- 1Key Laboratory of Energy Thermal Conversion and Control of Ministry of Education, School of Energy and Environment, Southeast University, Nanjing, China
- 2Jiangsu Key Laboratory of Micro and Nano Heat Fluid Flow Technology and Energy Application, School of Physical Science and Technology, Suzhfou University of Science and Technology, Suzhou, China
Introduction
70% of the earth’s surface is covered by water, but fresh water only accounts for 2.7% of total water in the world, and only 0.3% of freshwater can be directly used by humans (Zapata-Sierra et al., 2021). With the development of the world economy, population growth, and consumption of freshwater resources, the global per capita average of freshwater resources has dropped by half in the past 50 years, and the developed cities, island areas, and ships have more urgent demands for freshwater resources (Jones et al., 2019). It is predicted that three-quarters of the population in the world will suffer from freshwater shortages by 2050. In addition, the pollution of freshwater resources is more serious due to the impact of COVID-19, which has also accelerated research progress in the fields of freshwater protection and seawater desalination (Zhang et al., 2022).
Desalination technology, which produces freshwater by removing salt and other mineral components from seawater, has become one of the most important solutions for water treatment and drinking water production in the world (Lin et al., 2021). At present, there are 19,000 existing desalination plants, which can produce more than 1 × 108 m3/day of freshwater (Alrowais et al., 2022). Most desalination plants are located in countries with a shortage of freshwater resources but rich in energy, such as the United States and Gulf states. In addition, China and India have developed rapidly in the field of seawater desalination and carried out a great deal of seawater desalination research (Liu et al., 2019; Eke et al., 2020). An overview of the major desalination technologies in the world and the desalination technology based on ocean thermal energy is introduced in this paper, and the possible challenges and future development prospects of seawater desalination technology in the future application are discussed to provide a reference for the subsequent development of the seawater desalination industry (Rey and Lauro, 1981).
Development Status of Seawater Desalination Technology
Desalination technologies can be classified according to the driving energy required in the desalination process, namely thermally-driven, mechanically-driven, and electrically-driven desalination methods (Nassrullah et al., 2020). Thermally-driven desalination technologies mainly include multiple effect distillation (MED) and multi-stage flash (MSF) (Aly et al., 2021). Mechanically-driven desalination technology mainly refers to membrane processes, among which reverse osmosis (RO) has been widely used due to its lower energy consumption and flexible installed capacity (Qasim et al., 2019). Electrically driven desalination technology allows ions to pass through selectivity exchange membranes under a direct-current electric field to obtain fresh water. Due to this technology’s high consumption of direct electrical energy, it only accounts for 2% of the desalination capacity in the world (Subramani and Jacangelo, 2015). Additionally, emerging desalination technologies, such as membrane distillation (MD) combining thermal energy and membrane separation, can be driven by low-grade heat rather than electricity. They are promising although currently being investigated at laboratory.
Figure 1A shows the schematic of the MED system (Al-hotmani et al., 2020), the steam enters the first effect to heat the seawater as the heating medium. The seawater is heated to boiling temperature and then a part is evaporated. Condensation occurs once the generated vapor has flowed into the next effect, and the process repeats for all the effects. In the final condenser, the vapor produced in the last effect is condensed while the seawater is preheated (Wang et al., 2011). In the late 1970s, the technology of low-temperature multiple effect distillation (LT-MED) that utilizes low-grade steam at temperatures of 50–70°C is developed by IDE company, which reduces desalination costs, mitigates corrosion and scaling issues, resulting in the cost of producing fresh water is reduced to $0.738 per ton (Liu et al., 2021). The MSF is based on the flash evaporation principle, and the MSF process is shown in Figure 1B (Zhao et al., 2018). The seawater is heated by the steam and flows into the flash evaporator, and the hot seawater is rapidly vaporized due to the lower pressure in the flash evaporator than the saturation pressure. The generated vapor is condensed into fresh water while heating the front seawater, and the remaining seawater is sent to the next flash evaporator and vaporized at lower pressure (Khawaji et al., 2008). MSF technology is highly reliable and is easy to be scaled up, but it is often combined with thermal power plants to achieve “co-generation of fresh water and power” due to its large heat requirements, and the cost per ton of water production in this combined system is about $1.023 (Semiat, 2008).
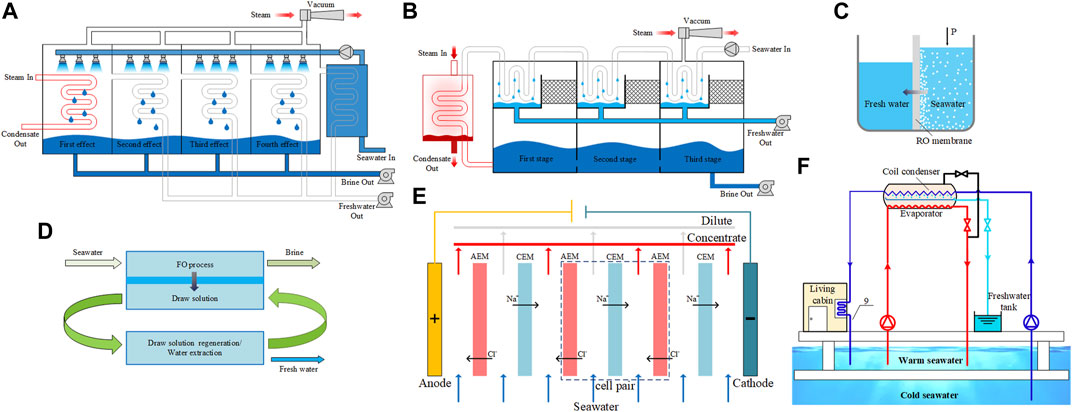
FIGURE 1. Schematic of the seawater desalination process: (A) MED, (B) MSF, (C) RO, (D) FO, (E) ED/EDR, (F) low-pressure flash evaporation system powered by ocean thermal energy.
As the most widely used seawater desalination technology in the world, RO is used in 84% of desalination plants (Ng et al., 2021). The principle of RO is as follows (Figure 1C): when the pressure applied to the seawater is greater than the osmotic pressure, the water molecules in the seawater will enter the freshwater side through the RO membrane, and the solute is isolated in the seawater side by the membrane. The research of RO technology mainly focuses on the improvement of membrane performance. The ideal RO membrane should have good mechanical properties, thermal stability, high desalination rate, low cost, and less impact on pH and temperature. RO technology has been substantially improved over the past few decades, resulting in the energy consumption per ton of fresh water produced has been significantly reduced to $0.749 (Patel et al., 2022). Nanomaterials, such as SiO2 nanoparticles (Nguyen et al., 2021), carbon nanotubes (Ghanbari et al., 2015), and metal-organic frameworks (Ji et al., 2019), are receiving increasing attention for the preparation of RO membranes based on thin-film nanocomposite (TFN). This TFN membrane has the advantages of high chemical, thermal stability, and water permeability. Forward osmosis (FO) technology is different from RO technology. In the FO process, water is permeated from seawater to the draw solution through the FO membrane because of the higher osmotic pressure than the seawater. The main problem with this technology is that the fresh water in the draw solution is difficult to separate, the principle of FO is shown in Figure 1D.
The scheme of electrodialysis and electrodialysis reversal (ED/EDR) driven by electricity is shown in Figure 1E. The ED/EDR system consists of repeated unit pairs, each unit pair includes a desalination chamber and a concentration chamber. When seawater is introduced and energized, the Na+ in the desalination chamber is migrated to the cathode through the cation exchange membrane (CEM), and the Cl− is migrated to the anode through the anion exchange membrane (AEM), thus the fresh water is generated from the seawater in the desalination chamber and the concentrated seawater is formed in the concentration chamber. The scaling of EDR is reduced by periodically switching the polarity of the electrodes. ED/EDR has the advantages such as simple pretreatment of feedwater because only the charged ions are affected by the electric potential during the desalination process, thus ED/EDR technology is suitable for treating feed water with high suspended solids and achieving high recovery rates. However, the capacity of seawater desalination plants using ED/EDR technology is generally small due to the high electricity consumption. In a study by Liu et al., the desalination rate of brackish water in ED/EDR reached 90% with electricity consumption of 1 kWh/m3 (Liu and Wang, 2017).
The traditional seawater desalination technologies have the problems such as high investment, high energy consumption, and environmental pollution, the seawater desalination technology based on renewable energy is an effective way to solve the above problems (Chen et al., 2014). As marine renewable energy, ocean thermal energy is considered to have great development potential due to its huge reserves and stable energy supply (Gao et al., 2021). The seawater desalination technology based on ocean thermal energy is suitable for low latitude regions with the most effective method of flash evaporation technology (Gao et al., 2019). In this flash evaporation system, the surface warm seawater is flash evaporated by a vacuum pump at a low temperature, and the vapor produced is exchanged with the deep cold seawater and condensed into fresh water (Jin et al., 2017). However, the non-condensable gas (NCG) dissolved in the seawater is gradually released during the flashing process, and then the condensation process is hindered by the NCG, resulting in the yield of fresh water being reduced (Zhang et al., 2017). Therefore, a part of the electric energy must be consumed to ensure the operation of the vacuum pump to extract the NCG in the desalination process. To reduce electricity consumption, natural vacuum technology can be used in the flash tank (Al-Kharabsheh and Goswami, 2003). The natural vacuum technology is based on the Torricelli phenomenon, which makes the warm seawater at 30°C vaporize at a 10.33 m-high water column under natural conditions (Figure 1F). The electricity consumption and recovery rate achieved by this technology are 0.126 kWh/kg and 1.5%, respectively (Wang et al., 2022). It can be seen that the cost of ocean thermal energy only used for seawater desalination is higher than that of traditional desalination technologies such as RO due to the difficulty in extracting deep cold seawater. Therefore, this desalination technology is usually used as a supplement to ocean thermal energy conversion (OTEC) to improve the utilization efficiency of ocean thermal energy.
Opportunities and Challenges
With the economic development and population growth, human demand for freshwater resources will continue to increase (Deng et al., 2017). This brings opportunities and challenges to the application and development of desalination technology, especially seawater desalination. This paper introduces the traditional seawater desalination technologies and the desalination technology based on ocean thermal energy with great development potential. Considering the research status and practical application requirements of seawater desalination technology, the following aspects need to be further studied in the future:
1) High cost is the worst problem for the development of the desalination industry, thus, more attention should be paid to the development of low-grade energy utilization for thermally-driven desalination processes. In addition, the performance of the membrane and the stability of membrane-based desalination processes should be improved.
2) Develop new technologies for desalination technology using renewable energy. Although renewable energy has abundant reserves and is environmentally friendly, it is generally inefficient and uneconomical. Therefore, a new desalination technology using renewable energy sources such as wind, solar, marine, and nuclear should be introduced to reduce traditional energy consumption and carbon emissions.
Author Contributions
EH contributed to conception of the study. JW wrote the first draft of the manuscript. All authors contributed to manuscript revision, read, and approved the submitted version.
Funding
This work is supported by National Natural Science Foundation of China (No. 52106023).
Conflict of Interest
The authors declare that the research was conducted in the absence of any commercial or financial relationships that could be construed as a potential conflict of interest.
Publisher’s Note
All claims expressed in this article are solely those of the authors and do not necessarily represent those of their affiliated organizations, or those of the publisher, the editors and the reviewers. Any product that may be evaluated in this article, or claim that may be made by its manufacturer, is not guaranteed or endorsed by the publisher.
References
Al-hotmani, O. M. A., Al-Obaidi, M. A., Filippini, G., Manenti, F., Patel, R., and Mujtaba, I. M. (2020). Optimisation of Multi Effect Distillation Based Desalination System for Minimum Production Cost for Freshwater via Repetitive Simulation. Comput. Chem. Eng. 135, 106710. doi:10.1016/j.compchemeng.2019.106710
Al-Kharabsheh, S., and Goswami, D. Y. (2003). Analysis of an Innovative Water Desalination System Using Low-Grade Solar Heat. Desalination 156, 323–332. doi:10.1016/S0011-9164(03)00363-1
Alrowais, R., Shahzad, M. W., Burhan, M., Bashir, M. T., Chen, Q., Xu, B. B., et al. (2022). A Thermally-Driven Seawater Desalination System: Proof of Concept and Vision for Future Sustainability. Case Stud. Therm. Eng. 35, 102084. doi:10.1016/j.csite.2022.102084
Aly, S., Manzoor, H., Simson, S., Abotaleb, A., Lawler, J., and Mabrouk, A. N. (2021). Pilot Testing of a Novel Multi Effect Distillation (MED) Technology for Seawater Desalination. Desalination 519, 115221. doi:10.1016/j.desal.2021.115221
Chen, Y., Shen, C., Shi, M., and Peterson, G. P. (2014). Visualization Study of Flow Condensation in Hydrophobic Microchannels. AICHE J. 60, 1182–1192. doi:10.1002/aic.14319
Deng, Z., Zhang, C., Shen, C., Cao, J., and Chen, Y. (2017). Self-propelled Dropwise Condensation on a Gradient Surface. Int. J. Heat Mass Transf. 114, 419–429. doi:10.1016/j.ijheatmasstransfer.2017.06.065
Eke, J., Yusuf, A., Giwa, A., and Sodiq, A. (2020). The Global Status of Desalination: An Assessment of Current Desalination Technologies, Plants and Capacity. Desalination 495, 114633. doi:10.1016/j.desal.2020.114633
Gao, W., Qi, J., Zhang, J., Chen, G., and Wu, D. (2019). An Experimental Study on Explosive Boiling of Superheated Droplets in Vacuum Spray Flash Evaporation. Int. J. Heat Mass Transf. 144, 118552. doi:10.1016/j.ijheatmasstransfer.2019.118552
Gao, W., Lei, Z., Liu, X., and Chen, Y. (2021). Dynamic Liquid Gating Artificially Spinning System for Self-Evolving Topographies and Microstructures. Langmuir 37, 1438–1445. doi:10.1021/acs.langmuir.0c02910
Ghanbari, M., Emadzadeh, D., Lau, W. J., Lai, S. O., Matsuura, T., and Ismail, A. F. (2015). Synthesis and Characterization of Novel Thin Film Nanocomposite (TFN) Membranes Embedded with Halloysite Nanotubes (HNTs) for Water Desalination. Desalination 358, 33–41. doi:10.1016/j.desal.2014.11.035
Ji, C., Xue, S., Tang, Y.-J., Ma, X.-H., and Xu, Z.-L. (2019). Polyamide Membranes with Net-like Nanostructures Induced by Different Charged MOFs for Elevated Nanofiltration. ACS Appl. Polym. Mat. 2, 585–593. doi:10.1021/acsapm.9b00973
Jin, Z.-j., Ye, H., Wang, H., Li, H., and Qian, J.-y. (2017). Thermodynamic Analysis of Siphon Flash Evaporation Desalination System Using Ocean Thermal Energy. Energy Convers. Manag. 136, 66–77. doi:10.1016/j.enconman.2017.01.002
Jones, E., Qadir, M., van Vliet, M. T. H., Smakhtin, V., and Kang, S.-m. (2019). The State of Desalination and Brine Production: A Global Outlook. Sci. Total Environ. 657, 1343–1356. doi:10.1016/j.scitotenv.2018.12.076
Khawaji, A. D., Kutubkhanah, I. K., and Wie, J.-M. (2008). Advances in Seawater Desalination Technologies. Desalination 221, 47–69. doi:10.1016/j.desal.2007.01.067
Lin, S., Zhao, H., Zhu, L., He, T., Chen, S., Gao, C., et al. (2021). Seawater Desalination Technology and Engineering in China: A Review. Desalination 498, 114728. doi:10.1016/j.desal.2020.114728
Liu, Y., and Wang, J. (2017). Energy-saving "NF/EDR" Integrated Membrane Process for Seawater Desalination. Part II. The Optimization of ED Process. Desalination 422, 142–152. doi:10.1016/j.desal.2017.07.010
Liu, S. Y., Zhang, G. X., Han, M. Y., Wu, X. D., Li, Y. L., Chen, K., et al. (2019). Freshwater Costs of Seawater Desalination: Systems Process Analysis for the Case Plant in China. J. Clean. Prod. 212, 677–686. doi:10.1016/j.jclepro.2018.12.012
Liu, S., Wang, Z., Han, M., and Zhang, J. (2021). Embodied Water Consumption between Typical Desalination Projects: Reverse Osmosis versus Low-Temperature Multi-Effect Distillation. J. Clean. Prod. 295, 126340. doi:10.1016/j.jclepro.2021.126340
Nassrullah, H., Anis, S. F., Hashaikeh, R., and Hilal, N. (2020). Energy for Desalination: A State-Of-The-Art Review. Desalination 491, 114569. doi:10.1016/j.desal.2020.114569
Ng, Z. C., Lau, W. J., Matsuura, T., and Ismail, A. F. (2021). Thin Film Nanocomposite RO Membranes: Review on Fabrication Techniques and Impacts of Nanofiller Characteristics on Membrane Properties. Chem. Eng. Res. Des. 165, 81–105. doi:10.1016/j.cherd.2020.10.003
Nguyen, T.-Q., Tung, K.-L., Lin, Y.-L., Dong, C.-D., Chen, C.-W., and Wu, C.-H. (2021). Modifying Thin-Film Composite Forward Osmosis Membranes Using Various SiO2 Nanoparticles for Aquaculture Wastewater Recovery. Chemosphere 281, 130796. doi:10.1016/j.chemosphere.2021.130796
Patel, C. G., Barad, D., and Swaminathan, J. (2022). Desalination Using Pressure or Electric Field? A Fundamental Comparison of RO and Electrodialysis. Desalination 530, 115620. doi:10.1016/j.desal.2022.115620
Qasim, M., Badrelzaman, M., Darwish, N. N., Darwish, N. A., and Hilal, N. (2019). Reverse Osmosis Desalination: A State-Of-The-Art Review. Desalination 459, 59–104. doi:10.1016/j.desal.2019.02.008
Rey, M., and Lauro, F. (1981). Ocean Thermal Energy and Desalination. Desalination 39, 159–168. doi:10.1016/S0011-9164(00)86118-4
Semiat, R. (2008). Energy Issues in Desalination Processes. Environ. Sci. Technol. 42, 8193–8201. doi:10.1021/es801330u
Subramani, A., and Jacangelo, J. G. (2015). Emerging Desalination Technologies for Water Treatment: A Critical Review. Water Res. 75, 164–187. doi:10.1016/j.watres.2015.02.032
Wang, X., Christ, A., Regenauer-Lieb, K., Hooman, K., and Chua, H. T. (2011). Low Grade Heat Driven Multi-Effect Distillation Technology. Int. J. Heat Mass Transf. 54, 5497–5503. doi:10.1016/j.ijheatmasstransfer.2011.07.041
Wang, L., Ma, X., Kong, H., Jin, R., and Zheng, H. (2022). Investigation of a Low-Pressure Flash Evaporation Desalination System Powered by Ocean Thermal Energy. Appl. Therm. Eng. 212, 118523. doi:10.1016/j.applthermaleng.2022.118523
Zapata-Sierra, A., Cascajares, M., Alcayde, A., and Manzano-Agugliaro, F. (2021). Worldwide Research Trends on Desalination. Desalination 519, 115305. doi:10.1016/j.desal.2021.115305
Zhang, C., Shen, C., and Chen, Y. (2017). Experimental Study on Flow Condensation of Mixture in a Hydrophobic Microchannel. Int. J. Heat Mass Transf. 104, 1135–1144. doi:10.1016/j.ijheatmasstransfer.2016.09.029
Zhang, X., Jiang, J., Yuan, F., Song, W., Li, J., Xing, D., et al. (2022). Estimation of Water Footprint in Seawater Desalination with Reverse Osmosis Process. Environ. Res. 204, 112374. doi:10.1016/j.envres.2021.112374
Keywords: seawater desalination, multiple effect distillation, multi-stage flash, reverse osmosis, ocean thermal energy
Citation: Wang J and Huo E (2022) Opportunities and Challenges of Seawater Desalination Technology. Front. Energy Res. 10:960537. doi: 10.3389/fenrg.2022.960537
Received: 03 June 2022; Accepted: 06 June 2022;
Published: 20 June 2022.
Edited by:
Xiangdong Liu, Yangzhou University, ChinaReviewed by:
Mengbing Cao, Zhejiang University, ChinaCopyright © 2022 Wang and Huo. This is an open-access article distributed under the terms of the Creative Commons Attribution License (CC BY). The use, distribution or reproduction in other forums is permitted, provided the original author(s) and the copyright owner(s) are credited and that the original publication in this journal is cited, in accordance with accepted academic practice. No use, distribution or reproduction is permitted which does not comply with these terms.
*Correspondence: Erguang Huo, aHVvZXJndWFuZ0AxMjYuY29t