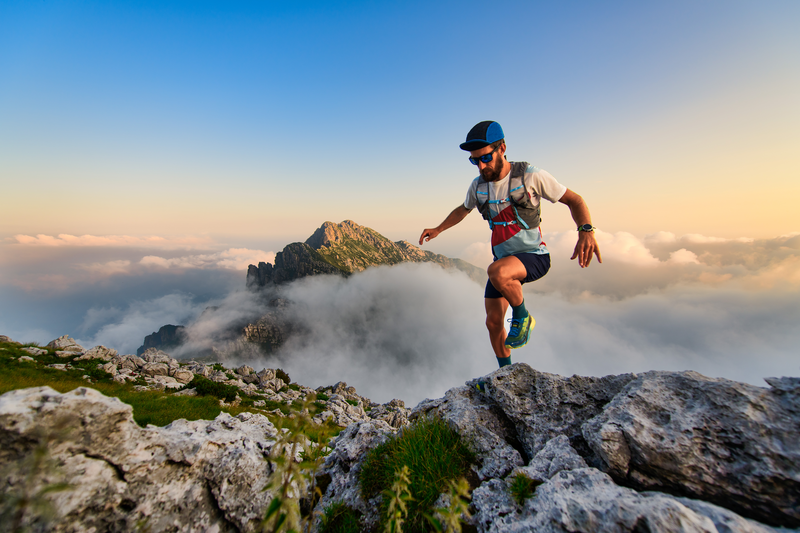
95% of researchers rate our articles as excellent or good
Learn more about the work of our research integrity team to safeguard the quality of each article we publish.
Find out more
EDITORIAL article
Front. Energy Res. , 06 April 2022
Sec. Solar Energy
Volume 10 - 2022 | https://doi.org/10.3389/fenrg.2022.885894
This article is part of the Research Topic Recent Advances in Solar-driven Thermochemical Fuel Production and Thermal Energy Storage View all 10 articles
Editorial on the Research Topic
Recent Advances in Solar-Driven Thermochemical Fuel Production and Thermal Energy Storage
Renewable energies are the core of the transition to a decarbonized and more sustainable energy sector. Among them, solar energy shows great potential since 430 EJ per hour of sunlight energy hit the Earth, which is about the annual world energy consumption (Lewis and Nocera, 2006). However, solar energy radiation is not equally distributed along the planet surface and it suffers from an inherent intermittence problem. Therefore, development of efficient and cost-effective energy storage technologies is fundamental for the implementation of solar energy worldwide. In this respect, concentrated solar power (CSP) plants incorporate thermal energy storage (TES) units that allows for a round-the-clock electricity production and are radically cheaper than other storage solutions such as batteries (Carrillo et al., 2019; Bayon et al., 2021). In addition, the high temperatures achieved in concentrated solar thermal (CST) plants based on cavity receiver, with heliostat field or dish concentrators, can be used to drive the thermal reduction of metal oxides than can be subsequently re-oxidized with steam and/or CO2 producing H2 or syngas, respectively. These renewable fuels can be used for industry and transportation or as precursors for liquid fuels or chemicals.
This Research Topic focuses on the recent advances both in thermal energy storage and in thermochemical cycles for hydrogen production. The published contributions cover several key aspects for the progress of these technologies, e.g., storage media and reactors development, computational tools for the discovery of new materials and techno-economic assessment of thermochemical energy storage (TCS) units. Regarding thermal energy storage, both latent and thermochemical technologies have been addressed. Gokon et al. reported on the use of copper-germanium alloys as phase-change materials (PCMs) for high-temperature latent heat storage. These PCM alloys, with varying the Cu-Ge ratio, showed chemical compatibility with alumina or SiC containers, albeit not with stainless steel or Inconel tanks. The authors assessed the thermophysical properties of these alloys which showed reversible phase transitions for 20 cycles, with a latent heat at 644°C of 232.8 kJ kg−1.
In the case of thermochemical energy storage, contributions covered both carbonate and redox-based systems, which are amongst the most promising. Regarding the former, Fedunik-Hofman et al. found that by supporting CaO on Ca3Al2O6 and Dy2O3, it was possible to overcome the multicycle deactivation caused by sintering of CaCO3/CaO particles. These novel materials exhibit an exothermic heat exchange of 582.2 kJ kg−1, retaining a carbonation capacity of 82.7% over the course of 40 calcination-carbonation cycles.
Wild et al. developed a reactor combining sensible and thermochemical heat storage. The thermochemical unit was based on carbonation/calcination reactions, using a tubular packed bed of CaO:MgO in a 58:42 wt%. The materials were tested in different particle conformations over 80 consecutive cycles. Agglomerates exhibited higher energy storage density (866 kJ kg− 1) than granules (450 kJ kg−1). However, the latter were more stable over cycling. These results validated previous heat and mass models performed by the authors, proving the feasibility of this modular reactor design.
An important piece of experimental research on materials for high temperature thermochemical reactions encompasses the use of electric furnaces in which a fast response for heating and cooling is required. In this regard, Gao et al. developed a low-cost radiant heater that could replace more expensive options, such as infrared furnaces. Their design could achieve temperatures above 1,500°C, with heating/cooling rates of 400°C min−1. Tests of more than 10,000 cycles and 350 h of operation confirmed the robustness of this device.
TCS based on reduction/oxidation reactions of metal oxides was also addressed in this special issue. Miller et al. report on the use of CaMnO3-δ perovskite oxides for high temperature energy storage. The authors analyzed the partial replacement of Ca and Mn in the perovskite formula by using a plethora of metal cations. The results indicated that CaAl0.2Mn0.8O3-δ and CaTi0.2Mn0.8O3-δ were the most promising materials, being the former the preferred choice in terms of cost.
Two more contributions concerning techno-economic assessments of redox-based TCS were published. Buck et al. analyzed three redox systems in a CSP plant with a particle receiver, namely, Co3O4/CoO, (Mn0.75Fe0.25)3O4/(Mn0.75Fe0.25)2O3 and the Ca0.9Sr0.1MnO3-δ perovskite compositions. The cobalt-based oxide requires lower storage mass and volume. However, its elevated cost makes this choice not economically appealing. In this sense, the perovskite-based system, which presents a lower price due the use of abundant elements, is an attractive option for further development.
Gorman et al. evaluated a CSP plant exploiting an air Brayton cycle for energy dispatch and using CaAl0.2Mn0.8O3-δ as TCS storage media. Their results indicated an increase in the plant capacity factor when using the redox-based energy storage system. In addition, their model predicted an LCOE of 5.98 ¢ kWhe−1, for a plant with 12 h of storage, which is very close to the U.S. Department of Energy 2030 target of 5.00 ¢ kWhe−1, illustrating the promise of redox-based TCS.
Two works were devoted to the production of hydrogen via thermochemical cycles at high temperatures. Boretti et al. contributed with a perspective on the production of green hydrogen using a beam-down concentrator coupled with a TES unit. The authors proposed the use of the three-step sulfur-iodine cycling scheme for hydrogen production.
Finally, Park et al. reported the discovery of a new redox oxide with promising properties for 2-step thermochemical hydrogen production. By using computational methods, the authors found that Gd0.5La0.5Co0.5Fe0.5O3 could have remarkable potential for water splitting. Experiments confirmed the ability of this perovskite oxide to produce hydrogen. Namely, after reduction in inert gas at 1,350°C, the material produced 101 μmol g−1 and 141 μmol g−1 of hydrogen, when the water splitting reaction was performed at temperatures of 850 and 1,000°C, respectively. This work shows the importance of computational assisted discovery of new class of oxides for solar-driven thermochemical cycles for hydrogen production and it could be extended to thermochemical heat storage materials.
We would like to thank all the authors and reviewers for their high quality contribution to this Research Topic. This Special Issue has covered a broad spectrum of relevant aspects that are key to continue with the development of these technologies, including computational discovery of new materials, reactor development and techno-economic assessments that validate the feasibility of these sustainable technologies.
AC prepared the editorial, AB, JC and EM provided feedback and revised the draft.
The authors declare that the research was conducted in the absence of any commercial or financial relationships that could be construed as a potential conflict of interest.
All claims expressed in this article are solely those of the authors and do not necessarily represent those of their affiliated organizations, or those of the publisher, the editors and the reviewers. Any product that may be evaluated in this article, or claim that may be made by its manufacturer, is not guaranteed or endorsed by the publisher.
AC thanks the support of a fellowship from “la Caixa” Foundation (ID 100010434). The fellowship code is LCF/BQ/PI20/11760015. JC acknowledge financial support from ACES 2030 (P2018/EMT-4319) from “Comunidad de Madrid” and European Structural Funds.
Bayon, A., Carrillo, A. J., Mastronardo, E., and Coronado, J. M. (2021). “Thermochemical Heat Storage at High Temperature,” in Adv. Chem. Eng. Editor W. Lipiński. 1st ed. (Elsevier), 247–295. doi:10.1016/bs.ache.2021.10.004
Carrillo, A. J., González-Aguilar, J., Romero, M., and Coronado, J. M. (2019). Solar Energy on Demand: A Review on High Temperature Thermochemical Heat Storage Systems and Materials. Chem. Rev. 119, 4777–4816. doi:10.1021/acs.chemrev.8b00315
Keywords: concentrated solar power, thermal energy storage, solar fuels, thermochemical cycles, hydrogen production
Citation: Carrillo AJ, Bayon A, Coronado JM and Mastronardo E (2022) Editorial: Recent Advances in Solar-Driven Thermochemical Fuel Production and Thermal Energy Storage. Front. Energy Res. 10:885894. doi: 10.3389/fenrg.2022.885894
Received: 28 February 2022; Accepted: 21 March 2022;
Published: 06 April 2022.
Edited and reviewed by:
Michael Folsom Toney, University of Colorado Boulder, United StatesCopyright © 2022 Carrillo, Bayon, Coronado and Mastronardo. This is an open-access article distributed under the terms of the Creative Commons Attribution License (CC BY). The use, distribution or reproduction in other forums is permitted, provided the original author(s) and the copyright owner(s) are credited and that the original publication in this journal is cited, in accordance with accepted academic practice. No use, distribution or reproduction is permitted which does not comply with these terms.
*Correspondence: Alfonso J. Carrillo, YWxjYXJkZWxAaXRxLnVwdi5lcw==; Alicia Bayon, YWJheW9uc2FAYXN1LmVkdQ==; Juan M. Coronado, am0uY29yb25hZG9AY3NpYy5lcw==; Emanuela Mastronardo, ZW1hbnVlbGEubWFzdHJvbmFyZG9AdW5pbWUuaXQ=
Disclaimer: All claims expressed in this article are solely those of the authors and do not necessarily represent those of their affiliated organizations, or those of the publisher, the editors and the reviewers. Any product that may be evaluated in this article or claim that may be made by its manufacturer is not guaranteed or endorsed by the publisher.
Research integrity at Frontiers
Learn more about the work of our research integrity team to safeguard the quality of each article we publish.