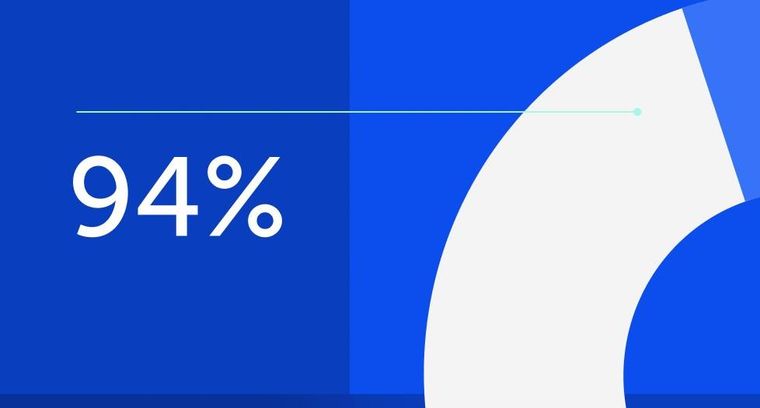
94% of researchers rate our articles as excellent or good
Learn more about the work of our research integrity team to safeguard the quality of each article we publish.
Find out more
ORIGINAL RESEARCH article
Front. Energy Res., 07 September 2022
Sec. Advanced Clean Fuel Technologies
Volume 10 - 2022 | https://doi.org/10.3389/fenrg.2022.958830
This article is part of the Research TopicThe Challenge and Opportunity of CCUS in the Development of Unconventional ResourceView all 15 articles
Production from unconventional oil reservoirs has always been a critical issue for the oil industry. In this regard, we implemented a set of experimental scenarios containing CO2/N2 cyclic injection, the foams generated by nitrogen and carbon dioxide, cyclic carbon dioxide injection, and a combined effect of carbon dioxide and surfactant enhanced oil recovery methods. As water injection has reached a plateau around two pore volume injection, we continued the tests from two pore volume injection for different scenarios. It was observed that CO2/N2-foam has the highest oil recovery factor of 34.9%; however, CO2-foam, regarding more feasibility than N2, witnessed the highest oil recovery factor after CO2/N2-foam. On the other hand, the incremental oil recovery factor for surfactant flooding, carbonated water flooding, and carbonated water with surfactant flooding is measured at 4.9, 8.5, and 10.6%, respectively.
Due to the importance of unconventional oil reservoirs to produce more oil volume and supply the various industrial demands, these reservoirs have become the most priority for oil production (Jia et al., 2012; Wang et al., 2015; Sheng, 2017). Another reason is reducing the oil production rate in conventional reservoirs, and they could not satisfy the petroleum industries’ subsections. Implementation of carbon dioxide would be of significance in unconventional reservoirs as it can reduce the crude oil viscosity during the injection of CO2 (Carbon dioxide) and increase the sweep efficiency in porous media (Holm et al., 1974; Chung et al., 1988; Manrique et al., 2010; Luo et al., 2017). Furthermore, it can provide better mobilization for oil, especially in trapped zones and low permeable layers during the CO2 injection (Tovar et al., 2014; Yu et al., 2015; Zhou et al., 2020). Another application of CO2 injection would be related to the geological storage of carbon dioxide, which enables proper climate change mitigation (Busch et al., 2008; Torres et al., 2018; Mahzari et al., 2020; Guo et al., 2021). Some researchers developed comprehensive models based on the geological and petrophysical data to determine the appropriateness of EOR (Enhance oil recovery) methods based on carbon dioxide injection. The following characteristics have been considered in the model to provide more valid results. These are minimum miscible pressure, hydraulic fracture processes, and observed data from swelling tests. It is concluded that injection of CO2 could increase the oil production rate from 43 to 58% in a single permeability-porosity model. However, this method is adequately efficient in unconventional reservoirs; it is utterly dependent on the relative permeability, which can change the simulation results significantly. Therefore, it was recommended to provide sensitivity analysis before any operational performances and field test applications to ensure the effectiveness of CO2 injection (Liu et al., 2014; Kanfaret al., 2017). Pu performed a set of experimental tests to evaluate the efficiency of CO2-huff-n-puff to improve oil recovery. It was observed that the solubility of carbon dioxide and oil swelling had been increased by the pressure increase, which corresponds to the appropriateness of this method for the oil recovery enhancement from tight oil reservoirs. Moreover, it was concluded that by increasing the number of injectivity cycles from one to four, the oil recovery factor had increased about 10%; however, the increase of oil recovery is not stable with the increase of cycle numbers, and pressure would be a significant parameter (Pu et al., 2016). When CO2 is injected into the formation, the gas-oil interfacial tension decreases, and the oil in the smaller pores begins to flow (Wei et al., 2021). Ding has performed other research activities to investigate the effect of miscibility and the bypassed oil size on the recovery and mobilization of bypassed oil (Ding et al., 2019). The EOR mechanism of foam flooding mainly includes stabilizing the displacement front, reducing the capillary forces, modifying the rock wettability, and enhancing the interfacial mass transfer (Talebian et al., 2014). When foam-enhanced CO2-EOR is implemented, foam formation is a kind of gas-liquid coexistence material; the water phase or oil phase is continuously distributed in the rock, while the gas phase is discontinuous. (Gauglitz et al., 2002; Li, et al., 2020). In tight reservoirs, CO2 and foam can improve displacement efficiency in small and large pores by implementing CO2-based surfactant-alternating-gas injection (Wei et al., 2021). The surfactant stabilizes foam and reduces the rate of foam and liquid discharge (Ahmed et al., 2017).
In this study, we aimed to provide a set of experimental investigations based on foams injection and CO2-EOR-based methods such as cyclic carbon dioxide injection and surfactant-CO2-based methods to determine the optimum oil recovery methods. The structure of the paper is as follows: in section 2, the materials and methods we used in this study are illustrated and discussed with previous literature. Next, the main experimental results were categorized and discussed explicitly. In the final section, the main findings are described in conclusion section.
Reservoir core samples were taken from the tight oil formation of Block F in the Shengli oilfield, and the exact core sample sizes with the approximate length of 5 cm and outer diameter of 2.54 cm were provided. In order to measure the permeability and porosity of tight core samples, purified nitrogen (99.9% purity) was used. Thereby, permeability has ranged from 0.1–3 mD, indicating the core samples have low permeabilities. Average porosity is recorded between 10 and 12%. The dead oil and produced hydrocarbon gas samples were collected in the field and were mixed based on the gas oil ratio of 79.3 m3/m3 in the lab to form a live oil sample. The compositional analysis results of dead oil produced hydrocarbon gas and live oil is shown in Table 1.
The viscosity and density of the crude oil were 2.14 mPa s and 0.77 g/cm3, measured at the temperature of 100°C. The analysis of formation water depicted that formation water salinity is about 6000 mg/L. Synthesized brine has been formed to present a proper match with reservoir features. Its salinity is recorded at 6,000 mg/L. Shengli Oilfield Company SINOPEC provides SLPM and SLBHJ foam agents and surfactants in this experiment.
To start the coreflooding procedure, we divided each vessel containing chemical and non-chemical agents separately, and they were connected to the system by the various control valve. It helped us to control the process via a computer and define the limitations and stopping points for each setup. Due to the reservoir temperature of 100°C, an oven is implemented to control the temperature of the core-holder system. The confining pressure in the system is controlled by using a syringe pump. Another syringe pump was used to control the injection rate of the fluids introduced into the system. The back pressure was set to 25 MPa and controlled by a back-pressure valve. To record the oil and water saturation, synthesized brine with 0.02 cm3/min was injected into the system, and then two PVs (Pore Volumes) of this prepared brine were injected to measure the oil production rate. The purpose of this scenario is to simulate the water injection performances through the system, especially in primary and secondary enhanced oil recovery methods. Then, each method was performed individually, and the recovery performances were recorded in a spreadsheet to normalize and compare them (see Figure 1).
As tight reservoirs have contained low permeable layers, the gaseous phase can mobilize more conveniently through the pores and throats regarding their lower viscosity. It can help to increase the sweep efficiency of trapped oil in those layers. Both CO2 and N2 (Nitrogen) were used for cyclic injection to reduce the oil viscosity and the interfacial tension respectively to study the oil recovery factor by increasing cycles. The primary purpose of cyclic carbon dioxide injection is to improve oil recovery by giving the core samples a soaking time called the huff cycle. Oil production on the core holder is followed by pressure depletion as a puff cycle. Previous studies review this method as huff-n-puff (Abedini and Torabi, 2014).
It can be observed in Figure 2 that the CO2 cyclic injection has achieved higher oil recoveries in each cycle. That is because compared with N2, CO2 could be more dissolved into the crude oil phase and has a more significant ability to cause oil expansion, a decrease in oil viscosity, and interfacial tension. However, by increasing the number of injection cycles, the oil recovery has been the same for CO2 and N2. It was observed that the oil recovery rose significantly in the first three cycles and increased very slightly after four cycles.
Foams are one of the practical agents in EOR processes as they can block the high permeable layers and provide higher sweep and displacement efficiency for fluid flow in the layers with lower permeability. Thereby, we considered foam qualities of 40–80% to measure the oil production in the system. First, we determined the best foam quality as a factor of permeability increase in the system, and then the oil recovery factor was calculated as seen in Figure 3, the increase of permeability in the presence of foam quality increases. It was observed that the increase of foam quality has increased the resistivity factor. Thereby, 80% of foam quality was selected for foams injection.
After defining the optimum foam quality, the generated foam was injected through the system to calculate the oil recovery factor. The pure CO2, N2, and the mixture of CO2 and N2 (the ratio of CO2/N2 is 80:20) were used to generate foams during the core flooding experiments in order to investigate how the foams generated by different gases (CO2, N2, and CO2/N2) would affect the oil recovery factor in tight oil reservoirs. All the experiments were performed after the primary water injection into the system, where there was no significant change in the oil recovery factor. The maximum oil recovery factors at the two-pore volumes of water injection are 24.45, 24.7, and 24.9% for pure CO2, N2, and mixed CO2/N2 foam injection, respectively. As shown in Figure 4, generated foams have been performed after water injection. At the beginning of foams injection (before 0.3 PVs foam was injected), the oil recovery factor increased slightly for all three cases. Then, the oil in the low permeable layers has been mobilized more quickly, during 0.3–0.7 pore volumes foams injection. This point is the starting point for the oil recovery increase by foam.
It was observed that CO2/N2-foam has the highest oil recovery factor of 34.9% and CO2-foam would be feasibility than N2-foam. CO2–foam increased the oil recovery factor of 32.56 and 28.87% for N2-foam. However, when N2 replaced 20% of CO2, the foam generated by mixed CO2/N2 achieved the largest oil recovery factor of 34.95%. The experimental results represent that adding N2 into the pure CO2 system can increase the strength of the generated foam, which is consistent with the previous results by Hassan et al. and Abdelaal et al. (Hassan and Gajbhiye, 2018; Abdelaal et al., 2020).
One of the purposes of adding surfactants to carbonated water is the wettability alteration and reduction of interfacial tension to increase the mobility of the oil phase through pores and throats. Wettability alteration is one of the most essential issues in unconventional reservoirs such as tight oil and shale oil reservoirs. This part includes surfactant-based EOR methods such as surfactant (SLBHJ) injection, carbonate water injection, and carbonated water-surfactant injection (the surfactant added into the carbonated water injection). Then, the experiments should be performed for three core samples that have similar permeability (0.3–0.4 mD) to determine the best injectivity scenario. As shown in Figure 5, the carbonated water-surfactant injection has the maximum total oil recovery of 36.57%. The incremental oil recovery was measured at 10.66%. Carbonated water flooding and surfactant flooding are the next steps of the oil recovery factor, respectively. There were 34.81 and 32.1%, respectively (more details can be seen in Table 2).
Regarding the importance of the gaseous phase to increase the mobility ratio in tight oil reservoirs, we compare a set of experiments based on carbon dioxide-EOR methods to determine the efficiency of each injectivity scenario on the oil recovery factor. The most notable features in this study are;
- It was observed that CO2/N2-foam has the maximum oil recovery factor of 34.9%; however, CO2-foam, regarding more feasibility than N2, has witnessed as the highest oil recovery factor after CO2/N2-foam.
- Introducing N2 into pure CO2 strengthens the generated foams’ stability and achieves higher oil recovery.
- Carbonated water with surfactant flooding has the highest oil recovery factor of 36.5%. Carbonated water flooding can be combined with surfactant flooding to enhance oil recovery further.
- The incremental oil recovery factor for surfactant flooding, carbonated water flooding, and carbonated water with surfactant flooding is measured at 4.9, 8.5, and 10.6%, respectively.
The raw data supporting the conclusions of this article will be made available by the authors, without undue reservation.
CZ: performed the experiment and wrote the draft; GW: assisst with performing the experiment and results analysis; HH: analyze the experimental results; HZ: review the draft.
Author CZ was employed by the company Exploration and Development Research Institute SINOPEC Shengli Oilfield Company. Author GW was employed by the company PetroChina Dagang Oilfield Company. Author HH was employed by the company 11th Oil Production Plant of Changqing Oilfield Company. Author HZ was employed by the company PetroChina Xinjiang Oilfield Company.
All claims expressed in this article are solely those of the authors and do not necessarily represent those of their affiliated organizations, or those of the publisher, the editors and the reviewers. Any product that may be evaluated in this article, or claim that may be made by its manufacturer, is not guaranteed or endorsed by the publisher.
CO2, Carbon dioxide; N2, Nitrogen; EOR, Enhanced oil recovery; PV, Pore Volume; SLPM, the foaming agent provided by Shengli Oilfield Company SINOPEC; SLBHJ, the surfactant provided by Shengli Oilfield Company SINOPEC.
Abdelaal, A., Gajbhiye, R., and Al-shehri, D. (2020). Mixed CO2/N2 foam for EOR as a novel solution for supercritical CO2 foam challenges in sandstone reservoirs. ACS Omega 5 (51), 33140–33150. doi:10.1021/acsomega.0c04801
Abedini, A., and Torabi, F. (2014). Oil recovery performance of immiscible and miscible CO2 huff-and-puff processes. Energy fuels. 28 (2), 774–784. doi:10.1021/ef401363b
Ahmed, S., Elraies, K. A., Tan, I. M., and Hashmet, M. R. (2017). Experimental investigation of associative polymer performance for CO2 foam enhanced oil recovery. J. Petroleum Sci. Eng. 157, 971–979. doi:10.1016/j.petrol.2017.08.018
Busch, A., Alles, S., Gensterblum, Y., Prinz, D., Dewhurst, D. N., Raven, M. D., et al. (2008). Carbon dioxide storage potential of shales. Int. J. Greenh. Gas Control 2 (3), 297–308. doi:10.1016/j.ijggc.2008.03.003
Chung, F. T. H., Johnes, R. A., and Nguyen, H. T. (1988). Measurement and physical properties of CO2/heavy-crude-oil mixtures. SPE Reserv. Eval. Eng. 3 (3), 822–828.
Ding, M., Wang, Y., Wang, Y., Gao, M., Liu, D., and Chen, W. (2019). Experimental investigation of bypassed-oil recovery via CO2 soaking plateau around two pore volume injection, we continued the tests from two pore volume injection for differenand huff and puff injection: Effects of miscibility and bypassed-oil size. Fuel 248, 152–160. doi:10.1016/j.fuel.2019.03.088
Gauglitz, P. A., Friedmann, F., Kam, S. I., and R. Rossen, W. (2002). Foam generation in homogeneous porous media. Chem. Eng. Sci. 57 (19), 4037–4052. doi:10.1016/s0009-2509(02)00340-8
Guo, Q., Ahmadi, M. H., Lahafdoozian, M., Palyanitsina, A., Kuzichkin, O. R., Alizadeh, S. M., et al. (2021). A laboratory approach on the improvement of oil recovery and carbon dioxide storage capacity improvement by cyclic carbon dioxide injection. Energy Rep. 7, 1571–1580. doi:10.1016/j.egyr.2021.03.012
Hassan, M., and Gajbhiye, R. (2018). “Performance of CO2/N2 foam in enhanced oil recovery,” in Paper presented at the SPE Trinidad and Tobago Section Energy Resources Conference, Port of Spain, Trinidad and Tobago, June 2018.
Holm, L. W., and Josendal, V. A. (1974). Mechanisms of oil displacement by carbon dioxide. J. Pet. Technol. 26 (12), 1427–1438. doi:10.2118/4736-PA
Jia, C., Zheng, M., and Zhang, Y. (2012). Unconventional hydrocarbon resources in China and the prospect of exploration and development. Petroleum Explor. Dev. 39 (2), 139–146. doi:10.1016/s1876-3804(12)60026-3
Kanfar, M. S., Ghaderi, S. M., Clarkson, C. R., Reynolds, M. M., and Hetherington, C. (2017). “A modeling study of EOR potential for CO2 huff-n-puff in tight oil reservoirs - example from the bakken formation,” in Paper presented at the SPE Unconventional Resources Conference, Calgary, Alberta, Canada, February 2017.
Li, S., Wang, Q., and Li, Z. (2020). Stability and flow properties of oil-based foam generated by CO2. SPE J. 25, 416–431. doi:10.2118/199339-pa
Liu, G., Sorensen, J. A., Braunberger, J. R., Klenner, R., Ge, J., Gorecki, C. D., et al. (2014). “CO2-base enhanced oil recovery from unconventional reservoirs: A case study of the bakken formation,” in Paper presented at the SPE Unconventional Resources Conference, The Woodlands, Texas, USA, April 2014.
Luo, P., Luo, W., and Li, S. (2017). Effectiveness of miscible and immiscible gas flooding in recovering tight oil from Bakken reservoirs in Saskatchewan, Canada. Fuel (Lond). 208, 626–636. doi:10.1016/j.fuel.2017.07.044
Mahzari, P., Jones, A. P., and Oelkers, E. H. (2020). Impact of in-situ gas liberation for enhanced oil recovery and CO2 storage in liquid-rich shale reservoirs. Energy Sources, Part A Recovery, Util. Environ. Eff. 1, 1–21. doi:10.1080/15567036.2020.1815907
Manrique, E. J., Thomas, C. P., Ravikiran, R., Kamouei, M. I., Lantz, M., Romero, J. L., et al. (2010). “Eor: Current status and opportunities,” in Paper presented at the SPE Improved Oil Recovery Symposium, Tulsa, Oklahoma, USA, April 2010.
Pu, W., Wei, B., Jin, F., Li, Y., Jia, H., Liu, P., et al. (2016). Experimental investigation of CO2 huff-n-puff process for enhancing oil recovery in tight reservoirs. Chem. Eng. Res. Des. 111, 269–276. doi:10.1016/j.cherd.2016.05.012
Sheng, J. J. (2017). Critical review of field EOR projects in shale and tight reservoirs. J. Pet. Sci. Eng. 159, 654–665. doi:10.1016/j.petrol.2017.09.022
Talebian, S. H., Masoudi, R., Tan, I. M., and Zitha, P. L. J. (2014). Foam assisted CO2-EOR: A review of concept, challenges, and future prospects. J. Petroleum Sci. Eng. 120, 202–215. doi:10.1016/j.petrol.2014.05.013
Torres, J. A., Lu, J., Bosshart, N. W., Pekot, L. J., Sorensen, J. A., Peterson, K., et al. (2018). “Multiscale modeling to evaluate the mechanisms controlling CO2-based enhanced oil recovery and CO2 storage in the bakken formation,” in Paper presented at the SPE/AAPG/SEG Unconventional Resources Technology Conference, Houston, Texas, USA, July 2018.
Tovar, F. D., Eide, O., Graue, A., and Schechter, D. S. (2014). “Experimental investigation of enhanced recovery in unconventional liquid reservoirs using CO2: A look ahead to the future of unconventional EOR,” in Paper presented at the SPE Unconventional Resources Conference, The Woodlands, Texas, USA, April 2014.
Wang, J., Feng, L., Steve, M., Tang, X., Gail, T. E., and Mikael, H. (2015). China’s unconventional oil: A review of its resources and outlook for long-term production. Energy 82, 31–42. doi:10.1016/j.energy.2014.12.042
Wei, J., Zhou, X., Zhou, J., Li, J., and Wang, A. (2021). Experimental and simulation investigations of carbon storage associated with CO2 EOR in low-permeability reservoir. Int. J. Greenh. Gas Control 104, 103203. doi:10.1016/j.ijggc.2020.103203
Yu, W., Lashgari, H., Wu, K., and Sepehrnoori, K. (2015). CO2 injection for enhanced oil recovery in Bakken tight oil reservoirs. Fuel 159, 354–363. doi:10.1016/j.fuel.2015.06.092
Keywords: enhanced oil recovery, carbon dioxide, cyclic injection, generated foam, carbonated water with surfactant, tight oil reservoirs
Citation: Zhang C, Wu G, Huang H and Zhan H (2022) Improvement of oil recovery factor in tight reservoirs: A laboratory approach based on carbon dioxide enhanced oil recovery methods. Front. Energy Res. 10:958830. doi: 10.3389/fenrg.2022.958830
Received: 01 June 2022; Accepted: 25 July 2022;
Published: 07 September 2022.
Edited by:
Yibo Li, Southwest Petroleum University, ChinaReviewed by:
Kang Zhou, Shandong University of Science and Technology, ChinaCopyright © 2022 Zhang, Wu, Huang and Zhan. This is an open-access article distributed under the terms of the Creative Commons Attribution License (CC BY). The use, distribution or reproduction in other forums is permitted, provided the original author(s) and the copyright owner(s) are credited and that the original publication in this journal is cited, in accordance with accepted academic practice. No use, distribution or reproduction is permitted which does not comply with these terms.
*Correspondence: Chuanbao Zhang, emhhbmdjaHVhbmJhbzMzM0AxMjYuY29t
Disclaimer: All claims expressed in this article are solely those of the authors and do not necessarily represent those of their affiliated organizations, or those of the publisher, the editors and the reviewers. Any product that may be evaluated in this article or claim that may be made by its manufacturer is not guaranteed or endorsed by the publisher.
Research integrity at Frontiers
Learn more about the work of our research integrity team to safeguard the quality of each article we publish.