- 1Boone Pickens School of Geology, Oklahoma State University, Stillwater, OK, United States
- 2Southern Company Services, Birmingham, AL, United States
- 3Advanced Resources International, Arlington, VA, United States
The Cretaceous and Tertiary deposits in Mississippi, Alabama, and the adjacent continental shelf constitute a widespread succession of sandstone, mudstone, and carbonate that has proven to be an important target for geologic CO2 storage in the onshore Gulf of Mexico basin. Integrated analysis of stratigraphy, sedimentology, and reservoir properties based on cores and geophysical well logs indicates that the Paluxy Formation and Washita- Fredericksburg interval present gigatonne-class storage opportunities. The distribution, geometry, and composition of the area is a direct reflection of the original depositional environments, and understanding these factors is essential for understanding the geologic storage potential of the Paluxy Formation and Washita-Fredericksburg interval at the Kemper County energy facility in Mississippi. Geologic characterization of the Mississippi Embayment at the energy facility focused primarily on characterizing the confinement potential of the storage complex. Integration of core analyses and geophysical well logs has yielded a high-resolution stratigraphic analysis of storage reservoirs, baffles, barriers, and seals. Scanning electron microscopy (SEM) coupled with energy dispersive X-ray spectroscopy (EDS), and quantitative X-ray diffraction (XRD) was used to characterize microfabric, pore types, and mineralogy within mudstone of the east-central Mississippi Embayment at the Kemper County energy facility. Mudstone beds in the Paluxy Formation and Washita-Fredericksburg interval have variable thickness and continuity. High water saturation in the Cretaceous mudstone units influences swelling smectite clay and mudrock permeability based on pulse decay analysis is 1–96 nD. These low permeability values indicate that the mudstone units are effective baffles, barriers, and confining intervals that make significant migration of injected CO2 out of the storage complex unlikely. The numerous baffles and barriers within the target reservoir intervals, moreover, favor the retention of multiple CO2 in plumes within the abundant stacked sandstone bodies.
1 Introduction
This project explored the confining potential of candidate geologic CO2 sinks in Lower Cretaceous strata in the Gulf of Mexico Basin in east-central Mississippi at the Kemper County energy facility, which is being developed by Southern Company (Figure 1). This research is sponsored by the Southern States Energy Board (SSEB) through the National Energy Laboratory of the U.S. Department of Energy’s (DOE/NETL) CarbonSAFE program (Esposito et al., 2017; Riestenberg et al., 2019; Pashin et al., 2020). Plant Ratcliffe, also known as the Kemper County energy facility, was designed to include a large Integrated Gasification Combined Cycle (IGCC) coal plant (Reitze, 2012) and utilize a post combustion carbon capture unit, but because of technical, regulatory, and market conditions is operating as a combined cycle natural gas power plant.
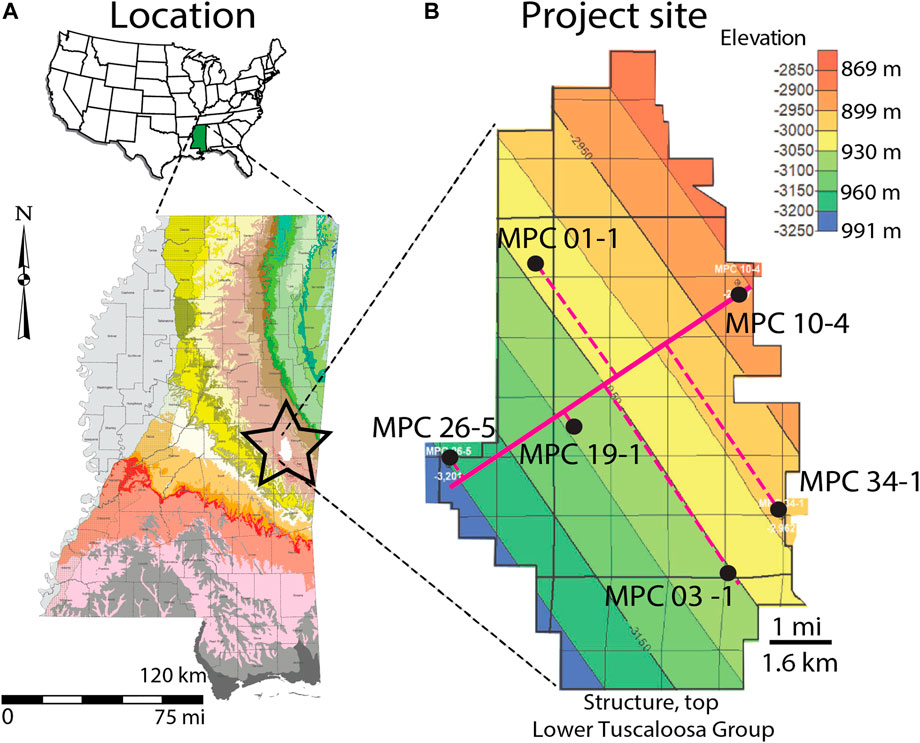
FIGURE 1. Location of study area. (A) Map showing the Kemper County energy facility in east-central Mississippi. (B) Structural contour map of the top of the lower Tuscaloosa Group showing locations of six exploratory boreholes and line of cross section (after Pashin et al., 2021).
Reservoir strata under the energy facility house a gigatonne (Gt)-scale storage resource (1.4 Gt at 14% storage efficiency using the method of Goodman et al., 2011) (Urban, 2020) and have the potential to host a regional storage hub for anthropogenic CO2 emissions (Pashin et al., 2020). The facility is highly flexible, able to receive large volumes of CO2 from local and regional sources and support a range of injection programs. The reservoirs are in multiple stacked sandstone units with geometric mean horizontal permeability of 3.9 Darcies; they have been interpreted as bedload-dominated fluvial deposits (Pashin et al., 2020; Urban, 2020). The bulk of the storage resource is in the Lower Cretaceous Paluxy Formation and Washita-Fredericksburg interval; numerous mudstone units define baffles and barriers separating the stacked sandstone bodies. The distribution, geometry, and composition of the mudstone units in the Paluxy Formation and Washita-Fredericksburg interval is hypothesized to be a product of the original depositional framework, and understanding these factors is essential for planning injection programs and determining the fate of CO2 injected in the target sandstone units, which are confined by the mudstone layers. Indeed, the heterogeneity in the thickness and continuity of the mudstone is considered a fundamental control on where injected CO2 plumes will migrate and where hydraulic communication may exist among the stacked sandstone bodies.
Accordingly, the principal objectives of this research are to characterize Paluxy and Washita-Fredericksburg mudstone through stratigraphic analysis, sedimentologic analysis, and petrologic analysis. A classification of intraformational mudstone units was used that is based on the thickness and continuity of mudstone units (Wethington, 2020). Baffles locally confine CO2, are on the order of 0.3–3 m (1–10 ft), and can be identified in geophysical well logs. Barriers are thicker, on the order of 3–30 m (10–100 ft), and are more likely to impede vertical CO2 migration based on greater thickness and lateral extent. The baffles and barriers are fine-grained, clay-rich, have low carbonate content, and are characterized by variable thickness and continuity. Mudstone units within subsurface reservoirs are a key source of depositional and reservoir heterogeneity and affect storage efficiency, reservoir extent, and plume morphology (Downey, 1994; Neuzil, 1994; Doughty and Pruess, 2004; Bachu et al., 2007; Goodman et al., 2011; Cavanagh and Haszeldine, 2014). The stacked reservoirs at the Kemper County energy facility are hypothesized to support lateral plume growth over cross-formational flow, and the high permeability helps limit pressure buildup during injection. The Kemper County energy facility is situated atop a world-class CO2 storage complex and represents an important target for CO2 sequestration in the United States that has exceptional potential for generational learning and transfer of concepts and technology to other sites.
2 Geologic Setting
The Kemper County energy facility is in the eastern part of the Mississippi Embayment in east-central Mississippi, which began forming during Proterozoic-Cambrian Iapetan rifting (Thomas, 1991) and Mesozoic-Cenozoic extension associated with the opening of the Gulf of Mexico (Salvador, 1987). Post-Paleozoic strata dip regionally southwest above a sub-Mesozoic angular unconformity (Cushing et al., 1964; Thomas, 1988; Pashin et al., 2008). The main storage targets are in Cretaceous deposits, and major sealing strata are developed in the Upper Cretaceous-Paleocene section of the Selma Group and the Porters Creek Clay. Underground sources of drinking water that need to be protected are in Paleogene strata of the Naheoloa and Nanafalia Formations. The Mississippi Embayment extends from southern Illinois to the Gulf of Mexico coastal plain in Louisiana, Mississippi, and Alabama; it lies west of the Nashville Dome and east of the Ozark Dome (Kolata et al., 1981; Abert, et al., 2016). Sediment fill in the embayment ranges in thickness from a feather-edge along the fringe of the embayment and thickens toward the south-southwest. Regional dip above the Paleozoic disconformity is <1° (Stearns and Marcher, 1962; Pashin et al., 2008).
Cretaceous-Paleogene strata are preserved below the Kemper County energy facility and overlie an angular unconformity atop deformed Paleozoic strata of the Appalachian-Ouachita Orogen (Thomas, 1973; Thomas, 1985; Thomas, 1991; Hale-Ehrlich and Coleman, 1993; Pashin et al., 2008). The upper 38 m (125 ft) of the Paleozoic section has been penetrated by wells at the energy facility. Subhorizontal Lower Cretaceous strata overlie the angular unconformity and constitute a thick succession dominated by siliciclastic deposits. These strata are assigned to the Mooringsport Formation, the Paluxy Formation, and the Washita-Fredericksburg interval (Pashin et al., 2008; Pashin et al., 2020). The Lower Cretaceous strata are restricted to the subsurface and are confined regionally by convergence of the sub-Mesozoic disconformity with the mudstone near the top of Washita-Fredericksburg interval. Upper Cretaceous strata of the Tuscaloosa Group disconformably overlie the Washita-Fredericksburg interval and contain basal conglomerate units that grade upward into poorly consolidated sand. These conglomerate and sandstone units make up the Massive sand of the lower Tuscaloosa Group. The overlying Marine Tuscaloosa shale is dominated by mudstone and claystone. The upper Tuscaloosa Group contains interbedded sandstone and mudstone. The Eutaw Formation disconformably overlies the Tuscaloosa Group and contains interbedded glauconitic sandstone and mudstone (Pashin et al., 2008). About 275 m (900 ft) of chalk assigned to the Selma Group caps the Upper Cretaceous section and is disconformably overlain by Paleocene-Eocene strata assigned to the Clayton Formation, Porters Creek Clay, Naheola Formation, and Nanafalia Formation (Rainwater, 1961; Wethington, 2020). Together, the Selma Group and Porters Creek Clay form a thick (365 m; 1,200 ft) sealing section that defines the top of the CO2 storage complex at the Kemper County energy facility. The Nanafalia Formation is the youngest unit in the study area and is included in the Wilcox Group (Paleocene-Eocene) (Bicker, 1969). The base of the Nanafalia Formation is a thick, fresh water-bearing sand called the Nanafalia Sand, which is the principal underground source of drinking water (USDW) in the study area (Figure 2). Surface and near-surface deposits in the area of the Kemper energy facility are sand, clay, and lignite.
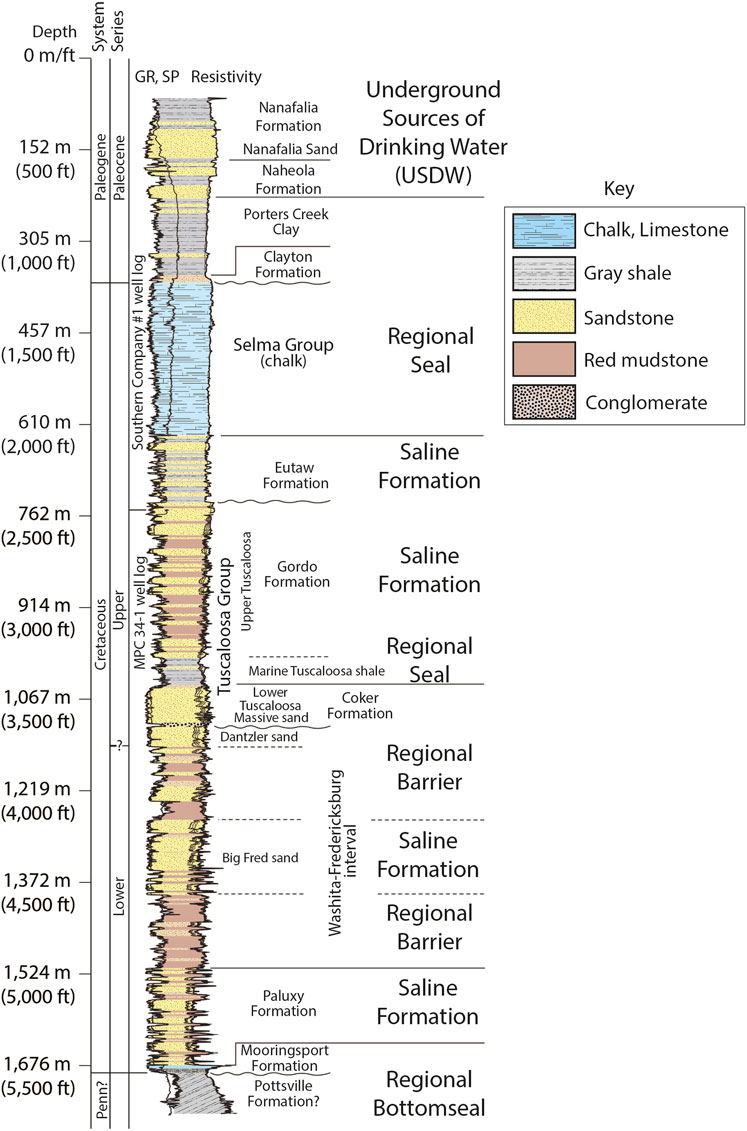
FIGURE 2. Composite stratigraphic section and geophysical well log of the Paleozoic-Mesozoic section at the Kemper County energy facility (after Pashin et al., 2020).
3 Materials and Methods
Data were collected from six wells and selected cored intervals of the Cretaceous section in the MPC 26-5, MPC 34-1, MPC 10-4, MPC 19-1, and MPC 01-1 wells. Seventeen cores were recovered spanning 100.5 m (330 ft) from the Paluxy Formation, the Washita-Fredericksburg interval, and the Tuscaloosa Group. The rocks were analyzed with respect to lithofacies and stratigraphic position. Lithofacies were defined and characterized based on rock type, texture, bedding, physical sedimentary structures, biogenic structures, and mineralogy.
The wells include a diverse suite of geophysical logs, including gamma ray, resistivity, density-neutron porosity, and spontaneous potential curves. Cores from the MPC 34-1 and MPC 10-4 cores were CT scanned in the original aluminum core sleeves prior to slabbing. Samples from the butt slabs of the cores were taken for thin sections, SEM billets, and bulk sampling for X-ray diffraction for mudstone samples from the MPC 26-5, MPC 34-1, and MPC 10-4 wells. Sample billets were ion milled for SEM/EDS analysis, and samples were crushed for semi-quantitative XRD analysis. Samples from the MPC 10-4 well were analyzed for total organic carbon content in gray mudstone in the Paluxy Formation.
Cores were described, photographed, and logged using standard stratigraphic and sedimentologic procedures. Graphic logs were constructed to document rock types, bedding contacts, sedimentary structures, and biological features. Grain size was characterized using the Wentworth scale (Wentworth, 1922). Intensity of bioturbation in CT images of the cores was characterized using the Bann bioturbation intensity index (MacEachern and Bann, 2008), and rock color was defined using the GSA Munsell color index (Munsell Color, 2009). Depositional environments were interpreted on the basis of rock types, grain size trends, bedding, sedimentary structures, fossils, and facies relationships. Samples of fine-grained rocks were taken from cores of the Paluxy Formation, Washita-Fredericksburg interval, and the Marine Tuscaloosa shale, and thin sections were milled to a thickness of 20 µm. The thin sections were vacuum impregnated with blue epoxy and stained with alizarin red and sodium cobaltinitrite to identify carbonate and feldspar. Descriptions and photography were done using a Leica DM EP and Olympus BX51 petrographic microscope. The grain size distribution in the mudstone in each thin section was used to build a microfacies description that includes clay, quartz, feldspar, accessory grains, cement, fabric, and porosity. To estimate the volume of clay and detrital grains, the comparison charts of Matthew et al. (1991) were used. Grain size percentages were then plotted in a ternary diagram (Picard, 1971) to characterize rock texture and determine facies characteristics.
Scanning electron microscopy (SEM) was performed on 12 mudstone samples using a FEI Quanta 600 FEG MK2 Environmental SEM. The samples were polished with a JEOL model IB-19500CP argon ion mill and plated with a gold-palladium alloy prior to imaging. The SEM is accompanied by a Bruker Quantax XFlash 6/60 unit with Quantax Esprit software for energy dispersive X-ray spectroscopy (EDS) analysis, which aided in mineral identification. The SEM samples have a corresponding thin sections that enable comparison of the features observed under transmitted light with those observed by SEM and EDS. Compositional data were used to supplement thin section descriptions and characterization of mineralogy, micro fabric/texture, and pore systems were plotted in a pore system ternary diagram (Loucks et al., 2012).
Semi-quantitative x-ray diffraction (XRD) data were gathered for two samples (Table 1) from the MPC 34-1 and MPC 10-4 wells from the Paluxy Formation the samples correspond to thin sections and SEM images. XRD analysis was performed to determine the mineralogy of the mudrocks with respect to weight percent, including clay species. The samples were extracted from butt slabs of the cores, ground with a ceramic mortar and pestle, and then dried in glass dishes inside a Lab Companion scientific oven for 72 h at a temperature of 40°C. The samples were shipped to Impac Exploration Services, and minerals were identified from the pattern of XRD peaks, and the percentage of each mineral was estimated by Rietveld analysis using the methods of Moore and Reynolds (1989).

TABLE 1. X-ray diffraction mineralogy of mudstone from the Paluxy Formation. Facies key: HM = Heterolithic mudstone and MM = Massive mudstone.
Total carbon (TC) and sulfur content were determined for one sample from well MPC 10-4 from the Paluxy Formation (Table 2). The sample was selected based on the availability of associated thin section, SEM, and XRD data. The sample was crushed with a ceramic mortar and pestle and dried in glass dishes inside a Lab Companion scientific oven for 96 h at a temperature of 45°C then re-crushed and dried for 48 h at a temperature of 45°C. Sample size was greater than 100 mg and analyzed in an Eltra Carbon-Sulfur Determinator with two repeats. A standard sample was used for calibration before and after measuring each sample. The carbon standard TC4007 sample, which is certified at 7.3% TOC, was used for standardization and calibration. Testing of the carbon standard established a maximum error of 0.23%. TC was determined by combustion and total inorganic carbon (TIC) was measured by acidizing the samples to dissolve carbonate. Total organic content (TOC) was then calculated by subtracting TIC from TC.
Pulse-decay permeability testing of core plugs obtained from Lower Cretaceous mudstone in the MPC-19-1 well was performed by the Stratum core laboratory. The plugs were drilled from butt slabs of the core. The testing was performed with air under a net confining stress of 10.3 MPa (1,500 psig). The core plugs have a diameter of 3.8 cm and length ranging between 3.2 and 4.5 cm and were placed in screened nickel-teflon sleeves for testing. The testing was performed on the samples on an as-received basis because high water content and fluid sensitivity precluded cleaning or drying prior to analysis.
4 Results
4.1 Stratigraphic Cross Section
The composite cross section based on the six well logs demonstrates complex spatial relationships among the mudstone and sandstone units and the nature of stratigraphic boundaries in the study interval (Figure 3). Correlations are dependent on stratigraphic position relative to a datum at the top of the lower Tuscaloosa Group, log curves, and depositional architecture. The sandstone and mudstone units have variable thickness and lateral extent throughout the cross section, with sandstone units clustered in the Paluxy Formation and the Big Fred sand of the Washita-Fredericksburg interval. Facies relationships are complex in the Paluxy Formation, where the basal sandstone units onlap Mooringsport limestone toward the northeast, and shallower sandstone units have variable thickness and geometry ranging from lensoid and tabular to continuous. The mudstone units are also geometrically complex, filling the gaps between the sandstone bodies. The Washita-Fredericksburg interval contains the greatest concentration and continuity of sandstone and conversely the least amount of mudstone in the middle of the interval, specifically the Big Fred sand, whereas the lower and upper parts of the interval are dominated by mudstone containing lensoid sandstone units with variable thickness and continuity. The Dantzler sand forms the top of the Washita-Fredericksburg interval and has undulatory basal and upper contacts. The Dantzler is disconformably overlain by the Massive sand of the lower Tuscaloosa Group, which has an undulatory basal contact and a relatively smooth upper contact, which was used as a datum to build the cross section.
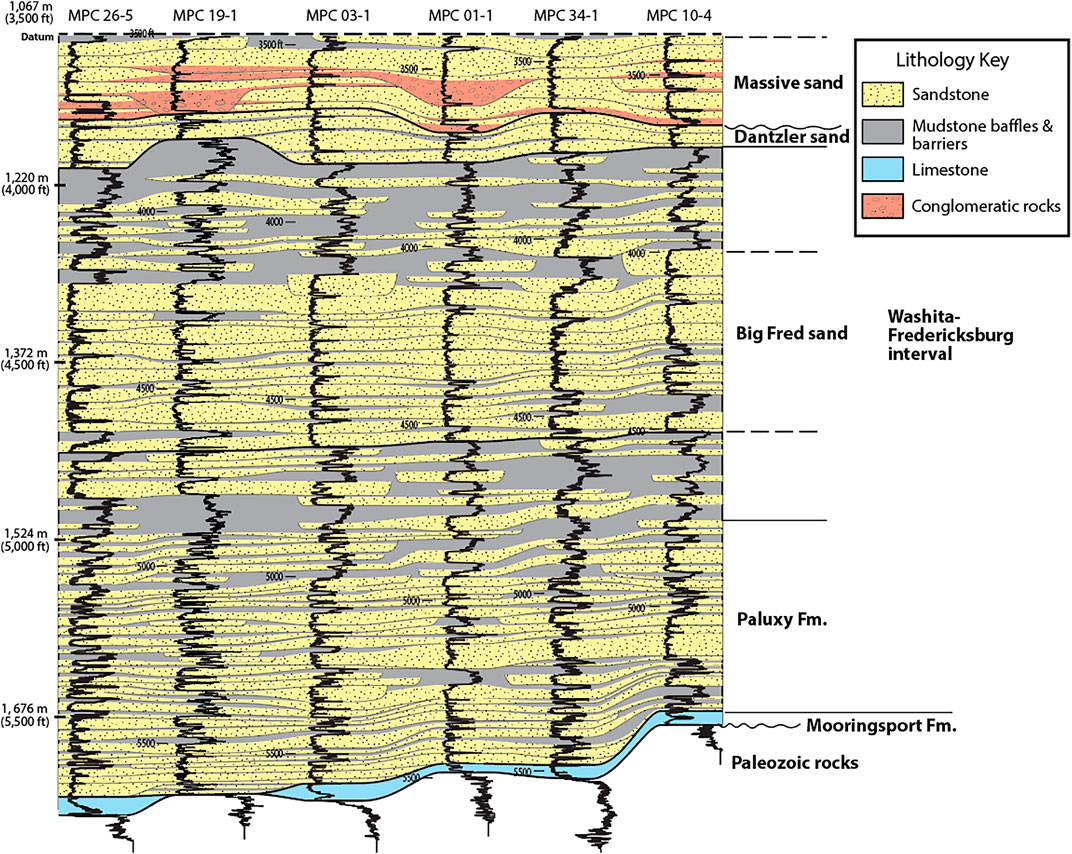
FIGURE 3. Stratigraphic cross section showing correlation of Paluxy and Washita-Fredericksburg strata using gamma logs (after Pashin et al., 2021).
The Paluxy Formation contains approximately 18 small-scale mudstone baffles per well that are on the scale of 0.3 m (1 ft) thick. Five of the baffles were correlated among most or all of the wells. There are approximately 3 mudstone barriers per well in the Paluxy Formation that are 3–10 m (10–30 ft) thick and can be correlated across the study area. The Washita-Fredericksburg interval contains 18 small-scale baffles per well with one correlatable mudstone package in the upper Big Fred sand and a moderately correlatable baffle in the upper Washita-Fredericksburg interval. There are 11 thicker mudstone barriers (>3 m; 10 ft) within the Washita-Fredericksburg interval; 8 of the barriers can be considered moderate due to incomplete lateral continuity and occurring at the same stratigraphic and structural interval between multiple wells. The lower Washita-Fredericksburg interval contains 4 barriers, the Big Fred sand contains 1, and 3 within the upper Washita-Fredericksburg interval. There is an occurrence of very thick baffle (>30 m; 100 ft) in the upper Washita-Fredericksburg interval in the downdip MPC 26-5 well, but does not maintain thickness across the study area and correlates with thinner barriers updip. In general, the Paluxy Formation mudstone units are thin, have limited continuity, and are thus considered baffles that contribute to reservoir heterogeneity. Basal Washita-Fredericksburg mudstone units are generally thicker (>3 m), more numerous, and maintain more uniform thickness in the study area. The Big Fred sand within the Washita-Fredericksburg interval contains mainly thin mudstone layers, and the baffles may be discontinuous and are capped by a series of thicker and more widespread barriers in the upper Washita-Fredericksburg interval.
4.2 Mudstone Lithofacies
Mudstone in the Paluxy Formation and Washita-Fredericksburg interval is typically sandy, contains diverse sedimentary, biogenic, pedogenic, and authigenic structures. The mudstone was pliable at the time of core recovery and remains highly reactive with water. This section describes the characteristics of individual mudstone facies recognized in the cores and lithofacies naming is based on hand sample features.
About 2.7 m (9 ft) of the variegated mudstone facies was cored in the Paluxy Formation in well MPC 34-1 at a depth of 1,620.3–1,623 m (Figure 4A). This facies appears to be the most typical component of the lower Cretaceous mudstone units at the Kemper County energy facility. The mudstone in this core is between two fining-upward sandstone units; it has a gradational lower contact and a sharp upper contact. The facies is texturally a sandy mudstone to clayey sandstone. It is characterized by variegated light bluish gray, with moderate yellow, and mixed grayish and moderate red colors throughout. Sedimentary structures include branching rootlets, desiccation cracks, and horizontal and vertical burrow networks that give this facies a mottled texture. Irregular sesquioxide nodules that are up to cobble size are common and weather with yellowish hues. Many of the nodules are not visible in hand sample but are readily observed in CT images because of high iron concentration, which increases rock density. Burrow mottling and adhesive meniscate burrows are common in this facies, and the bioturbation index is typically 3 (Figure 5A).
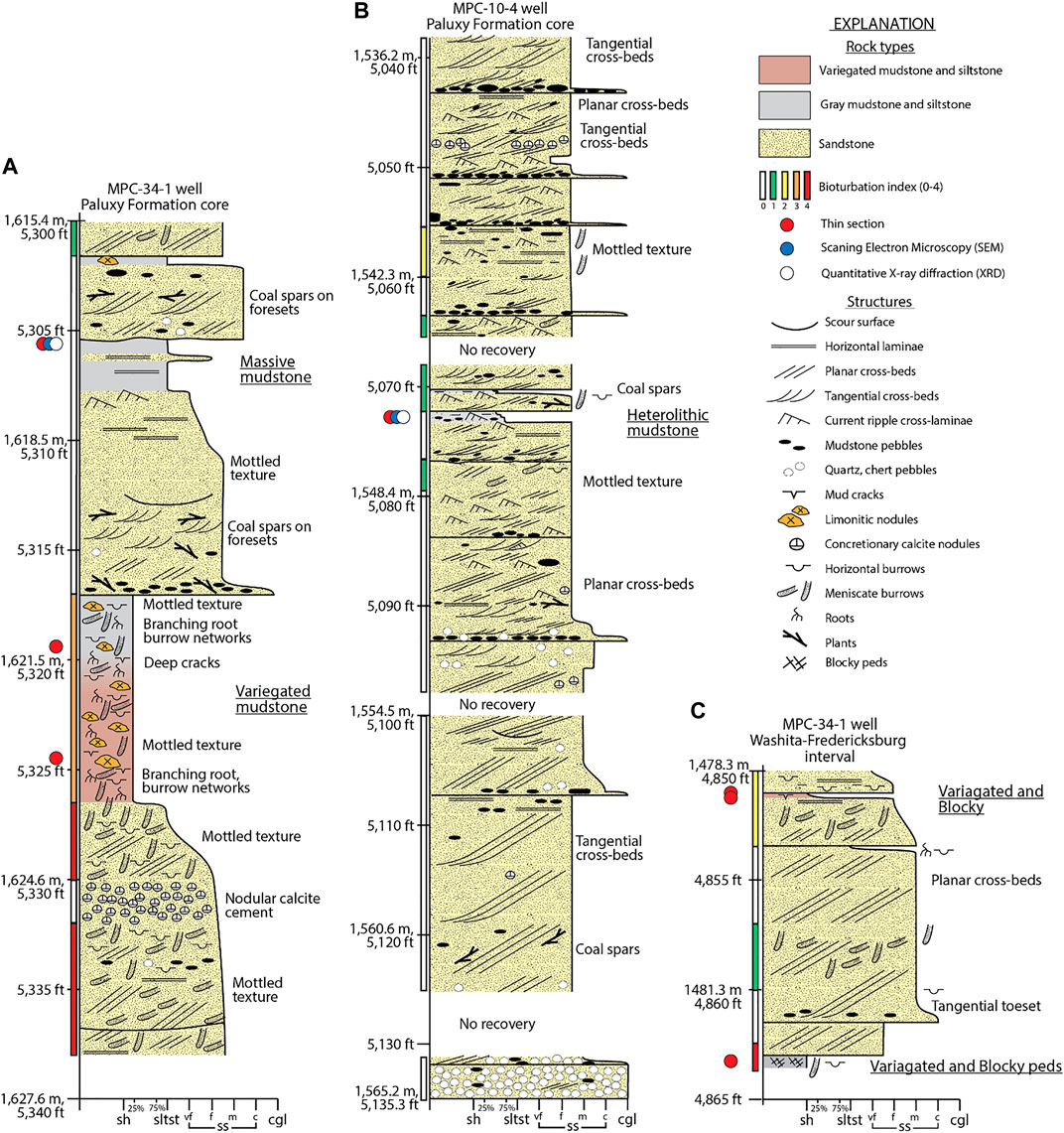
FIGURE 4. Graphic core logs from the Kemper County energy facility showing rock types, sedimentary structures, biogenic structures, diagenetic structures, and bioturbation index (modified from Pashin et al., 2020) (A) Graphic core log of the Paluxy Formation in the MPC 34-1 well. (B) Graphic core logs of the Paluxy Formation in the MPC 10-4 well. (C) Graphic core log of the Washita-Fredericksburg interval in the MPC 34-1 well.
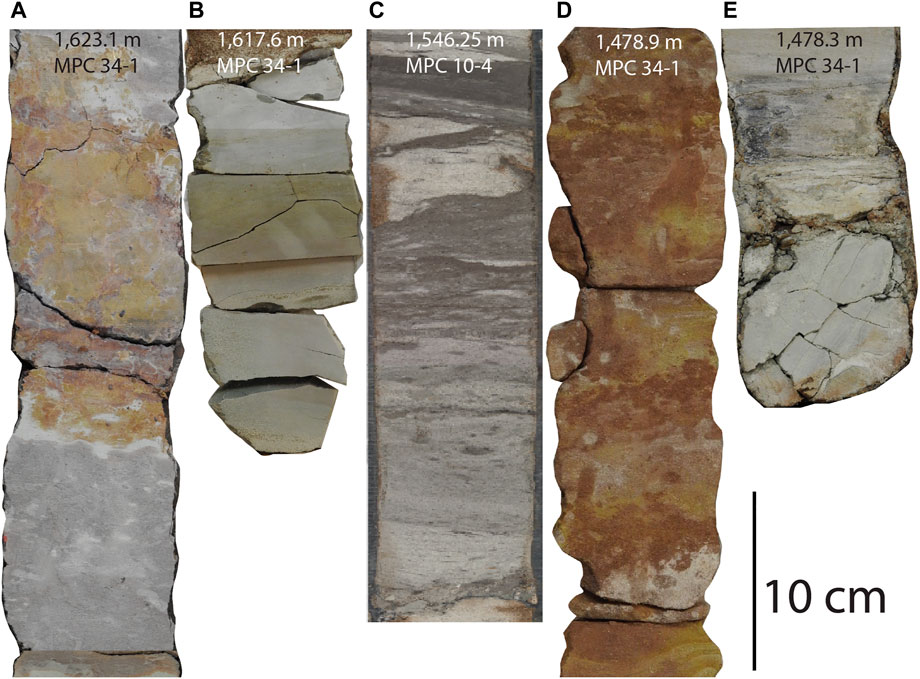
FIGURE 5. Core photographs of mudstone-bearing rocks in the Paluxy Formation and the Washita-Fredericksburg interval in the MPC 10-4 and MPC 34-1 wells. (A) Variegated mudstone facies, 1,623.1 m (5,325 ft) in the MPC 34-1 well. (B) Massive mudstone facies, 1,617.6 m (5,307 ft) in the MPC 34-1 well. (C) Heterolithic mudstone facies, 1,546.25 m (5,073 ft), in the MPC 10-4 well. (D) Variegated and blocky mudstone facies, 1,478.9 m (4,852 ft), with interbedded sandstone and variegated color in the MPC 34-1, Washita-Fredericksburg interval. (E) Blocky gray peds in the variegated and blocky mudstone facies 1,478.3 m (4,850 ft), in the MPC 34-1, Washita-Fredericksburg interval.
The massive mudstone facies was cored in the Paluxy Formation in the MPC 34-1 well (Figure 4A), and is not as common as the variegated mudstone described above. The massive mudstone is up to 0.3 m (1 ft) thick, and is predominantly structureless silty claystone with some faint horizontal laminae. The color ranges from very light gray to greenish gray and moderate greenish yellow, and the facies contains sesquioxide nodules resembling limonite that give this rock a greenish yellow tint (Figure 5B). This facies contains no apparent bioturbation.
Heterolithic mudstone occurs in two intervals in the Paluxy Formation in the MPC 10-4 well and is preserved as thin intervals within a multistorey sandstone unit (Figure 4B). The facies has a net thickness of 0.5 m (1.5 ft) and is characterized by an erosional basal contact mantled with mudstone pebble conglomerate that grades upward into thinly interbedded clay-rich siltstone, sandy mudstone, and light gray, very fine-grained sandstone. In the sandstone layers, current ripple cross-laminae are succeeded by horizontal laminae and quartz-rich siltstone; no bioturbation was observed (Figure 5C).
The variegated and blocky mudstone facies is in the basal Washita-Fredericksburg interval in the MPC 34-1 (Figure 4C) is approximately 1.2 m (4 ft) thick. The facies is thickly interbedded with very light gray sandstone and is a variegated moderate reddish-brown mudstone containing black streaks of organic matter (Figure 5D). The base of the mudstone is light gray and contains desiccation cracks and some horizontal laminae. The mudstone contains blocky peds and includes a sand filled dike at the bottom of the facies (Figure 5E). Some of the peds in the mudstone contain pedogenic slickensides. In general, the bioturbation index is 2-4, and vertical and horizontal burrows are present.
4.3 Mudstone Petrography
Mudstone petrography is discussed with respect to stratigraphic position and facies with emphasis on mudstone fabric, composition, porosity type and diagenetic features. X- ray diffraction data from mudstone in the Paluxy Formation are shown in Table 1 and organic content data are shown in Table 2.
4.3.1 Mudstone Fabric and Composition
The variegated mudstone facies of the Paluxy Formation contains sandy mudstone with some calcite cement. The sample contains quartz grains floating in clay-rich matrix and includes many clay-size quartz clasts (Figure 6). Quartz is the most abundant grain type, and accessory minerals include muscovite and biotite, sparse pyrite framboids, and isolated zircon crystals. The thin sections contain no apparent porosity since intergranular pores are occluded by fine-grained particles, organic matter, and calcite cement.
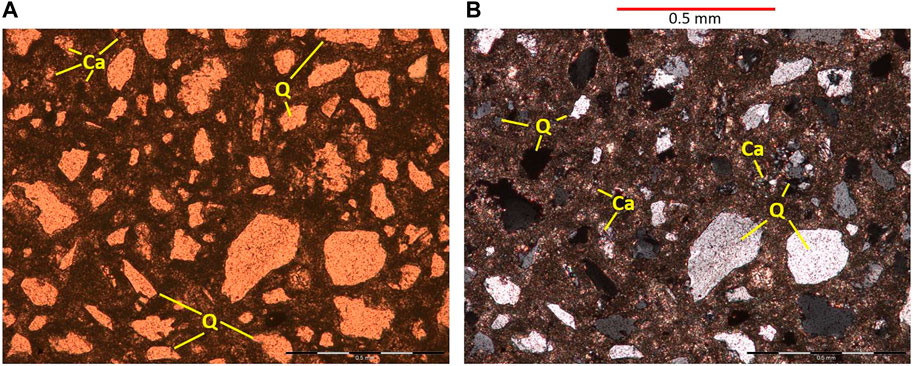
FIGURE 6. Thin section image of variegated mudstone from the MPC 34-1 well, Paluxy Formation, 1,621.5 m (5,319.9 ft). (A) Plane-polarized light (PPL) photomicrograph with calcite (Ca) and Quartz (Q). (B) Cross- polarized light (CPL) photomicrograph.
On the basis of XRD, the massive mudstone facies of the Paluxy Formation is a silty claystone with 57 percent clay minerals consisting of 18.2% kaolinite, 28.4% illite, and 9.7% smectite (Table 1). The sample contains 39.0% quartz and only 4.0% feldspar. The thin section shows weak laminae and abundant silt and clay-size grains (Figures 7A,B). Accessory grains include zircon, pyrite, muscovite, and biotite with micas that are typically elongate with bedding. In SEM images, most pores appear to be artifacts of desiccation and perhaps freezing of the sample, the artifacts contour grains and parallel bedding, the only primary pores observed are isolated intraparticle pores associated with fluid inclusions in quartz grains (Figures 7C,D).
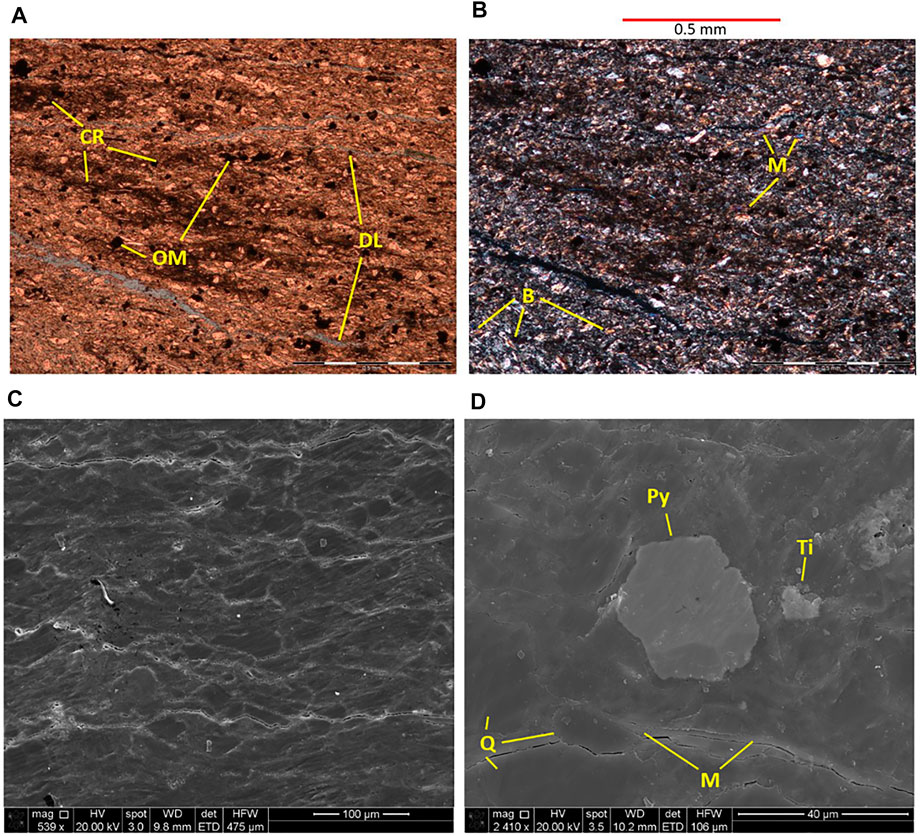
FIGURE 7. Thin section of the massive mudstone facies in the Paluxy Formation, MPC 34-1 well, 1,617.1 m (5,305.5 ft). (A) PPL photomicrograph showing clay-rich lenses (CR), organic matter (OM), and fissures or delamination (DL) along bedding. (B) CPL photomicrograph showing abundant muscovite (M) and less common biotite (B) grains. SEM image of the massive mudstone facies imaged perpendicular to bedding, from the Paluxy Formation MPC 34-1 well, 5,305.6 ft. (C) There is a lack of authigenic pores. (D) SEM/EDS image showing muscovite (M) parallel to bedding, pyrite (Py), quartz (Q), and an accessory mineral rich in titanium (Ti), possibly rutile or anatase.
The heterolithic mudstone facies in the Paluxy Formation is a sandy mudstone with clay laminae. The facies contains 27% clay minerals, 39% quartz, and 4% potassium feldspar (Table 1). This facies has abundant clay laminae and ripple cross-laminae in hand sample, and in thin section, the laminae are inversely graded (Figures 8A,B). Accessory minerals include muscovite, biotite, zircon and pyrite framboids. This facies contains up to 1% TOC and no inorganic carbon (Table 2). Bed-parallel and grain-contouring cracks reflect desiccation of the sample during exposure to atmospheric conditions following recovery. Primary interparticle pores are rare and have diameter on the order of 1 μm, and are partially occluded by clay-size pyrite cubes and kaolinite booklets (Figures 8C,D).
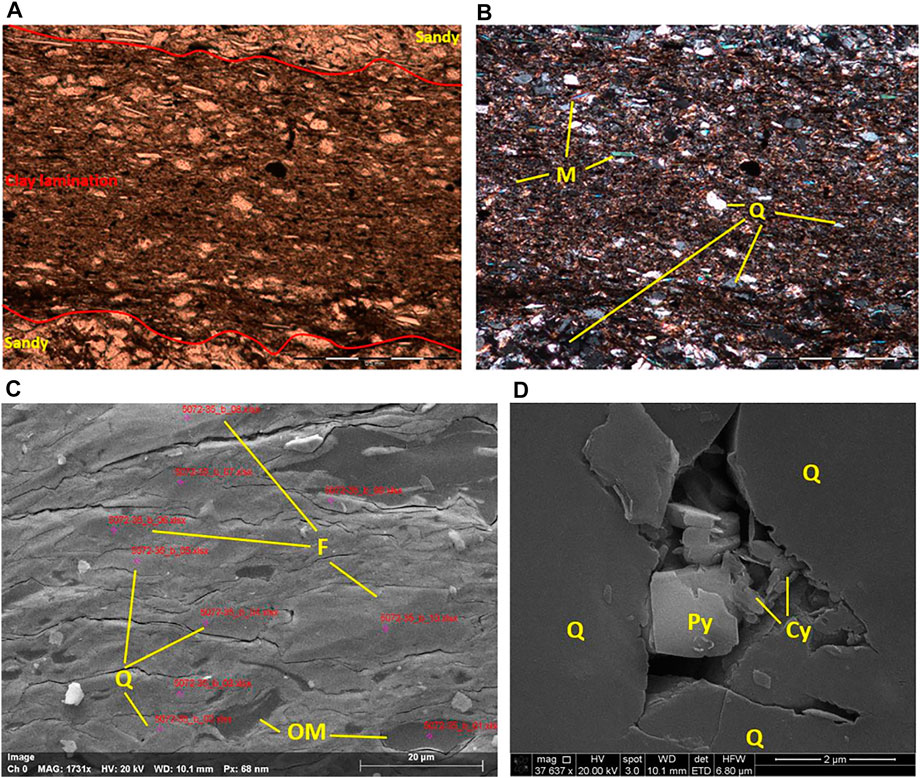
FIGURE 8. Thin section photomicrographs of the heterolithic mudstone facies, Paluxy Formation, MPC 10-4 well, 1,546.1 m (5,072.35 ft). (A) PPL photomicrograph with inversely graded laminar and faint ripple cross-laminae. Thin section in plane polarized light. (B) CPL microphotograph image showing imbricated quartz (Q) and muscovite (M). SEM image of heterolithic mudstone facies, sandy mudstone, Paluxy Formation MPC 10-4 well, 1,546.1 m (5,072.35 ft). (C) SEM image showing micro cracks parallel to bedding and contouring grains quartz (Q), potassium feldspar (F), and organic matter (OM). (D) SEM image of a primary.
The variegated and blocky mudstone facies is sandy to silty mudstone containing approximately 30% clay, 38% sand, and 32% silt. The coarsest grains are fine sand-size quartz and mica grains, the sample is cemented by calcite and pseudo cement clay (Figures 9A,B). Feldspar, microcline, albite, and biotite are accessory minerals. The matrix is abundant in silt- and clay-sized particles. Bioturbation is associated with poor bimodal grain sorting and chaotic bedding (Figures 9C,D). Intergranular space has largely been occluded by carbonate cement and clay-size matrix. Accessory minerals include zircon, pyrite, muscovite, biotite, and opaque minerals.
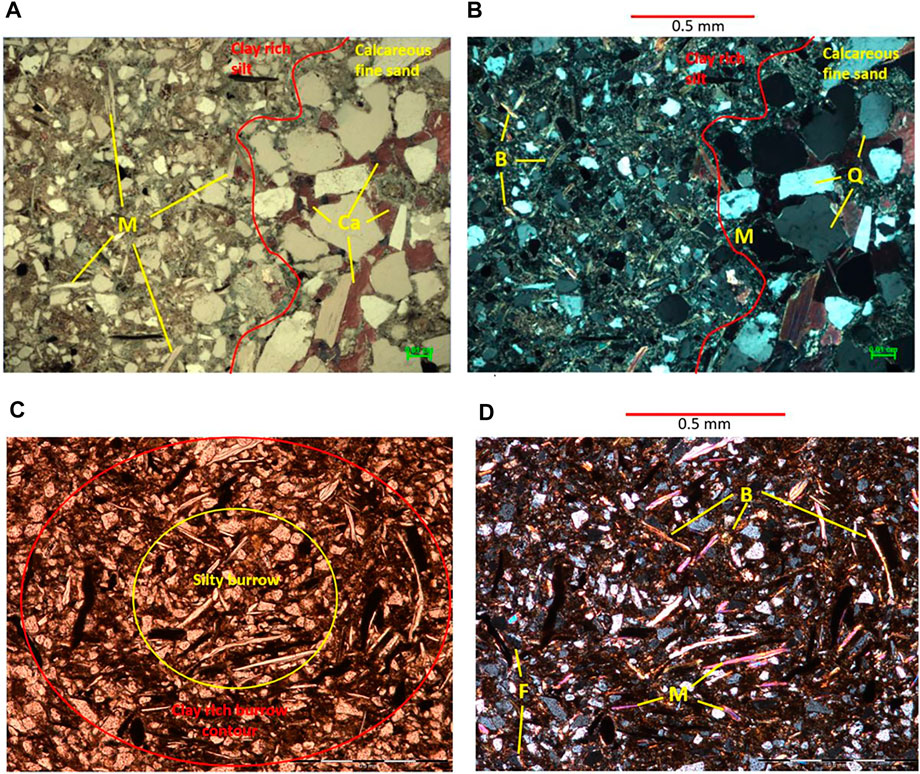
FIGURE 9. Variegated and blocky mudstone facies from the Washita-Fredericksburg interval, MPC 34-1 well 1,482.2 m (4,863.0 ft). (A) PPL photomicrograph showing distinct contact between fine-grained and coarser lithologies due to burrowing. (B) CPL image showing quartz, mica, and minor amounts of biotite. Variegated and blocky mudstone facies, clay rich thin section, from the Washita-Fredericksburg interval in the MPC 34-1 well, 1,478.5 m (4,850.6 ft). (C) PPL image showing a clay-rich, lined horizontal burrow. (D) CPL microphotograph showing muscovite (M) and biotite (B) with some albite and microcline silt (F) with chaotic sorting.
Mudstone in the Paluxy Formation and Washita-Fredericksburg interval is mixed with variable amounts of sand, silt, and clay minerals that give each facies a distinct textural and chemical maturity profile (Figure 10). The sandiest facies is the variegated mudstone, which contains abundant bioturbation and rootlets that resulted in differential textures within a single sample with little carbonate cement in coarser grained laminae. The heterolithic mudstone is largely matrix supported with abundant clay rich laminae and primary pores that are partially occluded by authigenic minerals. The massive mudstone facies is texturally mature with greater that 50% clay minerals in a matrix-supported rock that contains relatively few sand-sized particles and only trace amounts of carbonate cement (<1%). The variegated and blocky mudstone in the Washita-Fredericksburg interval contains abundant sand- and silt-size particles that are associated with burrows and are partially cemented with calcite. The mudstone intervals within the multi-storey sandstone reservoirs contain little organic matter, and intraparticle primary pores are associated with silty to sandy laminae and commonly have partial fills composed of clay, authigenic pyrite, and carbonate.
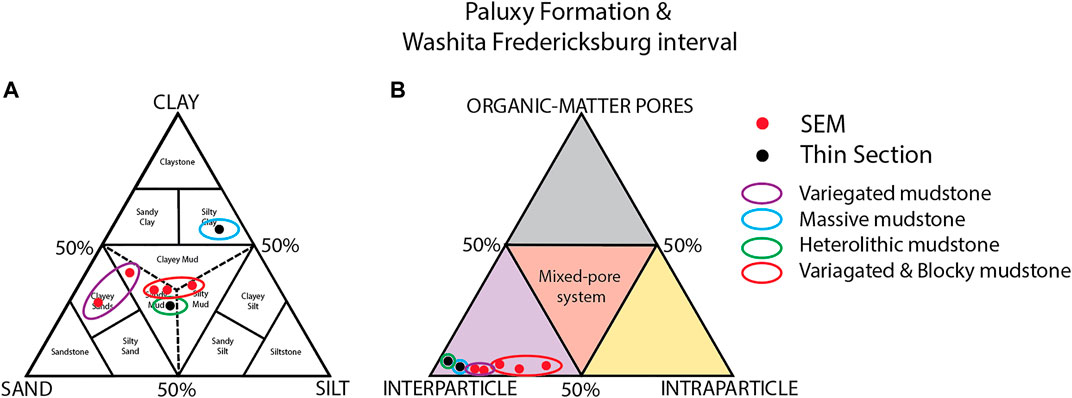
FIGURE 10. Ternary plots showing mudrock composition and pore types in the Paluxy Formation (purple, blue, and green circle) and Washita-Fredericksburg interval. (A) Mudrock classification based on grain size and XRD mineralogical analysis. (B) Pore system analysis from petrographic analysis of mudstone.
The results of pulse-decay permeability analysis indicate that nanodarcy-class permeability is typical. Permeability values range from 1 to 1,700 nD (Table 3). Three of the samples have values between 15 and 25 nD, indicating that the mudstone has significant confining potential. The 1,700 nD sample appears similar visually to the other samples, and the high value appears exceptional and may reflect either a fracture in the sample or imperfect sealing of the core plug in the sleeve.
5 Discussion
5.1 Depositional Environments
Mudstone in the Paluxy Formation and Washita-Fredericksburg interval represents fine-grained deposits associated with bedload-dominated fluvial depositional systems (Folaranmi, 2015; Pashin et al., 2020; Urban, 2020; Wethington, 2020) and resemble a broad range of modern and ancient sandy braided stream deposits (e.g., Miall, 1977; Cant and Walker, 1978; Blodgett and Stanley, 1980; Bridge and Lunt, 2006). Analysis of the sandstone cores indicates that these systems were dominated by channels with transverse bars, and where FMI logs facilitate paleocurrent analysis, results indicates that the streams at least locally flowed northwest, parallel to strike of the margin of the Mississippi Embayment (Pashin et al., 2020). The upper parts of the sandstone and the adjacent sandy mudstone contains root systems and adhesive meniscate burrows (Figures 4, 5), which are typically formed by insects (Hasiotis, 2002), and these structures are interpreted primarily as the products of transverse bars. Root systems, burrow networks, sesquioxide nodules, desiccation cracks, and blocky peds combined with reddened sediment and low organic content, save for scattered coalified plant fragments, provide evidence for stabilization of sediment in oxidizing environments, and these features are typical of spodic paleosols that form in transverse bars and in interfluves in semi-humid to environments (Brewer, 1980; Retallack, 1990).
The heterolithic facies in the Paluxy Formation is developed principally within multi-storey sandstone units and is interpreted as a bar-top deposit that formed in association with transverse and tangential bars. The interstratification of mud and sandstone provides evidence for episodic flows that occurred when the tops of bars were submerged, load structures record fluidized sediment, and the mudstone layers record waning flow and slack-water conditions. Most of these deposits probably formed during flood stage and resemble the sheet-flow deposits described by Hampton and Horton (2007) from bar tops and interfluves.
The paleosols in the variegated, variegated and blocky, and massive mudstone facies have varying degrees of maturity and some degree of soil horizonation. Where the sandstone transitions upward to mudstone, the paleosols are dominated by burrows and contain relict sedimentary structures, including laminae and cross-strata and are interpreted as transitional between the C and B horizon. Sand content generally decreases upward, and sesquioxide nodules are best developed in variegated sandy mudstone containing abundant root traces and burrows characteristic of a lower B horizon. Inversely graded sand layers may be a product of traction during sediment accumulation or may reflect mixing of sediment in the soil profile by burrowing organisms and root systems. The upper parts of the paleosol layers are characterized by blocky peds with pedogenic slickensides and appear to represent the upper part of the B horizon; localized laminae may in places represent remnants of surface layers. Overall, the variable successions of primary sedimentary, pedogenic, and biogenic structures indicate that the thicker mudstone successions represent composites of multiple stacked paleosols, and this stacking is a significant source of heterogeneity of in the baffles and barriers. Conglomeratic layers containing mudstone pebbles indicate that many of these soil layers were prone to erosion and resedimentation. These layers are most common at the bases of the sandstone units and help define individual sandstone storeys.
5.2 Implications of Mudstone Baffles and Barriers
Paluxy Formation mudstone facies vary in composition but in general are sandy to silty mudstone that are rich in clay. The dominant primary pore type is interparticle porosity associated with silty and sandy laminae and lenses, and this porosity appears to be poorly interconnected. The cracks that parallel bedding or contour and follow grain boundaries appear to be mainly secondary fractures that formed during core retrieval, preservation, and storage and reflect desiccation of mudrock with swellable clay and high water saturation that have underwent atmospheric stress. The low permeability of the mudstone units (1 nD–1 µD) relative to the sandstone units (multi-Darcy) favors confinement of CO2 within the sandstone layers where mudstone units maintain lateral continuity (Figure 3). The high clay content, which is dominated by water-sensitive kaolinite and smectite, contributes to low mudstone permeability.
The scale of heterogeneity in the thickness and continuity of the mudstone baffles and barriers (Figure 3) is a direct reflection of the original depositional systems where aggradation, episodic flooding, and shifting of fluvial systems facilitated mud deposition and the preservation of paleosols. Erosional process like channeling and exposure determined where discontinuities formed in the mudstone layers. Paluxy Formation mudstone beds are typically on the order of 0.3 m (1 ft) thick and have an upper thickness limit of about 9 m (30 ft). The lower and upper parts of the Washita-Fredericksburg interval contains significant mudstone units with respect abundance and thickness. There is an average of 18 baffles on the order of 0.3 m (1 ft) thick and 11 barriers on the order of 3 m (10 ft) in each well, and the thickness of individual mudstone units locally approaches 30 m (100 ft). Mudstone barriers in the lower Washita-Fredericksburg interval are thick and widespread enough to prevent significant hydraulic communication between the Paluxy Formation and the Big Fred sand. The barriers in the upper Washita-Fredericksburg interval will likely impede migration of CO2 into shallower strata. Indeed, the high salinity of fluid in the Paluxy and Washita-Fredericksburg (60,000–110,000 mg/L TDS) relative to shallower strata (≤20,000 mg/L TDS) (Pashin et al., 2020) points toward hydraulic isolation of the Lower Cretaceous sandstone units. Baffles will likely help keep injected CO2 in the target sandstone layers and promote lateral plume migration while impeding vertical migration of CO2 into shallower sandstone layers where discontinuities exist in the mudstone layers (Figure 11).
Regionally, restriction of the Lower Cretaceous section to the subsurface and convergence of the sub-Mesozoic disconformity with the mudstone at the top of the Washita-Fredericksburg interval forms a natural stratigraphic trap for CO2 as the reservoir sandstone and confining mudstone units onlap the disconformity. Within the Kemper County Energy facility, lateral confinement is not apparent, but the low reservoir dip (<1°) indicates that injected CO2 would form subsymmetrical plumes with limited updip migration. Facies heterogeneity in the sandstone-mudstone succession is hypothesized to have a stronger impact on plume geometry than any structural factor. Furthermore, it is hypothesized that only fugitive CO2 that is injected in the Big Fred sand and deeper strata would migrate into the Massive sand of lower Tuscaloosa Group, and the Marine Tuscaloosa shale and the major sealing stratum formed by the chalk of the Selma Group and the mudstone of the Porters Creek Clay would ensure protection of the USDWs in the Naheola and Nanafalia Formations (Figure 2). In addition, the sandstone units separating the baffles and barriers in the Lower Cretaceous section would likely capture fugitive CO2, thereby providing additional protection of the shallow USDWs. Ongoing research designed to test this hypothesis is focusing on developing flow models that incorporate the framework put forth in this paper and use scaling of heterogeneity based on the depositional architecture and its modern and ancient analogs to provide a rational prediction of how CO2 will migrate and be confined in the storage complex at the Kemper County energy facility.
6 Conclusion
With a storage resource of about 1.4 Gt in stacked sandstone reservoirs overlain by thick, continuous sealing strata that protect USDWs, the Kemper County energy facility provides the basis for a major geologic CO2 storage complex with capacity to serve as a regional storage hub. The bulk of this resource is in highly permeable sandstone in the Paluxy Formation and the Big Fred sand of the Washita-Fredericksburg interval, which contain numerous mudstone baffles and barriers that help confine the objective sandstone bodies. The mudstone is matrix supported and rich in clay, silt, and sand and is characterized by fluid-sensitive kaolinite and smectite with high water saturation; permeability is on the order of 10 nD in the claystone. The target sandstone reservoirs were deposited in bedload-dominated fluvial deposits, and the mudstone baffles and barriers are principally bar-top deposits and paleosols. Mudstone conglomerate layers form secondary baffles within multistorey sandstone bodies. The numerous baffles within the target reservoir units in the Lower Cretaceous section are hypothesized to help contain injected CO2 in the stacked sandstone units. Barriers are concentrated in the basal part of the Washita-Fredericksburg interval, separating Paluxy reservoirs from the Big Fred sand, and in the upper part of the Washita-Fredericksburg, separating the Big Fred sand from the Dantzler sand. These barriers have potential to confine injected CO2 within the target sandstone intervals, with plumes flattening at the bases of the baffles and barriers and smaller secondary plumes occurring where gaps exist in the mudstone layers. In the event that fugitive CO2 migrates into shallower strata, that CO2 would be contained in Upper Cretaceous strata, which contain many additional sandstone intervals, as well as numerous mudstone units, including the Marine Tuscaloosa shale. In the unlikely event that fugitive CO2 migrates above the Marine Tuscaloosa, the thick sealing section formed by the Selma Group chalk and Porters Creek Clay ensures that CO2 remains in the storage complex and the shallow USDWs of the Naheola and Nanfalia formations are protected.
Data Availability Statement
The datasets presented in this article are not readily available because they need to be downloaded from the US EDX system used by the Department of Energy and mined from grant deliverables. Requests to access the datasets should be directed to amFjay5wYXNoaW5Ab2tzdGF0ZS5lZHU=.
Author Contributions
CW—Data analysis, integration, curation, technical writing, and conceptual modeling. JP—Data curation and analysis, collaboration, and technical writing. JW—Research collaboration and conceptual model development. RE—Site access and research support. DR—Site and laboratory coordination, technical and analytical support.
Funding
This work is supported by the National Energy Technology Laboratory of the U.S Department of Energy through the Southern States Energy Board under prime contracts DE-FE001055 and DE-FE0031888 and the Paul Danheim Nelson named grant of the Grants-in-Aid program of the American Association of Petroleum Geologists.
Author Disclaimer
This paper is based upon work supported by the Department of Energy, Project Number: DE-FE0026086, and was prepared as an account of work sponsored by an agency of the United States Government. Neither the United States Government nor any agency thereof, nor any of their employees, makes any warranty, express or implied, or assumes any legal liability or responsibility for the accuracy, completeness, or usefulness of any information, apparatus, product, or process disclosed, or represents that its use would not infringe privately owned rights. Reference herein to any specific commercial product, process, or service by trade name, trademark, manufacturer, or otherwise does not necessarily constitute or imply its endorsement, recommendations, or favoring by the United States Government or any agency thereof. The views and opinions of authors expressed herein do not necessarily state or reflect those of the United States Government or any agency thereof.
Conflict of Interest
RE was employed by the company Southern Company Services and DR was employed by the company Advanced Resources International.
The remaining authors declare that the research was conducted in the absence of any commercial or financial relationships that could be construed as a potential conflict of interest.
Publisher’s Note
All claims expressed in this article are solely those of the authors and do not necessarily represent those of their affiliated organizations, or those of the publisher, the editors and the reviewers. Any product that may be evaluated in this article, or claim that may be made by its manufacturer, is not guaranteed or endorsed by the publisher.
Acknowledgments
The authors wish to acknowledge the support of Southern Company Services and Mississippi Power Company, who provided site access and Advanced Resources International, who coordinated site activities, including well logging and core analysis, Southern States Energy Board, who promote sequestration opportunities in the Gulf Coast region, and the journal reviewers whose, comments improved the quality of this contribution.
References
Abert, C. C., Marshak, S., and Larson, T. H. (2016). Geological and Geophysical Maps of the Illinois Basin-Ozark Dome Region. Champaign, Illinois: Illinois State Geological Survey, Prairie Research Institute. Map 23, scale 1:500,000.
Bachu, S., Bonijoly, D., Bradshaw, J., Burruss, R., Holloway, S., Christensen, N. P., et al. (2007). CO2 Storage Capacity Estimation: Methodology and Gaps. Int. J. Greenh. gas control 1, 430–443. doi:10.1016/s1750-5836(07)00086-2
Bicker, A. R. (1969). Geologic Map of Mississippi. Jackson, Mississippi: Department of Environmental Quality, Mississippi Office of Geology. scale 1:500,000.
Blodgett, R. H., and Stanley, K. O. (1980). Stratification, Bedforms, and Discharge Relations of the Platte Braided River System, Nebraska. J. Sediment. Petrology 50 (1), 139–148. doi:10.1306/212f7987-2b24-11d7-8648000102c1865d
Bridge, J. S., and Lunt, I. A. (2006). “Depositional Models of Braided Rivers,” in Braided Rivers, Processes, Deposits, Ecology and Management (Oxford: Blackwell Publishing), 36, 11–50.
Cant, D. J., and Walker, R. G. (1978). Fluvial Processes and Facies Sequences in the Sandy Braided South Saskatchewan River, Canada. Sedimentology 25, 625–648. doi:10.1111/j.1365-3091.1978.tb00323.x
Cavanagh, A. J., and Haszeldine, R. S. (2014). The Sleipner Storage Site: Capillary Flow Modeling of a Layered CO2 Plume Requires Fractured Shale Barriers within the Utsira Formation. Int. J. Greenh. Gas Control 21, 101–112. doi:10.1016/j.ijggc.2013.11.017
Cushing, E. M., Boswell, E. H., and Hosman, R. L. (1964). General Geology of the Mississippi Embayment. U.S. Geological Survey Professional Paper 448-B, 8.
Doughty, C., and Pruess, K. (2004). Modeling Supercritical Carbon Dioxide Injection in Heterogeneous Porous Media. Vadose Zone J. 3, 837–847. doi:10.2113/3.3.837
Downey, M. W. (1994). “Hydrocarbon Seal Rocks, Part II,” in The Petroleum System— from Source to Trap (Tulsa, Oklahoma: AAPG Memoir), 60, 159–164.
Esposito, R., Riestenberg, D., and Gray, K. S. (2017). Establishing an Early CO2 Storage Complex in Kemper County, Mississippi: Project ECO2S (FE0029465). United States: Southern States Energy Board, U.S. Department of Energy, 6–10.
Folaranmi, A. T. (2015). Geologic Characterization of a Saline Reservoir for Carbon Sequestration: The Paluxy Formation, Citronelle Dome, Gulf of Mexico Basin (Alabama: Stillwater, Oklahoma State University), 110. unpublished Master’s thesis.
Goodman, A., Hakala, A., Bromhal, G., Deel, D., Rodosta, T., Frailey, S., et al. (2011). U.S. DOE Methodology for the Development of Geologic Storage Potential for Carbon Dioxide at the National and Regional Scale. Int. J. Greenh. Gas Control 5, 952–965. doi:10.1016/j.ijggc.2011.03.010
Hale-Ehrlich, W. S., and Coleman, L. (1993). Ouachita-Appalachian Juncture: a Paleozoic Transpressional Zone in the Southeastern U.S.A. Am. Assoc. Petroleum Geol. Bull. 77, 552–568.
Hampton, B.,A., and Horton, B.,K. (2007). Sheetflow Fluvial Processes in a Rapidly Subsiding Basin, Altiplano Plateau, Bolivia. Sedimentology 54, 1129. doi:10.1111/j.1365-3091.2007.00875.x
Hasiotis, S. T. (2002). Continental Trace Fossils. SEPM Short. Course Notes. (Tulsa, Oklahoma: Society of Economic Paleontologists and Mineralogists (SEPM)) 51, 132.
Kolata, D. R., Treworgy, J. D., and Masters, J. M. (1981). Structural Framework of the Mississippi Embayment of Southern Illinois. Champaign, IL: Illinois State Geological Survey, 38. Circular 516.
Loucks, R. G., Reed, R. M., Ruppel, S. C., and Hammes, U. (2012). Spectrum of Pore Types and Networks in Mudrocks and a Descriptive Classification for Matrix-Related Mudrock Pores. Bulletin 96, 1071–1098. doi:10.1306/08171111061
MacEachern, J. A., and Bann, K. L. (2008). “The Role of Ichnology in Refining Shallow Marine Facies Models,” in Recent Advances in Models of Siliciclastic Shallow-Marine Stratigraphy. Editors G. J. Hampson, R. J. Steel, P. B. Burgess, and R. W. Dalrymple (Tulsa, Oklahoma: SEPM Special Publication), 90, 73–116. doi:10.2110/pec.08.90.0073
Matthew, A. J., Woods, A. J., and Oliver, C. (1991). “Spots before the Eyes: New Comparison Charts for Visual Percentage Estimation in Archaeological Material,” in Recent Developments in Ceramic Petrology (London: British Museumv), 81, 221–263.
Miall, A. D. (1977). A Review of the Braided-River Depositional Environment. Earth-Science Rev. 13, 62. doi:10.1016/0012-8252(77)90055-1
Moore, D.,M., and Reynolds, R.,C. (1989). X-ray Diffraction and the Identification and Analysis of Clay Minerals. Oxford, United Kingdom: Oxford University Press, Impac methodology XRD, 378.
Munsell Color (2009). Geological Rock-Color Chart with Genuine Munsell Color Chips. Boulder, CO: Geological Society of America, 6–12.
Neuzil, C. E. (1994). How Permeable Are Clays and Shales? Water Resour. Res. 30, 145–150. doi:10.1029/93wr02930
Pashin, J. C., Achang, Mercy., Martin, Sam., Urban, S. K., and Wethington, C. L. R. (2020). Commercial-scale CO2 Injection and Optimization of Storage Capacity in the Southeastern United States (Project ECO2S, Kemper County Energy Facility, Mississippi). Stillwater, Oklahoma: U.S. Department of Energy, National Energy Technology Laboratory, 65. Final Report, contract DE-FE001055.
Pashin, J. C., Hills, D. J., Kopaska-Merkel, D. C., and McIntyre, M. R. (2008). Geologic Evaluation of the Potential for CO2 Sequestration in Kemper County, Mississippi. Final Report, Southern Company Contract 8-08-00142, 53.
Pashin, J. C., Wethington, C. L. R., Urban, S., Achang, M., Riestenberg, D., and Esposito, R. A. (2021). “Stratigraphy and Sedimentology of Mesozoic-Cenozoic Strata in a World-Class CO2 Storage Complex, Kemper County Energy Facility, Gulf of Mexico Basin, Mississippi, USA,” in Society of Exploration Geophysicists and American Association of Petroleum Geologists IMAGE Conference Proceedings, unpaginated. (abstract and poster) (Tulsa, Oklahoma: AAPG/SEG Image).
Picard, M. D. (1971). Classification of Fine-Grained Sedimentary Rocks. J. Sediment. Res. 41, 179–195. doi:10.1306/74d7221b-2b21-11d7-8648000102c1865d
Rainwater, E. H. (1961). Outline of Geological History of Mississippi. Gulf Coast Assoc. Geol. Soc. Trans. 11, 43–45.
Reitze, A. W. (2012). Carbon Capture and Storage Program's NEPA Compliance. Environ. Law Rev. 42, 10853.
Retallack, G. J. (1990). Soils of the Past—An Introduction to Paleopedology. Boston: Unwin–Hyman, 520, 349.
Riestenberg, D. E., Koperna, G. J., Pashin, J. C., McLaughlin, J. F., Walsh, P. M., Myers, G. C., et al. (2019). “Project ECO2S: Characterization of a World Class Carbon Dioxide Storage Complex,” in Proceedings of the 14th International Conference on Greenhouse Gas Control Technologies, Melbourne, Australia (London, United Kingdom: International Energy Agency), 13. GHGT-14, SSRN id3366002.
Salvador, A. (1987). Late Triassic-Jurassic Paleogeography and Origin of Gulf of Mexico Basin. AAPG Bull. 71, 419–451. doi:10.1306/94886ec5-1704-11d7-8645000102c1865d
Stearns, R. G., and Marcher, M. V. (1962). Late Cretaceous and Subsequent Structural Development of the Northern Mississippi Embayment Area. Geol. Soc. Am. Bull. 73 (11), 1387–1394. doi:10.1130/0016-7606(1962)73[1387:lcassd]2.0.co;2
Thomas, W. A. (1973). Southwestern Appalachian Structural System beneath the Gulf Coastal Plain. Am. J. Sci. 273, 372–390.
Thomas, W. A. (1985). The Appalachian-Ouachita Connection: Paleozoic Orogenic Belt at the Southern Margin of North America. Annu. Rev. Earth Planet. Sci. 13, 175–199. doi:10.1146/annurev.ea.13.050185.001135
Thomas, W. A. (1991). The Appalachian-Ouachita Rifted Margin of Southeastern North America. Geol. Soc. Am. Bull. 103, 415–431. doi:10.1130/0016-7606(1991)103<0415:taormo>2.3.co;2
Thomas, W. A. (1988). “The Black Warrior Basin,” in Sedimentary Cover — North American Craton. Editor L. L. Sloss (Boulder, CO: Geological Society of America, The Geology of North America), D-2, 471–492.
Urban, S., K. (2020). Geologic Characterization of Cretaceous Sandstone at the Kemper County Energy Facility, Mississippi: A World-Class Site for CO2 Storage. Stillwater, Oklahoma: Masters of Science Thesis, Oklahoma State University, 14–22.
Wentworth, C. K. (1922). A Scale of Grade and Class Terms for Clastic Sediments. J. Geol. 30, 377–392. doi:10.1086/622910
Keywords: sequestration, CCS, kemper county, plant ratcliffe, gulf coast, paluxy, washita-fredericksburg
Citation: Wethington C, Pashin J, Wethington J, Esposito R and Riestenberg D (2022) Mudstone Baffles and Barriers in Lower Cretaceous Strata at a Proposed CO2 Storage Hub in Kemper County, Mississippi, United States. Front. Energy Res. 10:904850. doi: 10.3389/fenrg.2022.904850
Received: 26 March 2022; Accepted: 25 May 2022;
Published: 24 June 2022.
Edited by:
Yongfei Yang, China University of Petroleum (East China), ChinaReviewed by:
Hu Guo, China University of Petroleum, Beijing, ChinaHussein Hoteit, King Abdullah University of Science and Technology, Saudi Arabia
Copyright © 2022 Wethington, Pashin, Wethington, Esposito and Riestenberg. This is an open-access article distributed under the terms of the Creative Commons Attribution License (CC BY). The use, distribution or reproduction in other forums is permitted, provided the original author(s) and the copyright owner(s) are credited and that the original publication in this journal is cited, in accordance with accepted academic practice. No use, distribution or reproduction is permitted which does not comply with these terms.
*Correspondence: Conn Wethington, Y29ubi53ZXRoaW5ndG9uQG9rc3RhdGUuZWR1