Introduction
Phase-change heat transfer has attracted great attention in engineering disciplines in recent years due to the tremendous latent heat and significant efficiency for energy transfer involved in its process (Liu et al., 2013; Cho et al., 2016). As a typical process, vapor-liquid phase-change heat transfer has great potential in applications such as heat exchangers and electronic cooling (Zhang et al., 2011; Wen et al., 2018). When water vapor encounters solid surfaces at low temperature, a vapor to liquid phase-change process, i.e., condensation occurs, accompanied by the release of a large quantity of latent heat (Edalatpour et al., 2018). During this process, two types of condensation can occur, i.e., film condensation and dropwise condensation. In comparison with film condensation, the condensate in the dropwise condensation process exists in the form of droplets rather than a continuous film, which ensures good heat transfer between the vapor and surface (Goswami et al., 2021), shown in Figure 1A. Therefore, dropwise condensation has drawn growing research interest, especially on functional surfaces for enhanced dropwise condensation (Chu et al., 2017; Wang et al., 2018).
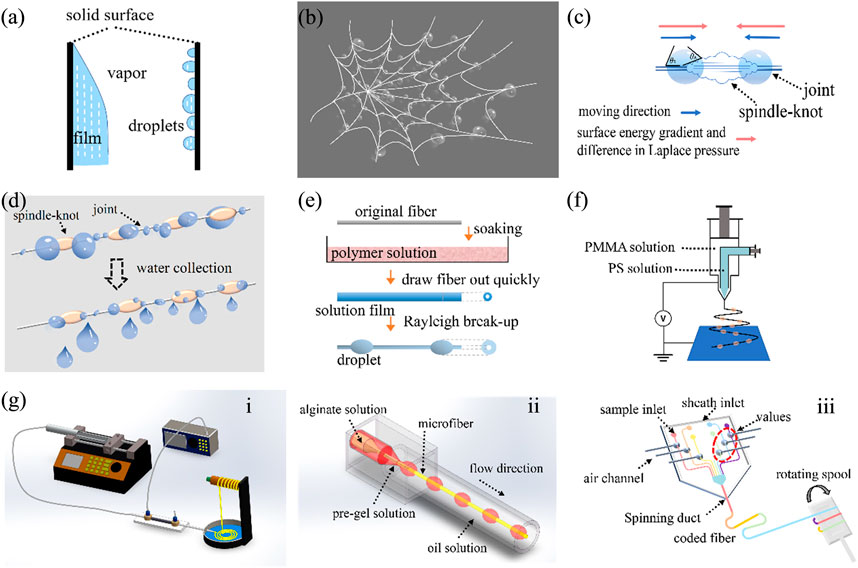
FIGURE 1. Principles, fabrication and application of Bioinspired fiber for directional droplet self-transportation. (A) Two condensation modes; (B) Water collection on spider silks; (C) Mechanism of directional droplet self-transportation; (D) Water-harvesting behavior of bioinspired microfibers; (E) Dip-coating method; (F) Electrospinning, method; (G) Microfluidic method, i) Emulsion-based coaxial microfluidic method, ii)Integrative spinning-coating-emulsification coaxial capillary microfluidic method, iii) Pneumatic-valve microfluidic chip spinning method.
In nature, spider silk (Zheng et al., 2010), cactus (Ju et al., 2012), nepenthes alata (Gorb et al., 2013), etc., with functional surfaces are capable of directional droplet self-transportation, which sheds light on the functional surface for enhanced dropwise condensation. In this paper, the mechanisms for dropwise condensation and directional droplet self-transportation are introduced. Then, several methods for fabricating bioinspired microfibers are summarized to discuss their potential for enhanced condensation.
Principles of Droplet Condensation
Whether it is film condensation or dropwise condensation, the condensate formed acts as a thermal resistance carrier for heat exchange between the vapor and solid surface. Compared with film condensation, the shedding of droplets during droplet condensation leads to a much smaller thermal resistance. The removal of larger droplets will enable increased vapor condensation on the solid surface, which enhances the vapor-liquid phase change heat transfer (Lv et al., 2022).
Directional Droplet Self-Transportation
Due to their unique structure, natural materials such as spider silk, cactus and nepenthes alata exhibit directional water transportation. Specifically, tiny droplets in fog can be captured and merged into larger droplets on the surface of these materials (Venkatesan et al., 2020). Spider silk will be taken as an example to describe its water collection principles and characteristics in detail, shown in Figure 1B.
In a foggy morning, a spider web is usually covered with tiny water droplets. Zheng et al. investigated the directional water collection behavior on spider silk (Zheng et al., 2010). The silk shows a unique structure, including periodic spindle-knots composed of random nanofibrils and joints comprising aligned nanofibrils, resulting in an excellent ability to harvest water (Liu et al., 2022). Moreover, there exists a surface roughness gradient and surface wettability gradient between the periodic spindle knots and the joint. Functional surface with wettability gradient is a promising option for the self-propelled droplet motion, including super-slippery surface (Wang et al., 2017; Wang et al., 2018), textured surface (Wu et al., 2011; Zhang et al., 2016). Thus, the surface energy gradient, induced by the surface roughness gradient and surface wettability gradient, drives the tiny droplet from the smooth joint to the spindle knot, shown in Figure 1C. In addition, due to the curvature gradient of materials, the Laplace pressure gradient is also beneficial for directional droplet self-transportation.
Bioinspired Microfiber Fabrication
Inspired by spider silk, several attempts have been made to fabricate microfibers for directional droplet self-transportation (Haefner et al., 2015; Tian and Wang, 2018), shown in Figure 1D. At present, the fabrication methods for such bioinspired microfibers can be roughly divided into three categories, namely, the Rayleigh instability method, electrodynamics method and microfluidic methods (Chen and Guo, 2019; Li et al., 2019). Dip-coating (Tian et al., 2011), a widely used Rayleigh instability method, utilizes a nylon/carbon fiber and a polymer solution as materials, shown in Figure 1E. The fiber is first placed horizontally on the polymer solution. Then, the fiber is immersed in the polymer solution and pulled out. Therefore, a film of the polymer solution covers the fiber, which breaks up into droplets that hang onto the fiber due to Rayleigh instability. For the electrodynamics method, a necklace structure, which is similar to the spindle-knot, is generated over the nanofibers by the influence of an electrostatic force and surface tension (Ura et al., 2021), shown in Figure 1F. However, the above methods have difficulty precisely controlling the size and spacing of the spindle-knots.
The microfluidic methods are capable of precisely controlling the size and spacing of spindle-knots by adjusting the flow rate or pressure, showing superiority in the preparation of spider-silk-like microfibers (Chen et al., 2013; Chen et al., 2015; Liu et al., 2020; Gao et al., 2021), shown in Figure 1G. The oil-in-water emulsion or gas-in-water emulsion can be formed under the shear force by injecting immiscible liquid or gas phase into the continuous flow. The microfibers with spindle-knots will be fabricated until the continuous phase enclosing the heterogeneous core is solidified and dehydrated. The emulsion-based coaxial microfluidic method is widely used in microfluidic spinning, especially for oil-in-water emulsions (Ji et al., 2015) and air-in-water emulsions (Liu et al., 2016; Tian et al., 2017). The injected air is formed as tiny bubbles with the same size and equal distance. The shell phase using sodium alginate solution will be solidified in the CaCl2 solution due to the cross-linked reaction between alginate solutions and Ca2+ ions. Thus, bioinspired water-harvesting microfibers with periodic spindle-knots can be successfully fabricated.
Performance of Bioinspired Microfibers
According to previous investigations, such bioinspired microfibers demonstrate excellent properties for droplet directional collection, with potential for enhanced condensation (Zhang et al., 2017; Majidi et al., 2021). Zhu et al. found three different condensation morphologies for droplets on microfibers both experimentally and numerically (Zhu et al., 2020). The difference in the condensation morphologies is contributed by the relationship between the thermal conduction resistance within the fiber and condensation heat transfer resistance on the microfiber surface. The water collection capacity of microfibers can be influenced by their own structure and environmental humidity. A test of the water-collecting capacity on different spindle knots for microfibers was conducted by Hou et al. Three types of 1 mm long bioinspired fibers with nearly the same knots (110 μm in length and 200 μm in height) were used (Hou et al., 2012). It can be observed that the fiber with one spindle knot has the ability to collect 1.71 μL water droplets in 160 s, and the fiber with two knots takes over 205 s to collect 3.38 μL droplets. The droplet moves on the artificial spider silk with nearly 0.2–0.3 mm/s (Zheng et al., 2010; Bai et al., 2011).
Conclusion and Outlook
In this paper, we briefly describe the mechanisms for dropwise condensation heat transfer and the importance of droplet removal for enhanced dropwise condensation. Two key factors that dominate droplet self-transportation: the surface energy gradient and Laplace pressure are summarized. Inspired by natural water-harvesting behavior, the enhancement of heat transfer can be realized by accelerating the merging and shedding of droplets on microfibers. Several fabrication methods for bioinspired microfibers and the performance of bioinspired microfibers are introduced. These microfibers are beneficial for achieving enhanced heat exchange, and their capacity to harvest water and save resources is of great significance. So far, the water collection capacity of most microfibers is extremely dependent on high humidity environments and the performance is not good at low humidity conditions. In addition, the effect of extrinsic factors such as temperature, wind and magnetic force on the water collection capacity of microfibers is not clear. Therefore, the performance of the bioinspired fiber in external environment should be improve to extend the application in enhanced condensation.
Author Contributions
WY contributed to the conception of the study. QR wrote the first draft of the manuscript. All authors contributed to manuscript revision and read and approved the submitted version.
Funding
This work is supported by Natural Science Foundation of the Jiangsu Higher Education Institutions of China (20KJB470006).
Conflict of Interest
The authors declare that the research was conducted in the absence of any commercial or financial relationships that could be construed as a potential conflict of interest.
Publisher’s Note
All claims expressed in this article are solely those of the authors and do not necessarily represent those of their affiliated organizations, or those of the publisher, the editors and the reviewers. Any product that may be evaluated in this article, or claim that may be made by its manufacturer, is not guaranteed or endorsed by the publisher.
References
Bai, H., Sun, R., Ju, J., Yao, X., Zheng, Y., and Jiang, L. (2011). Large-Scale Fabrication of Bioinspired Fibers for Directional Water Collection. Small 7 (24), 3429–3433. doi:10.1002/smll.201101408
Chen, W., and Guo, Z. (2019). Hierarchical Fibers for Water Collection Inspired by Spider Silk. Nanoscale 11 (33), 15448–15463. doi:10.1039/C9NR04065J
Chen, Y., Liu, X., and Shi, M. (2013). Hydrodynamics of Double Emulsion Droplet in Shear Flow. Appl. Phys. Lett. 102 (5), 051609. doi:10.1063/1.4789865
Chen, Y., Wu, L., and Zhang, L. (2015). Dynamic Behaviors of Double Emulsion Formation in a Flow-Focusing Device. Int. J. Heat Mass Transf. 82, 42–50. doi:10.1016/j.ijheatmasstransfer.2014.11.027
Cho, H. J., Preston, D. J., Zhu, Y., and Wang, E. N. (2016). Nanoengineered Materials for Liquid-Vapour Phase-Change Heat Transfer. Nat. Rev. Mater 2 (2), 16092. doi:10.1038/natrevmats.2016.92
Chu, F., Wu, X., Zhu, Y., and Yuan, Z. (2017). Relationship between Condensed Droplet Coalescence and Surface Wettability. Int. J. Heat Mass Transf. 111, 836–841. doi:10.1016/j.ijheatmasstransfer.2017.04.052
Edalatpour, M., Liu, L., Jacobi, A. M., Eid, K. F., and Sommers, A. D. (2018). Managing Water on Heat Transfer Surfaces: A Critical Review of Techniques to Modify Surface Wettability for Applications with Condensation or Evaporation. Appl. Energy 222, 967–992. doi:10.1016/j.apenergy.2018.03.178
Gao, W., Lei, Z., Liu, X., and Chen, Y. (2021). Dynamic Liquid Gating Artificially Spinning System for Self-Evolving Topographies and Microstructures. Langmuir 37 (4), 1438–1445. doi:10.1021/acs.langmuir.0c02910
Gorb, E. V., Baum, M. J., and Gorb, S. N. (2013). Development and Regeneration Ability of the Wax Coverage in Nepenthes Alata Pitchers: A Cryo-SEM Approach. Sci. Rep. 3, 3078. doi:10.1038/srep03078
Goswami, A., Pillai, S. C., and McGranaghan, G. (2021). Surface Modifications to Enhance Dropwise Condensation. Surfaces Interfaces 25, 101143. doi:10.1016/j.surfin.2021.101143
Haefner, S., Benzaquen, M., Bäumchen, O., Salez, T., Peters, R., McGraw, J. D., et al. (2015). Influence of Slip on the Plateau-Rayleigh Instability on a Fibre. Nat. Commun. 6 (1), 7409. doi:10.1038/ncomms8409
Hou, Y., Chen, Y., Xue, Y., Wang, L., Zheng, Y., and Jiang, L. (2012). Stronger Water Hanging Ability and Higher Water Collection Efficiency of Bioinspired Fiber with Multi-Gradient and Multi-Scale Spindle Knots. Soft Matter 8 (44), 11236. doi:10.1039/c2sm26421h
Ji, X., Guo, S., Zeng, C., Wang, C., and Zhang, L. (2015). Continuous Generation of Alginate Microfibers with Spindle-Knots by Using a Simple Microfluidic Device. RSC Adv. 5 (4), 2517–2522. doi:10.1039/c4ra10389k
Ju, J., Bai, H., Zheng, Y., Zhao, T., Fang, R., and Jiang, L. (2012). A Multi-Structural and Multi-Functional Integrated Fog Collection System in Cactus. Nat. Commun. 3, 1247. doi:10.1038/ncomms2253
Li, C., Liu, Y., Gao, C., Li, X., Xing, Y., and Zheng, Y. (2019). Fog Harvesting of a Bioinspired Nanocone-Decorated 3D Fiber Network. ACS Appl. Mat. Interfaces 11 (4), 4507–4513. doi:10.1021/acsami.8b15901
Liu, H., Wang, Y., Yin, W., Yuan, H., Guo, T., and Meng, T. (2022). Highly Efficient Water Harvesting of Bioinspired Spindle-Knotted Microfibers with Continuous Hollow Channels. J. Mat. Chem. A 10 (13), 7130–7137. doi:10.1039/D2TA00242F
Liu, X., Chen, Y., and Shi, M. (2013). Dynamic Performance Analysis on Start-Up of Closed-Loop Pulsating Heat Pipes (CLPHPs). Int. J. Therm. Sci. 65, 224–233. doi:10.1016/j.ijthermalsci.2012.10.012
Liu, X., Zhang, C., Yu, W., Deng, Z., and Chen, Y. (2016). Bubble Breakup in a Microfluidic T-Junction. Sci. Bull. 61 (10), 811–824. doi:10.1007/s11434-016-1067-1
Liu, Y., Yang, N., Li, X., Li, J., Pei, W., Xu, Y., et al. (2020). Water Harvesting of Bioinspired Microfibers with Rough Spindle‐Knots from Microfluidics. Small 16 (9), 1901819. doi:10.1002/smll.201901819
Lv, F., Zhao, F., Cheng, D., Dong, Z., Jia, H., Xiao, X., et al. (2022). Bioinspired Functional SLIPSs and Wettability Gradient Surfaces and Their Synergistic Cooperation and Opportunities for Enhanced Condensate and Fluid Transport. Adv. Colloid Interface Sci. 299, 102564. doi:10.1016/j.cis.2021.102564
Majidi, S. S., Su, Y., Jørgensen, M. L., Müller, C., Forooghi, P., Nie, G., et al. (2021). Rayleigh Instability-Driven Coaxial Spinning of Knotted Cell-Laden Alginate Fibers as Artificial Lymph Vessels. ACS Appl. Mat. Interfaces 13 (19), 22142–22149. doi:10.1021/acsami.1c00798
Tian, X., Chen, Y., Zheng, Y., Bai, H., and Jiang, L. (2011). Controlling Water Capture of Bioinspired Fibers with Hump Structures. Adv. Mat. 23 (46), 5486–5491. doi:10.1002/adma.201103111
Tian, Y., and Wang, L. (2018). Bioinspired Microfibers for Water Collection. J. Mat. Chem. A 6 (39), 18766–18781. doi:10.1039/C8TA08104B
Tian, Y., Zhu, P., Tang, X., Zhou, C., Wang, J., Kong, T., et al. (2017). Large-scale Water Collection of Bioinspired Cavity-Microfibers. Nat. Commun. 8 (1), 1080. doi:10.1038/s41467-017-01157-4
Ura, D. P., Knapczyk-Korczak, J., Szewczyk, P. K., Sroczyk, E. A., Busolo, T., Marzec, M. M., et al. (2021). Surface Potential Driven Water Harvesting from Fog. ACS Nano 15 (5), 8848–8859. doi:10.1021/acsnano.1c01437
Venkatesan, H., Chen, J., Liu, H., Liu, W., and Hu, J. (2020). A Spider‐Capture‐Silk‐Like Fiber with Extremely High‐Volume Directional Water Collection. Adv. Funct. Mat. 30 (30), 2002437. doi:10.1002/adfm.202002437
Wang, J., Gao, W., Zhang, H., Zou, M., Chen, Y., and Zhao, Y. (2018). Programmable Wettability on Photocontrolled Graphene Film. Sci. Adv. 4 (9), eaat7392. doi:10.1126/sciadv.aat7392
Wang, J., Sun, L., Zou, M., Gao, W., Liu, C., Shang, L., et al. (2017). Bioinspired Shape-Memory Graphene Film with Tunable Wettability. Sci. Adv. 3 (6), e1700004. doi:10.1126/sciadv.1700004
Wen, R., Ma, X., Lee, Y.-C., and Yang, R. (2018). Liquid-vapor Phase-Change Heat Transfer on Functionalized Nanowired Surfaces and beyond. Joule 2 (11), 2307–2347. doi:10.1016/j.joule.2018.08.014
Wu, J., Ma, R., Wang, Z., and Yao, S. (2011). Do droplets Always Move Following the Wettability Gradient? Appl. Phys. Lett. 98 (20), 204104. doi:10.1063/1.3592997
Zhang, B., Lei, Q., Wang, Z., and Zhang, X. (2016). Droplets Can Rebound toward Both Directions on Textured Surfaces with a Wettability Gradient. Langmuir 32 (1), 346–351. doi:10.1021/acs.langmuir.5b04365
Zhang, C., Chen, Y., Wu, R., and Shi, M. (2011). Flow Boiling in Constructal Tree-Shaped Minichannel Network. Int. J. Heat Mass Transf. 54 (1), 202–209. doi:10.1016/j.ijheatmasstransfer.2010.09.051
Zhang, S., Huang, J., Chen, Z., and Lai, Y. (2017). Bioinspired Special Wettability Surfaces: From Fundamental Research to Water Harvesting Applications. Small 13 (3), 1602992. doi:10.1002/smll.201602992
Zheng, Y., Bai, H., Huang, Z., Tian, X., Nie, F.-Q., Zhao, Y., et al. (2010). Directional Water Collection on Wetted Spider Silk. Nature 463 (7281), 640–643. doi:10.1038/nature08729
Keywords: bioinspired fiber, surface wettability, droplet, microfluidics, electrospinning
Citation: Rui Q and Yu W (2022) Bioinspired Fiber for Directional Droplet Self-Transportation and Its Potential for Enhanced Condensation. Front. Energy Res. 10:928811. doi: 10.3389/fenrg.2022.928811
Received: 26 April 2022; Accepted: 03 May 2022;
Published: 19 May 2022.
Edited by:
Chengbin Zhang, Southeast University, ChinaReviewed by:
Leigang Zhang, Zhengzhou University of Light Industry, ChinaQiang Ma, Jiangsu University, China
Copyright © 2022 Rui and Yu. This is an open-access article distributed under the terms of the Creative Commons Attribution License (CC BY). The use, distribution or reproduction in other forums is permitted, provided the original author(s) and the copyright owner(s) are credited and that the original publication in this journal is cited, in accordance with accepted academic practice. No use, distribution or reproduction is permitted which does not comply with these terms.
*Correspondence: Wei Yu, d2VpeXVAeXp1LmVkdS5jbg==bWFpbHRvOndlaXl1QHl6dS5lZHUuY24=