- College of Chemistry and Environmental Engineering, Yangtze University, Jingzhou, China
Hyperbranched polymer is a kind of polymer with a three-dimensional structure, which contains a highly branched structure and a large number of modified terminal functional groups. The desired properties can be obtained by modifying the active end groups appropriately. Hyperbranched polymers have been successfully used in the development of oilfield chemicals due to their excellent properties of solubility, rheology, temperature resistance, and salt resistance. Therefore, the application of hyperbranched polymer is helpful to the efficient development of oilfields. In this paper, the synthesis methods of hyperbranched polymers are reviewed. The advantages and disadvantages of different synthesis methods are analyzed. Furthermore, the research progress of hyperbranched polymers in oilfield chemistry is systematically described, and the advantages and existing problems of their application in polymer flooding as oil displacement agents, demulsification of crude oil, clay hydration expansion inhibition, and shale inhibition are discussed. Finally, the application prospect of hyperbranched polymer in drilling chemistry, oil recovery chemistry, and gathering and transportation chemistry is forecasted, which is expected to help the popularization and application of hyperbranched polymer in oilfields.
Introduction
Hyperbranched polymer is a kind of polymer with a three-dimensional structure that is highly branched and a large number of modified terminal functional groups. Hyperbranched polymers possess both the multi-terminal structure of dendritic macromolecules and the large molecular weight of linear polymers. The physical and chemical properties of hyperbranched polymers can be changed if the rich active end groups are appropriately modified. Moreover, the highly branched structure minimizes possible interactions and entanglement between molecular chains, resulting in a lower viscosity of hyperbranched polymers than linear polymers. Because of this special molecular structure, it shows better solubility, rheology, temperature, and salt resistance than linear polymers.
Hyperbranched polymers have attracted more and more attention in recent years. Their synthesis methods are constantly updated, and more monomers are used to synthesize hyperbranched polymers. Different synthesis methods have different requirements on reaction conditions and monomer structure, and the properties of hyperbranched polymers are also different. Although the concept of hyperbranched polymers was put forward less than 100 years ago, people have already completed laboratory research on industrial applications. Hyperbranched polymers have been successfully applied in medical treatment, textile, coatings, luminescent materials, and other fields (Zhang et al., 2020a; Zhang et al., 2020b; Shimizu et al., 2020; Wang et al., 2020).
With the extensive application of hyperbranched polymers in various fields, oilfield chemistry workers have also carried out research on hyperbranched polymers. In this paper, the synthesis methods of hyperbranched polymers are discussed, and the advantages and disadvantages of different synthesis methods are analyzed. Research progress in oilfield chemistry is reviewed, and the advantages and problems of applications in oil displacement, the demulsification of crude oil, control of clay hydration expansion, and shale control are analyzed. Moreover, the application prospect of hyperbranched polymer in other fields of oilfield chemistry is forecasted, which is expected to facilitate the popularization and application of hyperbranched polymer in oilfields.
Synthesis Methods of Hyperbranched Polymers
The history of hyperbranched polymers can be traced back to the end of the 19th century. In early studies, hyperbranched resins were prepared by polycondensation of organic acids and alcohols, but no name was given to this structure (Gao and Yan, 2004). It was not until 1952 that Flory first proposed the concept of hyperbranched polymers (Flory, 1952). In 1992, Kim and Webster (1992) successfully synthesized hyperbranched polystyrene, and since then, hyperbranched polymers have attracted much attention because of their unique properties.
Hyperbranched polymers can be divided into six types according to their structural characteristics, as shown in Figure 1.
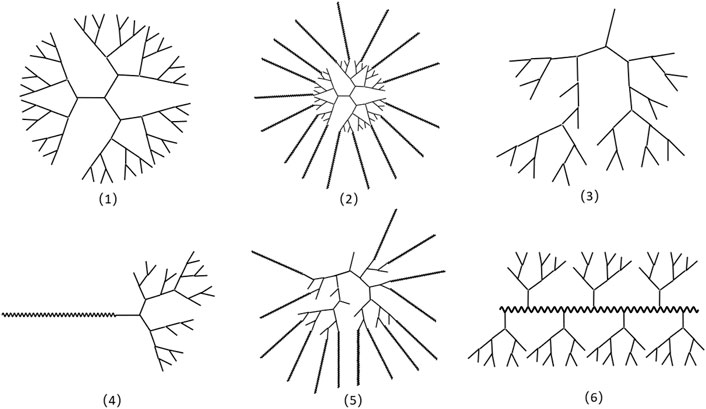
FIGURE 1. Schematic structure of hyperbranched polymers: 1) dendrimer structure without grafting; 2) dendritic-linear grafted polymer structure; 3) hyperbranched macromolecular structure without grafting; 4) hyperbranched-linear grafted single-arm structure; 5) hyperbranched-linear grafted multi-arm structure; 6) hyperbranched grafted structure (Gao and Yan, 2004).
The structures of hyperbranched polymers are divided into the dendrimer structure without grafting, dendritic-linear grafted polymer structure, hyperbranched macromolecular structure, hyperbranched-linear grafted single-arm structure, hyperbranched-linear grafted multi-arm structure, and hyperbranched grafted structure. Figure 1 shows the six existing hyperbranched polymer structure types.
The structural characteristics of these six types of hyperbranched polymers are determined by their synthesis methods. The dendritic structure and hyperbranched structure could be prepared through repeated reactions, and the molecular weight of polymers is relatively small. The other four kinds of structures are obtained by copolymerization of other monomers with a hyperbranched core, and the molecular weight is larger than that of dendritic macromolecules and hyperbranched macromolecules.
At present, the commonly used methods for synthesizing hyperbranched polymers are condensation polymerization, ring opening polymerization, and free radical polymerization. With the wide application of hyperbranched polymers, scholars have also tried to synthesize hyperbranched polymers by some novel synthesis methods, such as click chemistry, cross-coupling reaction, dipole cycloaddition reaction, and coupling monomer method.
Condensation Polymerization
Condensation polymerization refers to the process of repeated condensation reaction between monomers with two or more active groups to form polymers while releasing water and other small molecules. Most of the polycondensation reactions are reversible and stepwise, and the molecular weight increases gradually with the increase of reaction time. However, the conversion rate of monomers is almost independent of time.
Polycondensation of ABn-type monomers is a commonly used method for the synthesis of hyperbranched polymers. Many researchers have successfully synthesized polyphenylene, polyether, polyester, and polyamide by this method. The monomers used initially were AB2-type monomers, such as ethanolamine, ethylene glycol amine, and aminobenzene. With the wide application of this method, more and more monomers are used. Commonly used monomers are propylene glycol, trimethylol propane, and other AB3-type monomers (Zhang et al., 2019a), as well as ethylenediamine, pentaerythritol, p-diaminobenzene, and other AB4-type monomers (Wörl and Köster, 1999).
Guillaume (Rousseau et al., 2013) synthesized a hyperbranched polymer using ethanolamine and methyl acrylate as raw materials through Michael addition and condensation reaction of carboxylate ester with the amino group. The reaction process is shown in Figure 2. The hyperbranched polymer has the advantages of high yield and mild reaction conditions. However, this reaction is carried out under many repeated operations, and the products of each step need to be purified before the next step. Therefore, the cost of synthesis is expensive, which is not suitable for industrial applications. In addition, the reaction product contains a large number of ester groups, which are readily hydrolyzed at high temperature conditions. Therefore, the product is not suitable for high temperature conditions.
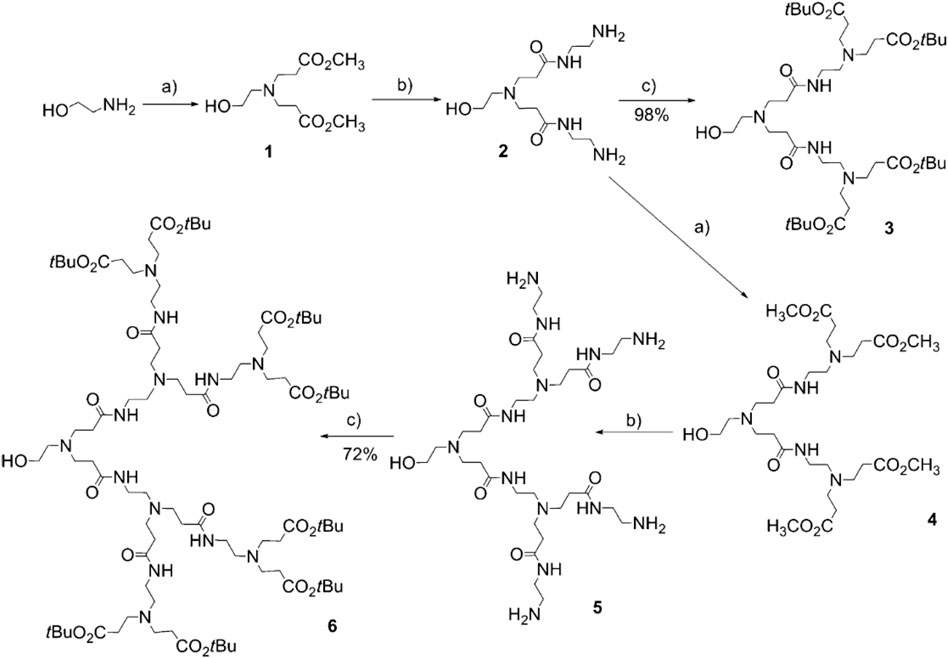
FIGURE 2. Condensation polymerization of AB2-type monomers into hyperbranched polymers (Rousseau et al., 2013).
The basic requirements for this reaction of ABn-type monomers are the following: 1) The A and B parts of the monomers will not have other side reactions during the polycondensation reaction. 2) All groups in part B have the same activity, and intramolecular cyclization does not occur in this group. To better control the molecular weight of the polymer and the geometric configuration of the molecular structure, a synthesis method of adding Bm to the ABn-type monomers to form ABn + Bm–type monomers was proposed. The method can control the molecular weight of the target polymer by controlling the different ratios of Bm and ABn. Li et al. (2019) synthesized a hyperbranched cationic clay stabilizer by condensation polymerization using ethylene glycol amine, trimethylolpropane, and maleic anhydride as materials.
The method of condensation polymerization has two advantages, high efficiency and wide-ranging sources of monomers, but its application is limited because the products are easily hydrolyzed.
Ring Opening Polymerization
Ring opening polymerization refers to the reaction in which cyclic compound monomers are converted into linear polymers through ring opening addition. The monomers used in the hyperbranched polymers synthesized by ring opening polymerization are heterocyclic compounds, such as ethylene oxide, caprolactone, tetrahydrofuran, and cyclic carbamate.
This method was first reported by Suzuki et al. in 1992. They synthesized hyperbranched polyamines by ring opening polymerization of cyclic carbamates under the catalysis of palladium. In 1998, they used the same method to synthesize hyperbranched polyamine from 5-methylene-1,3-oxazidinecyclo-2-one (Suzuki et al., 1998). Ring opening polymerization has been used to synthesize hyperbranched polyamide, polyether, and polyester.
Based on the ring opening polymerization method, Xing14 synthesized a hyperbranched polymer containing hydroxyl group from trimethylpropane triglycidyl ether, butanediol, and o-diphenol through ring opening polymerization and condensation reaction successively, in which tetrabutylammonium bromide (TBAB) was the phase transfer catalyst. The reaction process is shown in Figure 3. Xiao et al. (2020) used methyl eleostearate (ME), maleic anhydride (MA), and diglycidyl ether of bisphenol A (DGEBA) as raw materials and synthesized a multi-carboxyl hyperbranched polymer called TOHBP by ring opening polymerization. The reaction process is shown in Figure 4. The analysis of the synthesis routes of the two hyperbranched polymers shows that the reaction has significant advantages. First of all, the synthesis of hyperbranched polymer from epoxy monomer requires only one step reaction, and the reaction process is simple. Second, this reaction can be carried out under mild conditions, with fewer by-products than polycondensation, and it is easy to obtain polymers of high molecular weight. However, this reaction has a non-negligible disadvantage, that is, its raw materials must contain epoxy-based monomer, so the source of the reactive monomer is limited.
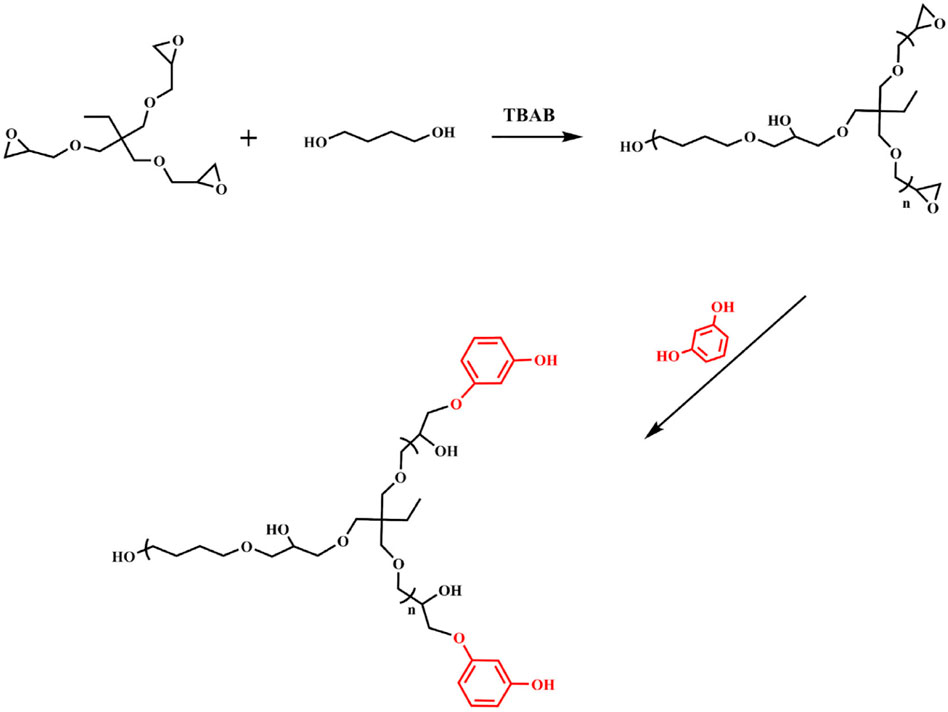
FIGURE 3. Synthesis route for the hydroxyl-containing hyperbranched polymer (Xing et al., 2020).
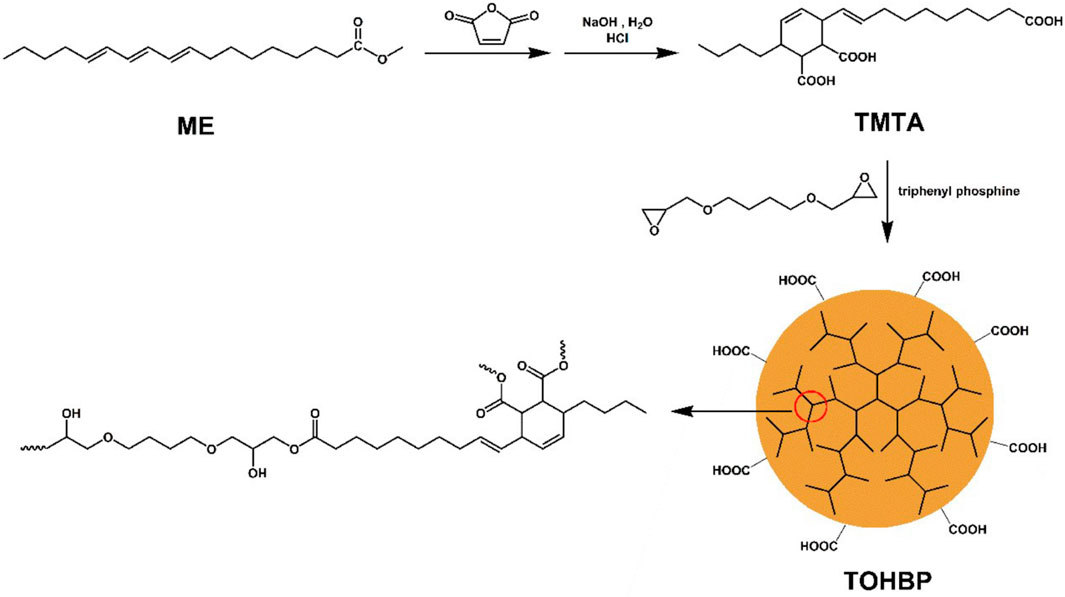
FIGURE 4. Synthesis route of TOHBP (Xiao et al., 2020).
The monomers used in ring opening polymerization require a special ring structure, so the sources of monomers are limited. However, due to the special structure of the monomer, the synthesized polymer is amphiphilic after terminal modification. Therefore, its application field has also been expanded.
Free Radical Polymerization
The monomers of free radical polymerization are mostly alkenes containing unsaturated double bonds. In the reaction, the double bond in the monomer is opened, and the addition reaction is repeated many times between the molecules, thus joining many monomers together to form the macromolecule.
The free radical polymerization of hyperbranched polymers was first reported by Fréchet et al. (1995). The monomers used generally contain a vinyl group and a reactive group capable of initiating vinyl polymerization. The reactive group can be a free radical, cationic or anionic. During the reaction, reactive groups can initiate the growth of vinyl groups and migrate to form new active sites during the chain growth process to continue to initiate vinyl polymerization.
Marasini et al. (2020) synthesized a hyperbranched polymer through the reversible addition–fragmentation chain transfer (RAFT) polymerization technique using N,N-dimethylformamide (DMF) as the solvent. In this reaction, 4-cyano-4((dodecylsulfanylthiocarbonyl)sulfanyl)pentanol (CTA), polyethylene glycol monomethyl ether methacrylate (PEGMA), 2-aminoethyl methacrylate hydrochloride (AEMA), and ethylene glycol dimethacrylate (EGDMA) or N, N-bis(acryloyl)cystamine (BAC) are used as monomers and 2,2-azobis(2-methylpropionitrile) (AIBN) as an initiator. The reaction process is shown in Figure 5.
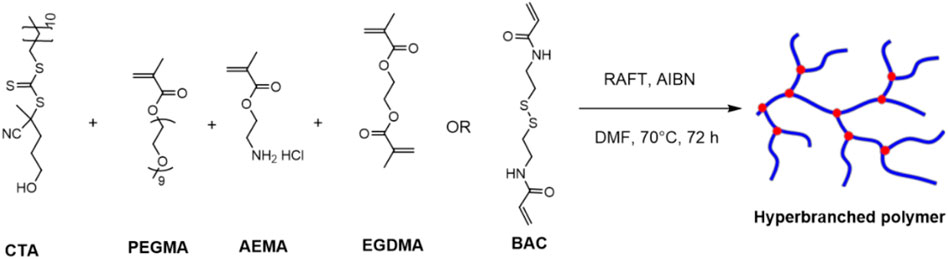
FIGURE 5. Radical polymerization to form hyperbranched polymers (Marasini et al., 2020).
The use of radical polymerization to synthesize hyperbranched polymers allows a wider range of monomers to be used, and polymers with a larger molecular weight can be obtained by taking advantage of the special interaction between vinyl and the active group excitation point. Unlike linear polymers, hyperbranched polymers synthesized by this method require multiple vinyl groups in the monomer. However, this method tends to produce gel in the reaction process, resulting in a very low polymerization conversion rate of monomer. And the degree of branched polymer cannot be measured by equipment. In addition, the molecular weight distribution of synthesized polymers is wide, so it is difficult to obtain fixed molecular weight polymers.
Other Synthetic Methods
With the further study of hyperbranched polymers, more and more novel synthesis methods have been used to prepare hyperbranched polymers.
1) Cross-coupling reaction
The Suzuki reaction, also known as the Suzuki coupling reaction or Suzuki–Miyaura reaction, is a relatively new organic coupling reaction. The reaction involves the cross-coupling of aryl or alkenyl boric acid (borate ester) with chlorine, bromine, iodinated aromatics, or olefins catalyzed by a zero-valent palladium complex. Zhang et al. (2020c) synthesized a type of hyperbranched polymer through Suzuki reaction using two monomers containing arylboronic acid groups and brominated aromatic hydrocarbons, as shown in Figure 6.
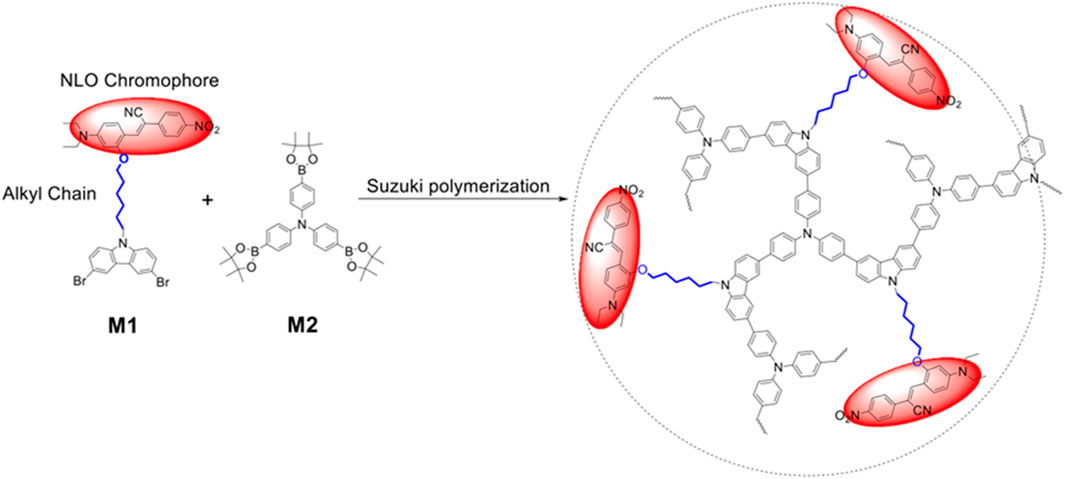
FIGURE 6. Synthesis of hyperbranched polymers by Suzuki–Miyaura reaction (Zhang et al., 2020c).
The Sonogashira coupling reaction is also a typical cross-coupling reaction, in which a carbon–carbon bond is formed between a terminal alkyne and an aryl or vinyl halide by using a palladium catalyst. Wei et al. (2020) used tris(4-acetylenylphenyl)amine (TPEP) and 9-ferrocenylidene-2,7-diiodo-9H-fluorene (FIF) as monomers and carried out Sonogashira coupling reaction under the action of Pd catalyst to synthesize two kinds of hyperbranched polymers, a spherical polymer called SP and a hollow polymer called HP. The polymer HP was synthesized according to the same procedure as SP; however, zeolitic imidazole framework-67 (ZIF-67) was added to the reaction mixture as the template. The reaction process is shown in Figure 7.
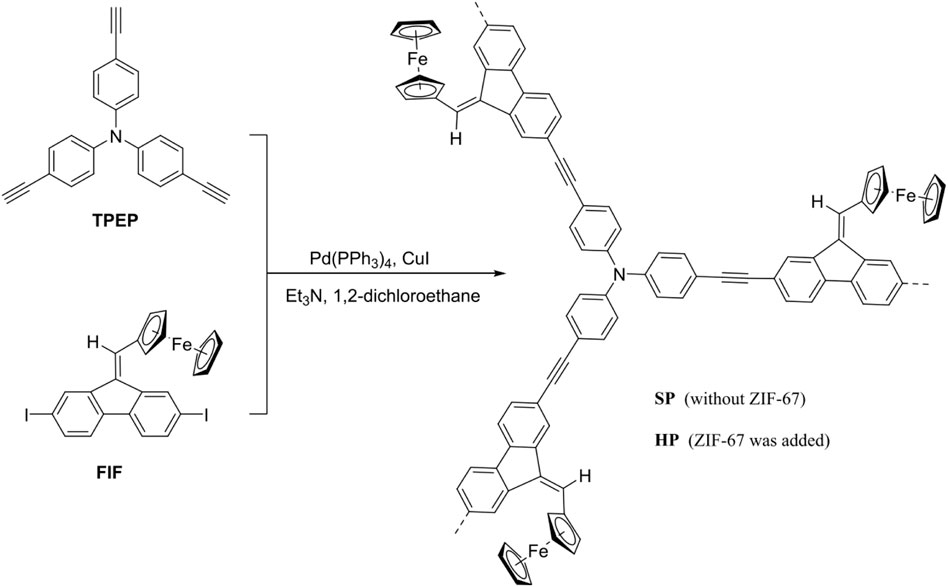
FIGURE 7. Sonogashira coupling reaction to synthesize hyperbranched polymers (Wei et al., 2020).
The Heck reaction is a reaction in which a halogenated hydrocarbon and activated unsaturated hydrocarbon generate coupling products under palladium catalysis. Gan et al. (2020) synthesized a hyperbranched polymer from an AB2-type monomer and B3-type monomer through Cu-catalyzed Heck reaction. The reaction process is shown in Figure 8.
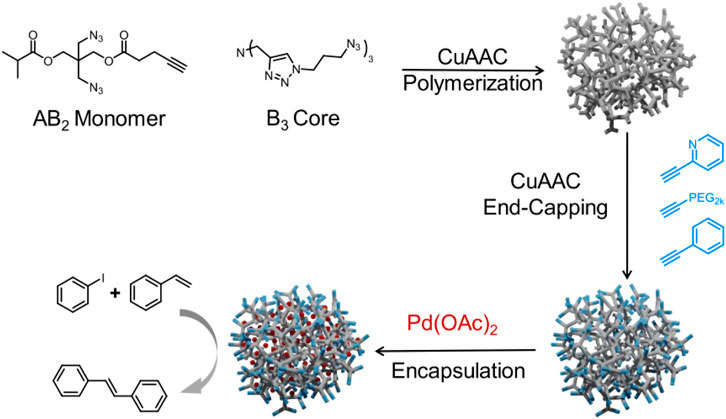
FIGURE 8. Heck reaction to synthesize hyperbranched polymers (Gan et al., 2020).
2) Huisgen reaction
The Huisgen reaction, also known as the 1,3-dipolar cycloaddition reaction, is a cycloaddition reaction between 1,3-dipole and alkene, alkyne, or derivatives of alkenes and alkynes. The product is a five-member heterocyclic compound. Based on click chemistry, Ye et al. (2020) synthesized a hyperbranched polymer containing a poly(ε-caprolactone) (PCL) chain and polystyrene (PS) chain through azide-alkyne Huisgen cycloaddition reaction, as shown in Figure 9.
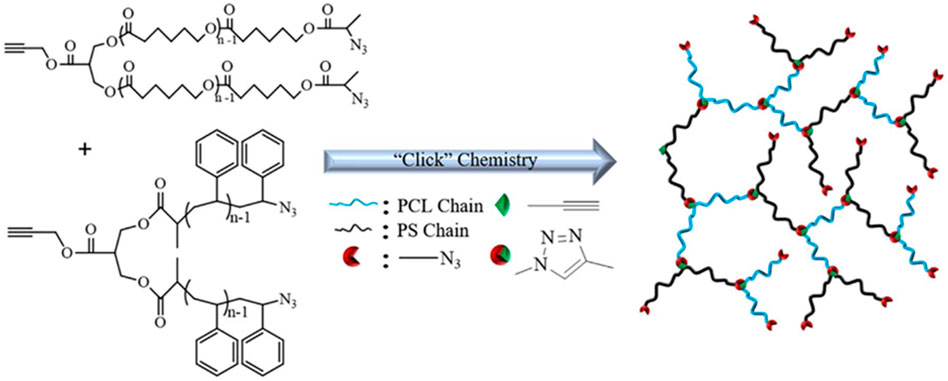
FIGURE 9. Synthesis of hyperbranched polymers by azide-alkyne Huisgen cycloaddition (Ye et al., 2020).
3) Coupling monomer method
Yan and Gao (2000) used the coupling monomer method to make the two monomers in situ generate ABn-type intermediates in the reaction system and then obtained hyperbranched polymers by further polymerization. At present, hyperbranched polyether, polyethoxylsilane, polyamide, and poly(ester-amide) have been successfully synthesized by this method. Based on this method, Zhang et al. (2020a) synthesized a hyperbranched polysulfide with abundant end-sulfhydryl groups called HBPTE by Michael addition reaction. The reaction process is shown in Figure 10.
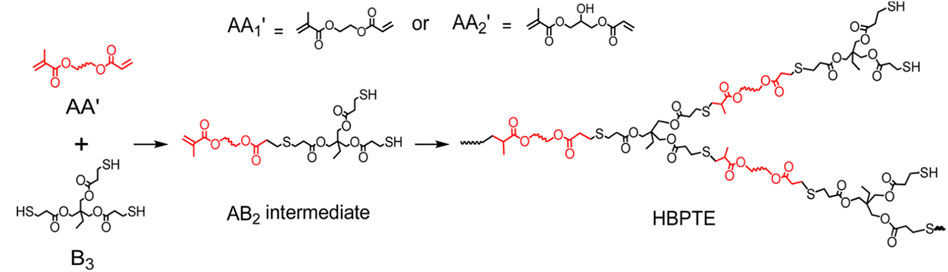
FIGURE 10. Synthesis of hyperbranched polymers by the coupling monomer method (Zhang et al., 2020a).
At present, condensation polymerization, ring opening polymerization, and free radical polymerization are commonly used in the synthesis of hyperbranched polymers. Although many scholars have studied some new methods for the synthesis of hyperbranched polymers, their application is limited due to the particularity of reaction conditions and monomer structure. And scholars have only discussed the feasibility of these novel synthetic methods for the synthesis of hyperbranched polymers, and these methods are still in the exploratory stage, and there is not enough evidence to prove their advantages and disadvantages. The advantages and disadvantages of three common synthesis methods for hyperbranched polymers are listed in Table 1.
By comparing the characteristics of different synthesis methods of hyperbranched polymers and the structural requirements of monomers, it can be seen that the most suitable synthesis method for industrial production is free radical polymerization because the synthesis method of hyperbranched polymers by radical polymerization has few steps and relatively low cost in the reaction process.
The limitations of the current synthesis of hyperbranched polymers mainly lie in the control of synthesis costs and the elimination of reaction amplification effects. By comparing the advantages and disadvantages, the free radical polymerization method is the most suitable for the industrial production of hyperbranched polymers because of its advantages, such as a simpler synthesis process and lower cost.
Application Progress of Hyperbranched Polymers in Oilfield Chemistry
Oil Displacement Agent
Polymers are frequently used oil displacement agents to enhance oil recovery by increasing the viscosity of the injected fluid, improving the oil–water ratio, and expanding the sweep volume of the displacement fluid (Guo et al., 2012; Liu et al., 2012). Polyacrylamide and its derivatives are widely used as oil displacement agents because of their good water solubility and viscosity enhancement (Shi et al., 2010). However, in the process of mixing and pumping, the molecular chain of polyacrylamide is broken due to shear degradation, resulting in a decrease in polymer molecular weight and solution viscosity. Obviously, its displacement effect will be greatly reduced. High temperature and high salinity conditions will aggravate the degradation of polyacrylamide. The network structure of a hyperbranched polymer can reduce the effect of shear on polymer molecular chain. The copolymerization with temperature- and salt-resistant monomers also allows the polymer to maintain effective viscosity under high temperature and salinity conditions. Therefore, hyperbranched polymers have significant advantages in polymer flooding under extreme conditions (Li et al., 2018).
Lai et al. (2013) synthesized a new hyperbranched polymer using acrylamide, acrylic acid, N-vinyl pyrrolidone, and hyperbranched functional monomer as raw materials, and the synthesis process is shown in Figure 11. The hyperbranched polymer has good temperature and salt resistance, and the viscosity retention rate is 22.89% higher than that of AM/AA copolymer at 95°C. The enhanced oil recovery was 23.51% with 1,500 mg/L polymer solution at 80°C.
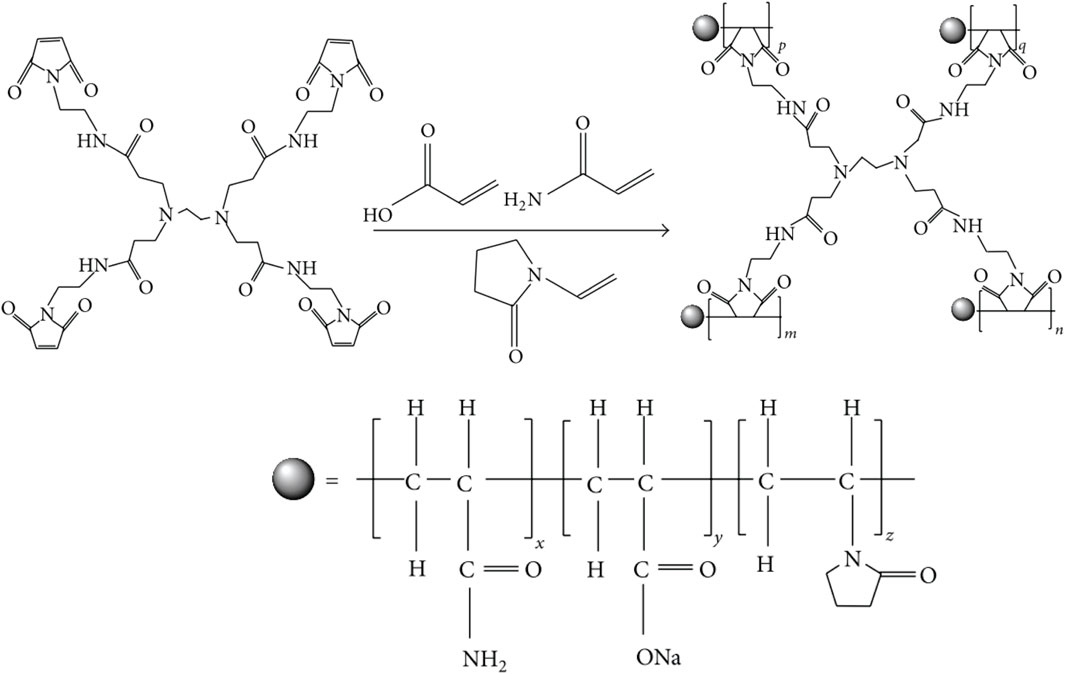
FIGURE 11. Synthesis route of the hyperbranched polymer as an oil displacement agent (Lai et al., 2013).
Offshore heavy oil development requires higher shear resistance of polymers. Chen et al. (2018) synthesized a hyperbranched polymer called HPG–HPAM–C10AM with a spherical parent nucleus and zwitterionic functional chain segment. The spherical parent nucleus is a polyether called HPG, which is obtained by the ring opening reaction of 1,1,1-trihydroxymethylpropane with glycidyl, as shown in Figure 12. Then, the copolymer HPG–HPAM–C10AM was obtained by copolymerization of acrylamide and 2-(dimethylamine)methacrylate ethyl bromide betaine with HPG as the parent nucleus. Under high shear action, the viscosity retention rate of HPG–HPAM–C10AM is about 70% and that of linear polymer HPAM is about 40%, which indicates that HPG–HPAM–C10AM has better shear resistance.
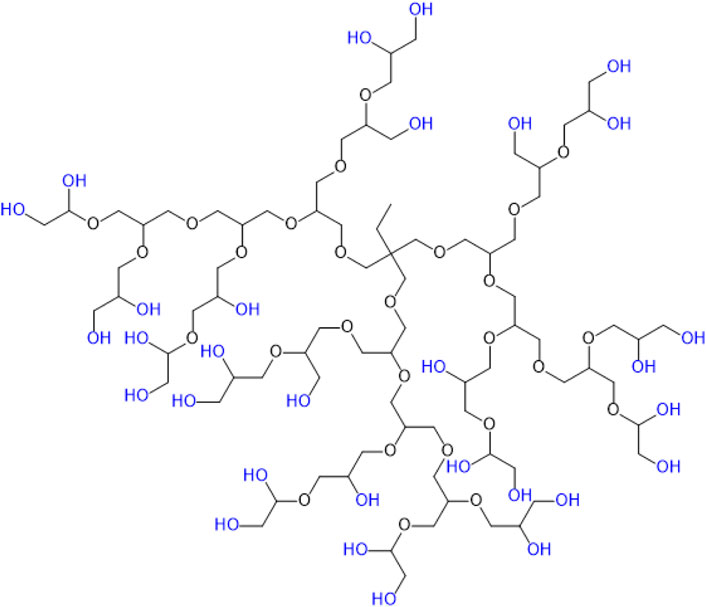
FIGURE 12. Spherical parent nucleus (HPG) (Chen et al., 2018).
Crude Oil Demulsifier
With the rapid development of water flooding, polymer flooding, and tertiary oil recovery technologies, the crude oil produced mostly exists in the form of oil-in-water (O/W) emulsion. The demulsification and dehydration of crude oil is particularly important (Liu et al., 2017). The common crude oil demulsification methods include physical demulsification, chemical demulsification, and biological demulsification, among which the chemical demulsification method is the simplest and most widely used (Yang et al., 2009; Liu et al., 2011). Most demulsifiers are amphiphilic compounds, which can adhere to the oil–water interface, reduce the interfacial tension, increase flocculation and coalescence, and promote phase separation. But these demulsifiers have many problems, such as high cost, complex modification, a large amount of addition, slow starting effect, and poor reservoir adaptability. The hyperbranched polymer is a kind of topological three-dimensional macromolecule with a highly branched structure. Its unique topological structure and modified terminal functional groups mean it has the potential to be a good demulsifier (Sun et al., 2019; Ye et al., 2019).
Zhang et al. (2018) synthesized a hyperbranched polyamidoamine called H-PAMAM with good demulsification ability by using methyl acrylate and ethylenediamine as synthetic materials through the “one-pot multi-step” method. H-PAMAM Figure 13 has a hyperbranched topological structure and abundant amino groups, so it acts quickly. The demulsification balance can be achieved within 30 min, and the oil removal rate is 91%. The product obtained by this method does not need to be purified, and the cost of synthesis is reduced.
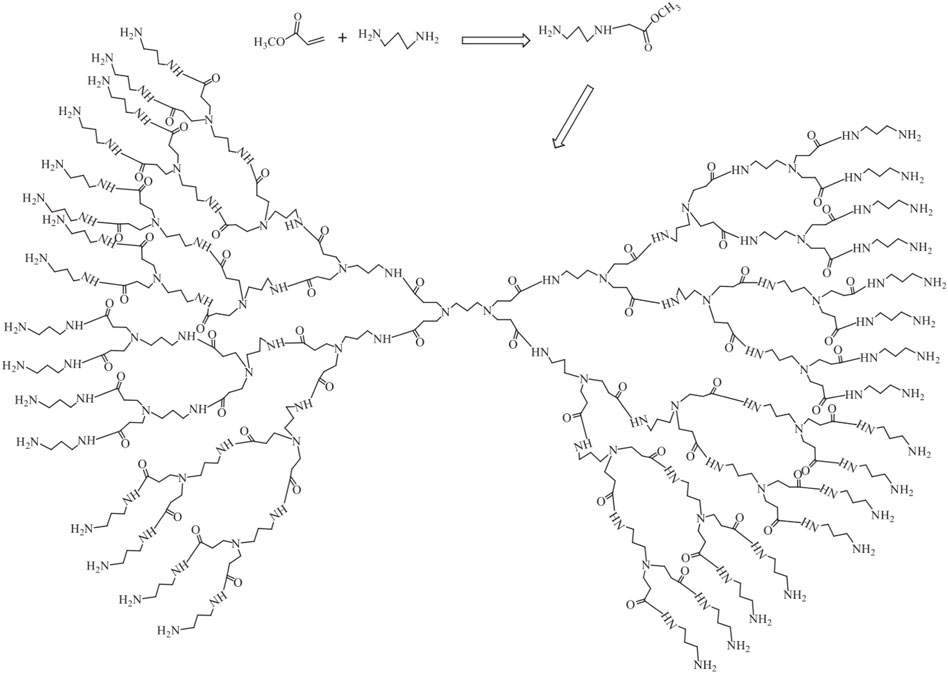
FIGURE 13. Synthesis route of H-PAMAM (Zhang et al., 2018).
In order to reduce the synthesis steps of hyperbranched polymers and production cost, Yan et al. (2020) obtained a novel core–shell amphiphilic polymer called HPEI-g-Cn with hyperbranched polyethyleneimine (HPEI) as the core and Cn as the shell through one-step synthesis. Cn is usually 10, 12, 14, 16, and 18 saturated fatty acids. The results show that, in the presence of HPEI-g-Cn, the oil–water emulsion can be completely separated into two phases within 40 min, and the oil removal rate is more than 99.9%. HPEI-g-Cn is evenly and closely distributed on the oil–water interface after demulsification, which is convenient for subsequent recycling. Therefore, HPEI-g-Cn is environmentally friendly and reduces the application cost.
Clay Stabilizer
In the process of oilfield development, clay minerals in the formation may swell, fall off, and migrate when they contact the external low salinity entry fluid. This can clog the pore channels of oil flow and reduce well productivity, especially in low-permeability reservoirs (Zou et al., 2013; Gao et al., 2014). The water sensitivity of the formation is mainly caused by clay minerals (Assemi et al., 2015; Marco-Brown et al., 2015). The commonly used clay stabilizers are mainly inorganic salts, cationic surfactants, and cationic polymers. Cationic polymer clay stabilizers are most widely used to inhibit clay swelling by forming irreversible adsorption on the surface of clay particles. The cationic polymers are mostly linear polymers with high molecular weight and easily entangled chains, which are easily absorbed and entangled in pores. Thus, pore channels are blocked, and reservoir permeability is reduced. Hyperbranched polymers have many active groups at their molecular ends, which is convenient for molecular structure design. And a clay stabilizer with low molecular weight, low entanglement rate, good solubility, low viscosity, easy film formation, and high reactivity was obtained (Morikawa and Akagi, 2012; Wang et al., 2015; Chen et al., 2018).
Feng et al. (2020) used maleic anhydride (MA) and diethanolamine (DEA) as raw materials to react to obtain a monomer called MD, which has one carboxyl group and four hydroxyl groups. Then, the polyhydroxyl group on trimethylpropane reacts with the carboxyl group on MD to obtain a hyperbranched unsaturated polyester containing multiple hydroxyl groups called HBP-OH. Through the ring opening polymerization of HBP-OH and epichlorohydrin, hyperbranched polyester amides with chloromethyl end groups called HBP-ECH were obtained. Finally, triethylamine was added to cationize the terminal group of HBP-OH to obtain a hyperbranched cationic polymer called HBP-QAT. The reaction process is shown in Figure 14. The results show that HBP-QAT compresses the electric bilayer by neutralizing the negative charge on the surface of clay particles, which strongly adsorbs on the surface of clay particles and forms a waterproof polymer film. It can prevent swelling by inhibiting water intrusion into the clay interlayer, which is scour resistant and effective for a long time. However, the synthesis process of HBP-OH is complicated and the production cost is expensive, which restricts its popularization and application.
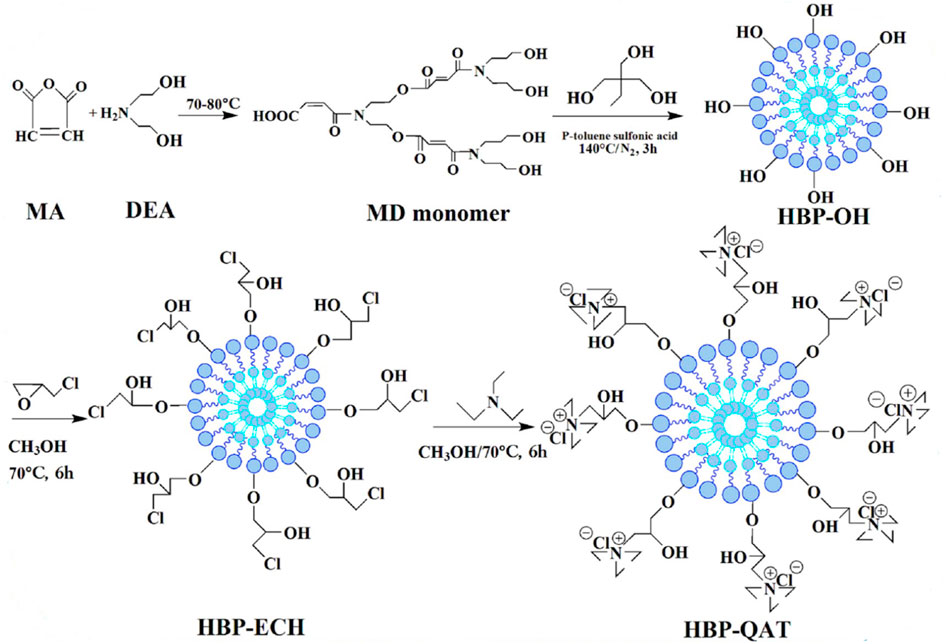
FIGURE 14. Synthesis route of HBP-QAT (Feng et al., 2020).
The hyperbranched cationic polymer has a higher cationic degree than the linear cationic polymer, so its inhibition of clay hydration expansion is more effective and lasting. Therefore, the hyperbranched cationic polymer is an ideal clay stabilizer, which can effectively reduce the permeability damage to the reservoir.
Heavy Oil Viscosity Reducer
Heavy oil is an important oil resource with abundant geological reserves all over the world (Zhang et al., 2019b). It is well known that resins and asphaltenes greatly increase the viscosity of crude oil, making flow difficult and resulting in low recovery of heavy oil. Therefore, effectively reducing the viscosity of heavy oil is the premise of enhancing oil recovery. Viscosity reduction technologies for heavy oil mainly include thermal viscosity reduction technology, diluting viscosity reduction technology, chemical viscosity reduction technology, and gas miscible oil flooding technology (Mao et al., 2017; Yang et al., 2017; Li et al., 2018; Sun et al., 2018). The thermal viscosity reduction technology consumes large amounts of energy, diluted viscosity reduction is limited by dilute oil resources in that the thin oil in oilfields with abundant heavy oil reserves is very limited, and gas miscible flooding technology has the problem of shortage of gas source. The addition of chemical viscosity reducers to reduce the viscosity of heavy oil is popular because of their high efficiency and low cost. At present, most of the reported heavy oil viscosity reducers are mainly linear polymers, but their effect on heavy oil viscosity reduction is limited. Some scholars have studied hyperbranched polymers as heavy oil viscosity reducers.
Shi et al. (2021) used third-generation polyamidoamine dendrimers (Figure 15), acrylamide, acrylic acid, and surfactants as raw materials, which are copolymerized in deionized water to form a hyperbranched polymer called HVR. The authors studied the apparent viscosity, crude oil viscosity reduction ability, interfacial tension, and other properties of HVR solution. The results show that the HVR solution has low apparent viscosity and good viscosity reduction ability for heavy oil. By comparing the asphaltene aggregation after the interaction of linear polymer (HPAM) and HVR, it was found that the interlayer spacing, interchain distance, and diameter of the aromatic layer were larger after the interaction with HVR. Due to the reaction with HVR, the aromatic cluster height and the effective number of aromatic flakes are reduced. Furthermore, the absorbance of HVR-treated asphaltenes in toluene decreases more significantly with increasing concentration compared with that of HPAM-treated asphaltenes. All of these suggest that the HVR has a better viscosity reduction effect than the linear polymer HPAM. It is worth noting that the synthesis process of the third-generation polyamidoamine dendrimers is complicated. Each generation of products is prepared by Michael addition reaction and amidation reaction, and purification operation is required after each step of reaction. So, the synthesis cost is very expensive.
Zheng et al. (2021) used octadecyl methacrylate, styrene, and ethylene glycol dimethacrylate as raw materials to synthesize a hyperbranched polymer viscosity reducer for heavy oil. The results show that the viscosity reduction rate is more than 60% at the concentration of 800 ppm. By comparing the influence of different concentrations of viscosity reducer on the settlement point, settlement amount, and asphalt particle size, it is found that adding a hyperbranched polymer viscosity reducer can increase the settlement point of asphalt and significantly reduce the settlement amount and average particle size of asphalt. In general, adding this kind of viscosity reducer can make the asphaltene precipitation have good dispersibility. The hyperbranched polymer viscosity reducer can change the polarity of asphaltene molecules, produce hydrogen bonds, disperse asphaltene molecules, and adsorb or embed an asphaltene molecular sheet, and other methods inhibit the formation of asphaltenes. The hyperbranched polymer viscosity reducer has good dispersion and inhibition effects on asphaltene precipitation. The polymer greatly reduces the synthesis cost of hyperbranched polymers while ensuring high performance.
Shale Inhibitors
Shale contains a large amount of clay minerals (sodium bentonite) and hard and brittle minerals, which are easily hydrated and swelled in contact with water-based drilling fluid during drilling, which eventually leads to wellbore instability and collapse (Al-Arfaj et al., 2014). To inhibit the hydration swelling of sodium bentonite, domestic and foreign scholars have developed a series of polymer shale inhibitors. Due to the limitation of the number of inhibited functional groups and the linear molecular structure, the adsorption of linear polymer shale inhibitors on sodium bentonite is often uneven and irregular, with relatively few adsorption sites. Under high temperature and high-speed turbulent flow conditions, the adsorption of linear polymer shale inhibitors on sodium bentonite was further weakened. Different from linear polymers, hyperbranched polymers are quasi-spherical polymers with many branched structures, and there are many terminal groups at the end of the molecular chain, which determine the chemical properties of the entire polymer molecule. Adding multiple amine groups to the end group can enhance its adsorption on clay, dehydrate the clay crystal layers, and reduce the swelling force. At the same time, the polyamine groups on the molecular chain can fix the clay wafer, destroy its hydration structure, and better exert the inhibitory effect of amine inhibitors on shale (Qu, 2009).
Ferreira et al. (2016) synthesized a non-ionic active shale inhibitor, hydrophobic hyperbranched polyglycerol, using glycerol, dimethyl carbonate, and trimethylolpropane as raw materials. In association with KCl, hydrophobic hyperbranched polyglycerol showed superior performance in comparison with unmodified hyperbranched polyglycerol, and intact cutting recoveries were around 80%. The proposed inhibition mechanism suggests the formation of a complex between hydrophobic hyperbranched polyglycerols and K+ ions and its penetration into the clay interlayer spacing to minimize the shale–water interactions and remove water molecules present in the clay galleries. In addition, these aggregates formed by the amphiphilic structures can obstruct the pore throats of clay minerals, thereby making it even more difficult for water molecules to penetrate. Although the hyperbranched polymer has excellent shale inhibition, the synthesis process needs to be completed in multiple steps, so its production cost is relatively high.
Zhang (2016) used succinic anhydride and diethylenetriamine as raw materials to synthesize a shale inhibitor with multiple amino-terminated hyperbranched polymers called HP-NH2 by a “one-pot” method. The results show that HP-NH2 has good inhibitory performance on the hydration dispersion and swelling of shale, and its inhibitory performance is enhanced with the increase of HP-NH2 content. HP-NH2 is effectively adsorbed on the surface of montmorillonite, and the unit adsorption amount can reach 0.35 g/g, and it can enter the interlayer spacing of montmorillonite and extrude the bound water by replacing the interlayer hydration cations, which can inhibit swell of montmorillonite. Compared with linear polymer amine inhibitors, HP-NH2 has more terminal amine groups, so its inhibition is better improved.
Conclusion and Prospects
It has been more than 100 years since hyperbranched polymers were proposed. With the excellent properties of hyperbranched polymers in various fields, their synthesis methods have also been developed from traditional condensation polymerization, ring opening polymerization, and free radical polymerization to click chemistry, cross-coupling reaction method, dipole cycloaddition reaction method, coupling monomer method, and other new synthesis methods, and each synthesis method has its advantages and scope of application. Scholars have also innovatively developed monomers used in different synthesis methods. Hyperbranched polymers have many advantages that linear polymers do not have. It is also the key research direction of hyperbranched polymers to explore the potential properties of hyperbranched polymers and further expand their application fields.
Hyperbranched polymers have the characteristics of temperature resistance, shear resistance, and easy modification. In view of the high temperature and high salinity conditions in oilfield development, the highly branched structure of hyperbranched polymers can provide enough sites for grafting different groups to improve the performance of various chemical additives. However, the application of hyperbranched polymers also has some limitations. The limitations of the application of hyperbranched polymers in oilfield chemistry are mainly the cost of synthesis and the amplification effect of the reaction. The research on hyperbranched polymers in oilfield chemistry is still in the stage of laboratory synthesis. To expand production and application, the two problems of synthesis cost and amplification effect must be solved.
In the field of oilfield chemistry, the application of hyperbranched polymers goes far beyond oil displacement agents, crude oil demulsifiers, clay stabilizers, and shale inhibitors. Studies have shown that hyperbranched polymers form a network structure in an aqueous solution, and the multi-terminal structure can introduce adsorption groups and charged ions, which can be used as efficient water treatment agents for the treatment of oilfield wastewater. Based on the hyperbranched polymer as an oil displacement agent, amphiphilic groups can be introduced to synthesize hyperbranched polymer surfactants, and the use of hyperbranched polymer surfactants as oil displacement agents is expected to greatly improve oil recovery. Hyperbranched polymers have low viscosity properties and unique network structures. After being modified by other functional monomers, it can be used as a rheology modifier for water-based drilling fluids to improve the rheology of drilling fluids. The unique properties of hyperbranched polymers make them have broad application prospects in the fields of drilling fluid chemistry, oil production chemistry, and gathering and transportation chemistry in the field of oilfield chemistry.
Author Contributions
WY and YZ conceptualized the research idea and investigated the data. YZ wrote the original draft.
Conflict of Interest
The authors declare that the research was conducted in the absence of any commercial or financial relationships that could be construed as a potential conflict of interest.
Publisher’s Note
All claims expressed in this article are solely those of the authors and do not necessarily represent those of their affiliated organizations, or those of the publisher, the editors, and the reviewers. Any product that may be evaluated in this article, or claim that may be made by its manufacturer, is not guaranteed or endorsed by the publisher.
References
Al-Arfaj, M. K., Amanullah, M., Sultan, A. S., Hossain, E., and Abdulraheem, A. (2014). “Chemical and Mechanical Aspects of Wellbore Stability in Shale Formations: A Literature Review,” in Abu Dhabi International Petroleum Exhibition and Conference, Abu Dhabi, UAE, November 10–13, 2014. Abu Dhabi. doi:10.2118/171682-ms
Assemi, S., Sharma, S., Tadjiki, S., Prisbrey, K., Ranville, J., and Miller, J. D. (2015). Effect of Surface Charge and Elemental Composition on the Swelling and Delamination of Montmorillonite Nanoclays Using Sedimentation Field-Flow Fractionation and Mass Spectroscopy. Clays Clay Min. 63 (6), 457–468. doi:10.1346/ccmn.2015.0630604
Chen, Y., Wang, N., Tong, G., Wu, D., Jin, X., and Zhu, X. (2018). Synthesis of Multiarm Star Polymer Based on Hyperbranched Polyester Core and Poly(ε-Caprolactone) Arms and its Application in UV-Curable Coating. ACS Omega 3 (10), 13928–13934. doi:10.1021/acsomega.8b02128
Feng, Q., Liu, H., Peng, Z. G., and Zheng, Y. (2020). Synthesis, Characterization and Evaluation of Long-Acting Hyperbranched Cationic Polymer Clay Stabilizer Used in Water Flooding. Polym. Test., 82, 106344. doi:10.1016/j.polymertesting.2020.106344
Ferreira, C. C., Teixeira, G. T., Lachter, E. R., and Nascimento, R. S. V. (2016). Partially Hydrophobized Hyperbranched Polyglycerols as Non-ionic Reactive Shale Inhibitors for Water-Based Drilling Fluids. Appl. Clay Sci. 132-133, 122–132. doi:10.1016/j.clay.2016.05.025
Flory, P. J. (1952). Molecular Size Distribution in Three Dimensional Polymers. VI. Branched Polymers Containing A-R-Bf-1 Type Units. J. Am. Chem. Soc. 74, 2718–2723. doi:10.1021/ja01131a008
Fréchet, J. M. J., Henmi, M., Gitsov, I., Aoshima, S., Leduc, M. R., and Grubbs, R. B. (1995). Self-condensing Vinyl Polymerization: an Approach to Dendritic Materials. Science 269 (5227), 1080–1083. doi:10.1126/science.269.5227.1080
Gan, W., Xu, H., Jin, X., Cao, X., and Gao, H. (2020). Recyclable Palladium-Loaded Hyperbranched Polytriazoles as Efficient Polymer Catalysts for Heck Reaction. ACS Appl. Polym. Mat. 2 (2), 677–684. doi:10.1021/acsapm.9b01011
Gao, C., and Yan, D. (2004). Hyperbranched Polymers: from Synthesis to Applications. Prog. Polym. Sci. 29 (3), 183–275. doi:10.1016/j.progpolymsci.2003.12.002
Gao, Y., Zhao, M., Wang, J., and Zong, C. (2014). Performance and Gas Breakthrough during CO2 Immiscible Flooding in Ultra-low Permeability Reservoirs. Petroleum Explor. Dev. 41 (1), 88–95. doi:10.1016/s1876-3804(14)60010-0
Guo, Z., Dong, M., Chen, Z., and Yao, J. (2012). Dominant Scaling Groups of Polymer Flooding for Enhanced Heavy Oil Recovery. Ind. Eng. Chem. Res. 52 (2), 911–921. doi:10.1021/ie300328y
Kim, Y. H., and Webster, O. W. (1992). Hyperbranched Polyphenylenes. Macromolecules 25 (21), 5561–5572. doi:10.1021/ma00047a001
Lai, N. J., Qin, X. P., Ye, Z. B., Peng, Q., Zhang, Y., and Ming, Z. (2013). Synthesis and Evaluation of a Water-Soluble Hyperbranched Polymer as Enhanced Oil Recovery Chemical. J. Chem. 2013, 824785. doi:10.1155/2013/824785
Li, X., Shi, L., Li, H., Liu, P., Luo, J., and Yuan, Z. (2018). Experimental Study on Viscosity Reducers for SAGD in Developing Extra-heavy Oil Reservoirs. J. Petroleum Sci. Eng. 166, 25–32. doi:10.1016/j.petrol.2018.03.022
Li, Z. H., Dong, P. H., Yue, M., and Liu, H. (2019). Preparation and Anti-swelling Properties of A Hyperbranched Cationic Polymer. Chin. J. .Syn. Chem. 27 (10), 788
Liu, J., Huang, X. F., Lu, L. J., Li, M. X., Xu, J. C., and Deng, H. P. (2011). Turbiscan Lab ® Expert Analysis of the Biological Demulsification of a Water-In-Oil Emulsion by Two Biodemulsifiers. J. Hazard Mater 190 (1-3), 214–221. doi:10.1016/j.jhazmat.2011.03.028
Liu, J., Wang, H., Li, X., Jia, W., Zhao, Y., and Ren, S. (2017). Recyclable Magnetic Graphene Oxide for Rapid and Efficient Demulsification of Crude Oil-In-Water Emulsion. Fuel 189, 79–87. doi:10.1016/j.fuel.2016.10.066
Liu, X.-J., Jiang, W.-C., Gou, S.-H., Ye, Z.-B., and Xie, X.-D. (2012). Synthesis and Evaluation of a Water-Soluble Acrylamide Binary Sulfonates Copolymer on MMT Crystalline Interspace and EOR. J. Appl. Polym. Sci. 125 (2), 1252–1260. doi:10.1002/app.35299
Mao, J., Liu, J., Wang, H., Yang, X., Zhang, Z., Yang, B., et al. (2017). Novel Terpolymers as Viscosity Reducing Agent for Tahe Super Heavy Oil. RSC Adv. 7 (31), 19257–19261. doi:10.1039/c7ra00508c
Marasini, N., Fu, C., Fletcher, N. L., Subasic, C., Er, G., Mardon, K., et al. (2020). The Impact of Polymer Size and Cleavability on the Intravenous Pharmacokinetics of PEG-Based Hyperbranched Polymers in Rats. Nanomater. (Basel) 10 (12), 22452. doi:10.3390/nano10122452
Marco-Brown, J. L., Trinelli, M. A., Gaigneaux, E. M., Torres Sánchez, R. M., and dos Santos Afonso, M. (2015). New Insights on the Structure of the Picloram-Montmorillonite Surface Complexes. J. Colloid Interface Sci. 444, 115–122. doi:10.1016/j.jcis.2014.12.045
Morikawa, A., and Akagi, M. (2012). Hyperbranched Poly(ether Ether Ketone)s: Preparation and Comparison of Properties with the Corresponding Dendrimers. Polym. J. 45 (6), 614–621. doi:10.1038/pj.2012.188
Qu, Y. Z. (2009). Development and Evaluation of Shale Inhibitor SIAT. Pet. Drill. Tech. 37 (6) 53–57. doi:10.3969/j.issn.1001-0890.2009.06.012
Rousseau, G., Fensterbank, H., Baczko, K., Cano, M., Stenger, I., Larpent, C., et al. (2013). Synthesis of Clickable Water-Soluble Poly(amidoamine) Fullerodendrimers and Their Use for Surface Functionalization of Azido-Coated Polymer Nanoparticles. ChemPlusChem 78 (4), 352–363. doi:10.1002/cplu.201300045
Shi, L., Liu, C., Chen, M., Hua, Z., Ye, Z., and Zhang, J. (2021). Synthesis and Evaluation of a Hyperbranched Copolymer as Viscosity Reducer for Offshore Heavy Oil. J. Petroleum Sci. Eng. doi:10.1016/j.petrol.2020.108011
Shi, L., Ye, Z., Zhang, Z., Zhou, C., Zhu, S., and Guo, Z. (2010). Necessity and Feasibility of Improving the Residual Resistance Factor of Polymer Flooding in Heavy Oil Reservoirs. Pet. Sci. 7, 251–256. doi:10.1007/s12182-010-0029-5
Shimizu, K., Metivier, R., and Kobatake, S. (2020). Synthesis and Fluorescence On/off Switching of Hyperbranched Polymers Having Diarylethene at the Branching Point. J. Photochem. Photobiol. a-Chemistry, 390, 112341. doi:10.1016/j.jphotochem.2019.112341
Sun, H., Lei, X., Shen, B., Zhang, H., Liu, J., Li, G., et al. (2018). Rheological Properties and Viscosity Reduction of South China Sea Crude Oil. J. Energy Chem. 27 (4), 1198–1207. doi:10.1016/j.jechem.2017.07.023
Sun, Y., Lu, F., Yang, H., Ding, C., Yuan, Z., and Lu, C. (2019). Fluorescent Sensor Array for Separation-free Dopamine Analogue Discrimination via Polyethyleneimine-Mediated Self-Polymerization Reaction. Nanoscale 11 (27), 12889–12897. doi:10.1039/c9nr03643a
Suzuki, M., Ii, A., and Saegusa, T. (1992). Multibranching Polymerization: Palladium-Catalyzed Ring-Opening Polymerization of Cyclic Carbamate to Produce Hyperbranched Dendritic Polyamine. Macromolecules 25 (25), 7071–7072. doi:10.1021/ma00051a055
Suzuki, M., Yoshida, S., Shiraga, K., and Saegusa, T. (1998). New Ring-Opening Polymerization via a π-Allylpalladium Complex. 5. Multibranching Polymerization of Cyclic Carbamate to Produce Hyperbranched Dendritic Polyamine. Macromolecules 31 (6), 1716–1719. doi:10.1021/ma971222t
Wang, G. Y., Wen, S. G., Qian, S. P., Wang, J. H., Wang, C. R., and Chen, Y. B. (2020). Synthesis of Novel Nano Hyperbranched Polymer Resin and its Corrosion Resistance in Coatings. Prog. Org. Coatings, 140, 105496. doi:10.1016/j.porgcoat.2019.105496
Wang, Y., Song, X., Tian, C., Shi, C., Li, J., Hui, G., et al. (2015). Dynamic Fractures Are an Emerging New Development Geological Attribute in Water-Flooding Development of Ultra-low Permeability Reservoirs. Petroleum Explor. Dev. 42 (2), 247–253. doi:10.1016/s1876-3804(15)30012-4
Wei, Z., Wang, D., Liu, Y., Guo, X., Zhu, Y., Meng, Z., et al. (2020). Ferrocene-based Hyperbranched Polymers: a Synthetic Strategy for Shape Control and Applications as Electroactive Materials and Precursor-Derived Magnetic Ceramics. J. Mat. Chem. C 8 (31), 10774–10780. doi:10.1039/d0tc01380c
Chen, W. J., Wang, X. J., and Hu, K. (2018). Synthesis and Properties of Polyacrylamide Polymer Flooding Agent with High Shear Resistance. J. Xi'an Shiyou Univ. Nat. Sci. Ed. 33 (7), 105–111. doi:10.3969/j.issn.1673-064X.2018.06.017
Wörl, R., and Köster, H. (1999). Synthesis of New Liquid Phase Carriers for Use in Large Scale Oligonucleotide Synthesis in Solution. Tetrahedron 55 (10), 2941
Xiao, L., Liu, Z., Li, N., Li, S., Fu, P., Wang, Y., et al. (2020). A Hyperbranched Polymer from Tung Oil for the Modification of Epoxy Thermoset with Simultaneous Improvement in Toughness and Strength. New J. Chem. 44 (39), 16856–16863. doi:10.1039/c9nj06373k
Xing, A., Sun, Q. N., Meng, Y., Zhang, Y. Y., Li, X. Y., and Han, B. (2020). A Hydroxyl-Containing Hyperbranched Polymer as a Multi-Purpose Modifier for a Dental Epoxy. React. Funct. Polym., 149, 104505. doi:10.1016/j.reactfunctpolym.2020.104505
Yan, D., and Gao, C. (2000). Hyperbranched Polymers Made from A2 and BB'2 Type Monomers. 1. Polyaddition of 1-(2-Aminoethyl)piperazine to Divinyl Sulfone. Macromolecules 33, 7693–7699. doi:10.1021/ma000438j
Yan, S., He, G., Ye, D., Guo, Y., and Fang, W. (2020). Amphiphilic Hyperbranched Polyethyleneimine for Highly Efficient Oil-Water Separation. J. Mat. Chem. A 8 (5), 2412–2423. doi:10.1039/c9ta09640j
Yang, X.-g., Tan, W., and Tan, X.-f. (2009). Demulsification of Crude Oil Emulsion via Ultrasonic Chemical Method. Petroleum Sci. Technol. 27 (17), 2010–2020. doi:10.1080/10916460802637577
Yang, Y., Guo, J., Cheng, Z., Wu, W., Zhang, J., Zhang, J., et al. (2017). New Composite Viscosity Reducer with Both Asphaltene Dispersion and Emulsifying Capability for Heavy and Ultraheavy Crude Oils. Energy fuels. 31 (2), 1159–1173. doi:10.1021/acs.energyfuels.6b02265
Ye, D., Mi, J., Bai, S., Zhang, L., Guo, Y., and Fang, W. (2019). Thermal Cracking of Jet Propellant-10 with the Addition of a Core-Shell Macroinitiator. Fuel, 254. doi:10.1016/j.fuel.2019.115667
Ye, W., Zaheer, M., Li, L., Wang, J., Xu, H., Wang, C., et al. (2020). Hyperbranched PCL/PS Copolymer-Based Solid Polymer Electrolytes Enable Long Cycle Life of Lithium Metal Batteries. J. Electrochem. Soc. 167 (119PP), 110532. doi:10.1149/1945-7111/aba3fd
Zhang, F. S., Liu, G. L., Li, X. N., Zhu, Z. Y., and Su, H. M. (2019). “Research and Existing Problems of Pour Point Depressant/Viscosity Reducer for Crude Oil Production,” in IOP Conference Series-Materials Science and Engineering, Sanya, China, November 17–19, 2018. doi:10.1088/1757-899x/479/1/012062
Zhang, H. B. (2016). Synthesis and Performance Evaluation of Amino-Terminated Hyperbranched Polymer as Shale Inhibitor. Petrochem. Technol. 45 (9), 1081–1087. doi:10.3969/j.issn.1000-8144.2016.09.010
Zhang, K. N., Yang, H. T., Li, M. M., Li, J., Wu, W. B., Xu, S. G., et al. (2020). A Hyperbranched Polymer with Enhanced Photorefractive Effect at Low and Zero Applied Electric Field. Dyes Pigments, 180, 108473. doi:10.1016/j.dyepig.2020.108473
Zhang, L., Ying, H., Yan, S., Zhan, N., Guo, Y., and Fang, W. (2018). Hyperbranched Poly(amido Amine) Demulsifiers with Ethylenediamine/1,3-Propanediamine as an Initiator for Oil-In-Water Emulsions with Microdroplets. Fuel 226, 381–388. doi:10.1016/j.fuel.2018.03.196
Zhang, R., Li, S., Liu, X., and Zhang, H. (2019). Development of a Nitrogen-Rich Hyperbranched Polymer as Adsorbent for Enrichment and Determination of Auxins in Plants. Anal. Bioanal. Chem. 411 (7), 1409–1419. doi:10.1007/s00216-018-01571-7
Zhang, S., Ding, F., Li, Z., Ren, X., and Huang, T.-S. (2020). Antibacterial PET Fabrics Modified with Quaternary Ammonium Functionalized Hyperbranched Polymers via Electron Beam Radiation. Fibers Polym. 21 (10), 2285–2291. doi:10.1007/s12221-020-1251-4
Zhang, Y., Li, X., Zhu, Q., Wei, W., and Liu, X. (2020). Photocurable Hyperbranched Polymer Medical Glue for Water-Resistant Bonding. Biomacromolecules 21 (12), 5222–5232. doi:10.1021/acs.biomac.0c01302
Zheng, C., Fu, H., Wang, Y., Zhang, T., huang, Z., and Duan, W. (2021). Preparation and Mechanism of Hyperbranched Heavy Oil Viscosity Reducer. J. Petroleum Sci. Eng., 196. doi:10.1016/j.petrol.2020.107941
Keywords: hyperbranched polymers, dendrimers, synthesis, oilfield chemistry, application prospects
Citation: Zhang Y and Yu W (2022) Synthesis of Hyperbranched Polymers and Prospects for Application in Oilfield Chemistry. Front. Energy Res. 10:894096. doi: 10.3389/fenrg.2022.894096
Received: 11 March 2022; Accepted: 19 April 2022;
Published: 31 May 2022.
Edited by:
Qi Zhang, China University of Geosciences Wuhan, ChinaReviewed by:
Yongpeng Sun, China University of Petroleum (East China), ChinaHu Guo, China University of Petroleum, China
Copyright © 2022 Zhang and Yu. This is an open-access article distributed under the terms of the Creative Commons Attribution License (CC BY). The use, distribution or reproduction in other forums is permitted, provided the original author(s) and the copyright owner(s) are credited and that the original publication in this journal is cited, in accordance with accepted academic practice. No use, distribution or reproduction is permitted which does not comply with these terms.
*Correspondence: Weichu Yu, yuweichu@126.com