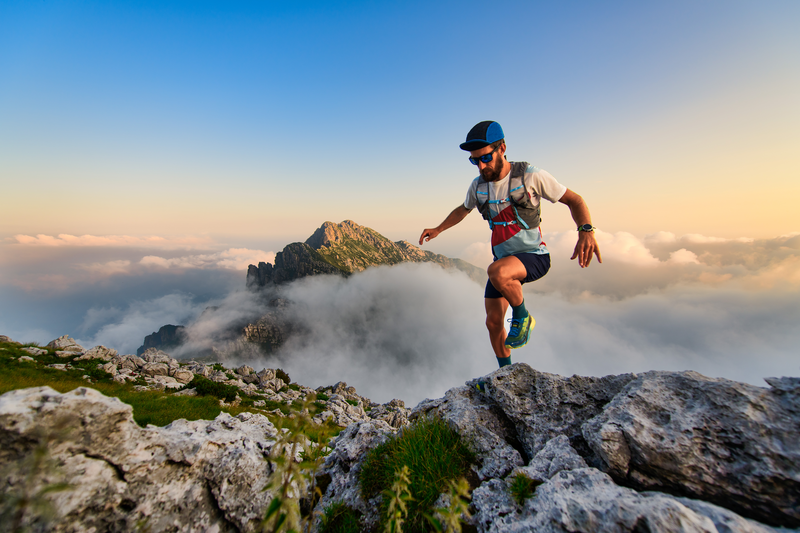
94% of researchers rate our articles as excellent or good
Learn more about the work of our research integrity team to safeguard the quality of each article we publish.
Find out more
ORIGINAL RESEARCH article
Front. Energy Res. , 25 August 2022
Sec. Bioenergy and Biofuels
Volume 10 - 2022 | https://doi.org/10.3389/fenrg.2022.911485
This article is part of the Research Topic Technoeconomic Analysis of Integrated Biorefinery Approaches through Process System Engineering View all 5 articles
In several countries, organic or municipal solid wastes, such as cattle and buffalo manure, have become a serious political and environmental issue owing to organic waste incineration and garbage disposal. To overcome this problem, biogas production from manure, a green treatment that both contributes to the availability of sustainable energy and assists in reducing global warming, was considered. The current study was conducted on the generation of green electricity using cattle and buffalo manure in Pakistan. In 2021, Pakistan has generated 102.742-terawatt hour (TWh) of electricity collectively; biogas share in total production accounted for only 0.98%, which is approximately 1 TWh. Unfortunately, most of the electricity was generated from nonrenewable energy sources. One large animal produces 9–10 kg of manure per day. A system for manure collection can be developed; 30% of total manure produced every day by cattle and buffalo can be collected. Such a type of system is already used for the collection of poultry manure. Pakistan has been blessed with almost 42.4 million buffaloes and 51.5 million cattle. The annual collection of manure from cattle and buffalo at the rate of 30% will be 92.53 million tons. From this manure, approximately 4.63 billion m3 of biogas can be produced and 70% can be collected, which will be 3.24 billion m3. Thus, Pakistan has the potential of generating 19.79 TWh of electricity per day from cattle and buffalo manure. Biogas has the potential to generate over 20% of Pakistan’s total electricity. At the farm level, 100 cattle ranches with 60% collected manure can create roughly 57% of their total consumed electricity. Slurry, a byproduct of anaerobic digestion, can be used as a biofertilizer in fields. It is possible to use cattle manure to make biogas, which is “made by fermentation of organic waste in the absence of oxygen.” It aids in the reduction of fossil fuel dependency, solid waste management, and air pollution control by lowering greenhouse gas emissions. Biogas contributes to the three pillars of sustainable development: economic, environmental, and social development. Biogas contributes significantly to the SDGs and other aspects of sustainable development.
Biomethane is widely known as biogas and also known as gobar gas in Pakistan; other names of biomethane are biofuel, biomass, and wet gas (Eswaran et al., 2021). Anecdotal evidence indicates that during the 10th century BC in Assyria, biogas was used for heating bath water. In the 13th century, the Chinese generated power by utilizing a covered sewage system. Jan Baptista van Helmont was the first who worked on evolving flammable gases from the decomposition of organic matter. Consequent to that in the 18th century, Jan Baptista van Helmont produced combustible gas by decaying organic matter (McCabe, 2012). In 1959, the first biogas plant in Pakistan was set out at Tando Jam Agriculture workshop by M.H Panhwar (Amjid et al., 2011). This was the first step to utilizing solid waste to overcome the shortfall of electricity and conversion of nonrenewable energy sources to renewable energy sources to produce electricity. However, until 2021, Pakistan produces only 0.98% of total electricity generation from biogas (Minister 2021).
Biogas mainly consists of 50%–70% methane, approximately 26%–40% carbon dioxide, 0%–11% of nitrogen, a minute amount of ammonia, 0%–1% hydrogen, and 0%–0.5% oxygen (Zhang et al., 2016; Tian et al., 2017).
Environmental changes are rapidly occurring due to the cutting of trees; approximately 15 billion trees are being chopped down every year (Ehrenberg 2015). We can minimize these figures by utilizing renewable sources. Living trees absorb carbon dioxide and in reverse carbon dioxide is released by burning them. Carbon dioxide makes the planet warmer by absorbing and emitting infrared radiation (Hanson and Ranganathan 2017). Due to the unsteady cost and environmental effects of using fossil fuels for energy, there is growing interest in the use of renewable energy (Inman 2013). Biogas plants aid in cutting dependency on the use of nonrenewable fuel sources, such as oil, natural gas, and coal. As such, the adoption of renewable energy is incrementally becoming paramount because of the negative effects exerted by greenhouse gases when released into the environment (Abbasi et al., 2011). Biogas plants crucially limit the greenhouse effect (Chuanchai and Ramaraj 2018; Desta et al., 2020). Through biogas plants, we obtain not only biogas but also its byproduct, which is called slurry, that can be used as a biofertilizer that is environmentally friendly and odorless (Meijering 2014). The other reason for producing biogas in the future is that nonrenewable energy sources will be exhausted as we already know how long they can last (Liu et al., 2021).
There are some limitations of biogas plants for small- to medium-scale farmers. The construction cost of a biogas plant varies from country to country. Its cost is higher compared with the income of small- to medium-scale farmers (Amigun and Von 2010). Small-scale farmers are still trailing behind in adopting this technology because the initial installment cost of a biogas plant is high and they have inadequate numbers of livestock to feed using a biogas plant (Khayal 2019). Environmental pollution is currently the most important topic; thus, global institutions and local governments are promoting the use of renewable sources of energy, including solar energy, wind energy, and biogas. The government of Pakistan (GOP) announced in October 2020 a new Alternative Renewable Energy Policy 2019 (AEDB 2019). The policy objectives were to create a favorable environment that is maintained by a vigorous framework for the sustainable development of the Alternative Renewable Energy Sector in Pakistan. The GOP’s strategic objectives were affordability of electricity, energy security, availability for all, sustainable development, social equity, environmental protection, and mitigation of climate change, which will further be harnessed under the Alternative Renewable Energy Policy 2019 (ARE Policy 2019) (GOP 2021). In the recent past, GOP also encouraged people especially farmers by facilitating them through low-interest and long-term loans. The Zarai Taraqiati Bank Limited initiates a program for biogas plant loans in monthly installments, and through the solar financing program of the state bank of Pakistan, 5–10 million loans have been granted (GOP 2021).
Biogas for electricity generation is a well-established method that has been extensively used globally. The biogas sector employs an estimated 344,000 people directly or indirectly (Devaraj et al., 2022). China has 110,448 biogas plants with the potential of producing 700,000 m3 gas per year, of which 8,576 are large-scale plants (Huang et al., 2022). Europe has 17,781 biogas plants, most of which are located in Germany. Germany is the leader in Europe, with 10,973 plants, followed by Italy (1655), France (742) and the UK (613). The US has currently housed 2,200 biogas plants (Torrijos 2016).
Globally, in 2010, the total production of biogas was 12.4 billion m3. With an increase of 9% every year, in 2021, biogas production reached 76.8 billion m3. China leads the world in biogas production by producing 3.8 TWh of electricity per year, and the US ranks second by producing 0.224 TWh of electricity per year (Sravan et al., 2021).
Pakistan has vast potential for generating electricity from biogas. In Pakistan, the annual growth rate of the livestock population is approximately 4%. In 2021, the livestock population in Pakistan comprises the following (in millions): cattle, 51.5; buffalo, 42.4; sheep, 31.6; goats, 80.3; camels, 1.1; horses, 0.4; ass, 5.6; and mules, 0.2 (Wing 2021). If manure is collected only from buffalo and cattle, 92.54 million tons can be collected, which is 30 % of the total manure amount. It can produce 4.63 billion m3 of biogas. Approximately 70% of the total biogas produced is lost during filling. We can utilize it to produce electricity and for cooking purposes at home. It will generate approximately 19.79 TWh of electricity.
The livestock population of Pakistan from 2001 to 2021 is shown in Figure 1.
If 30% of cattle and buffalo manure was collected from 2001 to 2021 and if 70% of biogas was produced from it and stored, it could produce 300 TWh of green electricity collectively from 2001 to 2021. Green electricity potential from 2001 to 2021 is shown in Figure 2.
The 10-year data of population and increment ratio in the population of cattle and buffalo from 2001 to 2001 were statistically analyzed. After analyzing the past potential for green electricity in Pakistan, future prediction is statistically shown in Figure 3. According to the analysis, Pakistan will have the potential of producing approximately 24 TWh of green electricity in 2030.
In 2021, Pakistan generated 102.742 TWh of electricity, and the biogas share in total production was only 0.98%, i.e., 1 TWh (Minister 2021). Based on its livestock population, Pakistan has the potential of generating 19.79 TWh of electricity from only cattle and buffalo manure. Cattle and buffalo manure is easy to collect than manure of other livestock. If the government will take serious initiatives and urge entrepreneurs and industrialists to produce electricity from biogas, Pakistan has great potential. Pakistan can not only overcome its electric crisis but also reduce its reliance on nonrenewable energy sources, thereby reducing electricity costs and environmental pollution (Uddin et al., 2016).
Biogas is suitable for cooking, water heating, and room heating; as fuel for generators; in internal combustion engines; and as a substitute for compressed natural gas (CNG) in vehicles (Dimitrov and Zlateva, 2019). After being compressed, it can also be utilized as fuel in automobiles (Figure 4). The use of compressed gas is widespread in Sweden, China, Switzerland, and Germany. The biogas-powered train named Amanda has been in service in Sweden since 2015 (Brauer and Khan 2021). Biogas can be used in dual-fuel engines as diesel engines have been modified to run on 100% biogas, and biogas can replace 80% diesel oil. Petrol and CNG engines can also be modified to run on the biogas. It will cost 75% less than liquefied petroleum gas (SECO 2012; Omer 2017; Stan 2022).
Wide varieties of biomass are available, but mostly, animal manure and crop residue are used (Agbro et al., 2012). Other organic wastes that can be used as biomass are urban waste, sugarcane trash, leaves and branches, tea waste, vegetable waste, fruit waste, and tobacco waste (Stephen and Periyasamy 2018). The biogas is produced because of the fermentation process through the anaerobic digestion of biomass. Carbon dioxide and ammonia are produced by aerobic bacteria if organic material decomposes in aerobic conditions. Biogas is produced by anaerobic bacteria, and this fermentation process takes place in a specific place, which is known as a digester. The airtight digester has a warm and dark environment, which is necessary for the growth of anaerobic bacteria, which take part in the fermentation process because they need a very little amount of oxygen for growth. The main content of the biomass is water, which provides a suitable environment for the growth of bacteria (Ioannou et al., 2021). The soluble nitrogen is present in the solution and produces good quality biofertilizer with methane gas (Ge et al., 2014; Holmes and Smith 2016; Selvankumar et al., 2017).
The chemical process for the generation of biogas occurs in three phases (Deepanraj et al., 2014), as shown in Figure 5. During the first phase, the hydrolysis of the complex compounds occurs. Hydrolysis is a process in which the complex compound of biomass such as fat, carbohydrate, and protein is broken down in the presence of water. Long-chain polymers disintegrate into monomers. This process will conclude in 1 or 2 days at 25°C.
In the second phase, the anaerobic bacteria, also known as acid formers, can grow in the absence of oxygen and produce propionic acid and acetic acid by liberating carbon dioxide. This process occurs in 1 day, with an optimal temperature of 25°C.
In the third phase, methane gas and carbon dioxide are produced because of bacterial reaction and a small amount of hydrogen gas is also released. At 25°C this phase takes approximately 2 weeks to complete the process (Itodo et al., 2021; Rathore and Panwar 2021).
The unique property of biogas is that it is 20% lighter than air as the density of biogas is 1.2 gram/L and the ignition temperature is 700°C. The calorific value of biogas is between 19 and 27 megajoules and without carbon dioxide, and the calorific value is between 32 and 36 megajoules. The methane-to-air ratio is 1:10 for combustion. The production of methane gas from a biogas plant depends on different factors such as temperature, pH value, feeding rate, pressure, and solid-to-moisture ratio in biomass. The methane content also relies on the organic material, which is used as biomass. Some important values are shown in Table 1.
The electricity generation from 100 cattle farms is calculated as shown in Table 2.. The volume of biogas from the 100 cattle manure is 45 m3 per day. Cattle manure generates 0.05 m3 biogas per kilogram (Amon et al., 2007). Cattle excreted approximately 8–12 kg of manure per day depending on the feeding contents and feeding ratio (Palma 2019). Approximately 9 kg of manure can be collected, after wastage during handling and collection (Smith and Williams, 2016). One cattle manure produces 0.45 m3 per day of biogas; hence, 100 cattle manure production of biogas is 45 m3 per day, as shown in Table 3.
Biogas has a calorific value of 20–23 megajoules/m3 (Sharma et al., 2022). The total energy produced from 45 m3 biogas is 990 megajoules. To convert megajoules into kWh, the value is multiplied by 3.6 (56); 275 kWh is the total energy produced by 45 m3 biogas, as shown in Table 2 and Figure 6.
During the conversion of this energy into electric energy, 65% of energy is lost as heat and additional mechanical losses as used by electrical generators (Kondaveeti et al., 2019). Approximately 35% of energy converted into electrical energy is given in Table 4. The total electricity produced per day is 96.25 kWh, and we obtain 2,887.5 kWh electricity per month by multiplying the value by 30 days.
According to farmer reviews, 100 cattle farms consumed 5000 kWh of electricity per month on average, as shown in Table 5. Thus, farmers can generate approximately 57.75 % of energy from their cattle manure. The current commercial unit price in Pakistan is 19.95 (MEPCO 2022), as shown in (Table 5.) If farmers utilize this electricity at their farm, they can redeem approximately PKR 57600.
The total biogas from 100 cattle manure is 45 m3, which generates 990 megajoules of energy, as shown in Table 6.
We can supply biogas to 25 houses because the average stove running time in a house is 4–5 h. At 100 cattle farms, the total energy production is 990 megajoules per day. Medium stoves use 8–10 megajoules per hour, as shown in (Figure 7; Table 7).
Biogas contributes to the three pillars of sustainable development dimensions (SDDs). The first pillar of SDD is “Economic.” SDGs related to this SDD are as follows: SDG number 1, “No poverty,” in which biogas contributes to this by providing affordable biofertilizer and eliminating the problems of the complex supply chain of fertilizer (Herrmann 2013); SDG number 2, “Zero hunger,” in which it contributes by generating new jobs by adopting new businesses and increasing the yield of crops due to the availability of reasonable price fertilizer and enhancing the soil fertility by providing them lost nutrients and carbon (Arthurson 2009); SDG number 3, “Decent work and economic growth,” in which it contributes by increasing the gross domestic product by utilizing the waste (Kondaveeti et al., 2019; MPECO 2022; Sharma et al., 2022); and SDG number 4, “Industry, Innovation, and infrastructure,” in which it contributes by building sustainable infrastructure and providing electricity to small-scale industries at the rural level (Verhoog et al., 2016; Aziz et al., 2019).
The second pillar of SDDs is “Social.” SDGs related to this SDD are as follows: SDG number 5, “Good health and well-being,” in which biogas contributes to this by reducing methane emission (Ilo et al., 2020; Zeng et al., 2020); SDG number 6, “Quality education,” in which it contributes by producing electricity in rural areas to improve education quality; SDG number 7, “Sustainable cities and communities,” in which biogas plants contribute by aiding in preventing diseases through waste organic material collection (Kelebe et al., 2017; Yasar et al., 2017; Chowdhury et al., 2020); and SDG number 8, “Peace and justice strong institutes,” in which, as some studies suggest, an increase in power availability is linked to peace (Winther 2008).
The third pillar of SDDs is “Environment.” The SDGs related to this SDD are as follows: SDG number 9, “Clean water and sanitation,” in which wastewater treatment facilities are developed at the rural level due to electricity production (Adnan et al., 2019); SDG number 10, “Affordable and clean energy,” in which energy from biogas increases the overall energy sustainability, reliability, affordability, and energy storage capacity (Khan et al., 2017); SDG number 11, “Responsible consumption and production,” in which as biogas utilization efficiency of using natural resources increases, air and water pollution decreases and waste recycle process improves (Jeong and Park, 2017; Paolini et al., 2018); SDG number 12, “Climate action,” in which biogas will reduce greenhouse gas (GHG) emission by producing low-quantity GHG production and decreasing livestock industry methane gas (Lima et al., 2018; Tsai 2018); SDG number 13, “Life below water,” in which biogas will prevent land source pollution; hence, aiding in marine pollution reduction. SDG number 14, “Life on land,” in which biogas will replace solid fossil fuels and help in reducing deforestation. Freshwater ecosystem will be improved by enchanting wastewater treatment (Studer et al., 2017; Tamburini et al., 2022).
Biogas electricity is the most effective renewable energy source. Other renewable energy sources, compared with biogas, have more limitations. We will discuss them one by one. Wind energy is a renewable energy source that is dependent on wind. Wind turbines usually work at 30% capacity, and high winds may harm wind turbines and cause more severe problems when struck by lightning. The edges of the wind turbine pose a threat to wildlife, particularly birds. It also poses a threat to people during heavy storms. It is an expensive setup and produces noise pollution. The wind turbine can only be installed at specific locations (Shifeng and Sicong 2015; Swofford and Slattery 2010; Adeyeye et al., 2019; Mittal et al., 2010).
Solar panels are another source of renewable energy, but they can only produce energy in the presence of sunlight. The massive disadvantage of solar energy is that it is not constant. Solar panels convert only 22% of the Sun’s energy, which necessitates a wide surface area to produce the required energy. Crystalline wafer solar panels are too bulky. Other less bulky panels are available, but their efficacy is less compared with crystalline wafer ones. Storage is another big problem. Batteries are bulky and have not yet reached their full potential (Lakatos et al., 2011; Ploetz et al., 2016; Sampaio et al., 2017; Ugli 2019).
Geothermal plants are renewable energy sources in which the energy of the Earth’s crust is utilized. Geothermal plants are limited to areas where energy is accessible. During digging, many stored harmful gases are released. Digging increases the possibility of an earthquake. Extensive management of geothermal plants is required for sustainability. The digging process is also expensive (Li et al., 2015; Anderson and Rezaie 2019; Kulasekara and Seynulabdeen 2019; Zimnukhova et al., 2019).
Hydroelectric energy is generated by utilizing water flow work as a renewable energy source. However, it has many disadvantages. The most important impact of hydroelectric energy is on the environment. To produce this energy, dams must be installed with new electric lines, which can impact the environment. Dams also block fish migration, and reservoirs of water damage the natural habitat. Reservoirs impact not only animal habitats but also the local human population by forcing them to migrate. Villages, cities, and towns have been displaced due to dams, which displace local culture. Dam construction is extremely costly and time-consuming. They are limited reservoirs although water is an unlimited resource because dam building conditions are limited. Lower-than-normal levels of water can also result in drought conditions. Dams are also not safe at all times, such as in an incident in China in 1975, when the destruction of a dam lead to the death of approximately 171,000 people (Kirmani et al., 2021; Alagumalai et al., 2022; Duong 2022; Sivapriya and Sherin 2022).
In contrast, biogas plants are less expensive and easier to build, depending on the requirements and availability of organic waste. Biogas plants should be built from the home level to the industrial level. They enable people to generate energy in the form of electricity, allowing them to use stoves for cooking purposes (Manesh et al., 2020; Czekała 2022; Syahri et al., 2022).
The study on green electricity derived from cattle manure has shown that organic waste is available everywhere, and if the government takes serious initiative, it can be a reliable source of renewable and green energy. Pakistan has the potential of producing almost 20% of total electricity production from biogas. However, according to a report of the Finance Department, Pakistan produced only 0.98 % of total electricity production from biogas in 2021. Biogas is more cost-effective, has a lower environmental impact, and has a higher calorific value than coal and wood. Biogas is a convenient electrical source that may be used for cooking and heating.
Natural gas has a carbon intensity of 469 g/kWh; oil, 840 g/kWh; coal, 1000 g/kWh; and biomass, only 18 g/kWh, as shown in Figure 8. Biomass emits 96.16%, 97.85%, and 98.2% less carbon per kWh compared with natural gas, oil, and electricity, respectively, as shown in Figure 8. The CO2 absorbs infrared radiation and re-emits it in all directions, with half of the energy emitted into space and the other half emitted as heat toward the planet, resulting in the greenhouse effect. We can reduce CO2 emissions and keep the Earth at a constant temperature by utilizing renewable energy sources.
There are approximately 35 million Pakistanis who work in the cattle industry, which accounts for 11% of the country’s GDP. Biogas facilities can help Pakistan’s economy by reducing the country’s reliance on expensive fuels. Backyard farmers can sell their excrement and make a small profit. The farmers may generate over 60% of their total electricity on their farms using manure, allowing them to develop their farms while saving money on bills.
There are numerous opportunities for a large number of farmers to produce biogas and use it on their farms for electricity generation, as well as for small business owners to provide gas to homes for cooking. It would be profitable for entrepreneurs to develop a biogas plant in the industry area and supply electricity to the workers.
The original contributions presented in the study are included in the article/supplementary material; further inquiries can be directed to the corresponding authors.
Author Contributions: Conceptualization, MA and ARA, experiments performed by, MA and ARA validation by, RQ and AN; formal analysis, MA and ARA writing—original draft preparation by MA, ARA, MHT, and NK, review and editing, TM and HA.
The authors acknowledge the support provided by King Abdullah City for Atomic and Renewable Energy (K.A.CARE) under K.A.CARE-King Abdul-Aziz University Collaboration Program.
The authors declare that the research was conducted in the absence of any commercial or financial relationships that could be construed as a potential conflict of interest.
All claims expressed in this article are solely those of the authors and do not necessarily represent those of their affiliated organizations, or those of the publisher, the editors, and the reviewers. Any product that may be evaluated in this article, or claim that may be made by its manufacturer, is not guaranteed or endorsed by the publisher.
Abbasi, T., Tauseef, S., and Abbasi, S. A. (2011). Biogas energy. Springer Science & Business Media.
Abusoglu, A., Tozlu, A., and Anvari-Moghaddam, A. (2021). District heating and electricity production based on biogas produced from municipal WWTPs in Turkey: A comprehensive case study. Energy 223, 119904. doi:10.1016/j.energy.2021.119904
Adeyeye, K. A., Ijumba, N., and Colton, J. S. J. P. (2019). A techno-economic model for wind energy costs analysis for low wind speed areas. Processes 9 (8), 1463. doi:10.3390/pr9081463
Adnan, A. I., Ong, M. Y., Nomanbhay, S., Chew, K. W., and Show, P. L. J. B. (2019). Technologies for biogas upgrading to biomethane: A review. Bioeng. (Basel). 6 (4), 92. doi:10.3390/bioengineering6040092
AEDB (2019). Alternative and renewable energy policy 2019. Islamabad, Pakistan: Government of Pakistan. (AEDB) AEDB.
Agbro, E. B., Ogie, N. A. J. Rjie, and sciences, a. (2012). A comprehensive review of biomass resources and biofuel production potential in Nigeria: Potential and prospects. Environ. Health Rev. 1 (3), 149–155. doi:10.1515/reveh-2015-0015
Alagumalai, A., Anvari, S., and Awad, M. M. (2022). Solar-driven water treatment. Elsevier, 1–35. doi:10.1016/B978-0-323-90991-4.00005-0Water: A global grand challenge and a path forward
Amigun, B., and Von Blottnitz, H. J. R. (2010). Capacity-cost and location-cost analyses for biogas plants in Africa. Resour. Conserv. Recycl. 55 (1), 63–73. doi:10.1016/j.resconrec.2010.07.004
Amjid, S. S., Bilal, M., Nazir, M. S., and Hussain, A. (2011). Biogas, renewable energy resource for Pakistan. Renew. Sustain. Energy Rev. 15, 2833–2837. doi:10.1016/j.rser.2011.02.041
Amon, T., Amon, B., Kryvoruchko, V., Zollitsch, W., Mayer, K., Gruber, L. J. A., et al. (2007). Biogas production from maize and dairy cattle manure—Influence of biomass composition on the methane yield. Agric. Ecosyst. Environ. 118 (1-4), 173–182. doi:10.1016/j.agee.2006.05.007
Anderson, A., and Rezaie, B. J. A. E. (2019). Geothermal technology: Trends and potential role in a sustainable future. Appl. Energy 248, 18–34. doi:10.1016/j.apenergy.2019.04.102
Arshad, M., Bano, I., Khan, N., Shahzad, M. I., Younus, M., Abbas, M., et al. (2018). Electricity generation from biogas of poultry waste: An assessment of potential and feasibility in Pakistan. Renew. Sustain. Energy Rev. 81, 1241–1246. doi:10.1016/j.rser.2017.09.007
Arthurson, V. J. E. (2009). Closing the global energy and nutrient cycles through application of biogas residue to agricultural land–potential benefits and drawback. Energies 2 (2), 226–242. doi:10.3390/en20200226
Aziz, N. I. H. A., Hanafiah, M. M., and Ali, M. Y. M. J. Re (2019). Sustainable biogas production from agrowaste and effluents–A promising step for small-scale industry income. Renew. Energy 132, 363–369. doi:10.1016/j.renene.2018.07.149
Banja, M., Jégard, M., Motola, V., and Sikkema, R. (2019). Support for biogas in the EU electricity sector – a comparative analysis. Biomass Bioenergy 128, 105313. doi:10.1016/j.biombioe.2019.105313
Bedoić, R., Jurić, F., Ćosić, B., Pukšec, T., Čuček, L., and Duić, N. (2020). Beyond energy crops and subsidised electricity – a study on sustainable biogas production and utilisation in advanced energy markets. Energy 201, 117651. doi:10.1016/j.energy.2020.117651
Bonten, L. T. C., Zwart, K. B., Rietra, R. P. J. J., Postma, R., and de Haas, M. J. G. (2014). Bio-slurry as fertilizer; Is bio-slurry from household digesters a better fertilizer than manure? A literature review. Wageningen, Alterra, Wageningen UR: University & Research centre. Alterra report 2519, 46.
Brauer, H. B., and Khan, J. J. JoC. P. (2021). Diffusion of biogas for freight transport in Sweden: A user perspective. J. Clean. Prod. 312, 127738. doi:10.1016/j.jclepro.2021.127738
Chowdhury, T., Chowdhury, H., Hossain, N., Ahmed, A., Hossen, M. S., Chowdhury, P., et al. (2020). Latest advancements on livestock waste management and biogas production: Bangladesh’s perspective. J. Clean. Prod. 272, 122818. doi:10.1016/j.jclepro.2020.122818
Chuanchai, A., and Ramaraj, R. (2018). Sustainability assessment of biogas production from buffalo grass and dung: Biogas purification and bio-fertilizer. 3 Biotech. 8 (3), 151. doi:10.1007/s13205-018-1170-x
Czekała, W. (2022). Biogas as a sustainable and renewable energy source. Clean Fuels for mobility. Springer, 201–214.
Deepanraj, B., Sivasubramanian, V., and Jayaraj, S.. (2014). Biogas generation through anaerobic digestion process-an overview. 18:5.
Desta, G. A., Melka, Y., Sime, G., Yirga, F., Marie, M., and Haile, M. (2020). Biogas technology in fuelwood saving and carbon emission reduction in southern Ethiopia. Heliyon 6 (10), e04791. doi:10.1016/j.heliyon.2020.e04791
Devaraj, T., Mani, Y., Aathika, S., Palaniyandi, S., Gurunathan, B., and Subramanian, S. (2022). Biofuels Bioenergy. Elsevier, 215–231. doi:10.1016/B978-0-323-85269-2.00009-5Biogas production potential in India, the latest biogas upgradation techniques and future application in a fuel cell
R. Dimitrov, and P. Zlateva (Editors) (2019). “Possible uses of biogas for power purposes,” 2019 11th electrical engineering faculty conference (BulEF) (IEEE).
Duong, T. H. (2022). Studying the water scarcity in the downstream due to factors in the upstream—a case study. Climate Change and water security. Springer, 325–333. doi:10.1007/978-981-16-5501-2_26
Duval, B. D., Anderson-Teixeira, K. J., Davis, S. C., Keogh, C., Long, S. P., Parton, W. J., et al. (2013). Predicting greenhouse gas emissions and soil carbon from changing pasture to an energy crop. PloS one 8 (8), e72019. doi:10.1371/journal.pone.0072019
Ehrenberg, R. (2015). Global forest survey finds trillions of trees. Nature. doi:10.1038/nature.2015.18287
Eswaran, N., Parameswaran, S., and Johnson, T. S. (2021). Biofuels and sustainability. Methods Mol. Biol. Clift. NJ) 2290, 317–342. doi:10.1007/978-1-0716-1323-8_20
Font-Palma, C. J. C. (2019). Methods for the treatment of cattle manure—A review. C (Basel). 5 (2), 27. doi:10.3390/c5020027
Ge, X., Matsumoto, T., Keith, L., and Li, Y. (2014). Biogas energy production from tropical biomass wastes by anaerobic digestion. Bioresour. Technol. 169, 38–44. doi:10.1016/j.biortech.2014.06.067
Go, Pakistan (2021). “Pakistan domestic biogas programme,” in Rural support programmes network (RSPN) P.
Gop, (2021). “Energy,” in Insider: Why burning trees for energy harms the climate. Editors F. Division, C. Hanson, and J. Ranganathan.
Herrmann, A. (2013). Biogas production from maize: Current state, challenges and prospects. 2. Agronomic and environmental aspects. Bioenergy Res. 6 (1), 372–387. doi:10.1007/s12155-012-9227-x
Holmes, D. E., and Smith, J. A. (2016). Biologically produced methane as a renewable energy source. Adv. Appl. Microbiol. 97, 1–61. doi:10.1016/bs.aambs.2016.09.001
Huang, X., Wang, S., Shi, Z., Fang, L., and Yin, C. J. E. (2022). Challenges and strategies for biogas production in the circular agricultural waste utilization model: A case study in rural China. Energy 241, 122889. doi:10.1016/j.energy.2021.122889
Ilo, O. P., Simatele, M. D., Mkhize, N. M., and Prabhu, N. G. J. S. (2020). The benefits of water hyacinth (Eichhornia crassipes) for southern africa: A review. Sustainability 12 (21), 9222. doi:10.3390/su12219222
Inman, M. J. S. A. (2013). The true cost of fossil fuels. Sci. Am. 308 (4), 58–61. doi:10.1038/scientificamerican0413-58
Ioannou-Ttofa, L., Foteinis, S., Moustafa, A. S., Abdelsalam, E., Samer, M., and Fatta-Kassinos DJJoCP, (2021). Life cycle assessment of household biogas production in Egypt: Influence of digester volume, biogas leakages, and digestate valorization as biofertilizer. J. Clean. Prod. 286, 125468. doi:10.1016/j.jclepro.2020.125468
Jeong YHermanowicz, S. W., and Park, C. J. Wr (2017). Treatment of food waste recycling wastewater using anaerobic ceramic membrane bioreactor for biogas production in mainstream treatment process of domestic wastewater. Water Res. 123, 86–95. doi:10.1016/j.watres.2017.06.049
Kelebe, H. E., Ayimut, K. M., Berhe, G. H., and Hintsa, K. J. E. E. (2017). Determinants for adoption decision of small scale biogas technology by rural households in Tigray, Ethiopia. Energy Econ. 66, 272–278. doi:10.1016/j.eneco.2017.06.022
Khan, I. U., Othman, M. H. D., Hashim, H., Matsuura, T., Ismail, A., Rezaei-DashtArzhandi, M., et al. (2017). Biogas as a renewable energy fuel–A review of biogas upgrading, utilisation and storage. Energy Convers. Manag. 150, 277–294. doi:10.1016/j.enconman.2017.08.035
Kirmani, F., Pal, A., Mudgal, A., Shrestha, A., Siddiqui, A. J. I. JoI. S., and Technology, R. (2021). Advantages and disadvantages of hydroelectric power plant. Int. J. Innov. Sci. Technol.
Kondaveeti, S., Patel, S. K. S., Pagolu, R., Li, J., Kalia, V. C., Choi, M-S., et al. (2019). Conversion of simulated biogas to electricity: Sequential operation of methanotrophic reactor effluents in microbial fuel cell. Energy 189, 116309. doi:10.1016/j.energy.2019.116309
H. Kulasekara, and V. Seynulabdeen (Editors) (2019). A review of geothermal energy for future power generation2019 5th International Conference on Advances in Electrical Engineering (ICAEE) (IEEE).
Lakatos, L., Hevessy, G., and Kovács, J. J. W. F. (2011). Advantages and disadvantages of solar energy and wind-power utilization. World Futur. 67 (6), 395–408. doi:10.1080/02604020903021776
Li, K., Bian, H., Liu, C., Zhang, D., Yang, Y. J. R., and Reviews, S. E. (2015). Comparison of geothermal with solar and wind power generation systems. Renew. Sustain. Energy Rev. 42, 1464–1474. doi:10.1016/j.rser.2014.10.049
Lima, R. M., Santos, A. H., Pereira, C. R., Flauzino, B. K., Pereira, A. C. O., Nogueira, F. J., et al. (2018). Spatially distributed potential of landfill biogas production and electric power generation in Brazil. Waste Manag. 74, 323–334. doi:10.1016/j.wasman.2017.12.011
Liu, Y., Cruz-Morales, P., Zargar, A., Belcher, M. S., Pang, B., Englund, E., et al. (2021). Biofuels for a sustainable future. Cell. 184 (6), 1636–1647. doi:10.1016/j.cell.2021.01.052
Manesh, M. K., Rezazadeh, A., and Kabiri, S. J. R. E. (2020). A feasibility study on the potential, economic, and environmental advantages of biogas production from poultry manure in Iran. Renew. Energy 159, 87–106. doi:10.1016/j.renene.2020.05.173
Martin, A.Al McGartland USEPA (2014). The nexus of biofuels, climate change, and human health. Judith Qualters CfDCaP. Washington, DC: National Academies Press (US). Roundtable on Environmental Health Sciences, Research, and Medicine; Board on Population Health and Public Health Practice; Institute of Medicine.Philbert
Martínez-Gutiérrez, E. (2018). Biogas production from different lignocellulosic biomass sources: Advances and perspectives. 3 Biotech. 8 (5), 233–238. doi:10.1007/s13205-018-1257-4
W. McCabe JE (Editor) (2012). A short history of anaerobic digestion (Washington, DC: National Academies Press (US)).
Megajoules to Kilowatt-hours Conversion (2022). inchcalculator. [database on the Internet]. Available from: https://www.inchcalculator.com/convert/megajoule-to-kilowatt-hour/.
Mittal, R., Sandhu, K., and Jain, D. J. I. JoEsdevelopment (2010). An overview of some important issues related to wind energy conversion system (WECS). Int. J. Environ. Sci. Dev. 1 (4), 351–363. doi:10.7763/ijesd.2010.v1.69
Nindhia, T. G. T., McDonald, M., and Styles, D. (2021). Greenhouse gas mitigation and rural electricity generation by a novel two-stroke biogas engine. J. Clean. Prod. 280, 124473. doi:10.1016/j.jclepro.2020.124473
Omer, A. (2017). Biogas technology for sustainable energy generation: Development and perspectives. MOJ App Bio Biomech. 1 (4), 137–148. doi:10.15406/mojabb.2017.01.00022
Paolini, V., Petracchini, F., Segreto, M., Tomassetti, L., Naja, N., Cecinato, A. J. JoE. S., et al. (2018). Environmental impact of biogas: A short review of current knowledge. J. Environ. Sci. Health Part A 53 (10), 899–906. doi:10.1080/10934529.2018.1459076
Pathak, H., Jain, N., Bhatia, A., Mohanty, S., and Gupta, N. (2009). Global warming mitigation potential of biogas plants in India. Environ. Monit. Assess. 157 (1-4), 407–418. doi:10.1007/s10661-008-0545-6
R. Ploetz, R. Rusdianasari, and E. Eviliana (Editors) (2016). “Renewable energy: Advantages and disadvantages,” Proc. Forum res. Sci. Technol. (FIRST) 2016. Politeknik negeri sriwijaya.
Sampaio, P. G. V., González, M. O. A. J. R., and Reviews, S. E. (2017). Photovoltaic solar energy: Conceptual framework. Renew. Sustain. Energy Rev. 74, 590–601. doi:10.1016/j.rser.2017.02.081
Selvankumar, T., Sudhakar, C., Govindaraju, M., Selvam, K., Aroulmoji, V., Sivakumar, N., et al. (2017). Process optimization of biogas energy production from cow dung with alkali pre-treated coffee pulp. 3 Biotech. 7 (4), 254. doi:10.1007/s13205-017-0884-5
Sharma, H., Mahla, S. K., and Dhir, A. J. I. JoH. E. (2022). Effect of utilization of hydrogen-rich reformed biogas on the performance and emission characteristics of common rail diesel engine. Int. J. Hydrogen Energy 47 (18), 10409–10419. doi:10.1016/j.ijhydene.2022.01.073
Sivapriya, S., and Anne Sherin, A. (2022). Causes and consequences of dam failures—case study. Sustainable Practices and Innovations in civil engineering. Springer, 155–159. doi:10.1007/978-981-16-5041-3_11
Smith, K., and Williams, A. G. (2016). Production and management of cattle manure in the UK and implications for land application practice. Soil Use Manag. 32, 73–82. doi:10.1111/sum.12247
Sravan, J. S., Tharak, A., and Mohan, S. V. (2021). “Chapter 1 - status of biogas production and biogas upgrading: A global scenario,” in Emerging technologies and biological systems for biogas upgrading. Editors N. Aryal, L. D. Mørck Ottosen, M. V. Wegener Kofoed, and D. Pant (Academic Press), 3–26. doi:10.1016/B978-0-12-822808-1.00002-7
Stan, C. (2022). “Heat, electricity and fuel from biogas,” in Energy versus carbon dioxide (Berlin, Heidelberg: Springer), 204–213. doi:10.1007/978-3-662-64162-0_17
Stephen, J. L., and Periyasamy, B. J. F. (2018). Innovative developments in biofuels production from organic waste materials: A review. Fuel 214, 623–633. doi:10.1016/j.fuel.2017.11.042
Studer, I., Boeker, C., and Geist, J. J. E. I. (2017). Physicochemical and microbiological indicators of surface water body contamination with different sources of digestate from biogas plants. Ecol. Indic. 77, 314–322. doi:10.1016/j.ecolind.2017.02.025
Stürmer, B., Theuretzbacher, F., and Saracevic, E. (2021). Opportunities for the integration of existing biogas plants into the Austrian electricity market. Renew. Sustain. Energy Rev. 138, 110548. doi:10.1016/j.rser.2020.110548
Swofford, J., and Slattery, M. (2010). Public attitudes of wind energy in Texas: Local communities in close proximity to wind farms and their effect on decision-making. Energy policy 38 (5), 2508–2519. doi:10.1016/j.enpol.2009.12.046
Syahri, S. N. K. M., Hasan, H. A., Abdullah, S. R. S., Othman, A. R., Abdul, P. M., Azmy, R. F. H. R., et al. (2022). Recent challenges of biogas production and its conversion to electrical energy. J. Ecol. Eng. 23 (3), 251–269. doi:10.12911/22998993/146132
Tamburini, E., Gaglio, M., Castaldelli, G., and Fano, E. A. J. S. (2022). Is bioenergy truly sustainable when land-use-change (LUC) emissions are accounted for? The case-study of biogas from agricultural biomass in emilia-romagna region. Italy. Sustain. 12 (8), 3260. doi:10.3390/su12083260
Tian, Y., Zhang, H., Chai, Y., Wang, L., Mi, X., Zhang, L., et al. (2017). Biogas properties and enzymatic analysis during anaerobic fermentation of phragmites australis straw and cow dung: Influence of nickel chloride supplement. Biodegradation 28 (1), 15–25. doi:10.1007/s10532-016-9774-5
TJTJIJoE, U., and Systems, I. (2019). The importance of alternative solar energy sources and the advantages and disadvantages of using solar panels in this process 3(4).
Torrijos, M. (2016). State of development of biogas production in Europe. Procedia Environ. Sci. 35, 881–889. doi:10.1016/j.proenv.2016.07.043
Tsai, W-T. J. F. (2018). Regulatory promotion and benefit analysis of biogas-power and biogas-digestate from anaerobic digestion in Taiwan’s livestock industry. Ferment. (Basel). 4 (3), 57. doi:10.3390/fermentation4030057
Uddin, W., Khan, B., Shaukat, N., Majid, M., Mujtaba, G., Mehmood, A., et al. (2016). Biogas potential for electric power generation in Pakistan: A survey. Renew. Sustain. Energy Rev. 54, 25–33. doi:10.1016/j.rser.2015.09.083
Verhoog, R., Ghorbani, A., and Dijkema, G. P. J. (2016). Modelling socio-ecological systems with maia: A biogas infrastructure simulation. Environ. Model. Softw. 81, 72–85. doi:10.1016/j.envsoft.2016.03.011
Wang, S., Wang, S. J. R., and Reviews, S. E. (2015). Impacts of wind energy on environment: A review. Renew. Sustain. Energy Rev. 49, 437–443. doi:10.1016/j.rser.2015.04.137
Wing, E. As (2021). in Pakistan ecnomic survay. Editors F. Division, and Pakistan Go (Islamabad, Pakistan: Finance Division).
Winther, T. (2008). The impact of electricity: Development, desires and dilemmas. Berghahn Books. Available at: https://doi.org/10.3167/9781845454951
Yasar, A., Nazir, S., Tabinda, A. B., Nazar, M., Rasheed, R., and Afzaal, M. J. R. E. (2017). Socio-economic, health and agriculture benefits of rural household biogas plants in energy scarce developing countries: A case study from Pakistan. Renew. Energy 108, 19–25. doi:10.1016/j.renene.2017.02.044
Zeng, J., Xu, R., Sun, R., Niu, L., Liu, Y., Zhou, Y., et al. (2020). Evaluation of methane emission flux from a typical biogas fermentation ecosystem in China. J. Clean. Prod. 257, 120441. doi:10.1016/j.jclepro.2020.120441
Zhang, B., Zhao, H., Yu, H., Chen, D., Li, X., Wang, W., et al. (2016). Evaluation of biogas production performance and archaeal microbial dynamics of corn straw during anaerobic Co-digestion with cattle manure liquid. J. Microbiol. Biotechnol. 26 (4), 739–747. doi:10.4014/jmb.1509.09043
Keywords: solid waste, green electricity, animal manure, greenhouse gas, cattle manure
Citation: Arshad M, Ansari AR, Qadir R, Tahir MH, Nadeem A, Mehmood T, Alhumade H and Khan N (2022) Green electricity generation from biogas of cattle manure: An assessment of potential and feasibility in Pakistan. Front. Energy Res. 10:911485. doi: 10.3389/fenrg.2022.911485
Received: 02 April 2022; Accepted: 21 July 2022;
Published: 25 August 2022.
Edited by:
Abdul-Sattar Nizami, Government College University, Lahore, PakistanReviewed by:
Israr Ahmad, Khwaja Fareed University of Engineering and Information Technology, PakistanCopyright © 2022 Arshad, Ansari, Qadir, Tahir, Nadeem, Mehmood, Alhumade and Khan. This is an open-access article distributed under the terms of the Creative Commons Attribution License (CC BY). The use, distribution or reproduction in other forums is permitted, provided the original author(s) and the copyright owner(s) are credited and that the original publication in this journal is cited, in accordance with accepted academic practice. No use, distribution or reproduction is permitted which does not comply with these terms.
*Correspondence: Muhammad Arshad, TXVoYW1tYWQuQXJzaGFkQHV2YXMuZWR1LnBr; Rahman Qadir, cnFzdW1yYUBnbWFpbC5jb20=
Disclaimer: All claims expressed in this article are solely those of the authors and do not necessarily represent those of their affiliated organizations, or those of the publisher, the editors and the reviewers. Any product that may be evaluated in this article or claim that may be made by its manufacturer is not guaranteed or endorsed by the publisher.
Research integrity at Frontiers
Learn more about the work of our research integrity team to safeguard the quality of each article we publish.