an example [J]. Xinjiang Shiyou Dizhi(Xinjiang Pet. Geol. 42 (04), 506–513. doi:10.7657/XJPG20210416
Jiang, W., Tang, S., and Zhang, J. (2013). Analysis of porosity and percolation characteristics of high metamorphic stone coal based on mercury injection fractal [J]. Meitian Dizhi Yu Kantan. Geol. Explor. 41 (04), 9–13.
Jin, J., Gao, W., and Sun, J. (2017). Geochemical characteristics and significance of coal forming environment in Central Guizhou Songhe mining area [J]. Meitan Kexue Jishu(Coal Sci. Technol. 45 (12), 166–173. doi:10.13199/j.cnki.cst.2017.12.029
Jin, J. (2016). Vertical distribution characteristics of coal reservoir physical properties of Songcan 1 well in Songhe well field, western Guizhou [J]. Meitan Kexue Jishu(Coal Sci. Technol. 44 (02), 27–32. doi:10.13199/j.cnki.cst.2016.02.005
Li, X., Gao, J., and Zhang, S. (2021). Combined characterization of scanning electron microscopy, pore and Crack analysis system, and gas adsorption on pore structure of coal with different volatilization[J]. Diqiu Kexue(Earth Sci. 47 (05), 1876–1888. doi:10.3799/dqkx.2021.195
Li, X., Shen, Z., and Liu, Y. (2017). Experimental study on the influence of pore structure of structural coal and primary structural coal on desorption characteristics in Northwest Guizhou [J]. Caikuang Yu Anquan Gongcheng Xuebao(Journal Min. Saf. Eng. 34 (01), 170–176. doi:10.13545/j.cnki.jmse.2017.01.026
Long, Z., Li, S., and Han, Z. (2011). Analysis on Influencing Factors of gas geology in Linhua coal mine. Wasi Dizhi Jichu Yu Yingyong Yanjiu, 105–110.
Luo, K., Jin, J., and Zhao, L. (2016). etc Feasibility study on combined seam gas drainage under condition of seam group in Songhe Mine Field. [J] Meitan Kexue Jishu (Coal Sci. Technol. 44 (02), 73–77. 103. doi:10.13199/j.cnki.cst.2016.02.013
Qi, L., Wang, Z., and Yang, H. (2012). Study on coal sample porosity based on low temperature nitrogen adsorption method and mercury injection method [J]. Meitan Kexue Jishu(Coal Sci. Technol. 40 (08), 36–39. doi:10.13199/j.cst.2012.08.39.qill.015
Wang, S., Gao, W., and Guo, T. (2017). Characteristics of coal quality parameters and significance of coal forming sedimentary environment in Linhua coalfield, Northern Guizhou [J]. Meitan Kexue Jishu(Coal Sci. Technol. 45 (10), 162–168. doi:10.13199/j.cnki.cst.2017.10.028
Wu, C., Yang, Z., and Qin, Y. (2018). Geochemical comparison and its geological significance of CBM produced water in the Songhe and Zhijin blocks[J]. Meitan Xuebao J. China Coal Soc. 43 (4), 1058–1064. doi:10.13225/j.cnki.jccs.2017.1087
Xu, H., Sang, S., and Jin, J. (2015). CBM resources distribution and assessment in Linhua Minefield, Jinsha, Guizhou[J]. Zhongguo Meitan Dizhi(Coal Geol. China) 27 (04), 16–20. doi:10.3969/j.issn.1674-1803.2015.04.04
Yang, Z., Qin, Y., Wu, C., Qin, Z., Li, G., and Li, C. (2019). Geochemical response of produced water in the CBM well group with multiple coal seams and its geological significance-A case study of the Songhe well group in Western Guizhou. Int. J. Coal Geol. 207, 39–51. doi:10.1016/j.coal.2019.03.017
Zhang, C., and Li, S. (2019). Pore structure characteristics and gas adsorption characteristics of low permeability coal [J]. Meikuang Anquan(Coal mine Saf. 50 (01), 21–24. doi:10.13347/j.cnki.mkaq.2019.01.006
Zhang, W., and Wang, J. (2021). Characterization of microscopic pore structure of different coal types based on micro CT[J]. Meitan Kexue Jishu (Coal Sci. Technol. 49 (S2), 85–92.
Keywords: Songhe mine, Linhua mine, pore structure, fractal dimension, coal
Citation: Lou Y, Su Y, Gao W, Wang W, Xiong W, Chen J and Xia P (2022) Pore characteristics and fractal characterization variability of typical middle- and high-rank coal reserves: A case study of middle- and high-rank coal samples in western Guizhou. Front. Energy Res. 10:981530. doi: 10.3389/fenrg.2022.981530
Received: 29 June 2022; Accepted: 08 August 2022;
Published: 06 September 2022.
Edited by:
Tao Zhang, Southwest Petroleum University, ChinaReviewed by:
Wei Li, Northeast Petroleum University, ChinaTao Hu, China University of Petroleum, China
Yanjun Meng, Taiyuan University of Technology, China
Copyright © 2022 Lou, Su, Gao, Wang, Xiong, Chen and Xia. This is an open-access article distributed under the terms of the Creative Commons Attribution License (CC BY). The use, distribution or reproduction in other forums is permitted, provided the original author(s) and the copyright owner(s) are credited and that the original publication in this journal is cited, in accordance with accepted academic practice. No use, distribution or reproduction is permitted which does not comply with these terms.
*Correspondence: Yuliang Su, suyl@cup.edu.cn