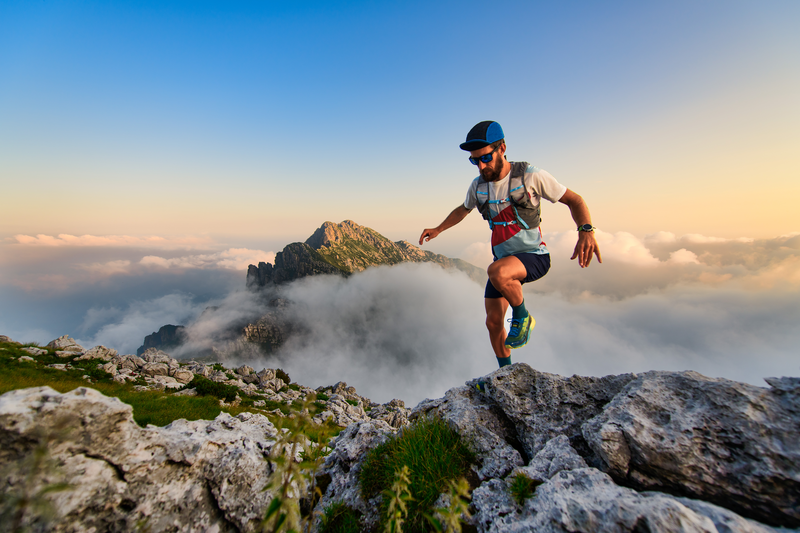
95% of researchers rate our articles as excellent or good
Learn more about the work of our research integrity team to safeguard the quality of each article we publish.
Find out more
OPINION article
Front. Energy Res. , 14 June 2022
Sec. Process and Energy Systems Engineering
Volume 10 - 2022 | https://doi.org/10.3389/fenrg.2022.939932
This article is part of the Research Topic Heat Transfer in Data Centers, Volume II View all 14 articles
Condensation is a ubiquitous gas–liquid phase change process and is widely applied in industrial fields, including chemical industry, heat transfer and exchange (Zhang et al., 2017), water collection, seawater desalination, thermal management (Liu et al., 2013), etc. There are fundamentally two modes of condensation, that is, filmwise and dropwise, whereas the dropwise condensation is supposed to be superior as it can provide greater nucleation density, faster droplet departure, and efficient surface renewal capability induced by faster droplet shedding. With the rising energy crisis across the globe, conceiving the dropwise condensation is highly necessary, which is deemed to be conducive in controlling and improving the energy utilization efficiency particularly in applications of heat transfer. Regarding this, advanced surface science has played a great role, leading to bioinspired functional surfaces (i.e., prepared by mimicking the surface structures, morphology, and chemistry of the lotus leaf, pitcher plant, desert beetle, and bamboo leaf) that have served as the competitive alternatives compared with the pristine surfaces (e.g., copper, aluminum, steel, and glass). In brief, various bioinspired functional surfaces have been recently proposed and investigated to realize the dropwise condensation, such as superhydrophobic surfaces that can promote droplet jumping; slippery liquid-infused porous surfaces (SLIPSs) that have self-healing ability and can lessen the adhesion force between the liquid and surfaces; wettability gradient surfaces that can promote droplet self-propulsion even on the horizontally positioned substrates and bi-philic surfaces that combine the advantages of both hydrophilic and hydrophobic characteristics. This review summarizes the fundamental factors affecting condensation, and provides the recent progress on bioinspired functional surfaces for dropwise condensation heat transfer enhancement.
Wettability is a significant factor influencing the condensation characteristics to the greatest extent, based on which the whole life cycle of the droplets, including nucleation, growth, and shedding, is decided. Wettability is further affected by the types of surfaces involved (flat or rough) and four surface regimes, encompassing the superhydrophobic, hydrophobic, hydrophilic, and superhydrophilic. According to the classical nucleation theory, nucleation prefers to take place on hydrophilic regions compared with hydrophobic regions because of the higher energy barrier on the hydrophobic regions (Varanasi et al., 2009). While droplets on hydrophobic regions can slide faster because of the less adhesion force than those on hydrophilic regions. Thus, wettability of pristine surfaces needs to be modified by adopting the bioinspired technology, ensuring that the droplet dynamics is efficient which is necessarily required even during dropwise condensation (Gulfam et al., 2022). Briefly, controlling the wetting behaviors of the droplets through rationally designed surfaces that can promote the droplet nucleation and detachment help enhance the dropwise condensation heat transfer.
Non-condensable gases (existing between the surface and vapors) are one of the main external factors influencing the condensation heat transfer, increasing the diffusion resistance of steam, and forming the additional thermal resistance at the interface. The quantitative study has demonstrated that even 1% of non-condensable gases can result in approximately 50% reduction in dropwise condensation heat transfer (Ma et al., 2008). Increasing the velocity (Ji et al., 2020), turbulence (Eimann et al., 2018) of the vapor, and promoting the droplet jumping (Zhang et al., 2020) can weaken the adverse effect of non-condensable gases. Besides, several studies have shown that superhydrophobic surfaces with hierarchical structures have better condensation heat transfer than hydrophobic surfaces in the presence of a tiny amount of non-condensable gases. This is due to the more stable Cassie state of droplets formed by the gases trapped in the cavities of micro-nano structures of superhydrophobic surfaces (Ma et al., 2012; Hu et al., 2015).
Surface subcooling provides the necessary driving force for condensation, which is defined as the temperature difference between the steam and surface. The droplet nucleation rate improves with the increasing subcooling degree, resulting in higher condensation heat flux. However, at higher subcooling degrees particularly for bioinspired functional surfaces, the heat transfer efficiency is underperformed due to overflow of condensate (Gulfam et al., 2022), for example, a decline in the condensation heat transfer coefficient has been experimentally reported (Lee et al., 2013). Therefore, it is a challenge to explore further ways aiming to improve dropwise condensation heat transfer under high subcooling degree for bioinspired functional surfaces (Wen et al., 2018).
Superhydrophobic slippery surfaces (e.g., lotus leaf) have the largest contact angle and lower sliding angle compared with other surfaces, provided that the droplets maintain a more stable Cassie state. During condensation, droplet jumping triggered by direct shedding or coalescence-induced shedding is an exclusive phenomenon on the superhydrophobic surfaces, promoting the efficient droplet removal and accelerating the refreshment of the surfaces (Donati et al., 2020). Boreyko et al. (Boreyko and Chen, 2009) first observed the droplet jumping caused by coalescence on the superhydrophobic surfaces with hierarchical structures. They demonstrated that this phenomenon was induced by the discharge of surplus surface energy during the coalescence process. Miljkovic et al. (2013b) experimentally proved that droplet jumping could advance the heat transfer coefficient by 30%. In addition, the return of the jumping droplets driven by gravity also influences the dropwise condensation heat transfer. In that case, Miljkovic et al. (2013a) further strengthened the condensation heat transfer performance by 50% enhancement by applying the electric field, with which the droplets could jump in a specific direction and suppress the return of the jumping droplets. Nevertheless, the superhydrophobic surfaces are not durable for longer condensation operations due to the emergence of the Wenzel state, resulting in the enhanced adhesion force, greater droplet pinning, and flooding on the surface.
Slippery liquid-infused porous surfaces (SLIPSs) are composed of the porous substrate and injected lubricant that is immiscible with the condensate (e.g., pitcher plant) (Wang et al., 2017; Wang et al., 2018). The porous substrate functions as the carrier of lubricant with low surface energy (Gulfam et al., 2020). SLIPSs have self-healing ability because the lubricant can spread evenly on the substrate driven by capillary flow (Gao et al., 2020). Different from the superhydrophobic surfaces, the lubricant infused in SLIPSs replaces the air entrapped in the micro-nano structures. In general, SLIPSs have lower adhesion and smaller contact angle hysteresis (Dai et al., 2018), which can prevent the droplet pinning and accelerate the faster removal of the droplets. Park et al. (2016) proposed asymmetric-bump–like SLIPSs, reporting the enhanced condensation heat transfer. Xiao et al. (2013) proved that SLIPSs can heighten the condensation heat transfer by 100% compared with pristine surfaces. Furthermore, the rational selection of the lubricant can implement the condensation heat transfer enhancement of the low surface tension liquids. For instance, ethanol and hexane can realize the firm dropwise condensation on SLIPSs (Sett et al., 2019), achieving 100% enhancement in heat transfer. However, loss of the lubricant is the major challenge for the application of SLIPSs that degrade their performance (Gulfam et al., 2022). Therefore, improving the operational and shelf-life durability of SLIPSs deserves further investigations, in which the lubricant loss via evaporation, physical shearing, and cloaking need to be avoided.
The bioinspired functional surfaces consisting of different wetting regimes, spanning between superhydrophobic, hydrophobic, hydrophilic, and superhydrophilic regimes, where the contact angle is changed as the spatial derivative (e.g., desert beetle), are called wettability gradient surfaces (WGS). They are widely known to provide directional self-propulsion of droplets, which, however, strongly depends on the sticky or slippery dynamics. For example, the directional self-driven motion of droplets from a hydrophobic regime to a hydrophilic regime has been achieved on WGS (Liu et al., 2017; Sommers et al., 2020), which has the great potential to further expedite droplet removal and enhance the condensation heat transfer (Tokunaga and Tsuruta, 2020; Deng et al., 2022) The mobility of droplets on the surface with radial wettability gradient was investigated experimentally in the pure steam (Daniel et al., 2001), where the droplets demonstrated coalescence with other droplets during the motion from a hydrophobic regime to a hydrophilic regime, resulting in the increased droplet velocity. Consequently, the surface promoted the droplet removal and heightened the dropwise condensation. Macner et al. (2014) further studied the droplet size distribution on a radial gradient surface during condensation, and results revealed that droplets would grow through direct condensation as well as via coalescence and then detach from the surface spontaneously, making room for new nucleation sites. Therefore, the gradient surface was occupied by small droplets, which guaranteed sufficient heat exchange area instead of developing into the liquid film and increasing the thermal resistance. However, more efforts are still indispensable to resolve the challenges encountered by WGS, including surface fabrication complexities, increasing the droplet velocity, achieving the longer droplet transport range, avoiding the droplet spreading, and pinning in the hydrophilic/superhydrophilic regimes, amongst others.
The bioinspired functional surfaces combining the bonus of both hydrophilic and hydrophobic regimes are called bi-philic surfaces (e.g., bamboo leaf). Indeed, droplets can shed swiftly on the slippery hydrophobic surface; however, the high-energy barrier of the hydrophobic regime is still a challenge, rendering the droplet nucleation more difficult (Shang et al., 2018). To overcome such deficiencies, various bi-philic surfaces have been investigated (Wilke et al., 2020), which can simultaneously enhance nucleation and remove the droplets. The design of bi-philic surfaces can include various structures, that is, stripes, grooves, patches, and fins. Peng et al. (2015) carried out an experimental study in pure steam, mainly exploring the influence of the size of hydrophilic stripes on condensation heat transfer. Results revealed that with the optimal size of hydrophilic stripe, heat flux of the hybrid bi-philic surface was 23% higher than that of the entire hydrophobic surface. Besides, hydrophilic groove structure can help reduce thickness of the condensate and transport it efficiently in the groove by surface tension (Peng et al., 2020; Chen et al., 2021), reducing the thermal resistance and improving the heat transfer performance (Ji et al., 2020). A 3D hybrid surface with a superhydrophobic ridge and hydrophilic microgrooves was prepared by Lo et al. (2019). The droplets on the superhydrophobic ridge moved spontaneously into the hydrophilic grooves, and the liquid bridges shed through the grooves, which increased the droplet detachment frequency at high subcooling and resulted in higher condensation hear transfer. Also, theoretical models have been recently presented (Xie et al., 2020), addressing the condensation heat transfer on the bi-philic surfaces. In addition to the aforementioned works, further efforts are recommended to establish theoretical models on the microgroove-based bi-philic surfaces.
Bioinspired functional surfaces have great potential to improve the condensation heat transfer by switching the filmwise mode into dropwise mode. The main factors affecting dropwise condensation were discussed. During dropwise condensation, the droplet nucleation, growth, and shedding are highly influenced by the surface wettability and droplet dynamics, which should indeed ensure slippery characteristics for efficient heat transfer. Non-condensable gases should be avoided in order to achieve faster condensation process. Subcooling degree should be controlled depending on the application demands because bioinspired functional surfaces normally lead to the best results at low subcooling as reported until now. Bioinspired functional surfaces, including superhydrophobic surfaces, slippery liquid-infused porous surfaces, wettability gradient surfaces, and bi-philic surfaces, need further efforts addressing the challenges such as durability enhancement, reducing the fabrication complexities, and subjecting them abundantly in condensation heat transfer applications at industrial scales.
ZX and RG developed the concept of the study. All authors revised the manuscript and read and approved the submitted version.
The authors declare that the research was conducted in the absence of any commercial or financial relationships that could be construed as a potential conflict of interest.
All claims expressed in this article are solely those of the authors and do not necessarily represent those of their affiliated organizations, or those of the publisher, the editors, and the reviewers. Any product that may be evaluated in this article, or claim that may be made by its manufacturer, is not guaranteed or endorsed by the publisher.
Boreyko, J. B., and Chen, C.-H. (2009). Self-Propelled Dropwise Condensate on Superhydrophobic Surfaces. Phys. Rev. Lett. 103 (18), 184501. doi:10.1103/PhysRevLett.103.184501
Chen, S., Wang, R., Wu, F., Zhang, H., Gao, X., and Jiang, L. (2021). Copper-based High-Efficiency Condensation Heat Transfer Interface Consisting of Superhydrophobic Hierarchical Microgroove and Nanocone Structure. Mater. Today Phys. 19, 100407. doi:10.1016/j.mtphys.2021.100407
Dai, X., Sun, N., Nielsen, S. O., Stogin, B. B., Wang, J., Yang, S., et al. (2018). Hydrophilic Directional Slippery Rough Surfaces for Water Harvesting. Sci. Adv. 4 (3), 0919. doi:10.1126/sciadv.aaq0919
Daniel, S., Chaudhury, M. K., and Chen, J. C. (2001). Fast Drop Movements Resulting from the Phase Change on a Gradient Surface. Science 291 (5504), 633–636. doi:10.1126/science.291.5504.633
Deng, Z., Gao, S., Wang, H., Liu, X., and Zhang, C. (2022). Visualization Study on the Condensation Heat Transfer on Vertical Surfaces with a Wettability Gradient. Int. J. Heat Mass Transf. 184, 122331. doi:10.1016/j.ijheatmasstransfer.2021.122331
Donati, M., Lam, C. W. E., Milionis, A., Sharma, C. S., Tripathy, A., Zendeli, A., et al. (2020). Sprayable Thin and Robust Carbon Nanofiber Composite Coating for Extreme Jumping Dropwise Condensation Performance. Adv. Mat. Interfaces 8 (1), 2001176. doi:10.1002/admi.202001176
Eimann, F., Zheng, S., Philipp, C., Fieback, T., and Gross, U. (2018). Convective Dropwise Condensation Out of Humid Air inside a Horizontal Channel - Experimental Investigation of the Condensate Heat Transfer Resistance. Int. J. Heat Mass Transf. 127, 448–464. doi:10.1016/j.ijheatmasstransfer.2018.08.015
Gao, W., Wang, J., Zhang, X., Sun, L., Chen, Y., and Zhao, Y. (2020). Electric-tunable Wettability on a Paraffin-Infused Slippery Pattern Surface. Chem. Eng. J. 381, 122612. doi:10.1016/j.cej.2019.122612
Gulfam, R., Huang, T.-e., Lv, C., Orejon, D., and Zhang, P. (2022). Condensation Heat Transfer on Phase Change Slippery Liquid-Infused Porous Surfaces. Int. J. Heat Mass Transf. 185, 122384. doi:10.1016/j.ijheatmasstransfer.2021.122384
Gulfam, R., Orejon, D., Choi, C.-H., and Zhang, P. (2020). Phase-Change Slippery Liquid-Infused Porous Surfaces with Thermo-Responsive Wetting and Shedding States. ACS Appl. Mat. Interfaces 12 (30), 34306–34316. doi:10.1021/acsami.0c06441
Hu, H. W., Tang, G. H., and Niu, D. (2015). Experimental Investigation of Condensation Heat Transfer on Hybrid Wettability Finned Tube with Large Amount of Noncondensable Gas. Int. J. Heat Mass Transf. 85, 513–523. doi:10.1016/j.ijheatmasstransfer.2015.02.006
Ji, D.-Y., Lee, J.-W., Kim, D., Hwang, W., and Lee, K.-Y. (2020). Effective Reduction of Non-condensable Gas Effects on Condensation Heat Transfer: Surface Modification and Steam Jet Injection. Appl. Therm. Eng. 174, 115264. doi:10.1016/j.applthermaleng.2020.115264
Lee, S., Cheng, K., Palmre, V., Bhuiya, M. M. H., Kim, K. J., Zhang, B. J., et al. (2013). Heat Transfer Measurement during Dropwise Condensation Using Micro/nano-Scale Porous Surface. Int. J. Heat Mass Transf. 65, 619–626. doi:10.1016/j.ijheatmasstransfer.2013.06.016
Liu, C., Sun, J., Li, J., Xiang, C., Che, L., Wang, Z., et al. (2017). Long-range Spontaneous Droplet Self-Propulsion on Wettability Gradient Surfaces. Sci. Rep. 7 (1), 7552. doi:10.1038/s41598-017-07867-5
Liu, X., Chen, Y., and Shi, M. (2013). Dynamic Performance Analysis on Start-Up of Closed-Loop Pulsating Heat Pipes (CLPHPs). Int. J. Therm. Sci. 65, 224–233. doi:10.1016/j.ijthermalsci.2012.10.012
Lo, C.-W., Chu, Y.-C., Yen, M.-H., and Lu, M.-C. (2019). Enhancing Condensation Heat Transfer on Three-Dimensional Hybrid Surfaces. Joule 3 (11), 2806–2823. doi:10.1016/j.joule.2019.08.005
Ma, X.-H., Zhou, X.-D., Lan, Z., Li, Y.-M., and Zhang, Y. (2008). Condensation Heat Transfer Enhancement in the Presence of Non-condensable Gas Using the Interfacial Effect of Dropwise Condensation. Int. J. Heat Mass Transf. 51 (7-8), 1728–1737. doi:10.1016/j.ijheatmasstransfer.2007.07.021
Ma, X., Wang, S., Lan, Z., Peng, B., Ma, H. B., and Cheng, P. (2012). Wetting Mode Evolution of Steam Dropwise Condensation on Superhydrophobic Surface in the Presence of Noncondensable Gas. J. Heat Transfer-Transactions Asme 134 (2), 021501. doi:10.1115/1.4005094
Macner, A. M., Daniel, S., and Steen, P. H. (2014). Condensation on Surface Energy Gradient Shifts Drop Size Distribution toward Small Drops. Langmuir 30 (7), 1788–1798. doi:10.1021/la404057g
Miljkovic, N., Enright, R., Nam, Y., Lopez, K., Dou, N., Sack, J., et al. (2013a). Jumping-droplet-enhanced Condensation on Scalable Superhydrophobic Nanostructured Surfaces. Nano Lett. 13 (1), 179–187. doi:10.1021/nl303835d
Miljkovic, N., Preston, D. J., Enright, R., and Wang, E. N. (2013b). Electric-field-enhanced Condensation on Superhydrophobic Nanostructured Surfaces. ACS Nano 7 (12), 11043–11054. doi:10.1021/nn404707j
Park, K.-C., Kim, P., Grinthal, A., He, N., Fox, D., Weaver, J. C., et al. (2016). Condensation on Slippery Asymmetric Bumps. Nature 531 (7592), 78–82. doi:10.1038/nature16956
Peng, B., Ma, X., Lan, Z., Xu, W., and Wen, R. (2015). Experimental Investigation on Steam Condensation Heat Transfer Enhancement with Vertically Patterned Hydrophobic-Hydrophilic Hybrid Surfaces. Int. J. Heat Mass Transf. 83, 27–38. doi:10.1016/j.ijheatmasstransfer.2014.11.069
Peng, Q., Jia, L., Ding, Y., Dang, C., Yin, L., and Yan, X. (2020). Influence of Groove Orientation on Dropwise Condensation on Hydrophobic and Hierarchical Superhydrophobic Surfaces with Microgroove Arrays. Int. Commun. Heat Mass Transf. 112, 104492. doi:10.1016/j.icheatmasstransfer.2020.104492
Sett, S., Sokalski, P., Boyina, K., Li, L., Rabbi, K. F., Auby, H., et al. (2019). Stable Dropwise Condensation of Ethanol and Hexane on Rationally Designed Ultrascalable Nanostructured Lubricant-Infused Surfaces. Nano Lett. 19 (8), 5287–5296. doi:10.1021/acs.nanolett.9b01754
Shang, Y., Hou, Y., Yu, M., and Yao, S. (2018). Modeling and Optimization of Condensation Heat Transfer at Biphilic Interface. Int. J. Heat Mass Transf. 122, 117–127. doi:10.1016/j.ijheatmasstransfer.2018.01.108
Sommers, A. D., Panth, M., and Eid, K. F. (2020). Self-propelled Water Droplet Movement on a Laser-Etched Radial Gradient Copper Surface. Appl. Therm. Eng. 173, 115226. doi:10.1016/j.applthermaleng.2020.115226
Tokunaga, A., and Tsuruta, T. (2020). Enhancement of Condensation Heat Transfer on a Microstructured Surface with Wettability Gradient. Int. J. Heat Mass Transf. 156, 119839. doi:10.1016/j.ijheatmasstransfer.2020.119839
Varanasi, K. K., Hsu, M., Bhate, N., Yang, W., and Deng, T. (2009). Spatial Control in the Heterogeneous Nucleation of Water. Appl. Phys. Lett. 95 (9), 094101. doi:10.1063/1.3200951
Wang, J., Gao, W., Zhang, H., Zou, M., Chen, Y., and Zhao, Y. (2018). Programmable Wettability on Photocontrolled Graphene Film. Sci. Adv. 4 (9), eaat7392. doi:10.1126/sciadv.aat7392
Wang, J., Sun, L., Zou, M., Gao, W., Liu, C., Shang, L., et al. (2017). Bioinspired Shape-Memory Graphene Film with Tunable Wettability. Sci. Adv. 3 (6), e1700004. doi:10.1126/sciadv.1700004
Wen, R., Xu, S., Zhao, D., Yang, L., Ma, X., Liu, W., et al. (2018). Sustaining Enhanced Condensation on Hierarchical Mesh-Covered Surfaces. Natl. Sci. Rev. 5 (6), 878–887. doi:10.1093/nsr/nwy098
Wilke, K. L., Antao, D. S., Cruz, S., Iwata, R., Zhao, Y., Leroy, A., et al. (2020). Polymer Infused Porous Surfaces for Robust, Thermally Conductive, Self-Healing Coatings for Dropwise Condensation. ACS Nano 14 (11), 14878–14886. doi:10.1021/acsnano.0c03961
Xiao, R., Miljkovic, N., Enright, R., and Wang, E. N. (2013). Immersion Condensation on Oil-Infused Heterogeneous Surfaces for Enhanced Heat Transfer. Sci. Rep. 3, 1988. doi:10.1038/srep01988
Xie, J., She, Q., Xu, J., Liang, C., and Li, W. (2020). Mixed Dropwise-Filmwise Condensation Heat Transfer on Biphilic Surface. Int. J. Heat Mass Transf. 150, 119273. doi:10.1016/j.ijheatmasstransfer.2019.119273
Zhang, C., Shen, C., and Chen, Y. (2017). Experimental Study on Flow Condensation of Mixture in a Hydrophobic Microchannel. Int. J. Heat Mass Transf. 104, 1135–1144. doi:10.1016/j.ijheatmasstransfer.2016.09.029
Zhang, T.-Y., Mou, L.-W., Zhang, J.-Y., Fan, L.-W., and Li, J.-Q. (2020). A Visualized Study of Enhanced Steam Condensation Heat Transfer on a Honeycomb-like Microporous Superhydrophobic Surface in the Presence of a Non-condensable Gas. Int. J. Heat Mass Transf. 150, 119352. doi:10.1016/j.ijheatmasstransfer.2020.119352
Keywords: bioinspired, functional surfaces, dropwise condensation, wettability, heat transfer
Citation: Xu Z and Gulfam R (2022) Perspectives on Bioinspired Functional Surfaces for Heat Transfer Enhancement via Dropwise Condensation. Front. Energy Res. 10:939932. doi: 10.3389/fenrg.2022.939932
Received: 09 May 2022; Accepted: 12 May 2022;
Published: 14 June 2022.
Edited by:
Xiangdong Liu, Yangzhou University, ChinaReviewed by:
Wei Gao, Harvard University, United StatesCopyright © 2022 Xu and Gulfam. This is an open-access article distributed under the terms of the Creative Commons Attribution License (CC BY). The use, distribution or reproduction in other forums is permitted, provided the original author(s) and the copyright owner(s) are credited and that the original publication in this journal is cited, in accordance with accepted academic practice. No use, distribution or reproduction is permitted which does not comply with these terms.
*Correspondence: Raza Gulfam, Z3VsZmFtQHNldS5lZHUuY24=, Z3VsZmFtcmF6YWhhaWRlcnlAaG90bWFpbC5jb20=
Disclaimer: All claims expressed in this article are solely those of the authors and do not necessarily represent those of their affiliated organizations, or those of the publisher, the editors and the reviewers. Any product that may be evaluated in this article or claim that may be made by its manufacturer is not guaranteed or endorsed by the publisher.
Research integrity at Frontiers
Learn more about the work of our research integrity team to safeguard the quality of each article we publish.