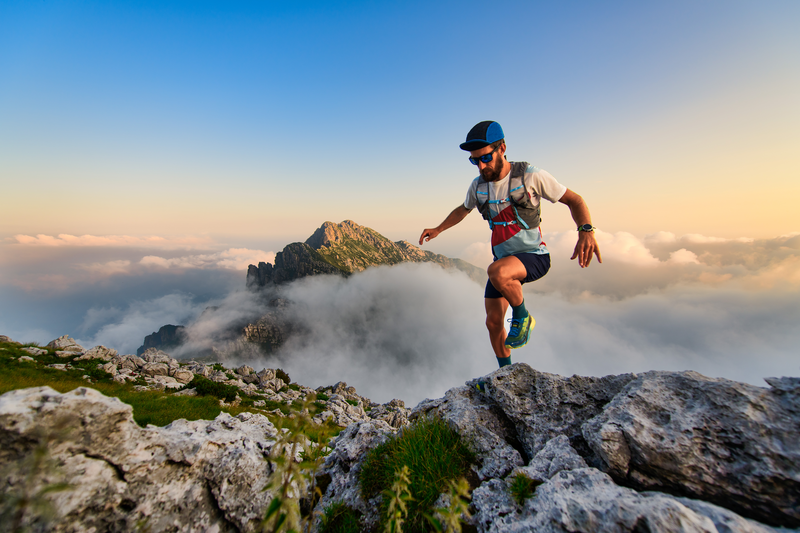
94% of researchers rate our articles as excellent or good
Learn more about the work of our research integrity team to safeguard the quality of each article we publish.
Find out more
ORIGINAL RESEARCH article
Front. Energy Res. , 13 June 2022
Sec. Bioenergy and Biofuels
Volume 10 - 2022 | https://doi.org/10.3389/fenrg.2022.878597
This article is part of the Research Topic Advancements in Biomass Feedstock Preprocessing: Conversion Ready Feedstocks, Volume II View all 16 articles
Arthrospira (Spirulina) platensis is a freshwater cyanobacterium that is commercially produced as a food source on a global scale and considered safe for human consumption. After C-phycocyanin (C-PC) extraction, the waste cell residue (WCR) is composed of nutrients with 30% protein content. Here, the potential of WCR as a nitrogen source for Bacillus coagulans cultivation and bioproducts was evaluated. Nitrogen substitute from WCR under different conditions of 20—100 g L−1 was performed. B. coagulans cultivation was achieved with maximal viable cells at 7.6–9.5 log CFU mL−1. Increasing WCR gave decreasing lactate production. The highest lactate production at 27 g L−1 was achieved from WCR 20 g L−1, highlighting the potential use of A. platensis waste biomass residue as a nitrogen source for the growth of lactic acid bacteria and zero-waste biotechnology methodology. The utilization of renewable resources is a crucial step toward developing a sustainable industrial society.
Arthrospira (Spirulina) platensis cyanobacteria have attracted increased commercial interest due to their high protein content, essential amino acids, fatty acids, and pigments (De la Jara et al., 2018). The rich source of biochemical composition has become an interest in the food and health industry, and Arthrospira has shown value from the medical point of view (Furmaniak et al., 2017). Microalgal biomass is a valuable natural source of bioactive compounds that can be used in a variety of applications including the food sector (Ferreira et al., 2021), pharmaceuticals and nutraceuticals (Jha et al., 2017; Mehariya et al., 2021), therapeutic potentials (Khavari et al., 2021), cosmetics (Yarkent et al., 2020), biodiesel and biogas (González-González et al., 2018), and wastewater bioremediation (De Souza et al., 2022). Arthrospira, also known as Spirulina, is a well-known microalgal strain that has been globally cultured on a large commercial scale (Mostafa and El-Gendy, 2017). A. platensis biomass showed high potential as a source of various value-added product applications including bioethanol from carbohydrate and feed supplements because of its high protein and fatty acid contents (Raja et al., 2016). Previous studies demonstrated bioethanol production from A. platensis biomass and biomethane production from waste cell residue (WCR) (Rempel et al., 2019). Chng et al. (2016) studied the production of bioethanol using lipid-extracted biomass from Scenedesmus dimorphus microalgae. Bioproducts from microalgae or waste cell residues are directly produced by fermentation or hydrolysis of the fraction before fermentation. Recent studies have shown the effectiveness of Arthrospira in the prevention of diabetes as an antiviral, immune-stimulator, and anticancer agent, with digestive improvement as well as growth of Lactobacilli (Christaki et al., 2011; Nicoletti, 2016). Several products from microalgal and cyanobacterial biorefineries with minimal waste outputs have been approved for the powerful valorization of biomass (Prabha et al., 2022).
Lactic acid fermentation as a food preservative method significantly improves the safety, shelf life, and nutritional properties of foods (De Marco Castro et al., 2019; Thompson et al., 2020) and is utilized in around 70% of food industry products (Eş et al., 2018). Fermentative biorefineries have wide-ranging product potential including fermented vegetables, fermented milk, meat industries, bio-preservatives, probiotics chemicals, biofuels, and pharmaceuticals (Raj et al., 2022).
Moreover, lactic acid fermentation has the potential for green production, biodegradation, and biocompatible polylactic acid (PLA) substitution for petroleum-based plastic (Nduko and Taguchi, 2021). Lactic acid fermentation can produce three forms of lactic acid, namely, l-lactate, d-lactate, or both d- and l-lactates depending on the microorganism used (Prasirtsak et al., 2019). Bacillus coagulans is a lactic acid-producing, spore-forming, catalase-positive bacterium with an optimal growth temperature of 35–50°C. These bacteria can utilize glucose, sucrose, lactose, and mannitol to produce l-lactic acid (Cao et al., 2020). Moreover, B. coagulans has also been accorded the Generally Recognized as Safe (GRAS) status by the US Food and Drug Administration (FDA) (Endres et al., 2009; Hazards, 2013). B. coagulans has significant benefits to the host immune system (Cao et al., 2020). Currently, B. coagulans is used as a functional food in chocolate, ice cream, and pasta. The spore-forming nature of B. coagulans is comparable to other probiotic bacterial strains, with high stability in functional food products (Majeed et al., 2016). Previous studies reported that lactic acid bacteria (LAB) have the ability to degrade the cell walls of plants and cyanobacteria through hydrolysis, resulting in the conversion of complex organic compounds such as polysaccharides, lipids, and proteins within the cells into smaller molecules with enhanced properties (De Marco Castro et al., 2019).
Algae and cyanobacteria have high nutritional values as suitable substrates for fermented food production. Fermented products from seaweed, microalgae, and Arthrospira were obtained as plant-derived substrates using various fermentation processes of lactic acid bacteria, yeast, or mixed cultures of microorganisms. These products are highly desired in the market with bioproduct applications (Gupta and Abu-Ghannam, 2011; Uchida and Miyoshi, 2013; Niccolai et al., 2019). Most previous reports studied microalgae as feedstock for lactic acid production using lactobacilli as the lactic acid bacteria. The hydrolysate of microalgae Chlorella vulgaris ESP-31 was used as feedstock for lactate production from Lactobacillus plantarum 23 (Chen et al., 2020) and L. brevis (Ścieszka and Klewicka, 2020), while whole freeze-dried Arthrospira biomass was used as the substrate for lactate production by L. plantarum ATCC 8014 (Niccolai et al., 2019; Niccolai et al., 2020) and wet Arthrospira biomass was used as the substrate in fermentation by L. plantarum ATCC 8014 for nutraceutical properties (De Marco Castro et al., 2019). However, large-scale lactate production from microorganisms involves the high cost of fermentation media as one of the most critical bottlenecks in industrial lactate production (Cubas-Cano et al., 2018). Lactic acid bacteria (LAB) require complex nutrition that consists of high carbon sources, amino acids, vitamins, and nucleotides (Mokoena, 2017). Nitrogen, vitamins, and other nitrogenous growth-stimulating elements are abundant in yeast extract and peptone (Abbasiliasi et al., 2017). Organic nitrogen sources such as yeast extract, peptone, and other proteinous substrates must be added to LAB culture media, increasing the cost of medium formulation. As a result, it is important to discover low-cost alternatives for these expensive ingredients. Bacillus strains can grow and produce l-lactic acid under minimal nutritional requirements (Poudel et al., 2016).
Few studies have focused on producing lactate from waste cells of microalgal biomass in a biorefinery as a zero-waste process, while lactate production from Bacillus coagulans has never been reported. Waste microalgae and cyanobacteria can be used as an alternative nitrogen source for lactic acid production, representing bioproducts as a renewable resource with reduced production cost. Therefore, this study was conducted to investigate the potential of novelty waste A. platensis cell residues after C-phycocyanin extraction in a culture medium by B. coagulans ATCC 7050 for lactate production as a renewable resource.
Analytical grade Zarrouk medium composed of sodium bicarbonate, sodium nitrate, dipotassium phosphate, potassium sulfate, sodium chloride, magnesium sulfate, calcium chloride, iron sulfate, ethylenediaminetetraacetic acid, and other reagents was purchased from Ajax Finechem Pty Ltd. (Auckland, New Zealand). GYP medium composed of glucose monohydrate, granulated yeast extract, peptone from meat, and other reagents was purchased from EMD Millipore Corporation (Burlington, United States). Bacillus coagulans ATCC 7050 was purchased from the American Type Culture Collection (ATCC, United States).
Arthrospira (Spirulina) platensis IFRPD 1182 was obtained from the Algae Laboratory at the Institute of Food Research and Product Development, Kasetsart University, Thailand. A. platensis was maintained and cultivated in a modified Zarrouk medium (Pan-utai et al., 2020) in photobioreactors and then scaled up to a 200-L outdoor open raceway pond. The biomass of A. platensis was grown to log phase and then harvested by nylon membrane filtration. The harvested cells were cleaned with fresh water to remove any residual culture medium. A. platensis biomass preparation was dried in a hot air oven (Thermo Scientific, Germany) at 60°C for 6 h and then milled to 0.5 mm particle size.
C-phycocyanin (C-PC) was extracted from A. platensis biomass under optimal conditions (Pan-utai et al., 2018) of biomass solvent ratio 1:15 (w/v) with 10 mM phosphate buffer at pH 7.0 and incubated under a controlled extraction temperature of 25°C for 24 h. The C-phycocyanin (C-PC) supernatant was then separated by centrifugation at 10,000
The prepared A. platensis biomass and waste cell residue (WCR) were analyzed to determine their biochemical compositions following NREL methods. Moisture content was determined by oven-drying at 105°C to constant weight (Sluiter et al., 2008a). Ash content was determined by ignition of the dried samples in an electric furnace at 550°C (Sluiter et al., 2008b). Protein content was determined by the Kjeldahl method (Hames et al., 2008), and lipid content was determined using a modified Bligh and Dyer method (Pan-utai et al., 2019). Briefly, the samples were suspended in distilled water, methanol, and chloroform at a ratio of 0.8:2.0:1.0 and mixed well. The mixture was ultrasonically homogenized for 15 min and then separated by centrifuging at 6,153
Chlorophyll was extracted from the samples with methanol and stored at 4°C for 24 h. The optical density of the supernatant was measured at 662 and 645 nm. The chlorophyll content is calculated from the combination of chlorophyll a and b using the following Eqs.2, 3 (Pan-utai et al., 2021):
Glucose in waste cell residues was determined by analysis of the carbohydrate in the biomass following Yuan et al. (2016). WCR A. platensis biomass was hydrolyzed by 72% (w/w) sulfuric acid at 30°C for 1 h. The acid concentration of the samples was then adjusted to 4% (w/w), and the samples were sterilized at 121°C for 1 h. The glucose concentration in the supernatant hydrolysate was determined on a Hewlett-Packard HP 1100 Series HPLC system with a refractive index detector (Agilent Technologies, United States), using a cross-linked resin hydrogen ionic 300 × 7.8 mm Aminex HPX-87H (9 µm) column (Bio-Rad). The column oven was set at 50°C, and the mobile phase was 5 mM sulfuric acid. The flow rate was set at 0.5 ml min−1 using a refractive index detector at 40°C. Glucose contents in the samples are calculated according to Eq. 4:
Lactate production was performed by B. coagulans ATCC 7050 maintained in GYP medium and 10% skim milk and stored at −20°C. The GYP medium had the following composition (g L−1): glucose 10 g, yeast extract 5 g, peptone 5 g, KH2PO4 0.25 g, K2HPO4 0.25 g, and salt solution 10 ml consisting of (L−1) MgSO4.7H2O 40 g, MnSO4.5H2O 2 g, FeSO4.7H2O 2 g, and NaCl 2 g. The pH value of the GYP medium was adjusted to 6.8 (Thitiprasert et al., 2017). Primary inoculum preparation of B. coagulans ATCC 7050 was cultured in GY medium consisting of (g L−1): glucose 10 g, yeast extract 15 g, NH4Cl 4 g, KH2PO4 0.5 g, K2HPO4 0.5 g, and salt solution 20 ml. The pH value of the GY medium was adjusted to 6.8 (Jaiaue et al., 2021). The inoculum culture was placed in an incubator shaker at 200 rpm, with temperature controlled at 37°C for 18 h. Then, 10% (v v−1) of B. coagulans ATCC 7050 cells were transferred to secondary inoculum preparation, cultured at 37°C, 200 rpm for 6 h, and used as the starter for B. coagulans cultivation.
WCR was used as the supplemented feedstock for lactate production under different concentrations of 20, 40, 60, 80, and 100 g L−1 in the culture medium. The control experiment was conducted without WCR. The medium formulation was modified by the culture medium corresponding to lactate fermentation from 120 g L−1 of initial glucose concentration (Tolieng et al., 2018). WCR was used to replace yeast extract in the culture medium, consisting of (g L−1): glucose 120 g, NH4Cl 2 g, KH2PO4 0.25 g K2HPO4 0.25 g, and salt solution 10 ml. Then, the culture medium was sterilized at 121°C for 15 min. B. coagulans ATCC 7050 inoculum at 10% (v v−1) was transferred into the culture medium for growth and lactate production. The cultivation was performed at a working volume of 50 ml medium in a 250-ml Erlenmeyer flask. The culture was incubated at 37°C in a shaker at 200 rpm. Fermented samples were collected at the start of fermentation and every 12 h for viable cell growth, glucose, and lactic acid determination. The pH was measured using a pH meter (Lab 850, Schott, Germany).
B. coagulans ATCC 7050 was collected during the fermentation process for viable cell determination. Colony-forming units (CFU) were counted in 1 ml sample collected from the fermentation broth. The fermentation broth was diluted to an appropriate multiple with sterile water, and then 100 µL sample was spread on a nutrient agar (NA) plate and cultured at 37°C for 48 h.
Fermentation samples were collected and centrifuged at 6,153
Viable cells of B. coagulans ATCC 7050 were assessed for growth and lactate production, and the kinetic parameters were calculated. The maximal value of viable cell growth was
The lactate yield coefficient is calculated using Eq. 6:
where
A. platensis IFRPD 1182 was used as the initial substrate, which was prepared as both microalgal biomass and waste cell residue (WCR) from C-PC extraction and also used as the substrate for fermentation as of renewable resource. Biochemical compositions of Arthrospira platensis IFRPD 1182 microalgal biomass and WCR are shown in Supplementary Table S1. The initial A. platensis biomass had the highest protein and C-PC contents. C-PC is a blue pigment composed of proteins. After C-PC extraction, the C-PC remaining contained 8.33% (mg g−1) as around 12.6% of C-PC in the initial biomass. However, 30% (DW) protein content and more than 63% of the initial biomass, including other compositions, remained in the WCR. Therefore, the potential of the remaining WCR nutrition was studied as an alternative nitrogen source for lactate production.
The potential of WCR substitution for nitrogen sources in the fermentation of lactic acid bacteria was studied as a low-cost medium and renewable resource. Lactate fermentation by B. coagulans ATCC 7050 with different WCR concentrations at 20–100 g L−1 substituted for yeast extract as the alternative nitrogen source was studied in the culture broth. Parameters of cell growth, glucose consumed, and lactate produced are shown in Figure 1. Various WCR concentrations were studied to substitute nitrogen sources in the fermentation medium of B. coagulans ATCC 7050. The fermentation medium without WCR and yeast extract was used as the control (Figure 1A). B. coagulans ATCC 7050 cells grown at different WCR concentrations and constant glucose of 120 g L−1 reached exponential values within 12 h of fermentation, while glucose concentration slightly reduced at 48 h of fermentation. A maximal lactate concentration was obtained after 12 h of fermentation at 4.5 g L−1. The results from the control experiments without a nitrogen source in the fermentation medium showed that B. coagulans was not appropriate for cell growth and lactate production. Fermentation in all experiments produced by-products, shown in Figure 2. At longer fermentation time, acetate and ethanol production increased with also large amounts of by-products.
FIGURE 1. Time course of B. coagulans ATCC 7050 growth and lactate production under various conditions as (A) control, (B) WCR 20 g L−1, (C) WCR 40 g L−1, (D) WCR 60 g L−1, (E) WCR 80 g L−1, and (F) 100 g L−1. All values are represented as means (±SD).
FIGURE 2. Time course of B. coagulans ATCC 7050 growth and acetate and ethanol production under various conditions as (A) control, (B) WCR 20 g L−1, (C) WCR 40 g L−1, (D) WCR 60 g L−1, (E) WCR 80 g L−1, and (F) 100 g L−1. All values are represented as means (±SD).
Viable B. coagulans ATCC 7050 growth at different WCR concentrations during fermentation are shown in Figures 1B–F. The results indicated that higher WCR concentration led to higher viable cell growth, while glucose as the carbon source in the culture media was almost completely consumed by B. coagulans at the end of fermentation. Lactate production at different treatments increased with cell growth and fermentation time. Supplementary Table S2 shows the pH values during fermentation at various treatments. B. coagulans ATCC 7050 growth and lactate production led to an increase in acidity from 6 to 4.6. Maximal B. coagulans ATCC 7050 viable growth at various WCR concentrations without a nitrogen source is shown in Figure 2. B. coagulans ATCC 7050 grew and increased during fermentation. Maximal cell growth at various WCR concentrations ranged from 7.6 to 9.5 log CFU mL−1 (Figure 3). Increasing WCR concentration in the fermentation media gave viable cell growth, whereas viable B. coagulans ATCC 7050 decreased in the treatment without WCR and other nitrogen sources (control experiment).
FIGURE 3. Maximal B. coagulans ATCC 7050 growth using waste Arthrospira cell residues at various concentrations. All values are represented as mean (±SD).
Parameters of B. coagulans ATCC 7050 fermentation at different WCR conditions for potential lactate production are shown in Supplementary Table S3. Maximal lactate concentration (
Arthrospira is well known as a rich protein source with high nutritional value. The nutritional composition of WCR showed useful protein, carbohydrate, and lipid content, with C-phycocyanin and chlorophyll contents of 8.33 ± 0.54 and 13.43 ± 0.17 mg g−1 DW, respectively. Previous reports recorded that wet biomass of Arthrospira gave enhanced nutraceutical properties by fermentation with Lactobacillus plantarum (De Marco Castro et al., 2019). Microalgal biomass of A. platensis was used as feedstock for biogas production (Dębowski et al., 2020), while A. platensis solid-state fermentation with LAB was performed for aromatic profile evaluation (Martelli et al., 2021). Generally, simple sugars such as glucose and sucrose are used as carbon sources for lactate fermentation (Olszewska-Widdrat et al., 2020). Most previous reports focused on Arthrospira with complete nutritional enrichment of microalgae. Carbohydrates from microalgae can be converted to fermentable sugars by hydrolytic processes. However, the fermentation requires large amounts of carbohydrate at up to more than 70% DW of the microalgal biomass, limiting control in large-scale cultivation of carbohydrate-enriched Arthrospira (Liu et al., 2019). Our results showed glucose content from WCR biomass using acid hydrolysis followed by HPLC determination as 35.50 ± 0.13% DW. Various WCR concentrations were used as feedstock addition fermentation at 20, 40, 60, 80, and 100 g L−1, with hydrolyzed glucose contents of 7.10, 14.20, 21.30, 28.40, and 35.50 g L−1, respectively. WCR was sterilized at 121°C for 15 min without pretreatment with acid hydrolysis and performed the same as culture broth giving glucose content at only 0.3% DW. Thus, the addition of WCR substitute in culture media at 0.06–0.30 g L−1 gave reduced glucose with increasing media formulation. Our results showed mainly protein content in the biochemical composition of nutrition values from WCR. Yeast extract is a nutrient source of vitamins and amino acids for microorganism growth, with high cost at approximately 38% of the total medium cost in lactate production (Meng et al., 2012; Ma et al., 2014). Therefore, WCR shows significant potential as a source of nitrogen feedstock to produce bioproducts. In our study, fermentation of WCR as the nitrogen source was evaluated for B. coagulans growth and lactate production.
B. coagulans cultivation from WCR addition in culture media at different loadings showed variable cell growth and lactate production. Increasing WCR gave higher maximal viable cell growth because the biomolecules of WCR containing amino acids, vitamins, and proteins supported cell growth. The control experiments without WCR and nitrogen source addition found that B. coagulans could use glucose as the carbon source. However, cell growth limitation was stimulated by other nutrients necessary for complete cell growth. Oh et al. (2003) confirmed that yeast extract was the most effective nitrogen source for cell growth and lactate production. Amino acids are important molecules that serve as the fundamental components or building blocks of proteins (Moini, 2019). They play a significant role in facilitating microbial cell growth (Idrees et al., 2020). Moreover, amino acids including glycine, serine, and tyrosine in yeast extract play a central role in promoting cell growth (Li et al., 2011). The protein content of Arthrospira is high and contains all the essential amino acids with good digestibility (Bernaerts et al., 2019; Niccolai et al., 2019). Khandual et al. (2021) found large amounts of each amino acid in the total biomass after C-phycocyanin extraction. Therefore, WCR addition in B. coagulans culture media stimulated microbial cell growth.
Viable cell growth increased when WCR feedstock loading increased, while lactate production increased with fermentation time. WCR loading in the culture medium stimulated cell growth and lactate production, as confirmed by the control experiments. The results showed glucose consumption to produce lactate. Moreover, during B. coagulans ATCC 7050 fermentation supplemented with WCR, various LAB fermentation metabolites such as lactate, acetate, ethanol, aroma compounds, and enzymes were produced (Raj et al., 2022). B. coagulans produces homofermentative lactic acid under anaerobic conditions (Okada et al., 1979). However, this homofermentation reaction also produces by-products including acetate kinase and alcohol dehydrogenase in the acetate and ethanol conversion process. Our results showed that both lactate and by-products were produced under unsuitable fermentation conditions. Su and Xu (2014) also determined ethanol and acetate as the primary fermentation products under aerobic conditions. The acetate kinase-encoding gene present in the B. coagulans genome prefers an energy-generating pathway, with acetic acid synthesis to metabolize accumulated NADH following the loss of the lactate dehydrogenase function (Sun et al., 2016). These reasons support our results. Moreover, lactate production by glucose fermentation of B. coagulans was inhibited by glucose concentrations higher than 100 g L−1 (Michelson et al., 2006), leading to decreased growth rate and underfermentation (Glaser and Venus, 2018). Lactic acid-producing bacteria are metabolized by the phosphogluconase pathway that produces various co-products such as acetate, CO2, and ethanol, with low lactate yield as the end product of fermentation (Bintsis, 2018; Abedi and Hashemi, 2020). Pyruvate molecules can be reduced to lactate, with acetate to ethanol and CO2 (Mendes Ferreira and Mendes-Faia, 2020). These were used as a carbon source for lactate and bioproducts in this study. Therefore, the relationship between the quantities of acetate and ethanol that decreased the theoretical yield to 0.50 g g−1 depends on the capacity of bacteria to reoxidize NADH created in the initial procedure, as well as its energy demands (Yu et al., 2020).
Kinetic parameters of B. coagulans ATCC 7050 cultivation using WCR substituted by yeast extract were calculated in terms of fermentation time for maximal lactate production at 12 h. WCR addition achieved higher lactate production, with increased nutrients in the culture media associated with cell growth. Maximal lactate yield was achieved at WCR addition of 20 g L−1, indicating sufficient nitrogen and other nutrients for direct utilization of cell growth. When WCR loading increased, the pigments of C-PC and chlorophyll also increased, promoting the nutraceutical properties of Arthrospira fermentation (De Marco Castro et al., 2019). However, higher pigments and total phenolic compounds may inhibit bacteria cultivation (Takó et al., 2020) as well as have a positive effect on microorganisms (Adebo and Gabriela Medina-Meza, 2020). Arthrospira biomass can also serve as a nutrient source during the fermentation process (Luiza Astolfi et al., 2020). Moreover, B. coagulans is catalase-positive and produces energy from glucose via glycolysis, the citric acid cycle (TCA), and the electron transport chain, resulting in high growth rates. Lactate was produced with high productivity at higher cell growth (Thitiprasert et al., 2017). Therefore, WCR was utilized as a renewable resource to replace expensive yeast extract for lactate production by B. coagulans.
Supplementary Table S4 compares previous fermentation studies of bioproducts produced from various substances. Lactate production from lyophilized biomass cyanobacterium Arthrospira platensis F&M-C256 by Lactobacillus plantarum ATCC 8014 reached 3.7 g L−1 lactate concentration (Niccolai et al., 2019). A. platensis F&M-C256 was also successfully evaluated in a vegetal soybean drink and in water by probiotic L. plantarum ATCC 8014 at 1.7 g L−1 (Niccolai et al., 2020). Our results showed the highest lactate production due to different bacterial strains and substrate consumed. Using WCR as the nitrogen source reduced the cost of the culture medium with direct utilization without pretreatment. Werlang et al. (2020) studied A. platensis as a raw material for d-lactic acid production via saccharification and fermentation enzymatic processes using the lactogenic Escherichia coli strain JU15, giving d-lactate at 25.5 g L−1, as an alternative option for renewable resources as the carbon source for bacterial cultivation. B. coagulans can produce l-lactate by fermentation generally in a synthetic medium. l-Lactate production by B. coagulans has been studied using different fermentation medium compositions and processes (Fan et al., 2016; Tolieng et al., 2018). Here, alternative nitrogen sources to replace yeast extract in the fermentation medium realized higher l-lactate concentrations. Other substrates as the fermentation medium and processes for lactate production were applied to cassava starch hydrolysates and sludge hydrolysates (Ma et al., 2014; Ooi and Wu, 2015). The complete GY culture medium obtained high lactate production (Tolieng et al., 2018). Our results gave lower lactate but still reduced medium cost and used WCR as a renewable resource for nutraceutical ingredients for health. Using Arthrospira biomass as the substrate for lactate production by the probiotic bacterium significantly increased essential amino acid contents and the bioactive profile through probiotic growth and pre- and probiotic bio-active substance production (De Marco Castro et al., 2019; Yu et al., 2020). Therefore, waste Arthrospira cell residue from C-PC extraction can act as an alternative substrate for probiotic B. coagulans cultivation and produce lactate as renewable bioproducts.
Waste cell residue of Arthrospira IFRPD 1182 biomass from C-PC extraction showed feasibility as an alternative substrate for B. coagulans ATCC 7050 cultivation. WCR acted as a nitrogen source that replaced yeast extract in culture media, resulting in reduced production cost. B. coagulans ATCC 7050 cultivation gave maximal lactate yield at 20 g L−1 WCR. However, the fermentation process should be further studied to give high lactate production in a shorter time, with Arthrospira saccharification and fermentation also investigated to enhance production efficiency. Our results demonstrated the feasibility of utilizing waste resources from the cyanobacterium Arthrospira to produce valuable products. WCR was proven as a significant alternative resource for Bacillus coagulans growth, bioproduct production, and also reducing waste in food systems.
The original contributions presented in the study are included in the article/Supplementary Material; further inquiries can be directed to the corresponding author.
WP-u: conceptualization, formal analysis, writing—review and editing, and visualization. ST: conceptualization and validation. SP: validation.
This project was partially funded by the National Research Council of Thailand, under No. 101/2020.
The authors declare that the research was conducted in the absence of any commercial or financial relationships that could be construed as a potential conflict of interest.
All claims expressed in this article are solely those of the authors and do not necessarily represent those of their affiliated organizations, or those of the publisher, the editors, and the reviewers. Any product that may be evaluated in this article, or claim that may be made by its manufacturer, is not guaranteed or endorsed by the publisher.
We acknowledge receipt of financial and instrument support from the National Research Council of Thailand, Kasetsart University Research and Development Institute (KURDI), and the Institute of Food Research and Development, Kasetsart University, Thailand.
The Supplementary Material for this article can be found online at: https://www.frontiersin.org/articles/10.3389/fenrg.2022.878597/full#supplementary-material
Abbasiliasi, S., Tan, J. S., Tengku Ibrahim, T. A., Bashokouh, F., Ramakrishnan, N. R., Mustafa, S., et al. (2017). Fermentation Factors Influencing the Production of Bacteriocins by Lactic Acid Bacteria: a Review. RSC Adv. 7, 29395–29420. doi:10.1039/c6ra24579j
Abedi, E., and Hashemi, S. M. B. (2020). Lactic Acid Production - Producing Microorganisms and Substrates Sources-State of Art. Heliyon 6, e04974. doi:10.1016/j.heliyon.2020.e04974
Adebo, O. A., and Gabriela Medina-Meza, I. (2020). Impact of Fermentation on the Phenolic Compounds and Antioxidant Activity of Whole Cereal Grains: A Mini Review. Molecules 25, 927. doi:10.3390/molecules25040927
Bernaerts, T. M. M., Gheysen, L., Foubert, I., Hendrickx, M. E., and Van Loey, A. M. (2019). The Potential of Microalgae and Their Biopolymers as Structuring Ingredients in Food: A Review. Biotechnol. Adv. 37, 107419. doi:10.1016/j.biotechadv.2019.107419
Bintsis, T. (2018). Lactic Acid Bacteria as Starter Cultures: An Update in Their Metabolism and Genetics. AIMS Microbiol. 4, 665–684. doi:10.3934/microbiol.2018.4.665
Cao, J., Yu, Z., Liu, W., Zhao, J., Zhang, H., Zhai, Q., et al. (2020). Probiotic Characteristics of Bacillus Coagulans and Associated Implications for Human Health and Diseases. J. Funct. Foods 64, 103643. doi:10.1016/j.jff.2019.103643
Chen, P.-T., Hong, Z.-S., Cheng, C.-L., Ng, I.-S., Lo, Y.-C., Nagarajan, D., et al. (2020). Exploring Fermentation Strategies for Enhanced Lactic Acid Production with Polyvinyl Alcohol-Immobilized Lactobacillus Plantarum 23 Using Microalgae as Feedstock. Bioresour. Technol. 308, 123266. doi:10.1016/j.biortech.2020.123266
Chng, L. M., Chan, D. J. C., and Lee, K. T. (2016). Sustainable Production of Bioethanol Using Lipid-Extracted Biomass from Scenedesmus Dimorphus. J. Clean. Prod. 130, 68–73. doi:10.1016/j.jclepro.2016.02.016
Christaki, E., Florou-Paneri, P., and Bonos, E. (2011). Microalgae: a Novel Ingredient in Nutrition. Int. J. Food Sci. Nutr. 62, 794–799. doi:10.3109/09637486.2011.582460
Cubas‐Cano, E., González‐Fernández, C., Ballesteros, M., and Tomás‐Pejó, E. (2018). Biotechnological Advances in Lactic Acid Production by Lactic Acid Bacteria: Lignocellulose as Novel Substrate. Biofuels, Bioprod. Biorefining 12, 290–303. doi:10.1002/bbb.1852
De La Jara, A., Ruano-Rodriguez, C., Polifrone, M., Assunçao, P., Brito-Casillas, Y., Wägner, A. M., et al. (2018). Impact of Dietary Arthrospira (Spirulina) Biomass Consumption on Human Health: Main Health Targets and Systematic Review. J. Appl. Phycol. 30, 2403–2423. doi:10.1007/s10811-018-1468-4
De Marco Castro, E., Shannon, E., and Abu-Ghannam, N. (2019). Effect of Fermentation on Enhancing the Nutraceutical Properties of Arthrospira Platensis (Spirulina). Fermentation 5, 28. doi:10.3390/fermentation5010028
De Souza, D. S., Valadão, R. C., De Souza, E. R. P., Barbosa, M. I. M. J., and de Mendonça, H. V. (2022). Enhanced Arthrospira Platensis Biomass Production Combined with Anaerobic Cattle Wastewater Bioremediation. Bioenerg. Res. 15, 412–425. doi:10.1007/s12155-021-10258-4
Dębowski, M., Kisielewska, M., Kazimierowicz, J., Rudnicka, A., Dudek, M., Romanowska-Duda, Z., et al. (2020). The Effects of Microalgae Biomass Co-substrate on Biogas Production from the Common Agricultural Biogas Plants Feedstock. Energies 13, 2186. doi:10.3390/en13092186
Endres, J. R., Clewell, A., Jade, K. A., Farber, T., Hauswirth, J., and Schauss, A. G. (2009). Safety Assessment of a Proprietary Preparation of a Novel Probiotic, Bacillus Coagulans, as a Food Ingredient. Food Chem. Toxicol. 47, 1231–1238. doi:10.1016/j.fct.2009.02.018
Eş, I., Mousavi Khaneghah, A., Barba, F. J., Saraiva, J. A., Sant'ana, A. S., and Hashemi, S. M. B. (2018). Recent Advancements in Lactic Acid Production - a Review. Food Res. Int. 107, 763–770. doi:10.1016/j.foodres.2018.01.001
Fan, R., Ebrahimi, M., Quitmann, H., Aden, M., and Czermak, P. (2016). An Innovative Optical Sensor for the Online Monitoring and Control of Biomass Concentration in a Membrane Bioreactor System for Lactic Acid Production. Sensors 16, 411. doi:10.3390/s16030411
Ferreira, A., Guerra, I., Costa, M., Silva, J., and Gouveia, L. (2021). “Future Perspectives of Microalgae in the Food Industry,” in Cultured Microalgae for the Food Industry. Editors T. Lafarga, and G. Acién (Academic Press), 387–433. doi:10.1016/b978-0-12-821080-2.00008-3
Furmaniak, M. A., Misztak, A. E., Franczuk, M. D., Wilmotte, A., Waleron, M., and Waleron, K. F. (2017). Edible Cyanobacterial Genus Arthrospira: Actual State of the Art in Cultivation Methods, Genetics, and Application in Medicine. Front. Microbiol. 8, 2541. doi:10.3389/fmicb.2017.02541
Glaser, R., and Venus, J. (2018). Co-fermentation of the Main Sugar Types from a Beechwood Organosolv Hydrolysate by Several Strains of Bacillus Coagulans Results in Effective Lactic Acid Production. Biotechnol. Rep. 18, e00245. doi:10.1016/j.btre.2018.e00245
González-González, L. M., Correa, D. F., Ryan, S., Jensen, P. D., Pratt, S., and Schenk, P. M. (2018). Integrated Biodiesel and Biogas Production from Microalgae: Towards a Sustainable Closed Loop through Nutrient Recycling. Renew. Sustain. Energy Rev. 82, 1137–1148. doi:10.1016/j.rser.2017.09.091
Gupta, S., and Abu-Ghannam, N. (2011). Bioactive Potential and Possible Health Effects of Edible Brown Seaweeds. Trends Food Sci. Technol. 22, 315–326. doi:10.1016/j.tifs.2011.03.011
Hames, B., Scarlata, C., and Sluiter, A. (2008). Determination of Protein Content in Biomass. Technical Report NREL/TP-510-42625. Golden, CO, USA: National Renewable Energy Laboratory.
Hazards, E. P. O. B. (2013). Scientific Opinion on the Maintenance of the List of QPS Biological Agents Intentionally Added to Food and Feed (2013 Update). EFSA J. 11, 3449. doi:10.2903/j.efsa.2013.3449
Idrees, M., Mohammad, A. R., Karodia, N., and Rahman, A. (2020). Multimodal Role of Amino Acids in Microbial Control and Drug Development. Antibiotics 9, 330. doi:10.3390/antibiotics9060330
Jaiaue, P., Srimongkol, P., Thitiprasert, S., Tanasupawat, S., Cheirsilp, B., Assabumrungrat, S., et al. (2021). A Modified Approach for High-Quality RNA Extraction of Spore-Forming Bacillus Subtilis at Varied Physiological Stages. Mol. Biol. Rep. 48, 6757–6768. doi:10.1007/s11033-021-06673-7
Jha, D., Jain, V., Sharma, B., Kant, A., and Garlapati, V. K. (2017). Microalgae-based Pharmaceuticals and Nutraceuticals: An Emerging Field with Immense Market Potential. ChemBioEng Rev. 4, 257–272. doi:10.1002/cben.201600023
Khandual, S., Sanchez, E. O. L., Andrews, H. E., and de la Rosa, J. D. P. (2021). Phycocyanin Content and Nutritional Profile of Arthrospira Platensis from Mexico: Efficient Extraction Process and Stability Evaluation of Phycocyanin. BMC Chemistry. 15, 24.
Khavari, F., Saidijam, M., Taheri, M., and Nouri, F. (2021). Microalgae: Therapeutic Potentials and Applications. Mol. Biol. Rep. 48, 4757–4765. doi:10.1007/s11033-021-06422-w
Li, M., Liao, X., Zhang, D., Du, G., and Chen, J. (2011). Yeast Extract Promotes Cell Growth and Induces Production of Polyvinyl Alcohol-Degrading Enzymes. Enzyme Res. 2011, 179819. doi:10.4061/2011/179819
Liu, Q., Yao, C., Sun, Y., Chen, W., Tan, H., Cao, X., et al. (2019). Production and Structural Characterization of a New Type of Polysaccharide from Nitrogen-Limited Arthrospira Platensis Cultivated in Outdoor Industrial-Scale Open Raceway Ponds. Biotechnol. Biofuels 12, 131. doi:10.1186/s13068-019-1470-3
Luiza Astolfi, A., Rempel, A., Cavanhi, V. A. F., Alves, M., Deamici, K. M., Colla, L. M., et al. (2020). Simultaneous Saccharification and Fermentation of Spirulina Sp. And Corn Starch for the Production of Bioethanol and Obtaining Biopeptides with High Antioxidant Activity. Bioresour. Technol. 301, 122698. doi:10.1016/j.biortech.2019.122698
Ma, K., Maeda, T., You, H., and Shirai, Y. (2014). Open Fermentative Production of L-Lactic Acid with High Optical Purity by Thermophilic Bacillus Coagulans Using Excess Sludge as Nutrient. Bioresour. Technol. 151, 28–35. doi:10.1016/j.biortech.2013.10.022
Majeed, M., Majeed, S., Nagabhushanam, K., Natarajan, S., Sivakumar, A., and Ali, F. (2016). Evaluation of the Stability of Bacillus Coagulans MTCC 5856 during Processing and Storage of Functional Foods. Int. J. Food Sci. Technol. 51, 894–901. doi:10.1111/ijfs.13044
Martelli, F., Cirlini, M., Lazzi, C., Neviani, E., and Bernini, V. (2021). Solid-State Fermentation of Arthrospira Platensis to Implement New Food Products: Evaluation of Stabilization Treatments and Bacterial Growth on the Volatile Fraction. Foods 10, 67. doi:10.3390/foods10010067
Mehariya, S., Goswami, R. K., Karthikeysan, O. P., and Verma, P. (2021). Microalgae for High-Value Products: A Way towards Green Nutraceutical and Pharmaceutical Compounds. Chemosphere 280, 130553. doi:10.1016/j.chemosphere.2021.130553
Mendes Ferreira, A., and Mendes-Faia, A. (2020). The Role of Yeasts and Lactic Acid Bacteria on the Metabolism of Organic Acids during Winemaking. Foods 9, 1231. doi:10.3390/foods9091231
Meng, Y., Xue, Y., Yu, B., Gao, C., and Ma, Y. (2012). Efficient Production of L-Lactic Acid with High Optical Purity by Alkaliphilic Bacillus Sp. WL-S20. Bioresour. Technol. 116, 334–339. doi:10.1016/j.biortech.2012.03.103
Michelson, T., Kask, K., Jõgi, E., Talpsep, E., Suitso, I., and Nurk, A. (2006). l(+)-Lactic Acid Producer Bacillus Coagulans SIM-7 DSM 14043 and its Comparison with Lactobacillus Delbrueckii Ssp. Lactis DSM 20073. lactis DSMEnzyme Microb. Technol. 39, 861–867. doi:10.1016/j.enzmictec.2006.01.015
Mokoena, M. P. (2017). Lactic Acid Bacteria and Their Bacteriocins: Classification, Biosynthesis and Applications against Uropathogens: A Mini-Review. Molecules 22, 1255. doi:10.3390/molecules22081255
Mostafa, S. S. M., and El-Gendy, N. S. (2017). Evaluation of Fuel Properties for Microalgae Spirulina Platensis Bio-Diesel and its Blends with Egyptian Petro-Diesel. Arabian J. Chem. 10, S2040–S2050. doi:10.1016/j.arabjc.2013.07.034
Nduko, J. M., and Taguchi, S. (2020). Microbial Production of Biodegradable Lactate-Based Polymers and Oligomeric Building Blocks from Renewable and Waste Resources. Front. Bioeng. Biotechnol. 8, 618077. doi:10.3389/fbioe.2020.618077
Niccolai, A., Bažec, K., Rodolfi, L., Biondi, N., Zlatić, E., Jamnik, P., et al. (2020). Lactic Acid Fermentation of Arthrospira Platensis (Spirulina) in a Vegetal Soybean Drink for Developing New Functional Lactose-free Beverages. Front. Microbiol. 11, 560684. doi:10.3389/fmicb.2020.560684
Niccolai, A., Shannon, E., Abu-Ghannam, N., Biondi, N., Rodolfi, L., and Tredici, M. R. (2019). Lactic Acid Fermentation of Arthrospira Platensis (Spirulina) Biomass for Probiotic-Based Products. J. Appl. Phycol. 31, 1077–1083. doi:10.1007/s10811-018-1602-3
Oh, H., Wee, Y. H., Yun, J. S., and Ryu, H. W. (2003). Lactic Acid Production Through Cell-Recycle Repeated-Batch Bioreactor. Appl Biochem Biotechnol. 105–108, 603–613.
Okada, S., Suzuki, Y., and Kozaki, M. (1979). A New Heterofermentative Lactobacillus Species with Meso-Diaminopimelic Acid in Peptidoglycan, Lactobacillus Vaccinostercus Kozaki and Okada Sp. Nov. J. Gen. Appl. Microbiol. 25, 215–221. doi:10.2323/jgam.25.215
Olszewska-Widdrat, A., Alexandri, M., López-Gómez, J. P., Schneider, R., and Venus, J. (2020). Batch and Continuous Lactic Acid Fermentation Based on A Multi-Substrate Approach. Microorganisms 8, 1084. doi:10.3390/microorganisms8071084
Ooi, K. Y., and Wu, J. C. (2015). Use of Dry Yeast Cells as a Cheap Nitrogen Source for Lactic Acid Production by Thermophilic Bacillus Coagulans WCP10-4. Front. Chem. Sci. Eng. 9, 381–385. doi:10.1007/s11705-015-1534-2
Pan-Utai, W., Boonpok, S., and Pornpukdeewattana, S. (2021). Combination of Mechanical and Chemical Extraction of Astaxanthin from Haematococcus pluvialis and its Properties of Microencapsulation. Biocatal. Agric. Biotechnol. 33, 101979. doi:10.1016/j.bcab.2021.101979
Pan-Utai, W., and Iamtham, S. (2019). Extraction, Purification and Antioxidant Activity of Phycobiliprotein from Arthrospira Platensis. Process Biochem. 82, 189–198. doi:10.1016/j.procbio.2019.04.014
Pan-Utai, W., Kahapana, W., and Iamtham, S. (2018). Extraction of C-Phycocyanin from Arthrospira (Spirulina) and its Thermal Stability with Citric Acid. J. Appl. Phycol. 30, 231–242. doi:10.1007/s10811-017-1155-x
Pan-Utai, W., Poopat, N., Parakulsuksatid, P., and Pan-Utai, W. (2020). Photoautotrophic Cultivation of Arthrospira Maxima for Protein Accumulation under Minimum Nutrient Availability. Appl. Food Biotechnol. 7, 225–233. doi:10.22037/afb.v7i4.30353
Pan-Utai, W., Srinophakun, P., and Inrung, W. (2019). Nutrients Formulation to Maximize Ankistrodesmus Sp. Microalgal Cell Biomass and Lipid Productivities. J. Biol. Res. (Italy) 92, 95–100. doi:10.4081/jbr.2019.8547
Poudel, P., Tashiro, Y., and Sakai, K. (2016). New Application of Bacillus Strains for Optically Pure L-Lactic Acid Production: General Overview and Future Prospects. Biosci. Biotechnol. Biochem. 80, 642–654. doi:10.1080/09168451.2015.1095069
Prabha, S., Vijay, A. K., Paul, R. R., and George, B. (2022). Cyanobacterial Biorefinery: Towards Economic Feasibility through the Maximum Valorization of Biomass. Sci. Total Environ. 814, 152795. doi:10.1016/j.scitotenv.2021.152795
Prasirtsak, B., Thitiprasert, S., Tolieng, V., Assabumrungrat, S., Tanasupawat, S., and Thongchul, N. (2019). D-lactic Acid Fermentation Performance and the Enzyme Activity of a Novel Bacterium Terrilactibacillus Laevilacticus SK5-6. Ann. Microbiol. 69, 1537–1546. doi:10.1007/s13213-019-01538-8
Raj, T., Chandrasekhar, K., Kumar, A. N., and Kim, S.-H. (2022). Recent Biotechnological Trends in Lactic Acid Bacterial Fermentation for Food Processing Industries. Syst Microbiol Biomanuf 2, 14–40. doi:10.1007/s43393-021-00044-w
Raja, R., Hemaiswarya, S., Ganesan, V., and Carvalho, I. S. (2016). Recent Developments in Therapeutic Applications of Cyanobacteria. Crit. Rev. Microbiol. 42, 394–405. doi:10.3109/1040841X.2014.957640
Rempel, A., De Souza Sossella, F., Margarites, A. C., Astolfi, A. L., Steinmetz, R. L. R., Kunz, A., et al. (2019). Bioethanol from Spirulina Platensis Biomass and the Use of Residuals to Produce Biomethane: An Energy Efficient Approach. Bioresour. Technol. 288, 121588. doi:10.1016/j.biortech.2019.121588
Ścieszka, S., and Klewicka, E. (2020). Influence of the Microalga Chlorella Vulgaris on the Growth and Metabolic Activity of Lactobacillus Spp. Bacteria. Foods (Basel, Switz. 9, 959. doi:10.3390/foods9070959
Sluiter, A., Hames, B., Hyman, D., Payne, C., Ruiz, R., Scarlata, C., et al. (2008a). Determination of Total Solids in Biomass and Total Dissolved Solids in Liquid Process Samples. Technical Report NREL/TP-510-42621. Golden, CO, USA: Laboratory Analytical Procedure (LAP)National Renewable Energy Laboratory.
Sluiter, A., Hames, B., Ruiz, R., Scarlata, C., Sluiter, J., and Templeton, D. (2008b). Determination of Ash in Biomass. Laboratory Analytical Procedure (LAP). Technical Report NREL/TP-510-42622. Golden, CO, USA: National Renewable Energy Laboratory.
Su, F., and Xu, P. (2014). Genomic Analysis of Thermophilic Bacillus Coagulans Strains: Efficient Producers for Platform Bio-Chemicals. Sci. Rep. 4, 3926. doi:10.1038/srep03926
Sun, L., Zhang, C., Lyu, P., Wang, Y., Wang, L., and Yu, B. (2016). Contributory Roles of Two L-Lactate Dehydrogenases for L-Lactic Acid Production in Thermotolerant Bacillus Coagulans. Sci. Rep. 6, 37916. doi:10.1038/srep37916
Takó, M., Kerekes, E. B., Zambrano, C., Kotogán, A., Papp, T., Krisch, J., et al. (2020). Plant Phenolics and Phenolic-Enriched Extracts as Antimicrobial Agents against Food-Contaminating Microorganisms. Antioxidants 9, 165. doi:10.3390/antiox9020165
Thitiprasert, S., Kodama, K., Tanasupawat, S., Prasitchoke, P., Rampai, T., Prasirtsak, B., et al. (2017). A Homofermentative Bacillus Sp. BC-001 and its Performance as a Potential L-Lactate Industrial Strain. Bioprocess Biosyst. Eng. 40, 1787–1799. doi:10.1007/s00449-017-1833-8
Thompson, H. O., Önning, G., Holmgren, K., Strandler, H. S., and Hultberg, M. (2020). Fermentation of Cauliflower and White Beans with Lactobacillus Plantarum - Impact on Levels of Riboflavin, Folate, Vitamin B12, and Amino Acid Composition. Plant Foods Hum. Nutr. 75, 236–242. doi:10.1007/s11130-020-00806-2
Tolieng, V., Nuhwa, R., Thongchul, N., and Tanasupawat, S. (2018). Characterization of Lactic Acid-Producing Bacillus Coagulans Strains with Their Antibacterial Activity and L-Lactic Acid Production. Thai J. Pharm. Sci. 42, 138–145.
Uchida, M., and Miyoshi, T. (2013). Algal Fermentation^|^mdash;The Seed for a New Fermentation Industry of Foods and Related Products. Jarq 47, 53–63. doi:10.6090/jarq.47.53
Werlang, E. B., Ibarra, E. S., Neves, F. d. F., Julich, J., Martinez, A., and Schneider, R. d. C. d. S. (2020). D-Lactate Production from Spirulina (Arthrospira Platensis) Biomass Using Lactogenic Escherichia coli. Bioresour. Technol. Rep. 12, 100598. doi:10.1016/j.biteb.2020.100598
Yarkent, Ç., Gürlek, C., and Oncel, S. S. (2020). Potential of Microalgal Compounds in Trending Natural Cosmetics: A Review. Sustain. Chem. Pharm. 17, 100304. doi:10.1016/j.scp.2020.100304
Yu, J., Ma, D., Qu, S., Liu, Y., Xia, H., Bian, F., et al. (2020). Effects of Different Probiotic Combinations on the Components and Bioactivity of Spirulina. J. Basic Microbiol. 60, 543–557. doi:10.1002/jobm.201900699
Keywords: Arthrospira, waste cell residue, Bacillus coagulans, bioproduct, zero-waste
Citation: Pan-utai W, Thitiprasert S and Pornpukdeewattana S (2022) Arthrospira Cell Residues for Lactic Acid Fermentation as Bioproducts From Waste Utilization. Front. Energy Res. 10:878597. doi: 10.3389/fenrg.2022.878597
Received: 18 February 2022; Accepted: 02 May 2022;
Published: 13 June 2022.
Edited by:
Qingfang He, University of Arkansas at Little Rock, United StatesReviewed by:
Joachim Venus, Leibniz Institute for Agricultural Engineering and Bioeconomy (ATB), GermanyCopyright © 2022 Pan-utai, Thitiprasert and Pornpukdeewattana. This is an open-access article distributed under the terms of the Creative Commons Attribution License (CC BY). The use, distribution or reproduction in other forums is permitted, provided the original author(s) and the copyright owner(s) are credited and that the original publication in this journal is cited, in accordance with accepted academic practice. No use, distribution or reproduction is permitted which does not comply with these terms.
*Correspondence: Wanida Pan-utai, aWZyd2RwQGt1LmFjLnRo
Disclaimer: All claims expressed in this article are solely those of the authors and do not necessarily represent those of their affiliated organizations, or those of the publisher, the editors and the reviewers. Any product that may be evaluated in this article or claim that may be made by its manufacturer is not guaranteed or endorsed by the publisher.
Research integrity at Frontiers
Learn more about the work of our research integrity team to safeguard the quality of each article we publish.