- 1School of Civil Engineering, Qingdao University of Technology, Qingdao, China
- 2Department of Civil and Architectural Engineering, Qingdao University of Technology (School of Linyi), Linyi, China
Since entering the new era, the economy has grown rapidly and the economic strength has improved significantly, but it is accompanied by resource and environmental problems caused by the high pollution and high energy consumption growth model. Realizing the transformation of economic development from “incremental” to “increasing quality” and promoting economic green transformation has become one of the main development goals of China in the next stage. With the rapid development of China’s social economy, the changes of industrialization, urbanization and agricultural intensification are getting faster and faster. The discharge of industrial “three wastes” and urban domestic wastewater has gradually increased, and a large amount of chemical fertilizers and pesticides have been applied to rural arable land. It accelerates soil heavy metal pollution. Electrodynamic remediation technology, as an emerging soil in situ remediation technology, has attracted wide attention due to its advantages of wide range of pollutant removal, low cost and good effect. In this paper, a remediation device for heavy metal-contaminated soil is designed based on electrodynamic remediation technology. That is, on the basis of the digital economy, an electrolyte circulation mechanism with high adsorption of heavy metal ions is introduced. In this paper, the feasibility of electrokinetic remediation of the contaminated soil was investigated, and the effects of electrolyte type, voltage, and remediation time on the removal of heavy metals were studied. The following results were obtained through a series of experimental studies: the pH values of region A in E1, E2, and E3 were 3.24, 3.18, and 3.09, respectively. The current peak values of E1, E2 and E4 are 0.44, 0.68 and 0.75 mA respectively. E4 had the best adsorption effect on heavy metal cadmium ions, with an average adsorption rate of 7.3%. The results show that the electrodynamic remediation device can effectively remove heavy metals in soil. It can also recycle the electrolyte solution, which is an environmentally friendly repair device.
Introduction
As the core of the new round of industrial revolution, the digital economy is a breakthrough for the economy to achieve green transformation. Its green, diverse and shared characteristics provide a possible path for cultivating new kinetic energy for economic development. In recent years, China’s digital economy industry has developed rapidly. From a domestic perspective, in 2019, the scale of the digital economy industry has increased from 2.6 trillion yuan in 2005 to 35.8 trillion yuan, accounting for 36.2% of GDP, an increase of 22 percentage points from 2005. Its growth rate is much higher than that of GDP, and it has become the main force driving the economy to shift gears. From an international perspective, it has become a major leader in the global digital economy industry, second only to the United States. The combined market value of digital platforms in China and the United States accounts for 90% of the total market value of the top 70 digital platforms in the world. Two of the world’s seven “super platforms” in the digital economy are companies, namely Alibaba and Tencent. The new industrial network chain structure formed under the digital background has promoted the deconstruction and reorganization of the existing industrial chain. Compared with the traditional industrial chain, it has the characteristics of being safer, more stable and more efficient, which provides a new idea and feasible path to optimize the industrial structure and achieve green development.
With the deterioration of human economic activities and production environment, soil pollution around the world has become increasingly serious, which has seriously affected the ecological structure and functional stability of soil. At present, many factories have left the urban area, and the old sites left behind are polluted and not suitable for planting plants or human life. And some oil refining and oil extraction factories have caused land pollution due to the leakage of crude oil. Due to the long-term industrialization, the “three wastes” (wastewater, waste gas, and waste residue) enter the soil through water bodies and the atmosphere. When accumulated to a certain extent, the ecological function of the soil will be degraded, which will have a certain impact on crops and groundwater, thereby reducing the yield and quality of food, thus posing a threat to human health. With people’s understanding of the importance of soil, soil heavy metal pollution has gradually become a hot spot in environmental science research. There are few studies on simulated soil pollution and remediation equipment in China, and less equipment used to study soil pollution diffusion and remediation laws. The research results of this paper enrich the research in this field. Based on the research on soil remediation technology and equipment at home and abroad, this paper fits the process of simulated soil remediation. It includes soil crushing, screening, simulated repair test, and test parameter monitoring on the soil to be tested. In this paper, the designed electrodynamic repair device is experimentally studied to demonstrate its feasibility. The device can be used for the remediation technology of soil heavy metal pollution, and provides a certain reference for the scientific research workers of soil pollution control.
Related Work
In terms of theoretical research, experts and scholars at home and abroad have discussed the application and development prospects of the digital economy in various fields. This paper has done relevant research on various aspects and applications of the digital economy. Simon C proposes a representative measurement method to quantify the digital economy. The comparison is made by measuring the market capitalization of selected countries using a financial database, which measures and compares the digital economy between countries. The results show that the U.S. leads in every way, with the 11 largest companies by market capitalization all coming from the U.S. Germany should take policy measures to increase its competitiveness in this field. This approach can be applied to different countries seeking to benchmark their economic performance and formulate policy measures to keep their digital economy ahead of others (Simon et al., 2017). Drahokoupil J discusses the impact of the digital economy on the workforce. According to him, the digitization of the economy refers to how ICTs change the goods and services we produce, how they are produced, and where they are produced. This involves many aspects of society: how labor is exchanged on digital platforms and how consumers become producers (so-called prosumers), how mass production can be repurposed into local micro-production, how underutilized assets can be shared up close to zero marginal cost, how companies can re-examine their decisions about where to produce in light of the use of robots, and how new monopolies can emerge. The most important point is the impact of big data on the economic structure (Drahokoupil and Jepsen, 2017). Teoh C S examines national cybersecurity strategies for the digital economy. It aims to gain an in-depth understanding of the relationship between the development of national cybersecurity and the digital economy based on literature such as journal articles, global reports, current industry dynamics and market trends. He analyzed the cybersecurity of nine countries centered on the digital economy, and found that countries with a high degree of digital informatization rely less on national cybersecurity to strengthen confidence in the digital space. And the top ten countries in the existing digital economy have all implemented national cybersecurity strategies, providing the necessary foundation for the further vigorous development of the digital economy (Teoh and Mahmood, 2017). Alizadeh T discussed urban governance and company development in the digital economy from three different perspectives, combined with a specific case - the launch of Google Fiber in Kansas City. First, he scrutinized urban governance and the Fiber project. He highlighted the numerous regulatory concessions and incentives offered to Fiber during the construction phase. Second, he explores how pre-existing digital divides and socioeconomic inequalities affect Kansas City’s fibre plans. Finally, he discusses the significant policy implications of projects such as Fiber on urban governance, emphasizing the stark realities facing such businesses (Alizadeh et al., 2017). Markova O V studies corporate innovation activities in the context of the digital economy from both economic and legal perspectives. He pointed out that the innovation activities of enterprises also include the development of artificial intelligence and robotics, and the innovative behavior of enterprises also includes artificial intelligence and robotics. Under the existing artificial intelligence technology and application conditions, it is particularly important to ensure the security of the country in the context of digitalization. In this context, the strategic goal of ensuring information security is to protect the vital interests of individuals and society from internal and external threats related to the application of information technology for various purposes in violation of civil law. Therefore, innovation will increase the investment attractiveness of enterprises, and enterprises should maintain a balance between creative freedom and internal control measures. It self-regulates in the field of digital technology and establishes a unified legal framework in the economic field (Markova et al., 2021). Leahovcenco A discusses issues related to the definition of cybersecurity and its elements in order to address current cyber threats and reduce the use of malware and complex infrastructure. And he queried a lot of data about cyber attacks. He used correlation analysis to analyze the closeness of the relationship between the proportion of the digital economy in GDP and the GCI index, and proposed an improvement method (Leahovcenco, 2021). Miao Z breaks the traditional research on digital economy and value chain. He proposed the concept of digital economy value chain, and discussed its connotation, value activity composition, and characteristics of value chain. The value chain of the digital economy is driven by digital elements (data, digital technology and digital models), and is a combination of the digital economy and value chain. It is based on digital elements, and its essence is a chain based on the value creation of the digital economy. In the value chain of the digital economy, each value-added link can generate value, and the flow of the value chain is the process of value generation and transmission (Miao, 2021).
Design Technology of Soil Remediation Device
Digital Economy
The concept of green development aims to promote the formation of a high-efficiency, low-pollution, and low-loss green economic growth model in the region. With the accelerated development of the digital economy, the influence of informatization and digitalization has been integrated into all aspects of economic development, and has had a significant impact on the social and economic growth mechanism. The digital economy is characterized by its interconnection and sharing, element innovation, breaking space constraints and near-zero cost. It effectively removes various obstacles to the upgrading of the industrial structure in the process of green development (Jepsen and Drahokoupil, 2017; Gulamov et al., 2021).
According to the nature and characteristics of the digital economy, the ways in which it affects regional green development can be divided into two categories. One is value reshaping, that is, the digital economy empowers the economic development process. It reshapes the way the economic system operates through its characteristics such as information dissemination across time and space, so as to improve the operating efficiency of the economy. The second is value creation, that is, the economic benefits brought about by the digital industry itself that has evolved from the digital economy. The classification of the digital economy is shown in Figure 1. Under the path of value reshaping, the digital economy is mainly used for regional green development through mechanisms such as information exchange, innovation efficiency, and non-spatial industrial agglomeration (Putrik, 2021). The development of the digital economy has improved the efficiency of information interaction and can optimize product matching and transactions. This is mainly due to the aggregation of fragmented demand information and supply information by the digital economy platform built by digital technology. It greatly reduces the search cost of enterprises and users, so that demand and supply are fully mobilized, thereby improving the efficiency of social resource allocation. The digital economy can break through the spatial limitations of industrial agglomeration and realize ultra-long-distance industrial agglomeration. In this way, the externalities of agglomeration can be used to improve the utilization efficiency of regional energy and resources, realize a circular economy, and then realize the transformation of economic green. Under the path of value creation, the digital economy mainly affects the economic structure through data, a key production factor, and promotes the green transformation of the economic structure. Human capital, material input and technological progress are the three factors that determine regional economic growth in the traditional economic growth model. Since the information revolution, data, an important product of the information age, has gradually evolved into the fourth element of economic growth. On the one hand, data itself as a factor of production gave birth to the information industry. The information industry has the characteristics of green industry such as rapid update, strong permeability, high income, zero marginal cost and no pollution discharge. The increase in the scale of the added value of the digital economy in the industrial structure of an economy is itself an optimization of the industrial structure, which can effectively promote the transformation of the economic structure to a green model (Makarova, 2021). On the other hand, the digital economy plays an important role in promoting the development of the financial industry, the upgrading of traditional industries and the optimization of systems, which is in line with the development direction of the concept of green development. Due to the characteristics of high risk and high investment in the digital economy, a large amount of financial support is required for R&D, promotion and post-maintenance operations. Therefore, the development of the digital industry will promote the development of the financial industry (Ogli, 2021).
Remediation of Heavy Metal Contaminated Soil
Since the 1960s, chemical waste in developed countries such as the Netherlands and the United States has caused serious soil pollution. So far, soils on five continents have been seriously polluted, with Europe being the main one, followed by Asia and the Americas. With the acceleration of industrialization, urbanization and rural intensification, the quality of land environment is deteriorating day by day, and the scope of land pollution is increasing, which seriously affects the strategic goal of national sustainable development. With the rapid economic development at home and abroad, the utilization rate of resources has been continuously improved, and the area of polluted land has also increased accordingly. Currently, some industrial cities have been redesigned to move heavy industrial parks out of urban areas. It causes a large amount of industrial waste water, waste gas, waste residue and so on, such as heavy metals, radioactivity, organic matter and other pollutants. Soil pollution will not only have a great impact on soil quality and productivity. It will also lead to the deterioration of the ecological environment such as water and air, and even pose a direct threat to human health and the ecological environment (Jepsen and Drahokoupil, 2017; Elder-Vass, 2021). Figure 2 shows a large area of soil contamination.
According to the national ambient air quality monitoring data in 2020, the proportion of heavy metals in soil exceeding the standard is 16.1%, and the arable land exceeds the standard by 19.4%. The pollution degree in the southern region is relatively high. The soil pollution in the Pearl River Delta region, the Yangtze River Delta region and the old industrial base in Northeast China is relatively serious. Tables 1, 2 are the main indicators of inorganic pollutants in Chinese soil, as well as the soil environmental quality of different land use patterns.
In recent years, a lot of research has been done on the treatment technologies and methods of various polluted soils at home and abroad. Some countries have formed systematic restoration technologies and accumulated a lot of treatment experience. At present, the treatment of polluted soil at home and abroad mainly adopts the following methods:
(1) Physical repair method
Physical repair methods are mainly physical repair, including soil replacement method, thermal repair method, vitrification method, electrical repair method, etc. (Liu, 2018). The soil replacement method is to replace or partially replace the contaminated land with clean, uncontaminated land, and use the uncontaminated land to reduce the concentration of pollution, thereby repairing soil pollution. Thermal remediation technology is to heat the polluted soil, commonly used heating methods include steam, radio frequency, infrared, microwave, etc, and then collect the flammable substances in the soil for reuse. The so-called “vitrification” is to put the polluted soil in a high temperature and high pressure environment, convert it into glass, and adsorb it in it, thus greatly reducing the pollution of heavy metals. Electroremediation technology is to remediate the soil by migrating the direction of pollutants or heavy metals into the cathode chamber by electrophoresis and electroosmosis on the polluted land.
(2) Chemical repair method
Chemical remediation is the use of chemical methods to chemically treat soil to achieve the purpose of soil remediation, mainly including leaching method, extraction method, etc. The leaching method is to use the injected water to flush out the impurities infiltrated into the soil, so that the flushing water flow infiltrated into the soil can be effectively restored. Extraction converts contaminated substances into soluble complexes. It chemically reacts with pollutants contained in the soil. Finally, physicochemical methods are used to separate the contaminants from the mixed extract and recover them (Watanabe et al., 2018).
(3) Bioremediation
Bioremediation methods are based on biological ventilation and phytoremediation. So-called bio-ventilation, air is drawn into contaminated land and then pulled away. It is then discharged into the atmosphere or passed through exhaust gas treatment equipment for further treatment (Paul, 2017). The so-called phytoremediation method is to use plants to absorb heavy metal elements in the polluted land and then extract them. The enrichment is transferred to the branching and harvesting places on the plant, or it uses its unique microbial and enzymatic system to combine heavy metals in the contaminated soil to reduce the toxicity of organisms and the activity of heavy metals (Gasiorek et al., 2017). It reduces the diffusion of heavy metals in the air or prevents heavy metals from flowing into groundwater during the leaching process.
At present, some research results have been achieved in soil treatment technology research. However, there is a big gap with the advanced countries in the world in terms of technical level and large-scale application of equipment (Ying et al., 2018). There are serious deficiencies in China’s independent research and development in in-situ and ex-situ repair technologies and equipment. Research on soil remediation technology, with its unique soil type, conditions and site conditions, determines that it must be developed and adapted to national conditions. It has practical equipment and technology with independent intellectual property rights. This is not only the need for soil environmental protection and technology industrialization, but also the need for a new round of international environmental remediation market competition in the field of soil pollution control. The data shows that soil remediation equipment is mainly based on large-scale equipment with strong processing capacity, supplemented by small-scale equipment with strong flexibility. The functions of the equipment used to study soil remediation are relatively simple (Zhao et al., 2020). Figure 3 shows some large soil remediation equipment.
Electric Power Repair Technology
People have discovered and exploited electric power technology for hundreds of years. Initially, electrodynamic remediation was applied to dams and soil dehydration. With the progress of human beings and the development of science and technology, electrodynamic remediation technology has been applied to the remediation of polluted soil (Yang et al., 2018). The basic principle of electrodynamic remediation of polluted soil is: select the appropriate electrode material, insert or bury the electrode in the polluted soil, select the appropriate voltage strength and energize, and an electric field is formed between the cathode and anode electrodes. Under the action of the electric field, the heavy metals or other pollutants in the polluted soil move directionally and migrate to the vicinity of the cathode or anode to accumulate. Finally, it centrally treats the cathodic or anodic soil to remove contaminants. The principle of electrodynamic remediation of contaminated soil is shown in Figure 4. In the process of electrodynamic remediation, the main mechanisms of directional migration of pollutants in soil are electrolysis, electromigration, electroosmotic flow, electrophoresis and free diffusion. Among them, electromigration and electroosmosis play a leading role and determine the effect of electrodynamic repair (He et al., 2017; Sun, 2017).
In the process of electrodynamic soil remediation by electrolysis, the main reactions of the two poles are as follows:
Electromigration refers to the orientational migration of ions or ion-ion complexes in soil under the action of an electric field. There are many metal ions and a variety of negatively charged acidic ions in pore water, which make it have obvious electromigration effect (Zehang et al., 2018). The electromigration rate of soil-water system is closely related to its conductivity, electric field strength, number of charges, temperature, porosity and soil pore distortion coefficient. Electromigration is independent of pore size, so the process can be used for both coarse- and fine-grained soils. The electromigration rate can be expressed as:
In the Formula,
Z is the charge number of the ion, D is the diffusion coefficient,
Since the migration of ions in soil is more complex than in water, the electromobility rate in actual soil should be:
The ion mobility can be expressed by the following Formula:
Namely:Where
Electrodialysis is the movement process of soil pore water relative to soil under the action of voltage gradient (Yümün, 2017). When the soil surface is negatively charged, the direction of electrodialysis is from anode to cathode. Electrodialysis works better in saturated fine-grained soils. Factors affecting pollutant transfer via electrodialysis include ion concentration, mobility and hydration, surface charge of soil particles, dielectric constants of organic and inorganic particles in pore fluids, and temperature. The magnitude of the electroosmotic flow depends on the voltage gradient, liquid viscosity, Zeta potential, dielectric constant of the medium and soil porosity. The electroosmotic flow velocity can be expressed as:
The Formula for calculating the electroosmotic flux is as follows:
Among them,
According to the basic principles of electrostatics, the Zeta potential can be expressed as:
Among them, d is the distance between the two electrical layers, and D is the dielectric constant of the medium.
Electrophoresis refers to the directional migration of charged colloids in an electric field during electrokinetic repair. Colloidal particles generally include fine soil particles, microorganisms, humus and other substances. Compared with electromigration and electrodialysis, electrophoresis plays a relatively weak role in electrokinetic repair (Farias et al., 2018).
The so-called free diffusion means that substances flow from the high concentration side through the porous medium to the low concentration side. In electrodynamic remediation, due to the uneven distribution of pollutants in the soil, there is a certain degree of free diffusion in the solid-liquid medium. The free diffusion flux can be quantified by the following Formula:
Where
System Research
Figure 5 shows an electric repair device. The equipment includes a soil chamber, an anode chamber, a cathode chamber and a DC power supply. The soil chamber and the electrode chamber were separated by a porous plexiglass plate and filter paper to prevent soil particles from entering the electrode chamber. The volume of the cathode and anode electrode chamber is L×H×W = 15 cm × 10 cm×5cm, and the graphite electrode plate is placed in the cathode and anode electrode chamber. The size of the electrode plate for experiment is L×H×W = 1 cm × 13 cm × 10 cm. The size of the soil chamber is L×H×W = 25 cm × 10 cm × 10cm, and the size of the graphite plate as the electrode plate is L×H×W = 2 cm × 10 cm × 16 cm. Graphite electrode plate has good properties such as good conductivity, high temperature resistance, acid resistance, alkali corrosion resistance and easy processing, so graphite electrode plate is selected for the experiment. The sampling holes are distributed equidistantly in the soil chamber to facilitate sampling. The gas generated during electrolysis is discharged the electrode chamber. Before the experiment, the equipment must be sealed to ensure that the equipment does not leak during the experiment. A uniform soil sample was placed in the soil chamber and compacted uniformly without leaving pores to avoid preferential passage. There are two drainage holes on the sides of the cathode and anode electrode chambers respectively, and a peristaltic pump is used to connect the solution reservoir to circulate the electrolyte (Anbazhagan et al., 2021).
The process flow of simulated contaminated soil remediation is shown in Figure 6.
Clarify the specific object: Clarify the specific soil to be repaired, and determine the soil depth at the same time. Soil at different depths is polluted differently.
Soil borrowing: Take the soil of the land to be tested, and determine the basic properties of the soil, such as water content, PH value, density, organic matter content, etc.
Soil crushing and screening: removal, crushing and screening of non-soil components. Non-soil substances and lumpy soils have a great impact on the test results in a limited test space.
Simulated remediation test: Pour the soil into the soil chamber, carry out the remediation test of the contaminated soil, and record and organize the test results.
Contaminated soil removal: During the simulated soil contamination process, the contaminated soil was removed and the test equipment was cleaned.
Remediation soil treatment and backfilling: After removing the remediation soil, check the remediation soil to ensure that the treated soil will not cause secondary pollution to the surrounding environment again.
Experimental Design of the Repair Device
The original soil of the experiment was taken from 15 cm below the surface of the former site of the Jiangxi ammonia plant, and the collected soil was naturally air-dried and ground to pass through a 20-mesh sieve and stored for later use. The basic properties of soil are shown in Table 3.
A filter paper (or filter paper + fiber membrane) was placed between the electrode chamber and the soil chamber to isolate the soil and electrolyte, and 100 g of lead-contaminated simulated soil samples were filled in the soil chamber of the electrodynamic remediation device. Add 200 ml of working solution (distilled water or 0.1 mol/L KCl solution) to the two electrode chambers respectively, and after equilibrating for 12 h, supplement the working solution to the height of the overflow pipe, connect the electrodes to the DC power supply, apply a constant voltage, and start the repair experiment. KCl has good conductivity, is easy to ionize, and is cheap and easy to obtain, so it is selected as the electrolyte. The specific experimental conditions are shown in Table 4.
During the experiment, the ammeter was used to record the current curve in real time, and the pH value of the electrolyte was measured at the specified time interval. After the test, the electrolysis working liquid was collected, and the soil was taken out and divided into five parts. It is marked as A, B, C, D, E, representing soils between 0 and 2 cm, 2–4 cm, 4–6 cm, 6–8 cm and 8–10 cm from the anode, respectively. The changes of soil pH, current and the concentration of heavy metal cadmium were measured, and the adsorption rate was calculated.
(1) Changes in soil pH.
Figure 7 studies the effect of electrolyte type and remediation time on soil pH. Among them, the electrolyte of E1 is distilled water, the electrolyte of E2 is 0.1 mol/L KCl solution, the experimental voltage is 10V, and the soil remediation time is 24 h. As can be seen from Figure 7, no matter whether the electrolyte is distilled water or KCl solution, there is no significant difference in the characteristics of soil pH change, all showing that the pH of the soil near the anode is lower, and the pH of the soil near the cathode is higher. In E2, the region A near the anode had a pH of 3.18, while the region E near the cathode had a pH of 10.05. And with the extension of remediation time, the soil pH in the anode area gradually decreased, and the pH values of area A in E2 and E3 were 3.18 and 3.09, respectively. The soil pH in the cathode area gradually increased, and the pH values of area E in E2 and E3 were 10.05 and 10.13, respectively. It is shown that the type of electrolyte has no obvious effect on the change of soil pH when the electrodynamic remediation device remediates the contaminated soil, and all causes the soil pH to surge. And with the extension of remediation time, soil pH mutation tends to be further deteriorated.
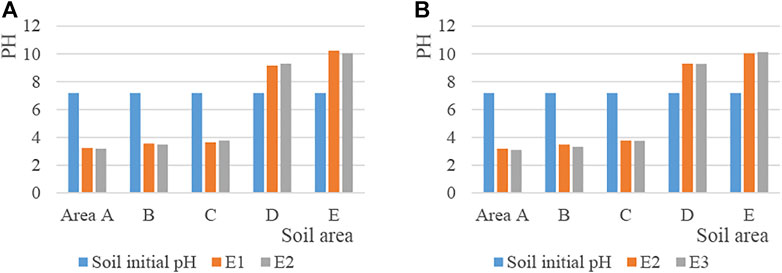
FIGURE 7. Variation of soil pH under different experimental conditions. (A) shows the effect of electrolyte types on soil pH. (B) shows the effect of remediation time on soil pH.
The existing forms of heavy metals in soil are divided into ion exchange state, carbonate bound state, Fe-Mn oxidation state, reducible state and residual state, and their solubility decreases in turn. The research of Kaur T (Kaur et al., 2021) et al. showed that under the action of electric field and electrolyzed water, >90% ion exchange state, 45–75% carbonate bound state, 15–30% Fe-Mn oxidation state and 8–30% Fe-Mn oxidation state can be removed. 20% reducible heavy metal pollutants. Therefore, when using electrodynamics to remediate heavy metal-contaminated soils, soil pH should be considered, because it affects the existence of heavy metals and the desorption process from the soil surface.
(2) Changes in current.
It can be seen from Figure 8 that in the electrodynamic repair process, although the electrolyte type and the experimental voltage are different, the current change trend is generally the same. The current increases rapidly in a short time, and then gradually decreases and becomes stable after reaching the maximum value. When the electrolyte is KCL solution, the soil current is significantly higher than that of distilled water. Adding electrolyte to water will increase the conductivity of water, KCl is a strong electrolyte, there is the transfer of k+ and cl-charges, so the current is larger (Njoh et al., 2021). With the increase of voltage, the electromigration is enhanced. The greater the migration of heavy metal Cd to the cathode, the greater the current. At 6h, the currents of E1 and E2 both reached their peak values, which were 0.44 and 0.68mA, respectively, after which the current began to decrease. When the voltage is 20V, the maximum current reaches 0.75 mA respectively. After reaching the peak value, it gradually decreased, and dropped to 0.68, 0.55, 0.48 and 0.34 mA at different times.
(3) Changes in heavy metal concentrations.
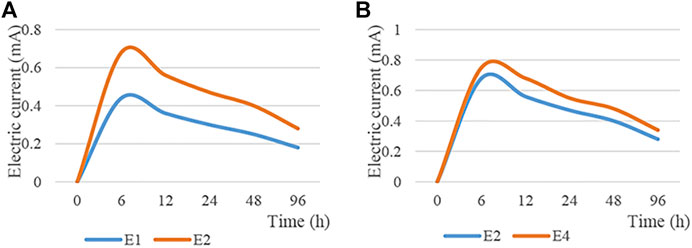
FIGURE 8. Variation of current under different experimental conditions. (A) shows the effect of electrolyte type on current. (B) shows the effect of different voltages on current.
Figure 9 shows the effect of electrolyte type and restoration time on the concentration of cadmium in soil. Among them, the electrolyte of E1 is distilled water, the electrolyte of E2 is 0.1 mol/L KCl solution, the voltages of E1 and E2 are both 10V, and the voltage of E4 is 20V. It can be seen from Figure 9 that the distribution of cadmium ions in the soil is not uniform. In the acidic area, the lead content is significantly reduced, and in the alkaline area, the lead content is relatively high, and there is a clear focusing phenomenon. This is because Cd2+ is more easily reduced under acidic conditions. With the prolongation of remediation time, the distribution of cadmium ions in the soil became more uneven. In E4, the lead concentrations in regions A, B, C, D and E were 0.22 mg/g, 0.64 mg/g, 0.79 mg/g, 1.41 mg/g and 1.22 mg/g.
(4) Heavy metal adsorption rate.
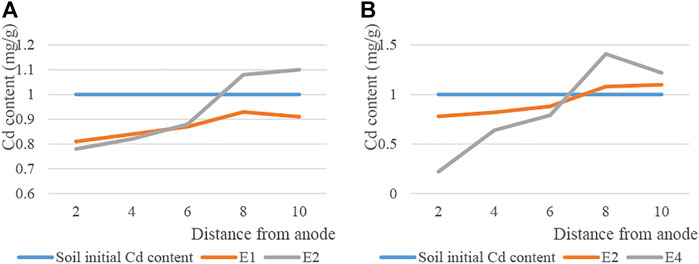
FIGURE 9. Changes of cadmium metal concentration under different experimental conditions. (A) shows the effect of electrolyte types on cadmium concentration in soil. (B) shows the effect of restoration time on cadmium concentration in soil.
Figure 10 shows the variation of the adsorption rate of cadmium ions in soil with time under each experimental condition. The adsorption rate of cadmium ions changes the same under different electrolytes and voltage gradients. With the prolongation of remediation time, the adsorption rate of cadmium ions in soil also increased gradually. When the voltage increased from 10V to 20V, the adsorption rates of cadmium ions in soil at 6, 12, 24, 48 and 96 h were 2.6, 4.5, 6.2, 10.7 and 12.5%, respectively, and the average adsorption rate was 7.3%. It can be seen from the figure that the change trend of cadmium ion adsorption rate under different electrolytes is the same, and the adsorption rate gradually increases from 6 to 96h, but the upward trend is not obvious. With the increase of voltage, the adsorption rate of heavy metal cadmium ions gradually increased, and when the voltage was from 10V to 20V, the adsorption rate of cadmium ions increased significantly.
(5) Energy consumption.
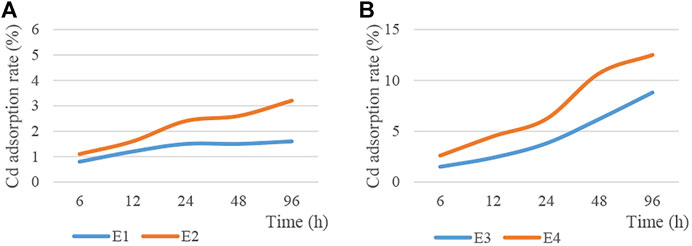
FIGURE 10. The adsorption rate of cadmium metal under different experimental conditions. (A) shows the adsorption rate of cadmium ions in soil by electrolyte types. (B) shows the adsorption rate of cadmium ions in soil by voltage.
Table 5 shows the power consumption (W/mg) of removing 1 mg of cadmium, and Figure 11 shows the change of power consumption with time under each experimental condition. The changes in energy consumption are roughly the same for different electrolytes and voltage gradients. As the repair time increases, the energy consumption also increases gradually. When the electrolyte is KCl, the energy consumption of soil at 6, 12, 24, 48 and 96 h is 0.3, 0.96, 1.42, 1.6 and 1.65W respectively, which is higher than that when the electrolyte is distilled water. When the voltage increased from 10V to 20V, the energy consumption of soil at each time point was 0.58, 1.24, 1.85, 2.27 and 2.38W respectively. It can be seen from the figure that the impact of voltage on energy consumption is greater than that of electrolyte on energy consumption, which shows that in the process of electrodynamic repair, the expected repair effect can be achieved by increasing the voltage (Arulnangai et al., 2021; Sangur et al., 2021).
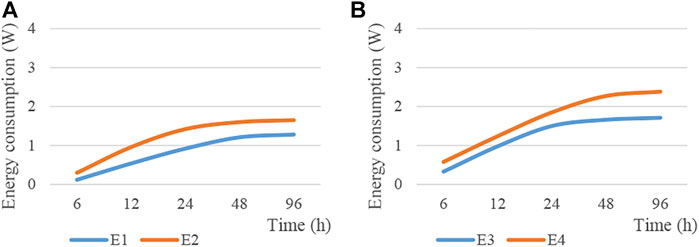
FIGURE 11. Comparison of energy consumption under different experimental conditions. (A) shows the effect of electrolyte type on energy consumption (B) shows the effect of voltage on energy consumption.
Conclusion
In this paper, a remediation device for electrokinetic remediation of heavy metal geophysical soil is designed, and the relevant experimental parameters such as soil pH, current, heavy metal concentration and adsorption rate are measured. Based on the digital economy, this paper recycles the electrolyte to promote the application of the circular economy in environmental protection. The device fully reacts the electrolyte with soil heavy metal ions, and also has the advantage of resource reuse, has a good removal effect on soil heavy metal pollution, and is conducive to improving soil remediation efficiency. Through the above experiments, it is recommended to carry out the follow-up work from the following. In this experiment, the electrokinetic remediation of cadmium-contaminated soil samples was only studied, and other heavy metals and organic matter were not considered. Subsequent experiments may consider using other heavy metal-contaminated soils for research.
Data Availability Statement
The original contributions presented in the study are included in the article/Supplementary Material, further inquiries can be directed to the corresponding author.
Author Contributions
All authors listed have made a substantial, direct, and intellectual contribution to the work and approved it for publication.
Funding
This work was supported by Nature Science Foundation of Shandong Province (ZR2020QE148), University research project of Shandong Province (J18KB059), Nature Science Research Incubation Program of Qingdao University of Technology, Linyi (2021C004).
Conflict of Interest
The authors declare that the research was conducted in the absence of any commercial or financial relationships that could be construed as a potential conflict of interest.
Publisher’s Note
All claims expressed in this article are solely those of the authors and do not necessarily represent those of their affiliated organizations, or those of the publisher, the editors and the reviewers. Any product that may be evaluated in this article, or claim that may be made by its manufacturer, is not guaranteed or endorsed by the publisher.
References
Alizadeh, T., Grubesic, T. H., and Helderop, E. (2017). Urban Governance and Big Corporations in the Digital Economy: An Investigation of Socio-Spatial Implications of Google Fiber in Kansas City. Telematics Inf. 34 (7), 973–986. doi:10.1016/j.tele.2017.04.007
Anbazhagan, V., Rajendran, R., Arumugam, G., and Ganeshan, A. (2021). Identification of Heavy Metal Pollution Source Due to Idol Immersion Activity across the Cauvery River Basin, Tamil Nadu, South India. Curr. Sci. 120 (1), 200–208. doi:10.18520/cs/v120/i1/200-208
Arulnangai, R., Sihabudeen, M. M., and Sirajudeen, J. (2021). Studies on Heavy Metal Pollution of Ground Water Analysis in and Around–Udayarpalayam Taluk at Ariyallur District- Tamilnadu. Int. J. Pharm. Biol. Sci. 9 (2), 14–19.
Drahokoupil, J., and Jepsen, M. (2017). The Digital Economy and its Implications for Labour. 1. The Platform Economy. Transf. Eur. Rev. Labour Res. 23 (2), 103–107. doi:10.1177/1024258917701380
Elder-Vass, D. (2021). Book Review: Tim Jordan, the Digital Economy. Glob. Media China 6 (2), 241–243. doi:10.1177/20594364211011686
Farias, D. R., Hurd, C. L., Eriksen, R. S., and Macleod, C. K. (2018). Macrophytes as Bioindicators of Heavy Metal Pollution in Estuarine and Coastal Environments. Mar. Pollut. Bull. 128 (MAR.), 175–184. doi:10.1016/j.marpolbul.2018.01.023
Gasiorek, M., Kowalska, J., and Mazurek, R. (2017). Comprehensive Assessment of Heavy Metal Pollution in Topsoil of Historical Urban Park on an Example of the Planty Park in Krakow (Poland). Chemosphere 179 (Jul), 148–158.
Gulamov, S. S., Shermukhamedov, A. T., and Haitmatov, U. T. (2021). Methodological Aspects of Statistical Analysis of the Digital Economy in Uzbekistan. Theor. Appl. Sci. 95 (3), 71–77. doi:10.15863/tas.2021.03.95.12
He, K., Sun, Z., Hu, Y., Zeng, X., Yu, Z., and Cheng, H. (2017). Comparison of Soil Heavy Metal Pollution Caused by E-Waste Recycling Activities and Traditional Industrial Operations. Environ. Sci. Pollut. Res. Int. 24 (10), 9387–9398. doi:10.1007/s11356-017-8548-x
Jepsen, M., and Drahokoupil, J. (2017). The Digital Economy and its Implications for Labour. 2. The Consequences of Digitalisation for the Labour Market. Transf. Eur. Rev. Labour Res. 23 (3), 249–252. doi:10.1177/1024258917714659
Kaur, T., Rajput, S., and Bhardwaj, R. (2021). Appraisal of Heavy Metal Pollution in Groundwater of Malwa Region, Punjab (India) Using Stress Biomarkers in Brassica Juncea. Environ. Earth Sci. 80 (9), 1–12. doi:10.1007/s12665-021-09657-9
Leahovcenco, A. (2021). Cybersecurity as A Fundamental Element of the Digital Economy. Mest 9 (1), 97–105. doi:10.12709/mest.09.09.01.13
Liu, N. (2018). China's Digital Economy: A Leading Global Force. Chinas Foreign Trade 567 (03), 20–21.
Makarova, A. I. (2021). Blockchain Impact on Public Administration Processes in the Digital Economy. revistageintec 11 (4), 390–401. doi:10.47059/revistageintec.v11i4.2115
Markova, O. V., Listopad, E. Y., and Shelygov, A. V. (2021). Economic and Legal Aspects of the Innovative Activity of Enterprises in the Context of the Digital Economy. Nexo Rev. Científica 34 (2), 964–972. doi:10.5377/nexo.v34i02.11623
Miao, Z. (2021). Digital Economy Value Chain: Concept, Model Structure, and Mechanism. Appl. Econ. 53 (2), 1–16. doi:10.1080/00036846.2021.1899121
Njoh, R. A., Kocadal, K., and Alkas, F. B. (2021). Heavy Metal Pollution of Agricultural Soils and Vegetables of Abandoned Mining District in Northern Cyprus. Fresenius Environ. Bull. 30 (2), 1415–1423.
Ogli, T. X. B. (2021). The Role of the Digital Economy in the Development of Information and Communication in Uzbekistan. Acad. Int. Multidiscip. Res. J. 11 (5), 307–311. doi:10.5958/2249-7137.2021.01395.1
Paul, D. (2017). Research on Heavy Metal Pollution of River Ganga: A Review. Ann. Agrar. Sci. 15 (2), 278–286. doi:10.1016/j.aasci.2017.04.001
Putrik, Y. S. (2021). The Effect of the Digital Economy on the Formation of the State Control System. revistageintec 11 (4), 1612–1620. doi:10.47059/revistageintec.v11i4.2213
Sangur, K., Leiwakabessy, F., Tuaputty, H., Tuwankotta, L., Samloy, S., Ratila, C., et al. (2021). Mudskipper as an Indicator Species for Lead, Cadmium and Cuprum Heavy Metal Pollution in the Mangrove, Ambon, Indonesia. J. Ecol. Eng. 22 (4), 1–19. doi:10.12911/22998993/134077
Simon, C. M., Bakhirev, A, Böhm, M, and Schrer, M (2017). Measuring and Mapping the Emergence of the Digital Economy: a Comparison of the Market Capitalization in Selected Countries. Digital Policy, Regul. Gov. 19 (5), 367–382. doi:10.1108/dprg-01-2017-0001
Sun, Y. (2017). Ecological Risk Evaluation of Heavy Metal Pollution in Soil Based on Simulation. Pol. J. Environ. Stud. 26 (4), 1693–1699. doi:10.15244/pjoes/68889
Teoh, C. S., and Mahmood, A. K. (2017). National Cyber Security Strategies for Digital Economy. J. Theor. Appl. Inf. Technol. 95 (23), 6510–6522. doi:10.1109/icriis.2017.8002519
Watanabe, C., Moriya, K., Tou, Y., and Neittaanmaki, P. (2018). Structural Sources of a Productivity Decline in the Digital Economy. Ijmit 10 (1), 01–20. doi:10.5121/ijmit.2018.10101
Yang, Q., Li, Z., Lu, X., Duan, Q., Huang, L., and Bi, J. (2018). A Review of Soil Heavy Metal Pollution from Industrial and Agricultural Regions in China: Pollution and Risk Assessment. Sci. Total Environ. 642 (nov.15), 690–700. doi:10.1016/j.scitotenv.2018.06.068
Ying, H., Chen, Q., and Deng, M. (2018). Heavy Metal Pollution and Health Risk Assessment of Agricultural Soils in a Typical Peri-Urban Area in Southeast China. J. Environ. Manag. 207 (FEB.1), 159–168. doi:10.1016/j.jenvman.2017.10.072
Yümün, Z. Ü. (2017). The Effect of Heavy Metal Pollution on Foraminifera in the Western Marmara Sea (Turkey). J. Afr. Earth Sci. 129 (MAY), 346–365. doi:10.1016/j.jafrearsci.2017.01.023
Zehang, S., Xiande, X., Ping, W., Yuanan, H., and Hefa, C. (2018). Heavy Metal Pollution Caused by Small-Scale Metal Ore Mining Activities: A Case Study from a Polymetallic Mine in South China. Sci. Total Environ. 639 (oct.15), 217–227. doi:10.1016/j.scitotenv.2018.05.176
Keywords: heavy metal pollution, soil remediation, electrodynamic remediation technology, device design, digital economy
Citation: Li Y, Liu J and Jia S (2022) The Design of a Remediation Device for Heavy Metal Contaminated Soil Under Digital Economy. Front. Energy Res. 10:893335. doi: 10.3389/fenrg.2022.893335
Received: 10 March 2022; Accepted: 29 April 2022;
Published: 13 May 2022.
Edited by:
Fadi Al-Turjman, Near East University, CyprusReviewed by:
Zubaida Sa'Id Ameen, Near East University, CyprusGongxing Yan, Chongqing Vocational Institute of Engineering, China
Jun Zheng, Baotou Teachers’College, China
Shuo Shi, Zaozhuang University, China
Copyright © 2022 Li, Liu and Jia. This is an open-access article distributed under the terms of the Creative Commons Attribution License (CC BY). The use, distribution or reproduction in other forums is permitted, provided the original author(s) and the copyright owner(s) are credited and that the original publication in this journal is cited, in accordance with accepted academic practice. No use, distribution or reproduction is permitted which does not comply with these terms.
*Correspondence: Yuanyuan Li, cmFpbmxpMDBAcXV0LmVkdS5jbg==