- Faculty of Environmental Engineering, The University of Kitakyushu, Kitakyushu, Japan
By optimizing the design of the building envelope, it is possible to create a more comfortable and energy-efficient indoor environment for the occupants. However, due to environmental issues in high-density cities, open balconies might affect the health of occupants. The current research focuses on the effect of a single balcony form in different climates, which could be detrimental to the diversity of balcony designs. Therefore, it is necessary to re-examine the rationality of traditional Japanese open balconies in terms of building envelope design and living environment to optimize the indoor thermal environment and reduce the energy consumption of dwellings. Focusing on Kitakyushu, Japan, which is in a warm climate, this paper conducts a comprehensive comparative study of the effects of building envelope design for residential buildings, including balcony form, thermal insulation performance, room orientation, and the window-to-wall ratio of balcony facades. The results of the study indicate that 1) the closed balcony design is strongly applicable to houses in warm climate regions in winter; 2) closed balconies facing south have a more positive impact on the indoor thermal environment, and energy-saving performance than open balconies; 3) even closed balconies without insulation could achieve higher thermal environment gains than open balconies with insulation. Designing a special type of closed balcony for residences in winter is more energy-efficient than insulation. This study proposes architectural envelope designs that are adapted to specific environmental and climatic conditions. It contributes to providing residential designers with a measure of how to provide a comfortable residential thermal environment and energy efficiency for occupants through architectural envelope design.
1 Introduction
By 2050, Asia’s energy consumption and carbon dioxide emissions will be about 1.5 times from 2015. Among them, the average annual energy consumption of the Japanese will increase by 20% from 2015 to 2050 (IEEJ Outlook 2018; IEEJ Outlook 2019). It is necessary to consider the resource constraints of energy supply and the impact of climate change and response measures. As buildings account for a significant portion of the total energy consumption, promoting energy efficiency in buildings is an effective measure to achieve energy conservation and help release environmental pollution and retard climate change (Chan, 2015). The number of multiple-dwelling houses in Japan accounted for 43.5% of the residential field by 2019, more than doubled in 30 years. In Japan’s large cities, the proportion of multiple-dwelling houses is greater than 70%, while the low-rise buildings (1–6 floors) account for 90.6% (Land Statistical Survey, 2019). However, with the increasing housing supply, large cities are facing environmental problems such as light pollution (Falchi et al., 2016; Hu et al., 2018), noise pollution (Mueller et al., 2017), and the heat island effect (Nieuwenhuijsen, 2021; Sedaghat and Sharif, 2022). Issues related to the living environment are undoubtedly important such as the indoor thermal environment and its impact on health (Wolkoff and Kjærgaard, 2007; Murtyas et al., 2020; Wolkoff et al., 2021). In the building design, spaces both inside and outside of buildings are paid special attention to because of their potential to save energy (Chun et al., 2004). Balcony plays the role of bridge between the internal and external environment in residential buildings, which has been studied in recent years. Subsequently, while the balcony space has the ability to save energy, it is also considered the “buffer space” of the indoor and outdoor environment, which can effectively improve the indoor environment and comfort and create an additional living space (Ribeiro et al., 2020). Furthermore, research shows that balcony space can increase residents’ satisfaction (Wing Chau et al., 2004; Kennedy et al., 2015; Omrani et al., 2017). Therefore, the rational design of residential balcony space is an essential part of realizing energy conservation and sustainable development.
In Japan, balconies (open type balconies) began to be popularized in multiple-dwelling housing in 1950. It is the only outdoor space in the special household space (Tomonari et al., 2003). Nowadays, every multiple-dwelling house in Japan has at least one traditional open balcony. As for the current situation of balcony utilization, it is mainly used for living services such as laundry, clothes drying, and garbage placement, and less for leisure such as cooling in the evening, sunbathing, and overlooking. Studies have shown that under certain optimized conditions, an open balcony could reduce the energy consumption for summer cooling by up to 12.3% (Chan and Chow, 2010). It occurs because the shading capacity provided by balconies is considered one of the most successful solutions to prevent overheating and achieve low energy consumption in summer (Hastings, 2004). Studies of open balconies have been conducted mainly in warm climates. Conversely, in cold climates, a closed balcony could positively impact and reduce heating energy consumption by up to 46% (Babaee et al., 2016). It indicates the importance of balcony design adapted to each specific environmental and climatic condition. The current research focuses on the effect of a single balcony form in different climates, which could be detrimental to the diversity of balcony designs. In this paper, the city of Kitakyushu in the Kyushu region of Japan, which is located in a warm climate region, was chosen as the study area for a comprehensive study of the effects of different balcony designs.
There are studies on closed balconies worldwide (Voss, 2000; Wilson et al., 2000; Fernandes et al., 2015), and there are also examples of changing open balconies into closed balconies. From 1987 to 1999, the number of cases of closed balcony modification has hardly changed worldwide but has increased sharply since 2001 (Tomonari et al., 2003). Besides the aforementioned impact on energy efficiency, a closed balcony can also reduce the cold, strong wind, and noise in winter and ensure privacy (Tomonari et al., 2003; Lu et al., 2004; Chan, 2015) to create a cleaner, quieter, and safer internal environment for dwellings (Lu et al., 2004). Due to the existing environmental problems in high-density cities, such as the high noise level of residential buildings located in high traffic areas. Designing open balconies for residential buildings, especially low-rise residential buildings, might not be the optimal solution and might have a negative impact on the health of the occupants. Distinguishing from the traditional Japanese open balconies, this paper will explore the applicability of closed balconies in warm climate regions for residential applications in winter, under the premise of diversifying balcony designs and living environments.
By optimizing the design of the building envelope, a more comfortable and energy-efficient indoor environment can be created for residents (Yu et al., 2008; Mitterer et al., 2012; Ascione et al., 2016). Meanwhile, proper balcony design is an essential component of energy efficiency and sustainability. In this paper, Kitakyushu City in the Kyushu region of Japan, which is a warm climate region, was selected as the study area. The Kyushu region is located in the southern part of Japan with a climate characterized by cold winters and hot summers and the fifth and sixth divisions of the building design zoning (Ministry of Land, 2020). According to the statistics of the Ministry of Land, Infrastructure, Transport, and Tourism of the Japanese government (Sakamoto et al., 2014), the penetration rate of thermal insulation for multiple-dwelling houses is lower in the fifth and sixth divisions, and the percentage without insulation is 86.4%. The percentage of dwellings with lower thermal insulation in the Kyushu region is 92.1%, the penetration rate of window thermal insulation is close to 0, and the percentage of double glazing used in new dwellings is only 15%. It indicates that the thermal insulation of dwellings in our study area is generally low. Therefore, this paper aims to explore the effectiveness of the application of dwellings insulation and closed balcony design in warm climate regions.
In this paper, indoor thermal environment measurements were conducted in multiple-dwelling houses with closed and open balconies, respectively, located in warm regions, with special attention to the role of balcony space. Focusing on Kitakyushu city, Japan, which is located in a warm climate, the design model of the building envelope design includes balcony form, thermal insulation performance, room orientation direction, and the window-to-wall ratio of the building façade is presented. In addition, a comparative study is conducted by simulation. The objectives are to: 1) comprehensively assess the effects of different balcony designs on the indoor thermal environment and energy efficiency of multiple-dwelling houses in winter in warm regions; 2) explore the effectiveness of envelope design measures to optimize the indoor thermal environment and energy efficiency; 3) propose architectural envelope designs that are adapted to specific environmental and climatic conditions. The finding contributes to providing residential designers with a measure of how to provide a comfortable residential thermal environment and energy efficiency for occupants through residential envelope design.
The remainder of this paper is organized as follows. Section 2 describes the research methods of field measurement and simulation. Firstly, it explains the climate of the area where the measured object is located, the profile of the measured object building, and the use characteristics of the balcony. Meanwhile, the process of field measurement is described. Then, the process of simulating the indoor thermal environment and heating load in winter is introduced. In Section 3, the summary of the measured survey was conducted in winter 2019, and the results measured on heating and non-heating days are analyzed. Meanwhile, the simulation results of four case combinations of balcony forms, window wall ratio of balcony facade, balcony orientation, and thermal insulation of enclosure structure are explained. Section 4 summarizes the discussion and limitations of this study. Finally, this study is concluded.
2 Materials and methods
In this study, we first selected a typical south-facing open balcony and two south-facing, a north-facing closed balcony, and the room connected with the balcony for measurement in winter. The measurement is divided into heating days and non-heating days. For analysis, we pay special attention to the role of the balcony to obtain theprimary data on the thermal environment of the balcony space and its adjacent rooms in the residence.
According to the measured typical balcony, an open and closed type balcony and its connected room are created as model cases. Then, using the expanded AMeDAS weather data (Akasaka, 2000) (130.52°N, 33.53°E), the indoor temperature and heat load are simulated by the dynamic heat load calculation program NewHASP/ACLD (https://www.jabmee.or.jp/hasp/). This program is applicable to Japan, where it is a representative heat load and air conditioning system calculation program. It calculates indoor temperature, humidity, and building space heat load and evaluates air conditioning energy consumption. Comparing the heat loads of standard building models in Japan, the NewHASP/ACLD and Energy Plus simulation programs exhibit the same accuracy (Yang et al., 2013). We set the thermal insulation performance of the room maintenance structure, the orientation of the balcony, the window-to-wall ratio of the balcony façade, and the design of closed and open balconies in a combination of 32 calculated patterns. By comparing and analyzing the simulation results, the best-case combination solution to achieve a comfortable indoor thermal environment and reduce energy consumption is determined. The content will be further detailed in Section 2.2 and Section 2.3.
2.1 Study objects
To obtain the basic data of the indoor thermal environment in the cold time of residential buildings with balcony space in the warm climate discussthe effects of open and closed balcony forms on the residential indoor thermal environment. In this paper, three multiple-dwelling houses in Kitakyushu (130.52°N, 33.53°E), Japan, Shanghai (120.51°N, 31.23°E), and Nanjing (118.35°N, 31.23°E), China, and the balcony space residence are measured. The three-case surveyed are residential with balconies, each using the acronym balcony and the acronym of the place name, abbreviated as B-K, B-S, and B-N. Specifically, B-K is residential with open balconies in Kitakyushu, Japan. B-S and B-N are residential with closed balconies in Shanghai and Nanjing, China. The balcony of B-N1 is facing south, and B-N2 is facing north. Table 1 shows the daily average temperature and humidity in Kitakyushu, Shanghai, and Nanjing in winter in recent 25 years. All three cities subject to the measurements are located in warm climate regions. There are climatic characteristics of hot summers and cold winters, and the average temperature of the coldest winter month is above 0°C. As shown in Figure 1, all three cities reported the coldest daily average winter temperatures in January, with 5.8°C in Kitakyushu, 4.8°C in Shanghai, and 3.0°C in Nanjing.
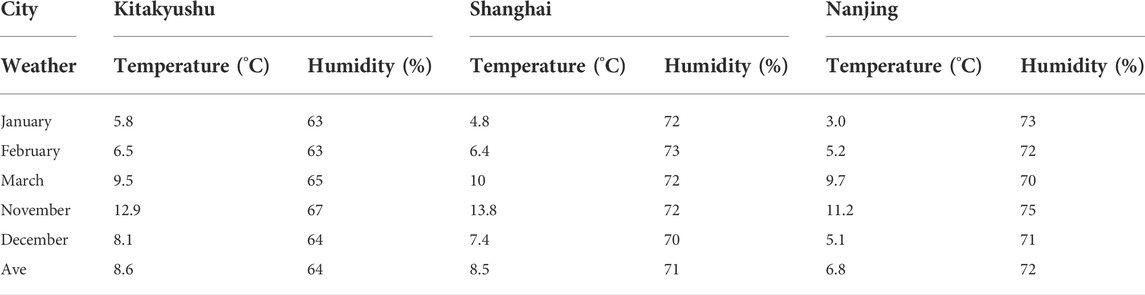
TABLE 1. The daily average temperature, and humidity in Kitakyushu, Shanghai, and Nanjing in winter.
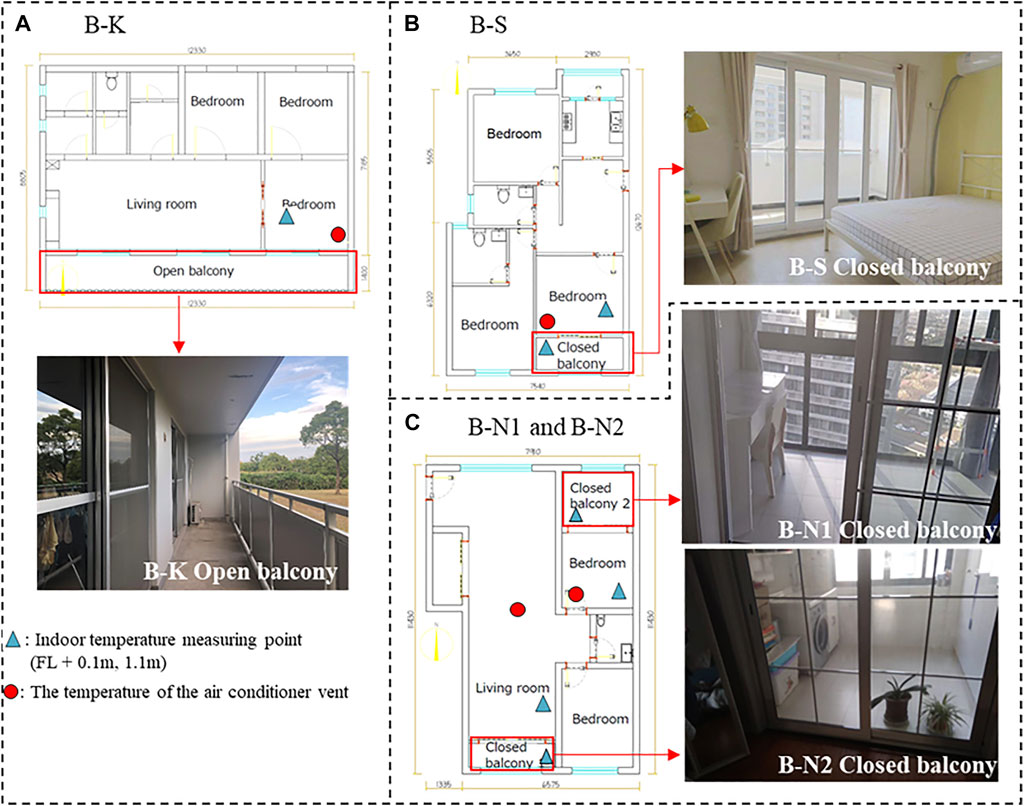
FIGURE 1. Plan view and measured point of measurements objects, (A) B-K, (B) B-S, and (C) B-N1, and B-N2.
The indoor plan of the measurement object and the summary of the measurement object are shown in Figure 1 and Table 2. B-K is a low-rise multiple-dwelling house located in the west of Kitakyushu City. Occupants are usually in their rooms in the morning and at night. The balcony of the measurement object is open and located outside the living room and the Japanese room on the south side. The room temperature is controlled by the wall-mounted air conditioner and installed in each bedroom. B-S is a middle-level multiple-dwelling house in Shanghai. Occupants are at home in the morning and evening on weekdays and rest days. The measurement object is a closed balcony set in the bedroom on the south side. The room temperature is controlled by the wall-mounted air conditioner. B-N is a multiple-dwelling house located in the west of Nanjing. Occupants are usually at home in the evenings and mornings on weekdays and all day on holidays. The balcony of the measurement object is the same as the case in Shanghai, a closed balcony, in the south living room (B-N1) and the north bedroom (B-N1). The room’s temperature is controlled by central air conditioning, and the two rooms adjacent to the balcony have air vents.
The balconies of multiple-dwelling houses in Shanghai, Nanjing, and Kitakyushu as measured objects are shown in Figure 1. The profile of the measured balcony is shown in Table 2. The open balcony of B-K is widely used in Japan (Tomonari et al., 2008). It extends from east to west outdoors and is not used except for drying clothes. There are three sliding glass windows on the wall between the open balcony and the interior. Two are between the living room and the balcony, and one is between the south side bedroom and the balcony connected by the living room. In the south bedroom, the glass windows are closed in winter. Occupants usually use air conditioning to adjust the indoor environment. In addition, the windows are surrounded by aluminum window frames, which have low thermal insulation performance compared with the resin window frames used in the target houses in B-S and B-N (Table 2). B-S closed balcony space has a sliding door with a glass window as the inner window. Balcony space is usually used to dry clothes and is used to store idle items. In winter, the inner window is closed all day. Curtains are not often used to make the light shine into the room during the day. Dark curtains and air conditioning are usually used at night to cover the outdoor temperature, adjust the indoor temperature and ensure privacy. In addition, the window frames of the inner and outer windows of the balcony space are resin frames with high heat insulation performance. Both balconies of B-N have sliding doors with glass windows (inner windows). B-N1 represents the closed balcony on the south side, used as a space for laundry and drying clothes. The inner window is open all year round and closed only when the air conditioner is used. B-N2 represents the closed balcony on the north side, with tables and chairs, which is used as a learning space with a low frequency of occupants. B-N2 has a large area of external windows and is easily affected by the external temperature. When the outdoor temperature is low at night in winter, dark curtains are usually used to ensure the indoor temperature. Internal windows are usually closed when heating. In addition, the window frames of the inner and outer windows are resin frames with high heat insulation performance.
2.2 Field measurement
The measurement was conducted from January to early February 2019. The surveyed multiple-dwelling houses are the three with open and closed balconies shown in Section 2.1. The summary of the measured objects is shown in Table 2, and the measured points are shown in Figure 1. We measured the temperature and humidity in the outdoors, balcony space, and the adjacent rooms during the non-heating and heating periods (Table 2). Data loggers are set 0.1 and 1.1 m above the floor. In order to confirm the running time of air conditioning, a data logger is also set near the air conditioner vent. The profile of the data loggers’ parameters is shown in Table 4. The data loggers were placed and measured with consideration to avoid direct sunlight. As for the opening and closing of the inner windows in the balcony space, the B-S balcony is closed all day, the B-N1 balcony is open all day, and the B-N2 is measured in the state of closing the balcony windows during the operation period of the indoor air conditioner. The air conditioner is only used in the room adjacent to the balcony. Since the measurement period was the coldest month of winter, natural ventilation was rarely taken in the three subject dwellings.
2.3 Simulation
In this study, the simulation by the dynamic heat load calculation program NewHASP/ACLD (https://www.jabmee.or.jp/hasp/) was provided by the Japanese association building mechanical and electrical engineers. The simulation of this paper used Expanded AMeDAS weather data for Kitakyushu city, Japan (Kitakyushu 130.52°N, 33.53°E) (Akasaka, 2000), focusing on the heat load, indoor temperature, and radiant temperature in winter (November 1–March 31). The measured multiple-dwelling house objects in 2.2 are used as a reference to highlight the role of balcony space. We created a typical simplified model of a house with a balcony (The type A in Figure 2.). It is used as a base case to develop the case combination research proposed in this study. The parameters of the model are shown in Table 3. In the parameter setting of the model house, we referred to the measured house characteristics. According to the study and NHK (Broadcasting Culture Research Institute), the statistical time of Japanese people’s work and commuting time on weekdays, the rate of people who generally commute and start or finish their work between 7:00–8:00 a.m. and 17:00–18:00 p.m. is more than 90% (Sakamoto, 2013; NHKNHK Broadcasting Culture Research Institute, 2021). Therefore, the non-occupied time of the simulated model is from 9:00 to 18:00 on weekdays and occupied all day on weekends, with heating operating during occupied hours only.
The case combination includes four basic aspects, as shown in Figure 2. As a simulation model, we create a closed balcony (type B shown in Figure 2) based on the basic case of the room with an open balcony (Type A) to illustrate the impact of balcony shape design on a comfortable indoor thermal environment and energy saving. The design dimensions of the balcony and the adjacent room are shown in Table 5. Next, the ratio of balcony width to the window is proposed to evaluate the impact of balcony window size. As shown in Table 3, type C (Figure 2) with a window area less than 0.5 times type B and type D (Figure 2) with a window area greater than 2 times type B are designed. For the parameter setting of the model’s envelope, we refer to the measured house characteristics and the Japanese government regulations for residential buildings (Building Research Institute, 2017), and the materials and structures used are shown in Table 3 (Japanese association building mechanical and electrical engineers, 2012). There is without insulation in the measurement houses, and the outer window of the balcony is aluminum-framed single-glazed windows (Table 3, 1-general type). It shows poor thermal performance. We proposed a case for increasing the thermal insulation of the envelope by adding a 20 mm insulation layer to the outer layer of the exterior walls, below the floor and the inner layer of the roof, and setting single-pane window glazing to double-pane (Table 3, 2-insulation type). In addition, since the room’s orientation is an important factor affecting the indoor thermal environment, we also propose applying different balcony designs to four orientations East, South, West, and North. As shown in Table 4, we simulated the indoor temperature, radiant temperature (Tr), and heat load for 32 patterns combinations. The 32 patterns compositions are named by the combination of four model orientations (West, East, North, and South), insulation performance (Two designs of insulation structures for the models), and four balcony model designs (Figures 2A–D), which are listed in columns 4 and 8 of Table 4. Through the calculation and comparative study of 32 patterns, the operable scheme is put forward for creating a comfortable indoor thermal environment and energy-saving performance that can reduce energy consumption.
3 Result
3.1 Measurement results
3.1.1 Measured data
Table 5 shows the data of the field measurement results, which were measured in two conditions divided into heating days and no heating days (Table 2). The temperature and humidity of outdoor (B-K, B-S, B-N1, and B-N2), indoor (B-K, B-S, B-N1, and B-N2) and balcony (B-S, B-N1, and B-N2) in the three subject dwelling areas were measured under the conditions with and without balcony. The measured data at the height of 1.1 and 0.1 m set at the measurement points show that the average temperature difference between 1.1 and 0.1 m in the unheated case is within 1°C, which indicates that there is almost no vertical temperature difference in the measured dwellings. On the heating days, a maximum vertical average temperature difference of 4.8°C was reported, which is not consistent with the ISO 7730 of 3°C (ISO, 2005), which will be detailed in Section 3.1.2 of this study.
3.1.2 Measurement of temperature variations
The indoor temperature of the four measurement objects during the non-heating days and the heating days are shown in Figure 3. Among the temperature variation on non-heating days (Figure 3A), the closed balcony B-S showed the largest temperature fluctuations and the highest temperature. In addition, the temperature fluctuations in the rooms adjacent to the balconies were very small because the solar heat accumulated in the closed balcony space, and the internal windows were closed, thus acting as a “greenhouse effect.” As the result, the temperature of the closed balcony of B-N1 fluctuates in about the same range as that of the adjacent room, because the inner windows of the balcony are open, and the heat transfer between the two rooms creates the same thermal environment. The temperature of the closed balcony of B-N1 and the temperature in the adjacent room are both higher than that of B-N2, which is the effect of transmitted solar radiation. In addition, the temperature fluctuation range of the adjacent room of the closed balcony is smaller than that of the adjacent room of the open balcony. It shows that the closed balcony can be used as a thermal buffer space between indoor and outdoor.
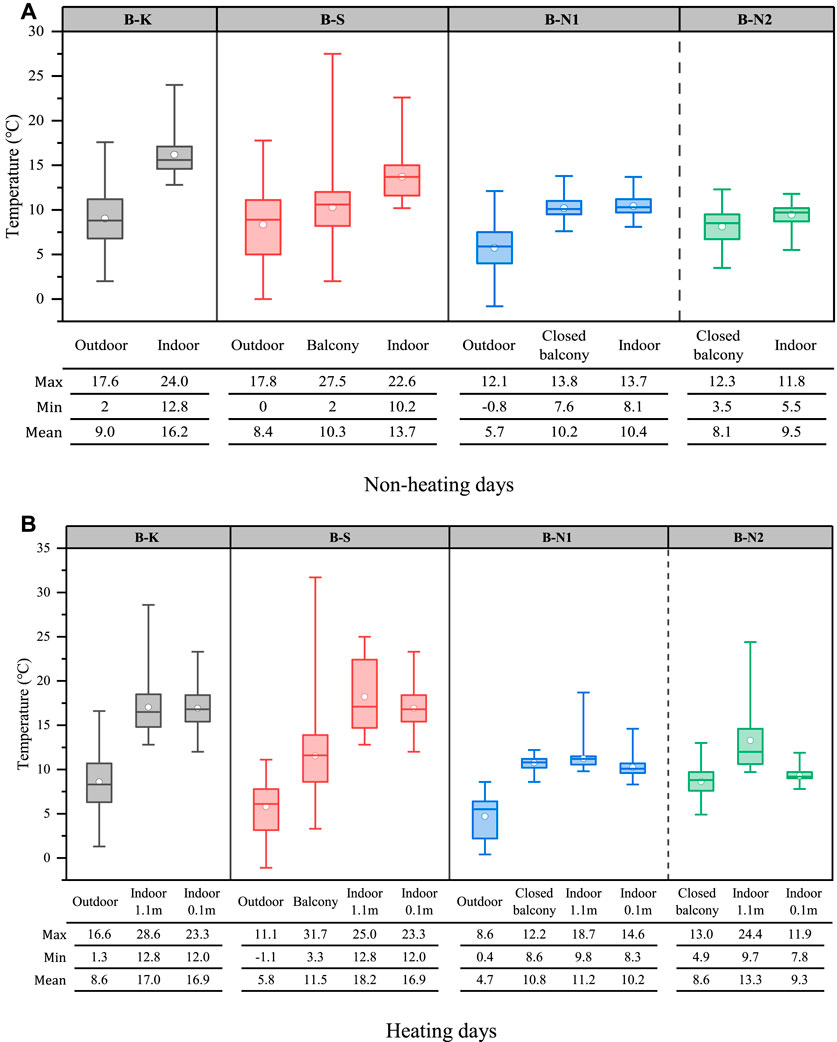
FIGURE 3. Indoor and outdoor air temperature of case B-K, B-S, B-N1, and B-N2, (A) on the non-heating days and (B) on the heating days.
The indoor temperature measured during the heating day is shown in Figure 3B. The measurements at 0.1 m show the minimum indoor temperature variation in three measured dwellings. This could be because the temperature measured at 1.1 m is affected by the air blown from the upper part of the room by the air conditioner during heating preheating, resulting in a higher temperature and greater temperature fluctuations than the temperature measured at 0.1 m. Meanwhile, the temperature variations of field measurement during the heating day show the same trend as during the non-heating day (Figure 3A). The temperature of the B-S closed balcony with the inner window closed is susceptible to solar radiation and fluctuates dramatically. However, the indoor temperature of the adjacent room fluctuates less. This result also proves that the closed balcony acts as an indoor-outdoor thermal buffer space.
In the typical daily temperature variation of cases B-S, B-N1, and B-N2 (Figure 4A), the balcony temperature of the B-S with the balcony inner window closed has been affected by solar radiation and outdoor air temperature, but the indoor temperature has not changed. The temperature fluctuations in the room are stable without being affected by disturbance. On the other hand, when the inner window of the balcony B-N1 is opened, the temperature of the room adjacent to the balcony is higher than the balcony temperature from night to early morning when the outdoor temperature is lower. However, this situation is reversed at 9:00 a.m. Then from 12:00 a.m., the balcony temperature was the same as the temperature of the adjacent room. We consider that a closed balcony can ingeniously transfer the solar radiant heat from the balcony to the room to make the room warmer. The north-facing B-N2 balcony temperature is affected by outdoor air, and its temperature fluctuation shows the same tendency as the outdoor air temperature fluctuation.
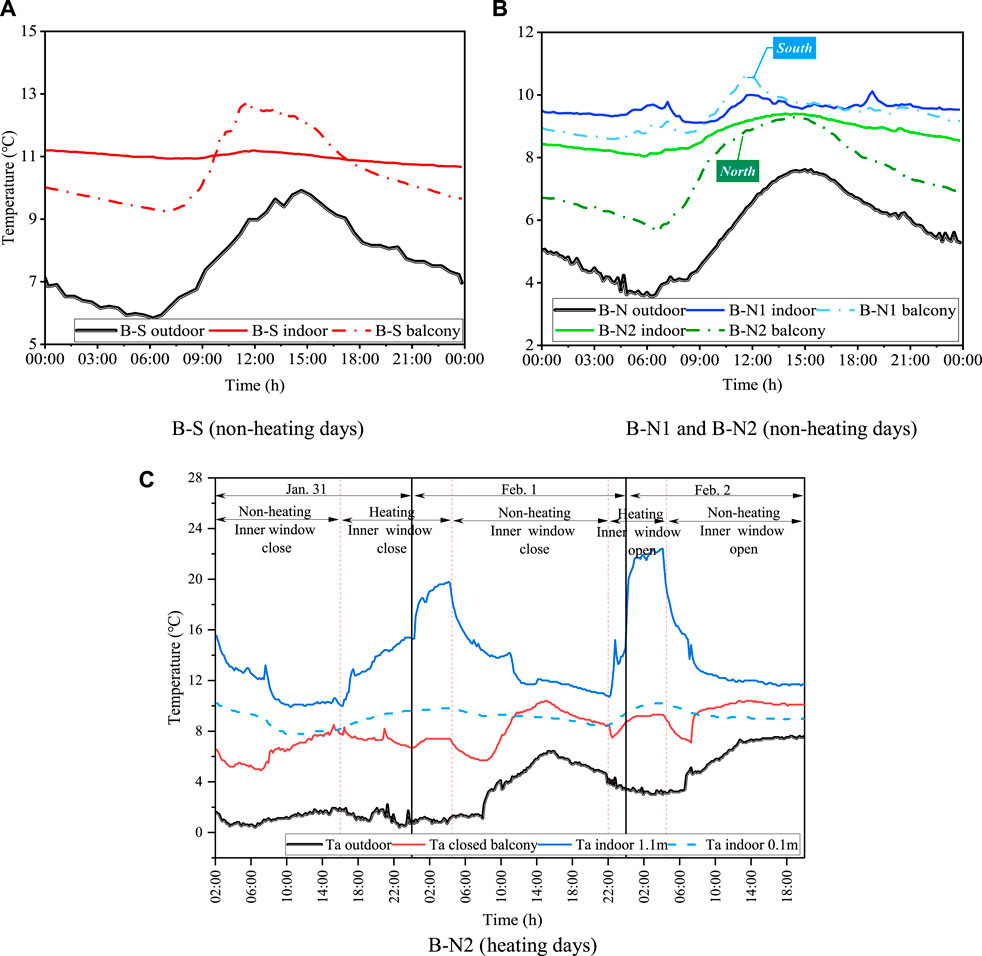
FIGURE 4. Temperature variations of outdoor, indoor, and balcony, (A) case B-S on the non-heating days, (B) case B-N1, B-N2 on the non-heating days, and (C) case B-N2 on the heating days.
Figure 4B shows the temperature variation of case B-N2 with intermittent heating for three consecutive days (31 January–2 February), where the inner window of the balcony space has an open state and a closed state. Among them, the fluctuation n trends of balcony temperature and outdoor temperature during the heating period are similar in the closed state of the inner window (16:00 on 31 January–04:30 on 1 February). Meanwhile, the indoor temperature in the rooms adjacent to the balcony during the non-heating time decreased more slowly (04:30–22:00 on 1 February). In the state where the inner windows are open and in heating (22:00 on 1 February–04:30 on 2 February), the temperature variations of the balcony space have a different trend from the outdoor air temperature fluctuation. They are more influenced by the indoor temperature of the adjacent rooms. The results of the change in indoor temperature (FL+1.1 m) in the adjacent room during the non-heating time decreased rapidly compared to when the window inside the balcony was closed (04:30 on 1 February–22:00 on 2 February).
3.2 Simulation results
3.2.1 Effect of the balcony form on the thermal environment
Table 6 summarizes the simulation results of indoor temperature (Ta) and radiant temperature (Tr) for the winter heating period for the four models. Winter average temperatures of Ta and Tr are almost not different between types B, C, and D. This indicates that the effect of the ratio of the external windows to the external walls of the balcony on the indoor temperature in the adjacent rooms is almost non-existent in winter. The indoor temperatures of the rooms adjacent to the balconies for the four models were first analyzed. The results are shown in Figure 5, which shows the average indoor temperatures of the four balcony types in winter for the west, east, north, and south orientations, where (1-general type) represents the general type of the model without insulation and (2-insulation type) represents the model’s insulation type. The results show that the indoor temperature of closed balcony types B, C, and D is higher than that of an open balcony. The insulation effect can be seen from the comparison of general type and insulation type. In addition, the indoor temperature of south-facing balconies is the highest among the four balcony types due to more solar heat gain from south-facing. The indoor temperature difference of types B, C, and D in different orientations is about 1.3°C, greater than type A by about 0.3°C. Therefore, closed balconies are more affected by different orientations than open balconies, which indicates that closed balconies with a south orientation have better benefits for the indoor thermal environment.
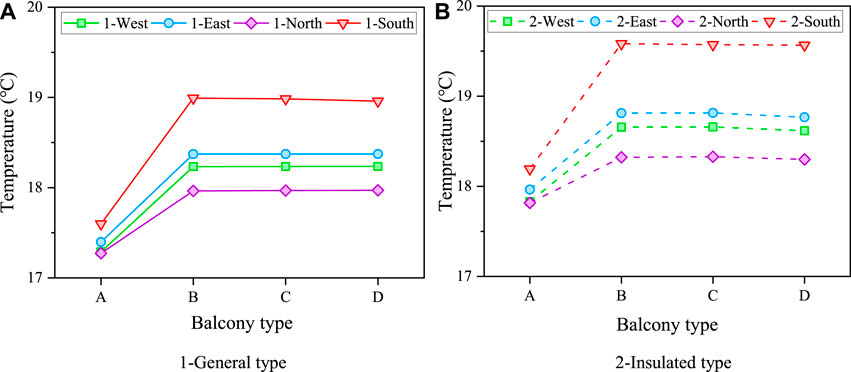
FIGURE 5. The average indoor temperature in winter, (A) simulated model for 1-general type, and (B) simulated model for 2-insulated type.
Except for the south orientation, both the general and insulated types show that the winter average temperature of the four models facing east is higher than that of the west orientation, which could be explained in Figure 6. Taking the simulation results of the average hourly indoor temperature in winter for Type B with four orientations during the heating period (Figure 6), the indoor temperature is maintained at 22°C during the heating period. The room is unheated from 9:00 to 21:00 due to the influence of solar heat, facing east is 0.3°C–1.1°C higher than that facing west from 9:00 to 14:30. Reversing between 14:30–19:00, the indoor temperature of the west-facing was 0.2°C–0.5°C higher than that of the east-facing. Finally, about an hour before heating, the indoor temperatures were almost equal (temperature difference of 0.1°C). Therefore, the difference in the time of obtaining the solar radiation leads to a higher average temperature in winter in the east than in the west (Figure 5).
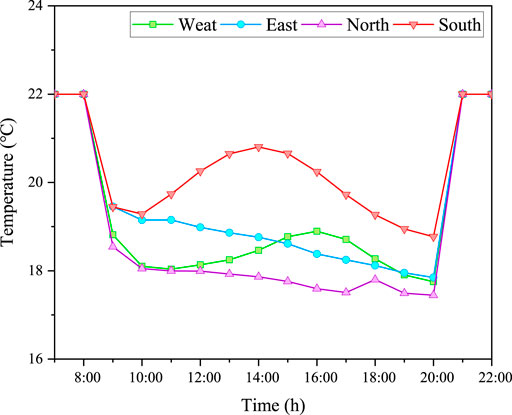
FIGURE 6. The average indoor temperature for each hour of winter in the west, east, south, and north orientation (Insulated Type B for heating days).
In terms of the average winter indoor temperature, the open balcony (Type A) with insulation in Figure 5B is less than the closed balcony (Type B, C, D) without insulation in Figure 5A. It is evident when the orientation is south, which is 0.6°C lower (Table 6). This result is confirmed by the indoor radiation temperature variation of a typical day in Figure 7. Since the temperature difference of the average radiation temperature in winter between Type B, C, and D is almost non-existent (maximum value is 0.2°C). We analyzed the change of radiant temperature of Type B, C, and D after averaging with Type A, divided into with (2-A, 2-Ave.B, C, D.) and without (1-A, 1-Ave.B, C, D.) insulation, the results are shown in Figure 7. The Tr of the closed balcony without insulation is always higher than that of the open balcony with insulation (temperature difference range: 0.2°C–2.7°C) during the non-heating hours of daytime. The situation during the heated nighttime is opposite to the daytime when the open balcony with insulation has a higher radiant temperature (temperature difference range: 0°C–0.8°C). Tr of a closed balcony with insulation is 1.1°C–4.7°C higher than that of an open balcony without insulation.
3.2.2 Effect of the balcony form on heat load
Table 6 and Figure 8 summarize the average heat load simulation results for the four models during the heating period. Different from the simulation results for the indoor thermal environment, the average heat load for Type B, C, and D is almost the same for the orientations of east, west, and north. while a difference of 5.0%–7.4% occurs between three types in the south orientation. It indicates that in winter, the difference in the area ratio of the balcony exterior windows to the exterior walls on the heat load only affects south orientation houses.
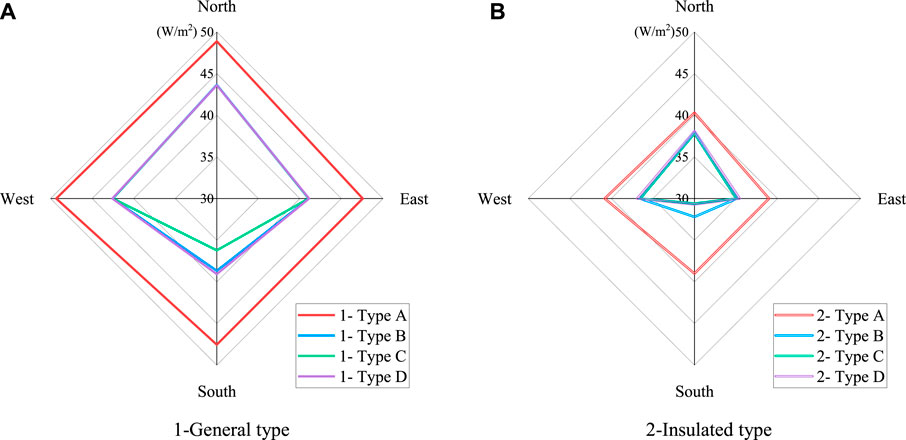
FIGURE 8. Simulation results of winter average heat load of four models. (A) simulated model for 1-general type, and (B) simulated model for 2-insulated type.
The heating loads during the heating (1 November–31 March) period were analyzed for each case. Figure 9 shows the comparison of the average winter heat load and its percentage reduction for the 32 calculated patterns in Table 4 compared to S1-A. Compared with S1-A, the closed balcony type reduces the heat load by 8%–24% for (1-General) and 14%–36% for (2-Insulation). Therefore, it can be confirmed that the closed balcony with improved insulation effectively saves energy. The largest rate of heat load reduction is for the closed balcony S2-C facing south with the smallest window area, which can reduce the heat load by 36% compared to the open balcony facing south. Therefore, the balcony with a small external window glazing area and slight heat loss has the best energy-saving effect. The heat load of type D is larger than that of type B. It may have happened due to the large external window area of a balcony of type D, which can get more solar heat, but at the same time, the heat loss is also large. Meanwhile, the heat load of type D of (2-insulation type) is smaller than that of type B. It may happen because the increase in solar heat generated exceeds the increase in heat transfer loss as the area of balcony exterior windows increases.
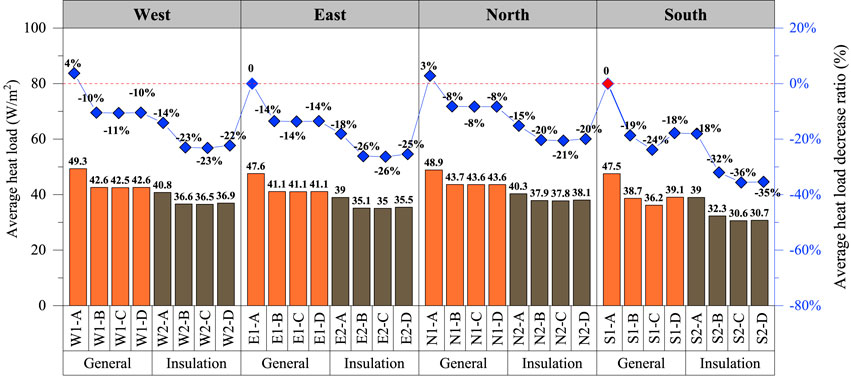
FIGURE 9. Comparison of the average heat load and its percentage reduction in winter for the 32 calculated patterns compared to Case S1-A.
In addition, the rate of heat load reduction for the open balcony with insulation in Figure 9 was compared with the closed balcony without insulation when the model’s orientation was south. By comparing S1-A, the reduction rates of S2-A, S1-B, and S1-D were found to be, 18%, 19%, and 18%, respectively. Moreover, the reduction rate of S1-C is 24%. This indicates that when the closed balcony has a smaller external window area and is without insulation, it could effectively reduce the energy by 7.2% more than an open balcony with insulation. This suggests that designing a specific type of closed balcony for a house in winter could be more energy efficient than adding insulation.
4 Discussion and limitations
Proper balcony design is essential for improving energy efficiency and sustainability in buildings. Research on open balconies has focused on environmental conditions in warm regions, mainly because open balconies can reduce summer overheating problems (Ribeiro et al., 2020). In this paper, Kitakyushu city in Japan, located in a warm climate region, was selected for the study. Recent studies have confirmed that 60%–70% of energy demand is allocated to cooling in some countries in warm climate zones (Touma and Ouahrani, 2018). However, Kazuaki et al., investigated residents’ lifestyles in eight major cities in Japan. The results showed that even in cities located in warmer regions, occupants’ demand for heating is still greater than their demand for cooling. Occupants typically spend 33% (120 days) of their annual time on heating and 19% (70 days) on cooling. Among them, 50% of the residential heating months are concentrated between November and March, and cooling months are concentrated between July and September (Kazuaki et al., 1998). It proves the need to study the design rationalization of these traditional open balconies in Japan during winter in warm regions.
In this study, we analyzed the indoor thermal environment and energy-saving effect of residential buildings in winter and concluded that closed balconies in residential buildings have great potential for energy saving and are beneficial for creating a comfortable indoor thermal environment. However, the part studied in this paper is only one aspect of the design phase of balconies in warm climate zones. Closed balconies may cause overheating problems and increased energy consumption in summer. Saleh (2015) study confirmed that even in warm regions, the thermal performance and energy-saving of closed balconies can be balanced throughout the annual by providing shading on the inside and outside of the closed balcony and by designing a closed balcony with air gaps and openable panes to effectively solve the problems of summer overheating.
By optimizing the design of the building envelope, a more comfortable and energy-efficient indoor environment can be created for residents (Mitterer et al., 2012; Ascione et al., 2016). Dhaka et al. (2012) evaluated the improvement in the energy efficiency of air-conditioned building masses using energy efficiency measures recommended by the National Energy Conservation Building Code (ECBC), The study recommends the implementation of envelope measures recommended in the building code to improve energy efficiency in warm climates. In addition, the study strongly recommends the use of roof insulation, a measure that alone provides a 20% energy savings. Wall insulation also provided significant energy savings. Florides et al. (2002) studied measures to reduce the heat load of modern houses in the subtropics and concluded that window gain is an important factor, and when Low-E double-glazed windows are used, annual savings in cooling load can be as high as 24% for a well-insulated house. This paper presents a comprehensive study of the effects of building envelope design, including balcony form, exterior walls, roof, floor, and window insulation performance, orientation, and the window-to-wall ratio of the building façade, on dwelling energy efficiency and indoor environment. It was found that the average winter temperature of open balconies with insulation was 0.6°C lower than that of closed balconies without insulation at maximum. When an enclosed balcony has a smaller external window area and is without insulation, it can provide an effective energy saving of 7.2% compared to an open balcony with insulation. A closed balcony design has strong applicability to dwellings in warm climate regions in winter.
In this study, the analysis of indoor thermal environment measurements and simulations of the subject dwelling is focused on indoor temperature and radiant temperature. Indoor relative humidity, wind speed, etc., were not specifically analyzed and measured. Therefore, the validity of the results of the thermal environment analysis in this paper includes indoor temperature and radiation temperature only. In addition, we lack the consideration of the adjacent building envelope and the shading of the adjacent buildings in the parameter setting of the model. The results of the applicability and rationality of the building envelope design for dwellings with closed balconies and insulation, etc., in this paper currently depend on whether it improves the indoor thermal environment and energy efficiency during heating periods. The economic benefits of this design should also be considered. The parameter settings and meteorological data in the modeling process in this paper represent only typical warm climate cities in Japan, due to possible differences in regulations, lifestyles, habits, etc., in each country. The applicability to other countries might need to be further explored.
5 Conclusion
The design of building envelope with different balcony forms, insulation, etc., is essential for the suitability of indoor thermal environment and energy efficiency of dwellings in different climatic conditions. In this paper, the city of Kitakyushu, Japan, located in a warm climate region, was chosen as the study area to investigate the measures for optimizing the building envelope of dwellings in winter in this region. The main findings are shown as follows.
(1) The results of the field measurements confirmed the effectiveness of the closed balcony space as an indoor-outdoor thermal buffer space. The orientation of the balcony was also considered another factor affecting the indoor thermal environment.
(2) Closed balcony design has strong applicability to houses in warm climate areas in winter. The simulation results showed that the heating load of the closed balcony is smaller than that of the open balcony. Compared with open balconies, closed balconies can reduce the heat load by 8%–24%, and closed balconies with insulation can reduce the heat load by 14%–36%. The relative reduction of the exterior glass window area can effectively improve the energy-saving effect by improving the insulation performance.
(3) South-facing closed balconies gain more positive impact on the indoor thermal environment and energy-saving performance than open balconies.
(4) The difference in the area ratio of exterior windows to exterior walls of closed balconies has no significant effect on the indoor thermal environment in winter and has an effect on the heat load of 5.0%–7.4% only when the orientation is south.
(5) Even closed balconies without insulation could obtain higher thermal environment gains than open balconies with insulation. Therefore, designing a particular type of closed balcony for the house in winter is more energy-efficient than insulation.
This paper proposes residential envelope designs that are adapted to specific environmental and climatic conditions. It contributes to providing residential designers with a measure of how to provide a comfortable residential thermal environment and energy efficiency for occupants through residential envelope design.
Data availability statement
The original contributions presented in the study are included in the article/Supplementary Material, further inquiries can be directed to the corresponding author.
Author contributions
XY and YR contributed to the design of the study. XY collated and analyzed the data. XY has analyzed the data of the manuscript. XY wrote the first draft of the manuscript. DS was involved in the revision of the manuscript. All authors contributed to manuscript revision, read, and approved the submitted version.
Conflict of interest
The authors declare that the research was conducted in the absence of any commercial or financial relationships that could be construed as a potential conflict of interest.
Publisher’s note
All claims expressed in this article are solely those of the authors and do not necessarily represent those of their affiliated organizations, or those of the publisher, the editors and the reviewers. Any product that may be evaluated in this article, or claim that may be made by its manufacturer, is not guaranteed or endorsed by the publisher.
References
Akasaka, Hiroshi (20002000). Expanded AMeDAS weather data. Proc. JSES/JWEA Jt. Conf. 31-36, 2000–1108. (In Japanese).
Ascione, F., de Masi, R. F., de Rossi, F., Ruggiero, S., and Vanoli, G. P. (2016). Optimization of building envelope design for nZEBs in Mediterranean climate: Performance analysis of residential case study. Appl. Energy 183, 938–957. doi:10.1016/j.apenergy.2016.09.027
Babaee, F., Fayaz, R., and Sarshar, M. (2016). The optimum design of sunspaces in apartment blocks in cold climate. Archit. Sci. Rev. 59, 239–253. doi:10.1080/00038628.2015.1077326
Building Research Institute (2017). Technical information on evaluation of energy consumption performance in compliance with the 2016 energy conservation standards (residential). Available at: https://www.kenken.go.jp/becc/house_h28manual.html (Accessed May 28, 2022).
Chan, A. L. S., and Chow, T. T. (2010). Investigation on energy performance and energy payback period of application of balcony for residential apartment in hong kong. Energy Build. 42, 2400–2405. doi:10.1016/j.enbuild.2010.08.009
Chan, A. L. S. (2015). Investigation on the appropriate floor level of residential building for installing balcony, from a view point of energy and environmental performance. a case study in subtropical hong kong. Energy 85, 620–634. doi:10.1016/j.energy.2015.04.001
Chun, C., Kwok, A., and Tamura, A. (2004). Thermal comfort in transitional spaces-basic concepts: literature review and trial measurement. Build. Environ. 39, 1187–1192. doi:10.1016/j.buildenv.2004.02.003
Dhaka, S., Mathur, J., and Garg, V. (2012). Combined effect of energy efficiency measures and thermal adaptation on air conditioned building in warm climatic conditions of India. Energy Build. 55, 351–360. doi:10.1016/j.enbuild.2012.09.038
Falchi, F., Cinzano, P., Duriscoe, D., Kyba, C. C. M., Elvidge, C. D., Baugh, K., et al. (2016). The new world atlas of artificial night sky brightness. Sci. Adv. 2, e1600377. doi:10.1126/sciadv.1600377
Fernandes, J., Pimenta, C., Mateus, R., Silva, S. M., and Bragança, L. (2015). Contribution of Portuguese vernacular building strategies to indoor thermal comfort and occupants’ perception. Buildings 5, 1242–1264. doi:10.3390/buildings5041242
Florides, G. A., Tassou, S. A., Kalogirou, S. A., and Wrobel, L. C. (2002). Measures used to lower building energy consumption and their cost effectiveness. Appl. Energy 73, 299–328. doi:10.1016/S0306-2619(02)00119-8
Hastings, S. R. (2004). “Breaking the “heating barrier”: Learning from the first houses without conventional heating,” in Energy and buildings. doi:10.1016/j.enbuild.2004.01.027
Hu, Z., Hu, H., and Huang, Y. (2018). Association between nighttime artificial light pollution and sea turtle nest density along florida coast: a geospatial study using viirs remote sensing data. Environ. Pollut. 239, 30–42. doi:10.1016/j.envpol.2018.04.021
IEEJ Outlook 2019 (2018). The Institute of energy economics. Japan. Available at: https://eneken.ieej.or.jp/data/8117.pdf (Accessed December 23, 2021).
ISO (2005). ISO 7730: Ergonomics of the thermal environment Analytical determination and interpretation of thermal comfort using calculation of the PMV and PPD indices and local thermal comfort criteria. Management 3.
Japanese association building mechanical and electrical engineers (2012). New HASP/ACLD operation manual. Available at: (In Japanese) https://www.jabmee.or.jp/hasp/(Accessed May 27, 2022).
Kazuaki, B., Takao, S., Hiroshi, Y., Suzuki, K., Shin−ichi Akaba, Y., Taleashi, I., et al. (1998). Study on the heating and cooling pattern and heating and cooling period in residential buildings on the basis of national scale surveys. J. Archit. Plan. Environ. Eng. 509, 41–47. Available at: https://www.jstage.jst.go.jp/article/aija/63/509/63_KJ00004222647/_pdf (Accessed May 27, 2022).
Kennedy, R., Buys, L., and Miller, E. (2015). Residents’ experiences of privacy and comfort in multi-storey apartment dwellings in subtropical Brisbane. Sustain. Switz. 7, 7741–7761. doi:10.3390/su7067741
Land Statistical Survey, (2019). Statistics bureau of Japan. Available at: https://www.stat.go.jp/data/jyutaku/2018/pdf/g_gaiyou.pdf (Accessed December 23, 2021).
Lu, Feijia, Manami, T. S. U. J. I., Wang, Danyang, and Komatsu, Hisashi (2004). A study on the refurbishment of the high-rise apartment buildings’ balconies in Shanghai. Architectural Institute of Japan.
Ministry of Land (2020). Energy conservation standard area classification. Available at: https://www.mlit.go.jp/jutakukentiku/shoenehou_assets/img/library/chiikikubun-sinkyuu.pdf (Accessed June 3, 2022).
Mitterer, C., Künzel, H. M., Herkel, S., and Holm, A. (2012). Optimizing energy efficiency and occupant comfort with climate specific design of the building. Front. Archit. Res. 1, 229–235. doi:10.1016/j.foar.2012.06.002
Mueller, N., Rojas-Rueda, D., Basagaña, X., Cirach, M., Hunter, T. C., Dadvand, P., et al. (2017). Urban and transport planning related exposures and mortality: a health impact assessment for cities. Environ. Health Perspect. 125, 89–96. doi:10.1289/EHP220
Murtyas, S., Hagishima, A., and Kusumaningdyah, N. H. (2020). On-site measurement and evaluations of indoor thermal environment in low-cost dwellings of urban Kampung district. Build. Environ. 184, 107239. doi:10.1016/j.buildenv.2020.107239
NHK, NHK Broadcasting Culture Research Institute (2021). National life time survey of Japan. Available at: https://www.nhk.or.jp/bunken/research/yoron/pdf/20210521_1.pdf (Accessed May 27, 2022).
Nieuwenhuijsen, M. J. (2021). New urban models for more sustainable, liveable and healthier cities post covid19; reducing air pollution, noise and heat island effects and increasing green space and physical activity. Environ. Int. 157, 106850. doi:10.1016/j.envint.2021.106850
Omrani, S., Garcia-Hansen, V., Capra, B. R., and Drogemuller, R. (2017). On the effect of provision of balconies on natural ventilation and thermal comfort in high-rise residential buildings. Build. Environ. 123, 504–516. doi:10.1016/j.buildenv.2017.07.016
Ribeiro, C., Ramos, N. M. M., and Flores-Colen, I. (2020). A review of balcony impacts on the indoor environmental quality of dwellings. Sustain. Switz. 12, 6453. doi:10.3390/su12166453
Sakamoto, Shogo (2013). Household activity-schedules generation method using national time use survey and PT survey. Pap. Environ. lInformation Sci. 27, 139–144. Available at: https://www.jstage.jst.go.jp/article/ceispapers/ceis27/0/ceis27_139/_pdf/-char/ja (Accessed May 27, 2022).
Sakamoto, Y, Takao, S., Yasuo, K, Miura, N., Nakamura, M., Sunagawa, M., et al. (2014). Basic survey on the target level of high thermal insulation of housing. Available at: https://www.kenken.go.jp/japanese/contents/publications/data/154/index.html (Accessed June 3, 2022).
Saleh, P. H. (2015). Thermal performance of glazed balconies within heavy weight/thermal mass buildings in beirut, Lebanon’s hot climate. Energy Build. 108, 291–303. doi:10.1016/j.enbuild.2015.09.009
Sedaghat, A., and Sharif, M. (2022). Mitigation of the impacts of heat islands on energy consumption in buildings:a case study of the city of tehran, iran. Sustain. Cities Soc. 76, 103435. doi:10.1016/j.scs.2021.103435
Tomonari, W. A. K. U., Seiichi, F. U. K. A. O., and Kadowaki, Kozo (2003). A study on the inner balcony in multi-unit residential building. Architectural Institute of Jaoan. (In Japanese).
Tomonari, W. A. K. U., Seiichi, F. U. K. A. O., and Kadowaki, Kozo (2008). Analysis on the actual conditions of balcony design of condominium. Architectural Institute of Japan, 997
Touma, A. al, and Ouahrani, D. (2018). The selection of brise soleil shading optical properties for energy conservation and glare removal: A case study in Qatar. J. Build. Eng. 20, 510–519. doi:10.1016/j.jobe.2018.08.020
Voss, K. (2000). Solar energy in building renovation - results and experience of international demonstration buildings. Energy Build. 32, 291–302. doi:10.1016/S0378-7788(00)00052-9
Wilson, M. P., Jorgensen, O. B., and Johannesen, G. (2000). Daylighting, energy and glazed balconies: a study of a refurbishment project in engelsby, near flensberg, Germany. Light. Res. Technol. 32, 127–132. doi:10.1177/096032710003200304
Wing Chau, K., Kei Wong, S., and Yim Yiu, C. (2004). The value of the provision of a balcony in apartments in Hong Kong. Prop. Manag. 22, 250–264. doi:10.1108/02637470410545020
Wolkoff, P., Azuma, K., and Carrer, P. (2021). Health, work performance, and risk of infection in office-like environments: the role of indoor temperature, air humidity, and ventilation. Int. J. Hyg. Environ. Health 233, 113709. doi:10.1016/j.ijheh.2021.113709
Wolkoff, P., and Kjærgaard, S. K. (2007). The dichotomy of relative humidity on indoor air quality. Environ. Int. 33, 850–857. doi:10.1016/j.envint.2007.04.004
Yang, Jinho, Kim, Hyuntae, and Tanabe, Shin-ichi (2013). “Comparison of simulation results between NewHASP/ACLD and ENEGYPLUS,” in Techinical papers of annual meeting the society of heating, air-conditioning and sanitary engineers of Japan. Available at: (In Japanese). doi:10.18948/shasetaikai.2013.5.0_217
Keywords: open balcony, closed balcony, indoor thermal environment, multiple-dwelling house, heat load, warm climate region
Citation: Yuan X, Ryu Y and Sekartaji D (2022) Effect of balcony forms difference on indoor thermal environment and energy saving performance of multiple-dwelling house. Front. Energy Res. 10:891946. doi: 10.3389/fenrg.2022.891946
Received: 08 March 2022; Accepted: 04 July 2022;
Published: 05 August 2022.
Edited by:
Xiaoliang Zeng, Shenzhen Institutes of Advanced Technology (CAS), ChinaReviewed by:
Yingdong He, University of California, Berkeley, United StatesTimothy O. Adekunle, University of Hartford, United States
Faming Wang, KU Leuven, Belgium
Copyright © 2022 Yuan, Ryu and Sekartaji. This is an open-access article distributed under the terms of the Creative Commons Attribution License (CC BY). The use, distribution or reproduction in other forums is permitted, provided the original author(s) and the copyright owner(s) are credited and that the original publication in this journal is cited, in accordance with accepted academic practice. No use, distribution or reproduction is permitted which does not comply with these terms.
*Correspondence: Xin Yuan, uanin03@gmail.com