- Chair of Energy Systems, TUM School of Engineering and Design, Technical University of Munich, Garching, Germany
Several unforeseen events have affected the energy market in recent years, both on the consumer side and on the primary energy supply side. Once again, the question is being asked, which impact conventional power plants can have on Germany’s energy transition. In this work various energy system studies from the last 4 years are evaluated, covering a time span of 30 years. Summarized, most studies expect an increasing use of natural gas, peaking in the years between 2030 and 2035. While the overall gross electricity generation from conventional power plants decreases, the share of synthetic energy carries will increase from around zero until 2040 to 100% in 2050. Hereby the technologically openness of the used energy system model strongly influences the final installed capacities. While more open simulations lead to a median of about 50 GW in 2050, more restrictive ones expect roughly 30 GW. However, the full load hours will decrease significantly in both cases. Since the synthesis of synthetic energy carriers requires large amounts of renewable electricity, this development is linked to a national electricity demand of over 200 TWh for power-to-x applications and an additional import of 350 TWh of power-to-x products.
1 Introduction
With the EU Taxonomy Complementary Climate Delegated Act, the European Commission approved the addition of certain nuclear and gas technologies in the coming years to be labeled as investments directing to climate neutral energy systems. This can ultimately lead to an increased investment in natural gas-based technologies, including pure power generation, co-generation of heat and power as well as district heating/cooling (European Commission, 2022; Meager, 2022). In short term, a higher share of gas-powered plants can push coal-based plants from the energy market and hereby decrease the overall greenhouse gas emissions, as the act proposes an appropriate emission limit for fossil gas utilization. In the long term, however, this could make it more difficult to achieve a complete defossilization of the energy supply that is purely market-driven. Even before the discussion of EU taxonomy, energy system studies from the last years already considered natural gas as one of the most important transition technologies (Buttler and Spliethoff, 2016), neglecting influences such as pandemic situations and political crises. Using natural gas to start sustainability developments of energy systems in the ASEAN region has already been evaluated (Mohammad et al., 2021). Regarding the German energy system, current meta studies only focus on other aspects of the energy transition. Research exists on the use of biomass for decarbonization (Hahn et al., 2020), sector coupling (AEE e.V., 2017),(de-)centrality (acatech et al., 2020) or hydrogen usage (Wietschel et al., 2021), while literature lacks an evaluation of the use of conventional power plants as key technology of the energy system. In this work the impact of conventional power plants on the German energy transition, fueled by natural gas or a sustainable alternative, is analyzed by comparing recent system studies in a meta analysis.
2 Methods
Energy system studies are normally based on energy system optimization, as they are used for scientific or economic debates. Compared to energy system simulations, optimization models are best suited for forecasting possible future energy system portfolios under specific boundary conditions and assumptions (Lund et al., 2017). However, as stated by Grunwald, the use of just one study or even only one scenario can be misleading and the “meta-knowledge” of various studies should be used for decision making (Grunwald, 2011). Moreover, he states, the results are always influenced by the actual author and/or client sponsoring the study. Therefore, this work uses various studies to balance the impact of uncertainties or the client’s and author’s bias. In order to limit the selection of studies that can be used for the targeted evaluations, the following selection criteria are defined:
• Timeline: The considered horizon should extend at least to the year 2030, in the best case to 2050.
• Relevance: The publication date should be at least 2017.
• Reference area: Only studies that look at the development of the German energy system are considered; studies that only depict a part of Germany are excluded.
• Topic: The studies should at least cover the electricity sector. General system parameters such as greenhouse gas emissions, energy demand and other sectors or sector coupling topics are desired.
• Type of study: Only studies with quantitative results will be considered for the analyses; purely qualitative descriptions of system developments will not be included in the comparison.
• Client: Clients from different fields of operation should be represented.
The majority of the studies found consists of between two and four scenarios, while the maximum is ten. The clients range from purely scientific (e.g., Fraunhofer) or political (e.g., BMWi) institutions to interest groups (e.g., WWF) and large companies (e.g., Shell, RWE). The list of authors, on the other hand, consists mainly of scientific institutions or consulting firms. Methodologically, with more than 75%, most of the studies use optimization of the overall system costs to calculate the scenarios, which is equivalent to the most reasonable solution from an economic point of view. At the same time this also means that social and corporate interests can only be considered to a limited extent. Since these limitations are based on the boundary conditions and assumptions used, the evaluation of a large number of scenarios allows the identification of overall trends without neglecting upper and lower extremes. Nevertheless, the basis of the scenario definitions must be considered. Using the above list of criteria, the following set of 38 energy system studies with over 100 scenarios in total is found:
In general, the scenarios can be divided into clusters such as trend or reference scenarios, as well as ambitious and less ambitious scenarios. Here, the first ones form the basis on which changing boundary conditions can be discussed, also referred to as “business as usual” in case of trend scenarios. Therefore, studies usually consist of one trend/reference scenario and at least one or more ambitious or less ambitious scenarios. While the classification is single-choice, typical characteristics such as the technology openness of a system can be used to analyze explicit boundary conditions independent of scenarios. In this study, however, no differentiation between scenario types is used. Instead, a high number of scenarios including all kinds, trend, reference, ambitious and low ambitious environments are combined, to be able to discuss a broad variety of assumptions.
To evaluate the collected data, a graphical representation over the entire range of data points, the interquartile range (inner 50%) and the median are used. Most of the studies use the years 2020, 2030, 2040 and 2050 as support year, some have additional data points for the intermediate years of 2025, 2035 or 2045. As the graphical presentation equals a linear interpolation, in all cases of one missing data point (e.g., 2030 and 2040 is given, but not 2035) these interpolated values are used to improve the presentation. Nevertheless, there are various missing values, as they are not calculated or not explicitly given in the publications. Therefore, the number of scenarios for the support year with the fewest data points is given (nmin), as well as the total number of scenarios used (nsum). Additionally, the historic development from 1990 until 2020, based on the Eurostat energy database, is shown.
3 Current Situation
Conventional power plants can be divided into coal, natural gas (NG) and nuclear power plants, which all can be used both as power plants or as combined heat and power plants. Regarding the German energy system, coal based and nuclear power plants are planned to be shut down latest by 20381 or end of 20222, respectively. While the atomic energy act is based on safety concerns, the coal-phase out is purely motivated by greenhouse gas emissions. However, in 2021 the net power generation was covered by more than 40% by fossil and more than 10% by nuclear fired power stations Fraunhofer ISE (2022). Neglecting current discussions regarding a longer utilization of coal and nuclear power stations to reduce the high NG dependence of the German energy system, this study focuses more detailed on the use of gas-fired power plants in current energy system studies.
As main indicator, the gross electricity generation is used, to describe the trends in use of gas-fired conventional power plants, combining power and co-generation plants. The illustration in Figure 1 hereby is separated into:
(A) NG: Historic NG-based electricity generation and their corresponding future development, based on the energy system studies
(B) NG + SNG: Assumed electricity generation, based on all (conventional and sustainable) gas-fired power plants
(C) SNG: Electricity generation based on sustainable gaseous fuels
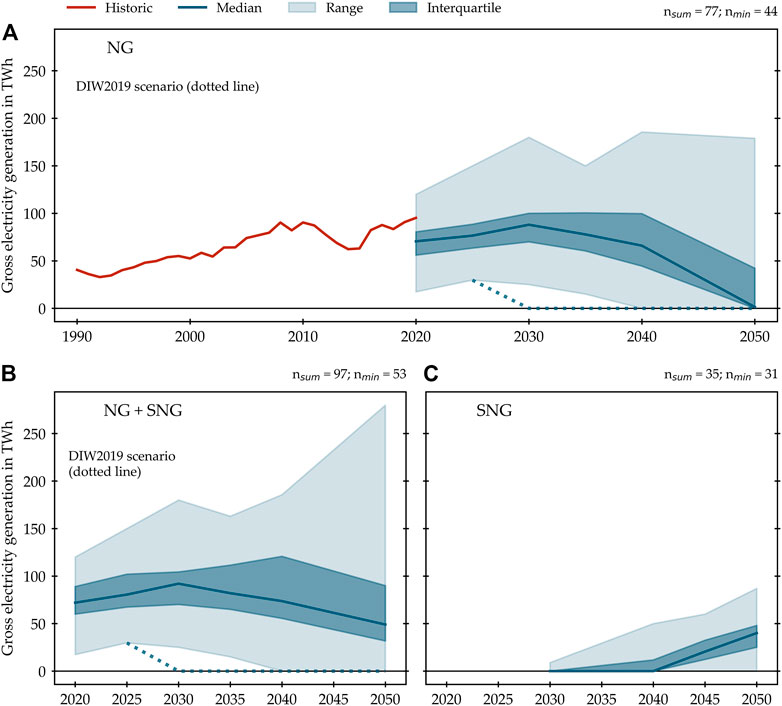
FIGURE 1. Gross electricity generation by gas-fired power plants based on natural gas including the historic development (A), overall generation (B) and use of sustainable energy carries (C).
First of all, the historic line in Figure 1A shows the growth in use of gas turbines in the early 2000s. The strong increase of renewable power generation, high NG prices and low CO2 emission allowance costs led to a decrease in the mid-2010s. Rising CO2 certificate prices, phase-out of nuclear power stations in Germany and an overall reduction of the hard coal utilization increased the demand on NG for power generation in Germany. Comparing the actual gross electricity generation of around 95 TWh in 2020 with the shown Median of 70 TWh, most of the studies underestimated the gas-based share. This higher share of NG in the German energy mix reflects the missed match with the targeted climate goals prior to 2020. The ultimate achievement of the emission targets in 2020 cannot be attributed to energy policy decisions, but rather to the combination of a mild winter and the subsequent pandemic situation. This is also confirmed by AGORA’s calculations, which show a significant increase and thus exceeding of the targets in 2021 and assume no relaxation for 2022 (Agora Energiewende, 2022).
4 Comparison of System Studies
Focusing on the system studies, a peak of NG usage between 2030 and 2040 can be identified (Figure 1A). At least 75% of the considered scenarios assume a maximum of 100 TWh of electricity generation via NG fired power plants. In contrast, in (Oei et al., 2019) one scenario already operates without NG in 2030, while all other scenarios require NG at least until 2040. However, most of the scenarios hold the zero-emission targets of Germany in 2050. At this point, it again must be referred to the widespread scenario structure. Looking at a reduced dataset by neglecting trend and “business as usual” scenarios, even the updated net-zero emissions target under the German climate protection act3 is largely achieved by 2045. In contrary, when using only trend and “business as usual” scenarios, the use of NG until beyond 2050 is not uncommon.
As in NG usage, the overall usage of NG and SNG peaks between 2030 and 2040 with a light shift towards 2040 (Figure 1B), what matches the expected global peak of NG demand (McKinsey and Company, 2021). More or less all studies consider gas-fired power plants to be necessary until at least 2050. While the overall NG utilization is assumed to end in 2050 (or 2045, respectively)4, an increase in the SNG demand can be observed (Figure 1C). As more gas power plants are installed in the mid-2020s, their availability until or beyond 2050 gives the energy system a flexibility option by simply synthesizing synthetic energy carriers as SNG to further use the power plants. As SNG production can be assumed as climate neutral by neglecting process-based emissions, this enables both achieving climate neutrality and security of supply.
The demand for synthetic energy carriers will increase not only due to the demand in power plants, but also due to other applications such as mobility and industry. As future energy systems will most likely be fully based on renewable power generation via photovoltaics and wind turbines, power-to-x technologies are assumed to cover a large share of this demand. If the median of the studies is used, an increase in the power-to-gas demand in Germany from 2.5 GW in 2030 to over 40 GW in 2050 is assumed. The corresponding electricity demand for all power-to-x applications adds up to over 200 TWh in 2050. This, however, is also expected to not cover the overall demand on power-to-x products, which will lead to an additional net import of synthetic energy carries of roughly 20 TWh in 2030 and more than 350 TWh in 2050. Nevertheless, most studies in principle would allow the import or use of natural gas. Therefore, more than 50% of the studies assume a natural gas price of between 25 and 35 €/MWh by 2050. Since the studies consider different approaches ranging from SNG synthesis, pure hydrogen-based gas turbines to blending hydrogen into the existing natural gas grid, it is not possible to analyze the respective production and import costs.
Looking deeper into the actual utilization of the installed capacities, key-performance-indicators like full load hours can be used to determine the mode of gas-fired power plant operation. Based on the actual 2020 data (Fraunhofer ISE, 2022), an average of 3,000 h a−1 can be calculated. Based on the medians of the installed capacities of the developments from the studies, as well as the respective energy quantities provided, average full-load hours of 3,030 h a−1, 2,180 h a−1 and 1,230 h a−1 emerge for the years 2030, 2040 and 2050 (Table 1). As the operating hours are distributed over several power plants, some of the units will be operated as base or mid-load power plants with up to 4,500 h a−1. The majority, however, will have fewer operating hours, covering only peak load or even being used only as back up, e.g., for black start events.

TABLE 1. Installed capacities and respective full load hours of gas fired power plants in future energy systems, depending of the restrictiveness of the energy system model.
Using a differentiation by the actual scenario definitions, two clusters can be identified. First scenarios with a more technologically open environment, and second, scenarios with more restrictive boundary conditions regarding the final technology portfolio. While both groups follow the mentioned trend during the energy transition peaking in 2030/2040, the actual medians of the installed capacities differ. In technologically open scenarios the median’s peak is at 53 GW, while the restrictive one is 39 GW. In summary, this implies that under the respective selected boundary conditions, cost-optimal energy systems would tend to use larger gas-fired power plant capacities, while at the same time meeting the emission targets. One reason is the possibility to reduce the required installed renewable energy technology and battery capacities by using SNG or equivalent energy carriers as a long-term storage.
Regarding the use of coal-fired power plants, almost all studies include the phase-out by 2038. Only individual studies still use coal-fired power plants to compare more ambitious and trend scenarios with strictly conservative ones. Nuclear power stations, on the other hand, basically are no longer a component of German energy system studies.
5 Conclusion
The evaluation of several current energy system studies on the subject of the energy transition in Germany and the associated need for conventional power plant capacities shows that gas-fired power plants in particular will play an important role. The demand will peak between 2030 and 2040, with natural gas still being used initially. In order to achieve the climate targets, however, most studies assume that power plants will continue to be operated, but using synthetic natural gas. Especially in view of the current discussions about designating investments in natural gas and nuclear power plants as green, these changing boundary conditions must be considered in future studies.
Since current system studies, respectively, the underlying model, partly still have limited possibilities of flexibility, new developments and technologies must always be implemented and added to the benchmark. For example, enabling bi-directional charging of electric cars could already lead to a reduction in the average flexibility requirement by conventional power plants. Alternative technologies such as reversible fuel cells, for example in direct coupling with wind farms, can also cover the flexibility requirement to a certain extent. Finally, a differentiated analysis of the synthetic energy carriers as well as the hydrogen integration into today’s natural gas system is necessary.
Author Contributions
AH: Writing original draft, data analysis and concept development, SF: Concept development and supervision, HS: Concept development and supervision. All authors reviewed the manuscript.
Funding
This study was carried out in the framework of the projects “VERENA” (project no.: 03EE5044B) sponsored by the Federal Ministry for Economic Affairs and Climate Action (Germany) and “Battle of the Studies - Update” (project no.: 441) supported by the VGB-FORSCHUNGSSTIFTUNG.
Conflict of Interest
The authors declare that the research was conducted in the absence of any commercial or financial relationships that could be construed as a potential conflict of interest.
Publisher’s Note
All claims expressed in this article are solely those of the authors and do not necessarily represent those of their affiliated organizations, or those of the publisher, the editors and the reviewers. Any product that may be evaluated in this article, or claim that may be made by its manufacturer, is not guaranteed or endorsed by the publisher.
Acknowledgments
Special thanks to Saskia Böck, Peggy Huang, and Philipp Räde for their assistance in researching and preparing the dataset. The financial support is gratefully acknowledged. The authors would also like to thank the Open Access Publishing Funding Program of the Technical University of Munich for their financial support.
Footnotes
1Law on the Reduction and End Coal-Fired Power Generation and further Amendments (Coal Phase-Out Act).
2Law on the Peaceful Use of Nuclear Energy and Protection against its Dangers (Atomic Energy Act).
3German climate protection act: Section 3 I KSG.
References
acatech, Leopoldina, Akademienunion (2020). Zentrale und dezentrale elemente im energiesystem: Der richtige mix für eine stabile und nachhaltige versorgung. Munich: acatech.
acatech, Leopoldina, Akademieunion (2017). Sektorkopplung - optionen für die nächste phase der energiewende. Munich: acatech.
AEE e.V. (2017). Metaanalyse: Zusammenspiel von strom- und wärmesektor für die energiewende. Available at: https://www.unendlich-viel-energie.de/media/file/3941.AEE_Metaanalyse_Strom_Waerme_jul17.pdf.
Agora Energiewende (2022). Die energiewende in deutschland: Stand der dinge 2021. rückblick auf die wesentlichen entwicklungen sowie ausblick auf 2022. Available at: https://www.agora-energiewende.de/veroeffentlichungen/die-energiewende-in-deutschland-stand-der-dinge-2021/.
Bareiß, K., Schönleber, K., and Hamacher, T. (2018). The Role of Hydrogen, Battery-Electric Vehicles and Heat as Flexibility Option in Future Energy Systems. Riga: EPE’18 ECCE Europe.
Bartholdsen, H.-K., Eidens, A., Löffler, K., Seehaus, F., Wejda, F., Burandt, T., et al. (2019). Pathways for Germany's Low-Carbon Energy Transformation towards 2050. Energies 12, 2988. doi:10.3390/en12152988
BEE e.V (2021). Das ”bee-szenario 2030”: 65 prozent treibhausgasminderung bis 2030 – ein szenario des bundesverbands erneuerbare energie. Berlin: Bee.
BEE e.V (2019). Das ”bee-szenario 2030”: 65% erneuerbare energien bis 2030 – ein szenario des bundesverbands erneuerbare energie e.v. Berlin: Bee.
BMU (2019). Projektionsbericht der bundesregierung 2019: Projektionsbericht 2019 für deutschland gemäßz verordnung (eu) nr. Available at: https://www.bmuv.de/download/projektionsbericht-der-bundesregierung-2019.
Brandstätt, C., Gabriel, J., Jahn, K., Peters, F., and Serkowsky, J. (2018). Innovation energiespeicher: Chancen der deutschen industrie. Study der Hans-Böckler-Stiftung 404. Düsseldorf: Hans-Böckler-Stiftung.
Burstedde, B., and Nicolosi, M. (2021). Strommarkt und klimaschutz: Transformation der stromerzeugung bis 2050: Abschlussbericht. Available at: https://www.baufachinformation.de/mobil/literatur/strommarkt-und-klimaschutz-transformation-der-stromerzeugung-bis-2050/2021079001065.
Buttler, A., and Spliethoff, H. (2016). Kampf der studien. Eine metaanalyse aktueller energiesystemstudien zum bedarf an speichern und konventionellen kraftwerken im kontext der annahmen und der historischen entwicklung. Available at: https://www.epe.ed.tum.de/fileadmin/w00bzo/es/pdf/Kampf_der_Studien_final.pdf.
Cao, K.-K., Pregger, T., Scholz, Y., Gils, H. C., Nienhaus, C., Deissenroth, M., et al. (2019). Analyse von strukturoptionen zur integration erneuerbarer energien in deutschland und europa unter berücksichtigung der versorgungssicherheit (inteever). Available at: https://elib.dlr.de/126264/.
Deutsche Energie-Agentur GmbH (2021). Dena-leitstudie Aufbruch Klimaneutralität: Zwischenbericht. Available at: https://www.dena.de/newsroom/publikationsdetailansicht/pub/zwischenbericht-dena-leitstudie-aufbruch-klimaneutralitaet/.
Deutsche Energie-Agentur GmbH (2018). Dena-leitstudie integrierte energiewende: Impulse für die gestaltung des energiesystems bis 2050. Available at: https://www.dena.de/fileadmin/dena/Dokumente/Pdf/9262_dena-Leitstudie_Integrierte_Energiewende_Ergebnisbericht.pdf.
Enervis Energy advisors GmbH (2020). Strommarktentwicklung und braunkohlebedarf unter der prämisse des braunkohleausstiegspfads. Berlin: GmbH.
Enervis Energy advisors GmbH (2021). Wasserstoffbasierte industrie in deutschland und europa: Potenziale und rahmenbedingungen für den wasserstoffbedarf und -ausbau sowie die preisentwicklungen für die industrie. Berlin: GmbH.
European Commission (2022). Commission Delegated Regulation (Eu). Available at: https://eur-lex.europa.eu/legal-content/EN/TXT/?uri=CELEX%3A32021R0236.
Fraunhofer ISE (2022). Energy-charts. Available at: https://energy-charts.info/?l=en&c=DE.
Fraunhofer ISI, Consentec GmbH, ifeu, TU, Wien, M-Five, TEP Energy GmbH (2017). Langfristszenarien für die transformation des energiesystems in deutschland. Available at: https://www.isi.fraunhofer.de/content/dam/isi/dokumente/ccx/2021/LFS_Kurzbericht.pdf.
Gerbert, P., Herhold, P., Burchardt, J., and Schönberger, S. (2018). Klimapfade für deutschland. Available at: https://bdi.eu/publikation/news/klimapfade-fuer-deutschland/.
Grunwald, A. (2011). Energy Futures: Diversity and the Need for Assessment. Futures 43, 820–830. doi:10.1016/j.futures.2011.05.024
Günther, J., Lehmann, H., Nuss, P., and Purr, K. (2019). Wege in eine ressourcenschonende treibhausgasneutralität. Available at: https://www.umweltbundesamt.de/rescue.
Hahn, A., Szarka, N., and Thrän, D. (2020). German Energy and Decarbonization Scenarios: "Blind Spots" with Respect to Biomass-Based Carbon Removal Options. Front. Energy Res. 8. doi:10.3389/fenrg.2020.00130
Hecking, H., Hintermayer, M., Lencz, D., and Wagner, J. (2017). Energiemarkt 2030 und 2050 – der beitrag von gas- und wärmeinfrastruktur zu einer effizienten co2-minderung. Cologne: Endbericht.
Hermann, H., Loreck, C., Ritter, D., Greiner, B., Keimeyer, F., Cook, V., et al. (2017). Klimaschutz im stromsektor 2030 - vergleich von instrumenten zur emissionsminderung. Available at: https://www.umweltbundesamt.de/sites/default/files/medien/1/publikationen/2017-01-11_cc_02-2017_strommarkt_endbericht.pdf.
KITÖ, ko-Institut, e.V., Deutsche Umwelthilfe, RWTH Aachen, and ewi (2020). Transformation des energiesystems bis zum jahr 2030. Available at: https://www.oeko.de/fileadmin/oekodoc/ENSURE-Broschuere-Storylines.pdf.
Klein, S., Steinert, T., Fricke, A., and Peschel, D. (2017). Erneuerbare gase - ein systemupdate der energiewende. Available at: https://www.wind-energie.de/presse/pressemitteilungen/detail/erneuerbare-gase-ein-systemupdate-der-energiewende/.
Lund, H., Arler, F., Østergaard, P., Hvelplund, F., Connolly, D., Mathiesen, B., et al. (2017). Simulation versus Optimisation: Theoretical Positions in Energy System Modelling. Energies 10, 840. doi:10.3390/en10070840
Matthes, F. C., Emele, L., Hermann, H., Loreck, C., Peter, F., Ziegenhagen, I., et al. (2017). Zukunft Stromsystem - Kohleausstieg 2035: Vom Ziel Her Denken. Available at: https://www.oeko.de/fileadmin/oekodoc/Stromsystem-Kohleausstieg-2035.pdf.
Matthes, F. C., Flachsbarth, F., Loreck, C., Hermann, H., Falkenberg, H., and Cook, V. (2019). Zukunft stromsystem ii: Regionalisierung der erneuerbaren stromerzeugung. Available at: https://www.oeko.de/fileadmin/oekodoc/Stromsystem-II-Regionalisierung-der-erneuerbaren-Stromerzeugung.pdf.
Max, G., Dominic, L., and Fabian, A. (2019). Auswirkungen einer beendigung der kohleverstromung bis 2038 auf den strommarkt, co2-emissionen und ausgewählte industrien. Available at: https://www.ewi.uni-koeln.de/cms/wp-content/uploads/2019/08/EWI-Studie_Auswirkungen-Kohleausstieg-bis-2038_20200515.pdf.
McKinsey and Company (2021). Global Energy Perspective 2021. Available at: https://www.mckinsey.com/∼/media/McKinsey/Industries/Oil%20and%20Gas/Our%20Insights/Global%20Energy%20Perspective%202021/Global-Energy-Perspective-2021-final.pdf.
Meager, E. (2022). What the Inclusion of Gas and Nuclear in the Eu Taxonomy Means. Capital Monitor. Available at: https://capitalmonitor.ai/factor/environmental/what-the-inclusion-of-gas-and-nuclear-in-the-eu-taxonomy-means/.
Mohammad, N., Mohamad Ishak, W. W., Mustapa, S. I., and Ayodele, B. V. (2021). Natural Gas as a Key Alternative Energy Source in Sustainable Renewable Energy Transition: A Mini Review. Front. Energy Res. 9. doi:10.3389/fenrg.2021.625023
Nitsch, J. (2019). Noch Ist Erfolgreicher Klimaschutz Möglich. Available at: https://co2abgabe.de/wp-content/uploads/2019/06/Nitsch_2019_Noch_ist_Klimaschutz_moeglich_LF.pdf.
Oei, P.-Y., Göke, L., Kemfert, C., Kendziorski, M., and von Hirschhausen, C. (2019). Erneuerbare energien als schlüssel für das erreichen der klimaschutzziele im stromsektor. Berlin DIW Berlin: Politikberatung kompakt.
Öko-Institut e.V., and Fraunhofer ISI (2020). Verbesserung der methodischen grundlagen und erstellung eines treibhausgasemissionsszenarios als grundlage für den projektionsbericht 2017 im rahmen des eu-treibhausgasmonitorings (”politikszenarien viii”). Available at: https://www.umweltbundesamt.de/publikationen/verbesserung-der-methodischen-grundlagen-erstellung.
Öko-Institut e.V, and Jacobs University Bremen gGmbH, and eclareon GmbH (2019). Modellbasierte szenarienuntersuchung der entwicklungen im deutschen stromsystem unter berücksichtigung des europäischen kontexts bis 2050. Available at: https://www.oeko.de/publikationen/p-details/modellbasierte-szenarienuntersuchung-der-entwicklungen-im-deutschen-stromsystem-unter-beruecksichtigu.
Prognos, Fraunhofer ISI, IINAS, and GWS (2021a). Energiewirtschaftliche projektionen und folgeabschätzungen 2030/2050. Basel: Gesamtdokumentation der szenarien.
Prognos, Öko-Institut e.V., and Wuppertal-Institut (2021b). Klimaneutrales deutschland 2045: Wie deutschland seine klimaziele schon vor 2050 erreichen kann. Available at: https://www.agora-verkehrswende.de/veroeffentlichungen/klimaneutrales-deutschland-2045-langfassung/.
Prognos, Öko-Institut e.V., and Wuppertal-Institut (2020). Klimaneutrales deutschland: Studie im auftrag von agora energiewende, agora verkehrswende und stiftung klimaneutralität. Available at: https://www.agora-verkehrswende.de/veroeffentlichungen/klimaneutrales-deutschland-langfassung/.
Repenning, J., Emele, L., Blanck, R., Cook, V., Harthan, R., Hermann, H., et al. (2018). Politikszenarien für den klimaschutz vii. treibhausgas-emissionsszenarien bis zum jahr 2035. Available at: https://www.umweltbundesamt.de/publikationen/politikszenarien-fuer-den-klimaschutz-vii.
Robinius, M., Markewitz, P., Lopion, P., Kullmann, F., Heuser, P.-M., Syranidis, K., et al. (2020). Wege für die energiewende: Kosteneffiziente und klimagerechte transformationsstrategien für das deutsche energiesystem bis zum jahr 2050. Jülich: Energie und Umwelt.
Schimek, F., Nauhauser, T., Robinius, M., Stolten, D., and Hille, C. (2020). Wasserstoffstudie: Chancen, potentiale and herausforderungen im globalen energiesystem. Available at: https://www.umlaut.com/uploads/documents/2020_umlaut_wasserstoff-studie_2020-10-15-072559.pdf.
Sterchele, P., Brandes, J., Heilig, J., Wrede, D., Kost, C., Schlegl, T., et al. (2020). Wege zu einem klimaneutralen energiesystem. Available at: https://www.ise.fraunhofer.de/de/veroeffentlichungen/studien/wege-zu-einem-klimaneutralen-energiesystem.html.
Übertragungsnetzbetreiber (2019). Netzentwicklungsplan Strom 2030. version 2019. Available at: https://www.netzentwicklungsplan.de/de/netzentwicklungsplaene/netzentwicklungsplan-2030-2019.
Übertragungsnetzbetreiber (2021). Netzentwicklungsplan Strom 2035. version 2021. Erster entwurf der Übertragungsnetzbetreiber.
Wietschel, M., Zheng, L., Arens, M., Hebling, C., Ranzmeyer, O., Schaadt, A., et al. (2021). Metastudie wasserstoff - auswertung von energiesystemstudien: Studie im auftrag des nationalen wasserstoffrats. Available at: https://www.wasserstoffrat.de/fileadmin/wasserstoffrat/media/Dokumente/Metastudie_Wasserstoff-Abschlussbericht.pdf.
Keywords: energy system studies, meta analysis, conventional power plants, energy transition (Energiewende), synthetic energy carriers
Citation: Hanel A, Fendt S and Spliethoff H (2022) Operation of Conventional Power Plants During the German Energy Transition: A Mini Review. Front. Energy Res. 10:907251. doi: 10.3389/fenrg.2022.907251
Received: 29 March 2022; Accepted: 09 May 2022;
Published: 14 June 2022.
Edited by:
Matthias Huber, Deggendorf Institute of Technology, GermanyReviewed by:
Hadi Farabi-Asl, Research Institute for Humanity and Nature, JapanCopyright © 2022 Hanel, Fendt and Spliethoff. This is an open-access article distributed under the terms of the Creative Commons Attribution License (CC BY). The use, distribution or reproduction in other forums is permitted, provided the original author(s) and the copyright owner(s) are credited and that the original publication in this journal is cited, in accordance with accepted academic practice. No use, distribution or reproduction is permitted which does not comply with these terms.
*Correspondence: Andreas Hanel, andreas.hanel@tum.de; Sebastian Fendt, sebastian.fendt@tum.de