- 1Collage of Materials Science and Engineering, Central South University of Forestry and Technology, Changsha, China
- 2College of Science, Central South University of Forestry and Technology, Changsha, China
Potassium ion battery (PIB) is one of the promising substitutes for lithium ion battery. Developing new anode materials is still one of the key factors to promote the electrochemical performances of PIB. Electrospun carbon nanofibers based binder-free electrodes have received widely researches because multi-advantages of good conductivity, large surface areas and structural stability. In this review, the latest research progress in enhancing the potassium ion storage properties based on electrospun carbon nanofibers through introducing porous structure, doping heteroatoms and preparing composites have been summarized. The effects of pore structure, heteroatom types and non-metal/metal loading on specific surface area, conductivity, lattice distance and potassium storage performance of the electrospun carbon nanofiber electrode were emphatically discussed. In addition, several suggestions for industrial application of electrospun carbon nanofiber electrodes for potassium ion storage were proposed in this review.
1 Introduction
As the main power source for portable electronic devices and electric vehicles (EVs), and energy storage systems for wind and solar energy, lithium-ion batteries have achieved great success in commercial applications (Hameer and van Niekerk, 2015; M. Gür, 2018; Zhong et al., 2022). It is supposed that the global demand for energy storage devices will be close to 900 Gwh in 2025. Nevertheless, the global reserves of lithium are scarce and unevenly distributed, resulting in difficulties in meeting the market demand. In addition, the failure to reduce the cost of lithium-ion batteries will seriously affect their long-term development (Gu et al., 2017; L. Kulova et al., 2020; Wang et al., 2022a). Therefore, it is imperative to investigate inexpensive battery systems to resolve the above problems.
Potassium, located in the alkali metals group, possesses analogous physical and chemical properties to lithium. Furthermore, the high crust abundance of potassium (K: 1.5 wt%; Li: 0.0017 wt%) and similar redox potential to lithium (K+/K: −2.93 V vs. SHE; Li+/Li: −3.04 V vs. SHE), making potassium ion battery being one of the potential substitutes for lithium ion battery (Hwang et al., 2018; Zhang et al., 2021; Li et al., 2022).
During the commercialization process of potassium ion battery, several problems still need to be resolved. For instance, the large radius of potassium ion (K+: 1.38 Å; Li+: 0.76 Å) may lead to pulverization of active materials during the repeated potassiation/depotassiation procedure, resulting in poor cycling stability (Zhang et al., 2018a; Deng et al., 2021; Sun et al., 2022a). In addition, the relatively sluggish diffusion kinetics of large K ions in the solid electrode leads to inferior rate performance (An et al., 2018; Liu et al., 2018; Cao et al., 2021a). Therefore, it is significant to select suitable anode material and optimize structure from the microscopic point of view to prompt the commercial application and development of potassium ion batteries.
Among numerous kinds of anode materials, carbon based materials are still considered to be the most promising electrode materials for commercial potassium ion batteries because of their advantages of good chemical stability, high conductivity and environmental friendliness (Bin et al., 2018; Cao et al., 2021b; Hong et al., 2021; Thakur et al., 2022). In particular, carbon nanofibers prepared via an electrospinning technique are regarded as a kind of potential anode materials for potassium ion storage based on the following advantages: 1) the one-dimensional structure of nanofibers can offer a rapid transfer channel for electrons; 2) the unique network architecture is good to infiltration of electrolyte, alleviating the concentration polarization; 3) electrospun carbon nanofibers can be directly used as self-supporting electrode for their high flexibility and excellent tensile strength. In conclusion, the above superiorities of electrospun carbon nanofibers can endow fast kinetics and robust structural integrity of electrodes, improving energy density, power density and cycling stability of battery (Chen et al., 2020; Zheng et al., 2021a; Yuan et al., 2021).
2 Application of electrospun carbon nanofibers in potassium ion battery
2.1 Electrospun carbon nanofibers anode
In recent years, electrospun carbon nanofibers electrode has been verified as a successful anode for potassium ion batteries (Zhang et al., 2020a; Touja et al., 2020). Zhao et al. applied free-standing porous carbon nanofiber paper as anode of potassium ion battery, and the results revealed enormous potential of carbon nanofibers as potassium ion anode electrode (reversible capacity of 211 mAh g−1 after 1,200 cycles at current density of 0.2 A g−1 and remain 100 mAh g−1 at 7.7 A g−1) (Zhao et al., 2017). Tian et al. (2020) prepared highly graphitized carbon nanofibers (HG-CNFs) through carbonization at 1,400°C after electrospinning and pre-oxidization procedures. When the free-standing electrode was served as the anode for potassium ion batteries, the HG-CNFs electrode exhibited a high reversible capacity (200 mAh g−1) below 0.2 V (Figure 1), which is beneficial to acquiring high energy density. In addition, the electrode also obtained superior rate (226 mAh g−1 at 35 C (1 C = 279 mA g−1)) and stability performances (less than 0.008% per cycle during 400 cycles) resulted from the freestanding fibrous networks and highly graphitic structure.
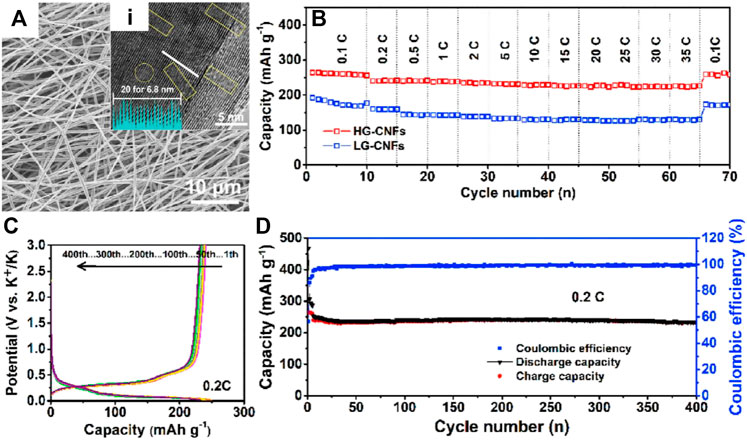
FIGURE 1. (A) SEM and (i) HRTEM images of HG-CNFs. (B) Comparison of the rate capability from 0.1 to 35 C of lowly and highly graphitized carbon nanofibers (LG- CNFs and HG-CNFs). (C) The charge-discharge curves after different cycles at 0.2 C (D) Long-term cycle stability and its coulombic efficiency of HG-CNFs at 0.2 C (Tian et al., 2020).
In order to further boost the potassium ion storage performances of electrospun carbon nanofibers, several kinds of modification methods on electrospun carbon nanofibers were studied. Particularly, designing porous structure in electrospun carbon nanofibers is an efficient way, which has been demonstrated to provide abundant active sites for the adsorption of K+, and further promoted the capacitive contribution to the potassium-storage capacity beyond the intercalation mechanism (Li et al., 2018; Zheng et al., 2021b; Zhou et al., 2021; Sun et al., 2022b). Furthermore, porous structure can provide sufficient spaces to mitigate large volume change, achieving long cycling stability (Xie et al., 2017; Chen et al., 2018). Zhang et al. (2019) fabricated a free-standing carbon nanofibers anode with hierarchical porous structure (Figures 2Ai–iv) by using polymethyl methacrylate (PMMA) as foaming agent, which was thermal decomposed under 240–280°C. The electrode exhibited great potential as binding-free anode in potassium-based dual-ion batteries, which showed a high reversible capacity of 197 mAh g−1 (50 mA g−1) and excellent cycling stability (65 mAh g−1 after 346 cycles at a specific current of 100 mA g−1). Chen et al. (2022) further investigated the influence of PMMA content on pore structure and battery performances, demonstrating the porous richness and surface roughness of nanofibers were enhanced with the increase of PMMA and obtaining enhanced potassium ion storage performance (Figure 2B). Wu et al. (2021) synthesized macroporous honeycomb-like carbon nanofibers (MHCNFs) by using poly(vinyl alcohol) (PVA) and poly(tetrafluoroethylene) (PTFE) as precursors, where PTFE mainly played the role in forming pore substance. The binder-free electrode exhibited ultrahigh potassium storage ability and competitive rate capability, maintaining 252.6 mAh g−1 over repeating 2,500 cycles at 1,000 mA g−1 (Figure 2C). Besides these easily decomposed organic matter as pore-making agent, metal based substance containing ZnCl2, ZIF-8, Sb2S3 and so on were applied as well, and the obtained electrode exhibited good potassium ion storage performances (Kim et al., 2013; Hu et al., 2020; Liu et al., 2022).
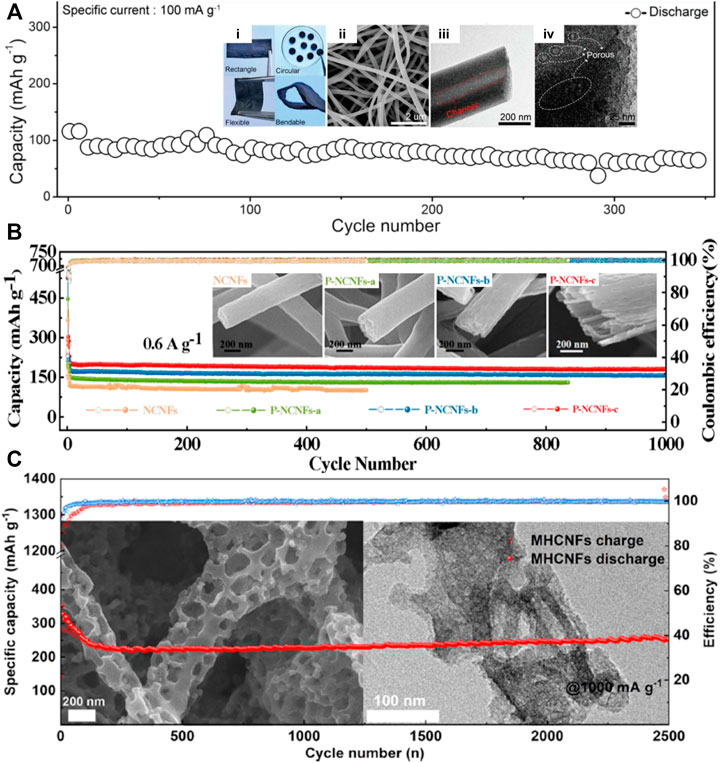
FIGURE 2. (A) Cycling stability of the potassium-based dual-ion batteries based on the hierarchically porous carbon fibers anode at a specific current of 100 mA g−1. (i) Optical images of the hierarchically porous carbon fibers tailored into different shapes and bended to different states. (ii) SEM image of the hierarchically porous carbon fibers electrode. (iii) and (iv) Normal and enlarged TEM images of a single fiber in the electrode (Zhang et al., 2019). (B) Cycling potassium ion storage performances of electrodes with different PMMA contents (a: 0.4 g; b 0.8 g; c 1.2 g) at a current density of 0.6 A g−1 (Chen et al., 2022). (C) Cycling behavior of MHCNFs at 1,000 mA g−1 (Wu et al., 2021).
Doping heteroatom (N, O, S, P etc.) in carbon nanofibers is also verified an as effective strategy to boost the potassium ion storage property on the basis of the following reasons: 1) heteroatom doping can enhance the electronic conductivity of carbon nanofibers through tuning the local electronic structure to store charge on the surface of the carbon lattice (Ma et al., 2019); 2) heteroatom doping can provide large numbers of active sites for adsorbing K+ (Tao et al., 2020); 3) heteroatom doping can increase the interlayer distance of carbon nanofibers for their large covalent radius (Wang et al., 2022b).
In particular, the N atom is the most common doped element in electrospun carbon nanofibers because the carbon resource is always derived from PAN, which contains N element. Therefore, the in-situ the N doped carbon nanofibers can be easily synthesized during the carbonation process. N-doping has been verified to enhance reactivity by generating more defects and increase conductivity by ameliorating the locally electronic configuration (Xu et al., 2018; Yang et al., 2019; Pei et al., 2020). N-doped carbon nanofibers prepared by Chen et al. (2022) showed higher specific capacity and better rate performance than the non-atom doped carbon nanofibers used PVA as precursor.
On this basis, bi-heteroatom co-doped in carbon nanofibers were also received extensively research and expected to acquire high-performance carbon nanofibers anodes through synergistic effect between two different heteroatoms. Adams et al. (2017) has obtained binder-free N- and O- rich carbon nanofiber anodes (CNF-O) through plasma oxidation treatment with electrospun carbon nanofibers derived from PAN. They discovered that the surface area of CNF-O has been increased from 139 to 153 m2 g−1 after plasma oxidation treatment, which resulted in a higher coulombic efficiency (99.5%) than CNF (97.5%) and increased pseudo-capacitance behavior (Figure 3A). Li et al. (2020) also obtained N and O codoped electrospun carbon nanofibers via the precursor of PAN and preasphaltene (PA), and the electrode delivered an impressive potassium ion storage rate (103 mA h g−1 at 1 A g−1) and discernible cycling performance with a capacity retention of 98% even after 320 cycles. Recently, Zheng et al. (2022) still optimized the interlayer spacing through N, O codoping in carbon nanofibers, and assosiated with the active sites induced by N, O heteroatom-work together to provide an intercalation-adsorption mechanism for storing K+ in carbonaceous materials.
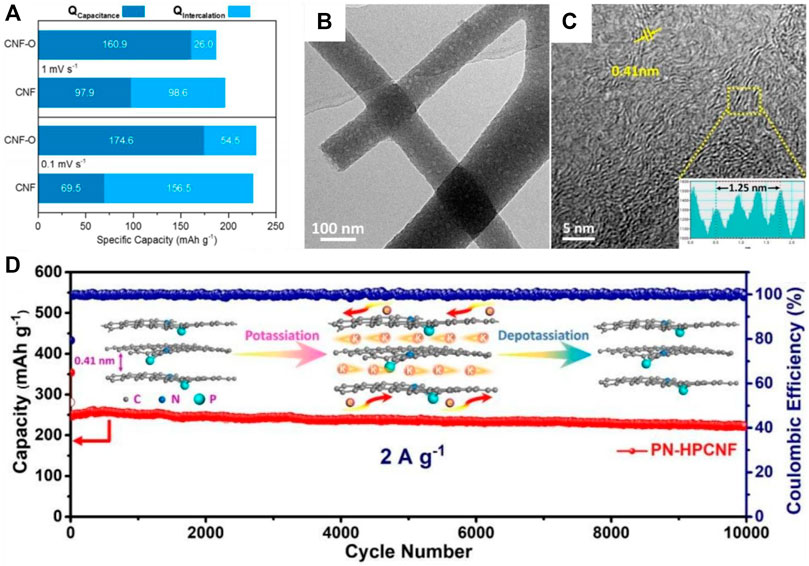
FIGURE 3. (A) Comparison of charge storage mechanism from intercalation and capacitance contributions for CNF and CNF-O at 0.1 and 1 mV s−1 scan rates (Adams et al., 2017). (B) TEM image of PN-CNF. (C) HRTEM image of PN-HPCNF. (D) Long-term cycling performance of the PN-HPCNF at 2.0 A g−1 (Hu et al., 2020)
Hu et al. (2020) obtained phosphorus/nitrogen co-doped hierarchical porous carbon nanofibers (PN-HPCNF) by using triphenylphosphine (TPP), urea and polyacrylonitrile (PAN) as P, N and C resources. Characterization results displayed that P/N co-doped can introduce numerous mesopores and micropores in the carbon nanofibers (PN-CNF) for the reason of released gases (such as PH3, NH3, etc.) during annealing procedures (Figure 3B). In addition, an expanded interlayer spacing (0.41 nm) of PN-HPCNF has been obtained, which is much wider than that of N-HPCNF (0.37 nm) and HPCNF (0.35 nm) (Figure 3C). The unique structure rooted in P/N doping is contributing to accelerating K-ion intercalation/deintercalation, offering sufficient space to buffer volume variation, and possessing superior potassium ion storage performances (Figure 3D).
2.2 Electrospun carbon nanofibers based composites anode
Numerous attentions have been focused on the electrospun carbon nanofibers based composites anode to further boost the potassium ion storage properties. On one hand, many kinds of active materials were embedded in the electrospun carbon nanofibers, which played a significant role in protecting active materials and obtained high specific capacity, good rate and long cycling performances (Zhang et al., 2016; Wang et al., 2022c). On the other hand, electrospun carbon nanofibers were also applied as the carrier, where the active materials can grow on the surface to prevent agglomeration and further promote the potassium ion storage properties (Xue et al., 2019; YaoLin et al., 2022). At present, active materials, such as non-metal, metallide, metal and alloy and so on, were associated with electrospun carbon nanofibers to acquire composite electrodes.
2.2.1 Non metal
Niu et al. (2021) obtained a high performance potassium ion storage material consisting of porous nitrogen-doped carbon nanofibers with graphene (G-PCNFs). The electrochemical performance manifested that graphene can improve the electrical conductivity of the composite materials (the electrical conductivity of 5.5 S m−1 for G-PCNFs and 1.6 S m−1 for PCNFs. The electrode displayed a high reversible capacity (358 mA h g−1 at 0.1 A g−1 after 200 cycles), excellent rate performance (101 mAh g−1 at 5 A g−1) and ultralong cycling stability (276 mAh g−1 at 2 A g−1 after 2000 cycles). Shen et al. (2019) also investigated the potassium ion storage properties of self-supporting carbon nanotubes encapsulated in sub-micro carbon fiber electrode (SMCF@CNTs). As displayed in Figures 4A,B, the SMCF@CNT anode exhibited high specific capacity, good rate performance, and cycling stability compared with sub-micro carbon fiber (SMCFs). Furthermore, the SMCF@CNT anode was coupled with K0.3MnO2 cathode, and the pouch cell delivered a capacity of 74.0 mAh g−1 at 20 mA g−1 with good flexibility (Figures 4C,D). Wu et al. (2019) designed a unique structure of embedding red phosphorus into free-standing nitrogen-doped porous hollow carbon nanofibers to circumvent the problems of pulverization and aggregation of P particles simultaneously. In this work, the electrode realized unprecedented long cycle life with high reversible capacity (465 mAh g−1 at 2 A g−1 after 800 cycles) as well as outstanding rate capability (342 mAh g−1 at 5 A g−1).
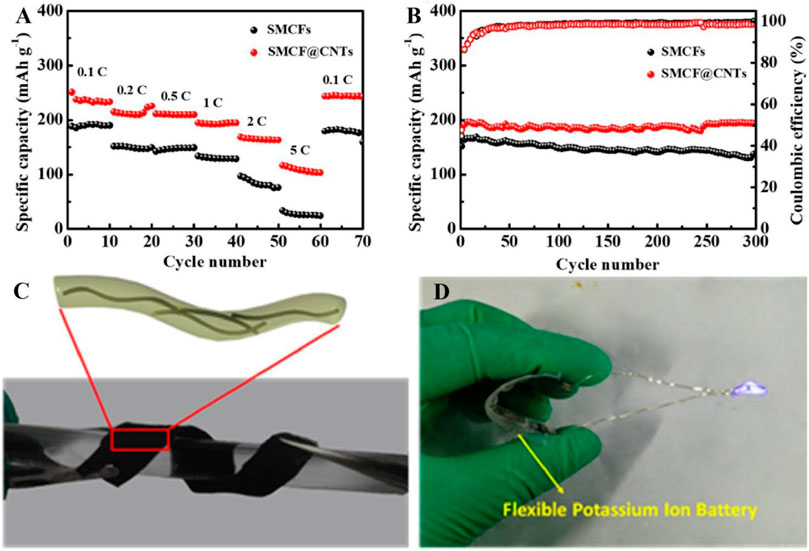
FIGURE 4. (A) Rate performance of SMCFs and SMCF@CNTs from 0.1 to 5 C (1 C = 279 mA g−1). (B) Cycling performance of SMCFs and SMCF@CNTs at a current rate of 1 C. (C) Digital photo illustrating the flexibility of SMCF@CNTs. (D) LED lit by the SMCF@CNT/K0.3MnO2 pouch cell under bending (Shen et al., 2019).
2.2.2 Metallide
Metal oxides showed high specific capacity in lithium storage performance, so the potassium storage performance based on the metal oxides has been widely studied. Huang et al. (2018) synthesized SnO2-graphene-carbon nanofibers electrode for anode of PIBs, where carbon nanofibers and the encapsuled graphene protected the SnO2 from destruction. The electrode exhibited great cycling stability (202.06 mAh g−1 at 100 mA g−1 after 100 cycles) and high rate capacity (114.81 mAh g−1 at 1 A g−1). Jin et al. (2018) fabricated a flexible and self-standing electrode that consisted of V2O3 nanoparticles embedded in porous N-doped carbon nanofibers. The electrode mainly followed the mechanism of intercalation pseudocapacitance and displayed long cycle life.
Large numbers of studies showed that metal sulfide also has good potassium storage performance. Hence, metal sulfides were also used with electrospun carbon fibers to improve potassium storage. Chen et al. (2019) encapsuled FeS2 and graphene in the electrospun carbon nanofibers, resulting in long-term life of K+ storage (120 mAh g−1 at 1 A g−1 after 680 cycles). Lai et al. (2021) closely coupled Co-based ultrafine nanoparticles (CoS2, Co3O4, CoSe, CoTe) and hierarchical porous carbon nanofibers through using precursors of ZIF-67 and PAN (Figure 5). Particularly, the ultrasmall CoS2@hierarchical porous carbon nanofibers (u-CoS2@HCFs) electrode showed high reversible capacity, excellent rate property, and ultralong cycling life (268 mAh g−1 at 500 mA g−1 after 1,000 cycles) when evaluated as anode for PIBs. He et al., (2022) proposed a facile approach to confine SnS nanocrystals in the walls of hollow multichannel carbon nanofibers to tackle the poor electronic conductivity and huge volume expansion of SnS. The electrode showed 415.5 mAh g−1 at 0.1 A g−1 after 300 cycles and 245.5 mAh g−1 at 1 A g−1 after 1,000 cycles. Yang et al. (2021) explored the potassium ion storage performances of In2S3/carbon nanofibers electrode. Owing to the presence of carbon nanofibers and the nano-scale of In2S3 particles, the volume effect of In2S3 was restrained and the conductivity of electrode was improved. Hence, the electrode delivered reversible capacities of 212.2 mAh g−1 even at 1 A g−1. Mao et al. (2018) anchored ReS2 nanosheets onto nitrogen doped carbon nanofibers (ReS2/N-CNFs). The doped nitrogen can absorb sulfur and polysulfide generated by the conversion reaction. As a result, the ReS2/N-CNFs composite anode maintains a reversible capacity of 253 mAh g−1 after 100 cycles at 50 mA g−1 in PIBs. Geng et al. (2021) designed a freestanding PIBs anode through supporting carbon-coated WS2 nanosheets on carbon nanofibers, which possessed fast K+ diffusion kinetics and high K-adsorption ability that verified by first-principles calculations and displayed good potassium ion storage properties. In addition, V5S8 (Liu et al., 2021), NiCo2S4 (Zhang et al., 2020b) etc. were also investigated as anode for PIBs with electrospun carbon nanofibers and all exhibited enhanced potassium ion storage properties than pure metal sulfides.
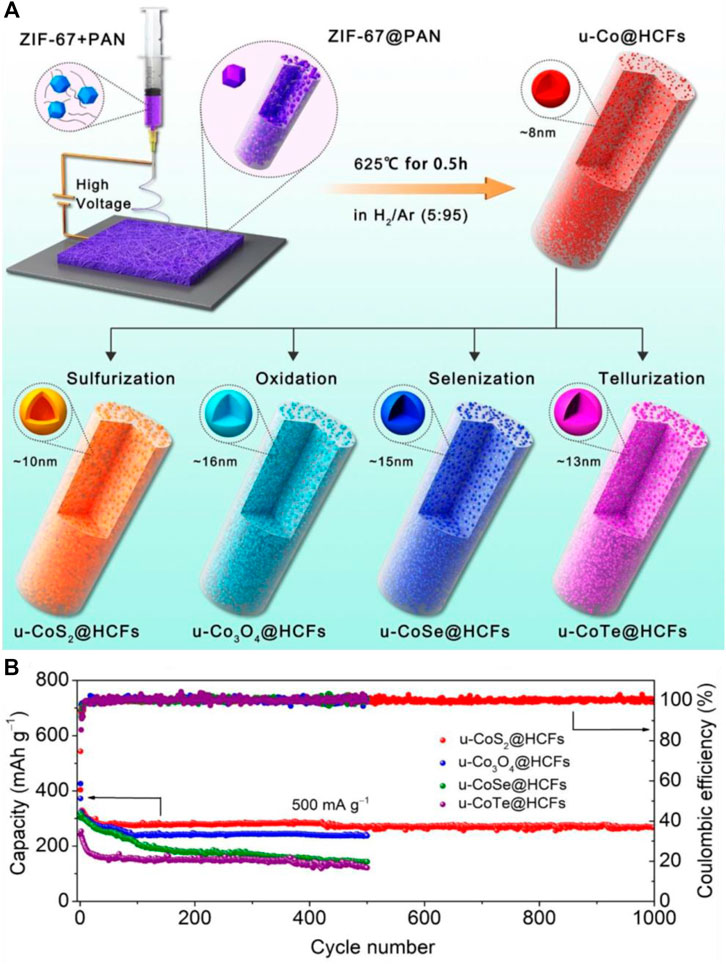
FIGURE 5. (A) Schematic illustration of fabricating hierarchical porous nanofibers with closely integrated ultrasmall CoMx (M = S, O, Se, and Te) nanoparticles and carbon matrix. (B) Long-term cycling stability under 500 mA g−1 of u-CoMx@HCFs (Lai et al., 2021).
Metal selenides have similar properties to metal sulfides, so their potassium storage properties have been studied extensively as well Liao et al., (2019). Xu et al. (2021) embedded V3Se4 nanoparticles in nitrogen/phosphorus co-doped carbon nanofibers, which exhibited an excellent electrochemical performance for PIBs (450 mAh g−1 after 100 cycles at 50 mA g−1 and 207 mAh g−1 after 800 cycles at 2 A g−1). Atangana Etogo et al. (2020) fabricated Co0.85Se@carbon nanoboxes embedded in carbon nanofibers films with flexible and freestanding features. As a result, the unique electrode demonstrated excellent cycling stability (reversible capacity of 299 mA h g−1 at 1 A g−1 after 400 cycles) and remarkable rate performance (166 mA h g−1 at 5 A g−1) as an anode for PIBs. Ho Na et al. (2021) also prepared ultrafine ZnSe nanocrystals encapsuled in N-doped porous carbon nanofibers electrode and applied as anode for PIBs. Consequently, the electrode exhibited excellent long-term cycling stability with a reversible capacity of 270 mAh g−1 at 0.5 A g−1 for 1,000 cycles and a high-rate capacity of 139 mA h g−1 at 2.0 A g−1.
Besides, metallic carbide (MoC2(Min et al., 2021)), metal phosphide (CoP (Miao et al., 2019), FeP (Wang et al., 2020), MoP (Yi et al., 2020)), et al. were applied as anode materials of potassium ion battery with electrospun carbon nanofibers. All electrodes exhibited remarkable potassium ion storage performances.
2.2.3 Metal and alloy
Metal and alloys always displayed high specific capacities for electrochemical storage Zhang et al. (2018b). Therefore, Metal and alloys were also applied as anode for PIBs. Ge et al. (2019) presented a simple strategy that ultrasmall Sb nanocrystals uniformly impregnated in carbon nanofibers containing an array of hollow nanochannels (Figure 6), and the electrode yielded high-performance PIBs with a reversible capacity of 225 mAh g−1 after 2000 cycles at 1 A g−1. In addition, Huang et al. (2021) also fabricated porous Sb-graphene-carbon nanofibers electrode, presenting a great cycle life (204.95 mAh g−1 at 100 mA g−1 after 100 cycles) and excellent rate capability (120.83 mAh g−1 at 1 A g−1). Han et al. (2019) embedded CoSb nanoparticles in carbon nanofibers to facilitate the pseudocapacitive behaviors, and the capacity of electrode maintained 250 mA h g−1 after 500 cycles at 1 A g−1.
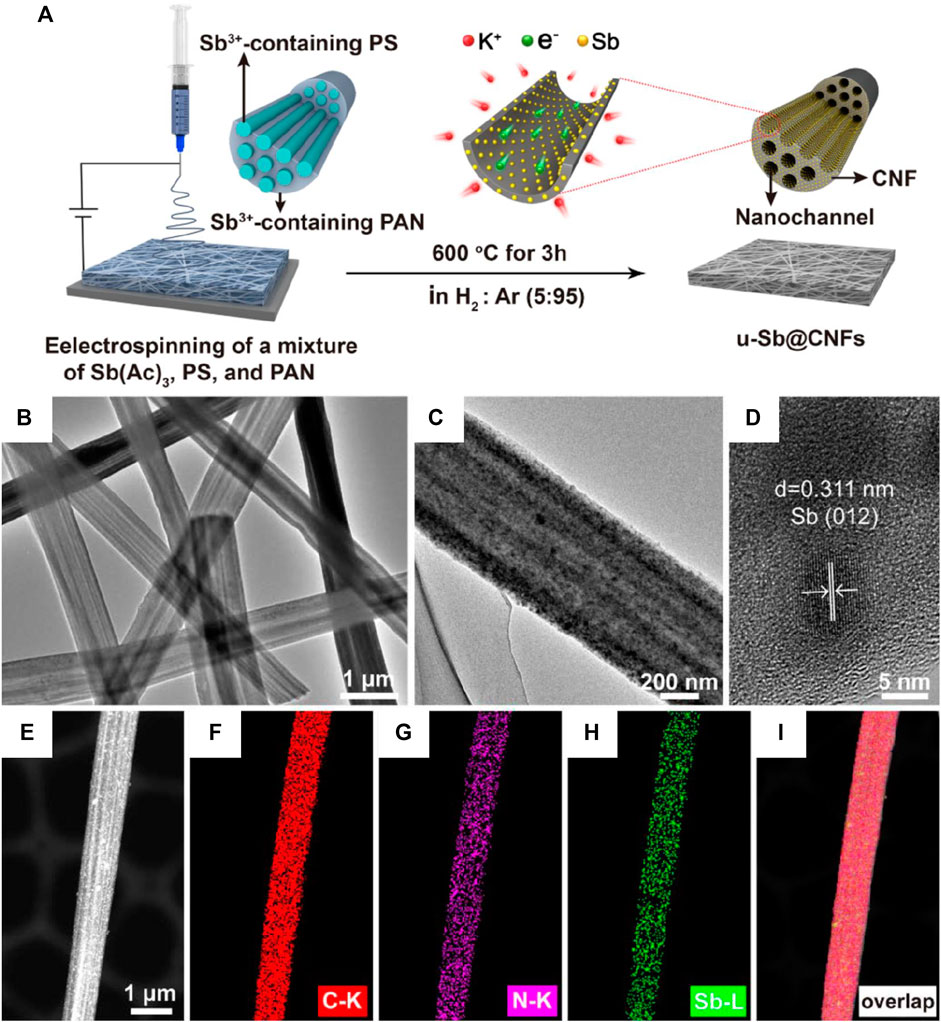
FIGURE 6. (A) Schematic illustration of a simple two-step route to ultrasmall Sb nanocrystals-impregnated carbon nanofibers containing an array of hollow nanochannels (denoted u-Sb@CNFs). (B) and (C) TEM images, (D) HRTEM image, and (E–I) the corresponding elemental mappings of u-Sb@CNFs. (Ge et al., 2019)
3 Conclusion and perspectives
In this review, the potassium ion anode electrodes based on electrospun carbon nanofibers have been concluded. The modification method for electrospun carbon nanofibers including porous structure designation, heteroatoms doping and composites synthesizing were discussed in detail. The effects of modification on electrospun carbon nanofibers specific surface area, conductivity, lattice distance and potassium storage performance of the electrospun carbon nanofiber electrode were emphatically summarized. The treatment of porous structure designation and heteroatoms doping can boost the cycling stability of electrospun carbon nanofibers. The composite preparation always bring high specific capacity. Therefore, combining the advantages of the three modification methods to prepare high performance potassium storage electrode is one of the future development direction.
Although electrospun carbon nanofibers based anodes have exhibited remarkable potassium ion storage performances, the potassium-based full cells and dual-ion batteries should be further researched, including their properties of energy density, power density and cycling life. In addition, Electrospun carbon nanofibers based electrode has been realized the application of PIBs anode directly and possess flexibility feature at some extent. Nevertheless, with the deeply development of flexible batteries, electrospun carbon nanofibers with higher strength will be requested.
Author contributions
MX, Writing manuscript; RL, Consulting and collecting literatures; TY, Writing manuscript; YD, Checking manuscript.
Funding
This work was supported by the National Natural Science Foundation of China (Grant Number 42007138) and the Education Department of Hunan Province (Grant Number 21C0135).
Conflict of interest
The authors declare that the research was conducted in the absence of any commercial or financial relationships that could be construed as a potential conflict of interest.
Publisher’s note
All claims expressed in this article are solely those of the authors and do not necessarily represent those of their affiliated organizations, or those of the publisher, the editors and the reviewers. Any product that may be evaluated in this article, or claim that may be made by its manufacturer, is not guaranteed or endorsed by the publisher.
References
Adams, R. A., Syu, J.-M., Zhao, Y., LoVarma, C.-T. A., Varma, A., and Pol, V. G. (2017). Binder-free N- and O-rich carbon nanofiber anodes for long cycle life K-ion batteries. ACS Appl. Mat. Interfaces 9 (21), 17872–17881. doi:10.1021/acsami.7b02476
An, Y., Fei, H., Zeng, G., Ci, L., Xi, B., Xiong, S., et al. (2018). Commercial expanded graphite as A low-cost, long-cycling life anode for potassium-ion batteries with conventional carbonate electrolyte. J. Power Sources 378, 66–72. doi:10.1016/j.jpowsour.2017.12.033
Atangana Etogo, C., Huang, H., Hong, H., Liu, G., and Zhang, L. (2020). Metal-organic-frameworks-engaged formation of Co0.85Se@C nanoboxes embedded in carbon nanofibers film for enhanced potassium-ion storage. Energy Storage Mater. 24, 167–176. doi:10.1016/j.ensm.2019.08.022
Bin, D.-S., Lin, X.-J., Sun, Y.-G., Xu, Y.-S., Zhang, K., Cao, A.-M., et al. (2018). Engineering hollow carbon architecture for high-performance K-ion battery anode. J. Am. Chem. Soc. 140 (23), 7127–7134. doi:10.1021/jacs.8b02178
Cao, J., Xu, H., Zhong, J., Li, X., Li, S., Wang, Y., et al. (2021). Dual-carbon electrode-based high-energy-density potassium-ion hybrid capacitor. ACS Appl. Mat. Interfaces 13 (7), 8497–8506. doi:10.1021/acsami.1c00115
Cao, J., Zhong, J., Xu, H., Li, S., Deng, H., Wang, T., et al. (2021). N/S Co-doped carbon nanosheet bundles as high-capacity anode for potassium-ion battery. Nano Res. 15 (3), 2040–2046. doi:10.1007/s12274-021-3773-5
Chen, C., Yang, Y., Tang, X., Qiu, R., Wang, S., Cao, G., et al. (2019). Graphene-encapsulated FeS2 in carbon fibers as high reversible anodes for Na+/K+ batteries in a wide temperature range. Small 15, 1804740. doi:10.1002/smll.201804740
Chen, L., Lin, X., Gao, J., Zou, M., Huang, Y., Zhao, G., et al. (2022). Porous carbon nanofibers as anode for high-performance potassium-ion batteries. Electrochimica Acta 403, 139654. doi:10.1016/j.electacta.2021.139654
Chen, M., Wang, W., Liang, X., Gong, S., Liu, J., Wang, Q., et al. (2018). Sulfur/oxygen codoped porous hard carbon microspheres for high-performance potassium-ion batteries. Adv. Energy Mat. 8 (19), 1800171. doi:10.1002/aenm.201800171
Chen, S., Qiu, L., and Cheng, H.-M. (2020). Carbon-based fibers for advanced electrochemical energy storage devices. Chem. Rev. 120 (5), 2811–2878. doi:10.1021/acs.chemrev.9b00466
Deng, H., Wang, L., Li, S., Zhang, M., Wang, T., Zhou, J., et al. (2021). Radial pores in nitrogen/oxygen dual‐doped carbon nanospheres anode boost high‐power and ultrastable potassium‐ion batteries. Adv. Funct. Mater. 31 (51), 2107246. doi:10.1002/adfm.202107246
Ge, X., Liu, S., Qiao, M., Du, Y., Li, Y., Bao, J., et al. (2019). Enabling superior electrochemical properties for highly efficient potassium storage by impregnating ultrafine Sb nanocrystals within nanochannel‐containing carbon nanofibers. Angew. Chem. Int. Ed. 58 (41), 14578–14583. doi:10.1002/anie.201908918
Geng, S., Zhou, T., Jia, M., Shen, X., Gao, P., Tian, S., et al. (2021). Carbon-coated WS2 nanosheets supported on carbon nanofibers for high-rate potassium-ion capacitors. Energy Environ. Sci. 14 (5), 3184–3193. doi:10.1039/d1ee00193k
Gu, F., Guo, J., Yao, X., Summers, P. A., Widijatmoko, S. D., and Hall, P. (2017). An investigation of the current status of recycling spent lithium-ion batteries from consumer electronics in China. J. Clean. Prod. 161, 765–780. doi:10.1016/j.jclepro.2017.05.181
Hameer, S., and van Niekerk, J. L. (2015). A review of large-scale electrical energy storage. Int. J. Energy Res. 39, 1179–1195. doi:10.1002/er.3294
Han, J., Zhu, K., Liu, P., Si, Y., Chai, Y., and Jiao, L. (2019). N-doped CoSb@C nanofibers as A self-supporting anode for high-performance K-ion and Na-ion batteries. J. Mat. Chem. A 7 (44), 25268–25273. doi:10.1039/c9ta09643d
He, Y., Xu, Y., Zhang, M., Xu, J., Chen, B., Zhang, Y., et al. (2022). Confining ultrafine SnS nanoparticles in hollow multichannel carbon nanofibers for boosting potassium storage properties. Sci. Bull. 67 (2), 151–160. doi:10.1016/j.scib.2021.09.020
Ho Na, J., Chan Kang, Y., and Park, S.-K. (2021). Electrospun MOF-based ZnSe nanocrystals confined in N-doped mesoporous carbon fibers as anode materials for potassium ion batteries with long-term cycling stability. Chem. Eng. J. 425, 131651. doi:10.1016/j.cej.2021.131651
Hong, Z., Maleki, H., Ludwig, T., Zhen, Y., Wilhelm, M., Lee, D., et al. (2021). New insights into carbon-based and MXene anodes for Na and K-ion storage: A review. J. Energy Chem. 62, 660–691. doi:10.1016/j.jechem.2021.04.031
Hu, X., Zhong, G., Li, J., Liu, Y., Yuan, J., Chen, J., et al. (2020). Hierarchical porous carbon nanofibers for compatible anode and cathode of potassium-ion hybrid capacitor. Energy Environ. Sci. 13, 2431–2440. doi:10.1039/D0EE00477D
Huang, Z., Chen, Z., Ding, S., Chen, C., and Zhang, M. (2018). Enhanced conductivity and properties of SnO2-graphene-carbon nanofibers for potassium-ion batteries by graphene modification. Mater. Lett. 219, 19–22. doi:10.1016/j.matlet.2018.02.053
Huang, Z., Ding, S., Li, P., Chen, C., and Zhang, M. (2021). Flexible Sb-Graphene-Carbon nanofibers as binder-free anodes for potassium-ion batteries with enhanced properties. Nanotechnology 32 (2), 025401. doi:10.1088/1361-6528/abbb4d
Hwang, J.-Y., Myung, S.-T., and Sun, Y.-K. (2018). Recent progress in rechargeable potassium batteries. Adv. Funct. Mat. 28, 1802938. doi:10.1002/adfm.201802938
Jin, T., Li, H., Li, Y., Jiao, L., and Chen, J. (2018). Intercalation pseudocapacitance in flexible and self-standing V2O3 porous nanofibers for high-rate and ultra-stable K ion storage. Nano Energy 50, 462–467. doi:10.1016/j.nanoen.2018.05.056
Kim, S., Kim, B.-H., and Yang, K. (2013). Electrochemical behavior of porous carbon nanofibers developed from polyacrylonitrile/ graphene solutions containing zinc chloride. Coc 17 (13), 1455–1462. doi:10.2174/1385272811317130012
Lai, C., Zhang, Z., Xu, Y., Liao, J., Xu, Z., Yi, Z., et al. (2021). A general strategy for embedding ultrasmall CoMx nanocrystals (M = S, O, Se, and Te) in hierarchical porous carbon nanofibers for high-performance potassium storage. J. Mat. Chem. A 9 (3), 1487–1494. doi:10.1039/d0ta11273a
Li, D., Ren, X., Ai, Q., Sun, Q., Zhu, L., Liu, Y., et al. (2018). Facile fabrication of nitrogen-doped porous carbon as superior anode material for potassium-ion batteries. Adv. Energy Mat. 8 (34), 1802386. doi:10.1002/aenm.201802386
Li, S., Cao, J., Wang, T., Wang, L., Deng, H., Zhang, Q., et al. (2022). Intercalation and covalent bonding strategies for constructing A stable cathode for high-energy density and long-cycling potassium-organic batteries. Chem. Eng. J. 431, 133215. doi:10.1016/j.cej.2021.133215
Li, X., Sun, N., Tian, X., Yang, T., Song, Y., Xu, B., et al. (2020). Electrospun coal liquefaction residues/polyacrylonitrile composite carbon nanofiber nonwoven fabrics as high-performance electrodes for lithium/potassium batteries. Energy fuels. 34, 2445–2451. doi:10.1021/acs.energyfuels.9b03637
Liao, Y., Chen, C., Yin, D., Cai, Y., He, R., and Zhang, M. (2019). Improved Na+/K+ storage properties of ReSe2-carbon nanofibers based on graphene modifications. Nano-Micro Lett. 11 (1), 22. doi:10.1007/s40820-019-0248-2
Liu, A., Zhang, L., Li, H., Ma, Q., Chen, Q., Hua, R., et al. (2022). Rational design of highly porous carbon nanofibers with outstanding potassium storage. J. Alloys Compd. 902, 163734. doi:10.1016/j.jallcom.2022.163734
Liu, S., Zhang, H., Zhou, M., Chen, X., Sun, Y., and Zhang, Y. (2021). V5S8 nanoparticles anchored on carbon nanofibers for fast and durable sodium and potassium ion storage. J. Electroanal. Chem. 903, 115841. doi:10.1016/j.jelechem.2021.115841
Liu, Y., Tai, Z., Zhang, J., Pang, W. K., Zhang, Q., Feng, H., et al. (2018). Boosting potassium-ion batteries by few-layered composite anodes prepared via solution-triggered one-step shear exfoliation. Nat. Commun. 9, 3645. doi:10.1038/s41467-018-05786-1
L. Kulova, T., Vladimir, N. F., Ekaterina, A. S., and Alexander, S. G. (2020). A brief review of post-lithium-ion batteries. Int. J. Electrochem. Sci. 15, 7242–7259. doi:10.20964/2020.08.22
Ma, H., Qi, X., Peng, D., Chen, Y., Wei, D., Ju, Z., et al. (2019). Novel fabrication of N/S Co‐doped hierarchically porous carbon for potassium‐ion batteries. ChemistrySelect 4 (39), 11488–11495. doi:10.1002/slct.201903244
Mao, M., Cui, C., Wu, M., Zhang, M., Gao, T., Fan, X., et al. (2018). Flexible ReS2 nanosheets/N-doped carbon nanofibers-based paper as a universal anode for alkali (Li, Na, K) ion battery. Nano Energy 45, 346–352. doi:10.1016/j.nanoen.2018.01.001
M. Gür, T. (2018). Correction: Review of electrical energy storage technologies, materials and systems: Challenges and prospects for large-scale grid storage. Energy Environ. Sci. 11, 3055. doi:10.1039/C8EE90053A
Miao, W., Zhao, X., Wang, R., Liu, Y., Li, L., Zhang, Z., et al. (2019). Carbon shell encapsulated cobalt phosphide nanoparticles embedded in carbon nanotubes supported on carbon nanofibers: A promising anode for potassium ion battery. J. Colloid Interface Sci. 556, 432–440. doi:10.1016/j.jcis.2019.08.090
Min, H., Zhang, X., Shu, H., Luo, L., Zeng, X., Liao, Y., et al. (2021). Mo2C quantum dots inlaid in nitrogen-doped carbon nanofibers as free-standing anodes with long-term stability K-ion storage. J. Alloys Compd. 888, 161498. doi:10.1016/j.jallcom.2021.161498
Niu, P., Wang, P., Xu, Y., Li, Z., Wei, L., Yao, G., et al. (2021). Tuning the electronic conductivity of porous nitrogen-doped carbon nanofibers with graphene for high-performance potassium-ion storage. Inorg. Chem. Front. 8 (15), 3926–3933. doi:10.1039/d1qi00664a
Pei, Y. R., Zhao, M., Zhou, H. Y., Yang, C. C., and Jiang, Q. (2020). Hollow N-doped carbon nanofibers provide superior potassium-storage performance. Nanoscale Adv. 2 (9), 4187–4198. doi:10.1039/d0na00585a
Shen, C., Yuan, K., Tian, T., Bai, M., Wang, J.-G., Li, X., et al. (2019). Flexible sub-micro carbon Fiber@CNTs as anodes for potassium-ion batteries. ACS Appl. Mat. Interfaces 11, 5015–5021. doi:10.1021/acsami.8b18834
Sun, H., Zhu, W., Yuan, F., Zhang, D., Li, Z., Wang, Q., et al. (2022). Hierarchical porous carbon nanofibers with enhanced capacitive behavior as A flexible self-supporting anode for boosting potassium storage. J. Power Sources 523, 231043. doi:10.1016/j.jpowsour.2022.231043
Sun, Y., Shi, X. L., Yang, Y. L., Suo, G., Zhang, L., Lu, S., et al. (2022). Biomass‐derived carbon for high‐performance batteries: From structure to properties. Adv. Funct. Mater. 32, 2201584. doi:10.1002/adfm.202201584
Tao, L., Yang, Y., Wang, H., Zheng, Y., Hao, H., Song, W., et al. (2020). Sulfur-nitrogen rich carbon as stable high capacity potassium ion battery anode: Performance and storage mechanisms. Energy Storage Mater. 27, 212–225. doi:10.1016/j.ensm.2020.02.004
Thakur, A. K., Ahmed, M. S., Park, J., Prabakaran, R., SidneySathyamurthy, S. R., Sathyamurthy, R., et al. (2022). A review on carbon nanomaterials for K‐ion battery anode: Progress and perspectives. Intl J Energy Res. 46, 4033–4070. doi:10.1002/er.7508
Tian, S., Jiang, Q., Cai, T., Wang, Y., Wang, D., Kong, D., et al. (2020). Graphitized electrospun carbon fibers with superior cyclability as A free-standing anode of potassium-ion batteries. J. Power Sources 474, 228479. doi:10.1016/j.jpowsour.2020.228479
Touja, J., Gabaudan, V., Farina, F., Cavaliere, S., Caracciolo, L., Madec, L., et al. (2020). Self-supported carbon nanofibers as negative electrodes for K-ion batteries: Performance and mechanism. Electrochimica Acta 362, 137125. doi:10.1016/j.electacta.2020.137125
Wang, J., Wang, Z., Ni, J., and Li, L. (2022). Electrospun materials for batteries moving beyond lithium-ion technologies. Electrochem. Energy Rev. 5, 211–241. doi:10.1007/s41918-021-00103-9
Wang, L., Wang, T., Peng, L., Wang, Y., Zhang, M., Zhou, J., et al. (2022). The promises, challenges and pathways to room-temperature sodium-sulfur batteries. Natl. Sci. Rev. 9. nwab050. doi:10.1093/nsr/nwab050
Wang, X., Ma, J., Wang, J., and Li, X. (2020). N-doped hollow carbon nanofibers anchored hierarchical FeP nanosheets as high-performance anode for potassium-ion batteries. J. Alloys Compd. 821, 153268. doi:10.1016/j.jallcom.2019.153268
Wang, Y., Yuan, F., Li, Z., Zhang, D., Yu, Q., and Wang, B. (2022). Heteroatom-doped carbon anode materials for potassium-ion batteries: From mechanism, synthesis to electrochemical performance. Apl. Mater. 10, 030902. doi:10.1063/5.0086874
Wu, Y., Hu, S., Xu, R., Wang, J., Peng, Z., Zhang, Q., et al. (2019). Boosting potassium-ion battery performance by encapsulating red phosphorus in free-standing nitrogen-doped porous hollow carbon nanofibers. Nano Lett. 19 (2), 1351–1358. doi:10.1021/acs.nanolett.8b04957
Wu, Y., Wu, Z., Yue, L., Zhong, B., Liang, J., Luo, Y., et al. (2021). Directionally tailoring macroporous honeycomb-like structured carbon nanofibers toward high-capacitive potassium storage. ACS Appl. Mat. Interfaces 13 (26), 30693–30702. doi:10.1021/acsami.1c07111
Xie, Y., Chen, Y., Liu, L., Tao, P., Fan, M., Xu, N., et al. (2017). Ultra-high pyridinic N-doped porous carbon monolith enabling high-capacity K-ion battery anodes for both half-cell and full-cell applications. Adv. Mat. 29 (35), 1702268. doi:10.1002/adma.201702268
Xu, L., Guo, W., Zeng, L., Xia, X., Wang, Y., Xiong, P., et al. (2021). V3Se4 embedded within N/P co-doped carbon fibers for sodium/potassium ion batteries. Chem. Eng. J. 419, 129607. doi:10.1016/j.cej.2021.129607
Xu, Y., Zhang, C., Zhou, M., Fu, Q., Zhao, C., Wu, M., et al. (2018). Highly nitrogen doped carbon nanofibers with superior rate capability and cyclability for potassium ion batteries. Nat. Commun. 9, 1720. doi:10.1038/s41467-018-04190-z
Xue, J., Wu, T., Dai, Y., and Xia, Y. (2019). Electrospinning and electrospun nanofibers: Methods, materials, and applications. Chem. Rev. 119 (8), 5298–5415. doi:10.1021/acs.chemrev.8b00593
Yang, M., Su, D., Zhang, W., Wen, J., Liu, W., Luo, Q., et al. (2021). Potassium storage mechanism of In2S3/C nanofibers as the anode for potassium ion batteries. Electrochimica Acta 400, 139461. doi:10.1016/j.electacta.2021.139461
Yang, W., Zhou, J., Wang, S., Zhang, W., Wang, Z., Lv, F., et al. (2019). Freestanding film made by necklace-like N-doped hollow carbon with hierarchical pores for high-performance potassium-ion storage. Energy Environ. Sci. 12 (5), 1605–1612. doi:10.1039/c9ee00536f
YaoLin, G. M. X., Lin, M., Yang, J., Wei, L., niu, H., Luo, Q., et al. (2022). Stabilizing V2O3 in carbon nanofiber flexible films for ultrastable potassium storage. Inorg. Chem. Front. 9 (7), 1434–1445. doi:10.1039/d1qi01611c
Yi, Z., Liu, Y., Li, Y., Zhou, L., Wang, Z., Zhang, J., et al. (2020). Flexible membrane consisting of MoP ultrafine nanoparticles highly distributed inside N and P codoped carbon nanofibers as high‐performance anode for potassium‐ion batteries. Small 16 (2), 1905301. doi:10.1002/smll.201905301
Yuan, F., Zhang, W., Zhang, D., Wang, Q., Li, Z., Li, W., et al. (2021). Recent progress in electrochemical performance of binder-free anodes for potassium-ion batteries. Nanoscale 13 (12), 5965–5984. doi:10.1039/d1nr00077b
Zhang, B., Kang, F., Tarascon, J.-M., and Kim, J.-K. (2016). Recent advances in electrospun carbon nanofibers and their application in electrochemical energy storage. Prog. Mater. Sci. 76, 319–380. doi:10.1016/j.pmatsci.2015.08.002
Zhang, M., Shoaib, M., Fei, H., Wang, T., Zhong, J., Fan, L., et al. (2019). Hierarchically porous N‐doped carbon fibers as a free‐standing anode for high‐capacity potassium‐based dual‐ion battery. Adv. Energy Mat. 9 (37), 1901663. doi:10.1002/aenm.201901663
Zhang, Q., Wang, Z., ZhangZhou, S. T., Zhou, T., Mao, J., and Guo, Z. (2018). Cathode materials for potassium-ion batteries: Current status and perspective. Electrochem. Energy Rev. 1, 625–658. doi:10.1007/s41918-018-0023-y
Zhang, S., Xu, Z., Duan, H., Xu, A., Xia, Q., Yan, Y., et al. (2020). N-doped carbon nanofibers with internal cross-linked multiple pores for both ultra-long cycling life and high capacity in highly durable K-ion battery anodes. Electrochimica Acta 337, 135767. doi:10.1016/j.electacta.2020.135767
Zhang, W., Chen, J., Liu, Y., Liu, S., Li, X., Yang, K., et al. (2020). Decoration of hollow nitrogen-doped carbon nanofibers with tapered rod-shaped NiCo2S4 as a 3D structural high-rate and long-lifespan self-supported anode material for potassium-ion batteries. J. Alloys Compd. 823, 153631. doi:10.1016/j.jallcom.2019.153631
Zhang, W., Miao, W., Liu, X., Li, L., Yu, Z., and Zhang, Q. (2018). High-rate and ultralong-stable potassium-ion batteries based on antimony-nanoparticles encapsulated in nitrogen and phosphorus co-doped mesoporous carbon nanofibers as an anode material. J. Alloys Compd. 769, 141–148. doi:10.1016/j.jallcom.2018.07.369
Zhang, W., Yin, J., Wang, W., Bayhan, Z., and Alshareef, H. N. (2021). Status of rechargeable potassium batteries. Nano Energy 83, 105792. doi:10.1016/j.nanoen.2021.105792
Zhao, X., Xiong, P., Meng, J., Liang, Y., Wang, J., and Xu, Y. (2017). High rate and long cycle life porous carbon nanofiber paper anodes for potassium-ion batteries. J. Mat. Chem. A 5, 19237–19244. doi:10.1039/C7TA04264G
Zheng, F., Chu, K., Yang, Y., Li, Z., Wei, L., Xu, Y., et al. (2022). Optimizing the interlayer spacing of heteroatom-doped carbon nanofibers toward ultrahigh potassium-storage performances. ACS Appl. Mat. Interfaces 14 (7), 9212–9221. doi:10.1021/acsami.1c24275
Zheng, J., Sun, Y., Wu, Y., Rong, J., Wang, Z., Li, H., et al. (2021). Ultralong cycle life and high rate potassium ion batteries enabled by multi-level porous carbon. J. Power Sources 492, 229614. doi:10.1016/j.jpowsour.2021.229614
Zheng, J., Wu, Y., Sun, Y., Rong, J., Li, H., and Niu, L. (2021). Advanced anode materials of potassium ion batteries: From zero dimension to three dimensions. Nano-Micro Lett. 13 (1), 12. doi:10.1007/s40820-020-00541-y
Zhong, J., Wang, T., Wang, L., Peng, L., Fu, S., Zhang, M., et al. (2022). A silicon monoxide lithium-ion battery anode with ultrahigh areal capacity. Nano-Micro Lett. 14, 50. doi:10.1007/s40820-022-00790-z
Keywords: potassium ion storage, anode electrode, electrospun carbon nanofibers, porous structure, heteroatom doping, composites
Citation: Xiao M, Li R, Yang T and Dai Y (2022) The progress and perspective of electrospun carbon nanofibers based anode materials for potassium ion storage: A mini review. Front. Energy Res. 10:966825. doi: 10.3389/fenrg.2022.966825
Received: 11 June 2022; Accepted: 27 June 2022;
Published: 12 July 2022.
Edited by:
Hongtao Sun, The Pennsylvania State University (PSU), United StatesReviewed by:
Lei Wang, Chongqing University, ChinaBo Nie, The Pennsylvania State University, United States
Copyright © 2022 Xiao, Li, Yang and Dai. This is an open-access article distributed under the terms of the Creative Commons Attribution License (CC BY). The use, distribution or reproduction in other forums is permitted, provided the original author(s) and the copyright owner(s) are credited and that the original publication in this journal is cited, in accordance with accepted academic practice. No use, distribution or reproduction is permitted which does not comply with these terms.
*Correspondence: Ting Yang, eXQtMjlAMTYzLmNvbQ==