- Key Laboratory of Microelectronics and Energy of Henan Province, College of Physics and Electronic Engineering, Xinyang Normal University, Xinyang, China
Layered metal sulfides are regarded as potential candidates for supercapacitive electrode materials due to the unique spatial dimensions for charge transport. Herein, self-supported SnS2 nanosheet arrays on nickel (Ni) foam were successfully fabricated via a facile solvothermal approach. Interestingly, the continuous and high-density SnS2 nanosheet arrays are interconnected to form porous Ni@SnS2 electrode materials, which suppress the self-aggregation of SnS2 and provide outstanding conductivity with 3D-networked Ni framework. The Ni@SnS2 electrode demonstrates a high areal specific capacitance of 1965.56 mF cm−2 at a current density of 1 mA cm−2 and satisfactory cycling stability (78.3% capacity retention after 10,000 cycles). This self-supported porous structure provides a promising way to build advanced electrode material for supercapacitors.
Introduction
Supercapacitors, due to their fast recharge rate, high power densities, and outstanding durability, have attracted considerable attention from scientists to industrialists (Javed et al., 2019; Zhang et al., 2019; Javed et al., 2020a; Fu et al., 2021). The energy storage mechanisms of supercapacitors can be divided into pseudocapacitors and electric double-layer capacitors. Among them, pseudocapacitors have attracted extensive interest because their Faradaic redox reaction can achieve a higher energy density than electric double-layer capacitors. In the past few years, transition metal oxides/hydroxides/sulfides including V2O5 (Javed et al., 2020b), MnO2 (Bai et al., 2018), Co.(OH)2 (Wang et al., 2016), and Ni3S2 (Xie et al., 2021), were mainly studied as pseudocapacitor electrode materials.
SnS2, has been used as a promising electrode material for batteries, photocatalysts and supercapacitors. For example, Cao et al. designed and synthesized a N,S-doped carbon/SnS2 nanosheets hybrid as an anode material for potassium ion batteries (Cao et al., 2021), Sun et al. prepared a graphene/SnS2 hybrid material to enhance absorption in the visible region (Sun et al., 2019), and, Parveen et al. reported flower-like SnS2 with a high specific capacitance ( ∼ 431.82 F g−1 at 1 A g−1) (Parveen et al., 2018). However, the agglomeration of 2D SnS2 nanosheets seriously hinders the active sites and redox reaction as a supercapacitive electrode material over extended cycle.
Herein, we prepared self-supported SnS2 nanosheet arrays on nickel foam (Ni@SnS2) by a simple solvothermal method. In this architecture, the porous SnS2 nanosheet arrays provided the fast charge transfer passages, and the 3D-networked Ni foam improved the high conductivity of the material, endowing the outstanding electrochemical performance overall. The as-prepared Ni@SnS2 electrode exhibited a high capacity of 1965.56 mF cm−2 at 1 mA cm−2 and a long cycling life (78.3% retention after 10,000 cycles at 20 mA cm−2).
Experimental
Synthesis of the Ni@SnS2 Nanosheet Arrays
The nickel (Ni) foam was cleaned with acetone, anhydrous ethanol, and deionized water. First, 0.701 g of SnCl4.5H2O and 0.225 g of thioacetamide (TAA) were added to 60 ml of glycol and stirred for 20 min to form a uniform solution. Then, the prepared solution and a piece of cleaned Ni foam were transferred to a 100 ml lined Teflon stainless steel autoclave and maintained at 130°C for 8 h. Afterward, the sample was taken out, cleaned with anhydrous ethanol and deionized water, and then placed in the oven at 60°C for 12 h to obtain Ni@SnS2 samples.
Characterization
The morphology was characterized by cold field emission scanning electron microscopy (SEM, Hitachi 4,800) and transmission electron microscopy (TEM, JEM 2100F). The phase structure of the sample was analyzed by X-ray Powder Diffractometer (XRD, Rigaku D/Max-2400 diffractometer) and micro-Raman spectroscopy (Jobin-Yvon LabRAM HR800 UV, YAG 532 nm). The surface composition and chemical state of the sample are analyzed by X-ray photoelectron spectroscopy (XPS, K-ALPHA 0.5 eV). The specific surface area was calculated by the Brunauer-Emmett-Teller (BET) method. The pore size distribution (PSD) was derived from the adsorption branches of isotherms by the Barrett-Joyner-Halenda (BJH) method.
Electrochemical Measurements
The electrochemical performance was measured using an electrochemical workstation (CHI660e). Ni@SnS2 was directly used to test the electrochemical performance of three electrodes. Saturated calomel was used as the reference electrode, a platinum electrode was used as the counter electrode, Ni@SnS2 (1 × 1 cm2) was used as the working electrode, and 2 M KOH was used as the electrolyte. Ni@SnS2 was tested by cyclic voltammetry (CV, sweep speed 5–100 mV s−1) and constant current charge-discharge curves (GCD, current density 1–100 mA cm−2). Electrochemical impedance spectroscopy (EIS) was performed at AC frequency of 0.01 Hz–100 kHz and an amplitude of 5 mV.
Results and Discussion
Composition and Morphology Analysis
Figure 1Ashows the XRD patterns of the Ni@SnS2 nanosheet arrays. As is seen, the strong diffraction peaks centered at 44.5°, 51.7°, and 76.3°, correspond to the (111), (200), and (220) crystalline facets of Ni foam (PDF#04-0850), respectively. Due to the weak peak intensity, the characteristic reflection at 28.1° can be assigned to the (100) plane of SnS2 (PDF#23-0677) (Li et al., 2018). In compared to the SnS2 samples left from the Ni foam (Supplementary Figure S1), the pure SnS2 samples (Supplementary Figure S2) exhibit the same diffraction peaks in the XRD patterns, confirming the existence of SnS2 used as real active materials. Raman spectrum (Figure 1B) proves the existence of SnS2 on the surface of Ni foam with a high band at ∼ 305 cm−1, which is assigned to the A1g mode of SnS2 (Jiang et al., 2013; Qu et al., 2014). Furthermore, Supplementary Figure S3 shows the XPS full survey spectrum, confirming the existence of Sn, Ni, and S elements. In the high-resolution narrow spectrum of S 2p (Figure 1C), the two peaks located at 161.9 and 163.1 eV respectively correspond to S 2p3/2 and S 2p1/2, accompanying the satellite peak. The Sn 3d spectrum (Figure 1D) contains two-orbit peaks at 487.1 and 495.4 eV, corresponding to Sn 3d3/2 and Sn 2d5/2. The BET surface area and pore size distribution of Ni@SnS2 were conducted by nitrogen isothermal adsorption/desorption measurement. The typical type IV isotherm curves (Supplementary Figure S4A) exhibit the evident hysteresis loop and the according surface area is about 1.14 m2⋅g−1. The pore diameter (Supplementary Figure S4B) is located at 2.17 and 10.97 nm, indicating the existence of mesoporous structure.
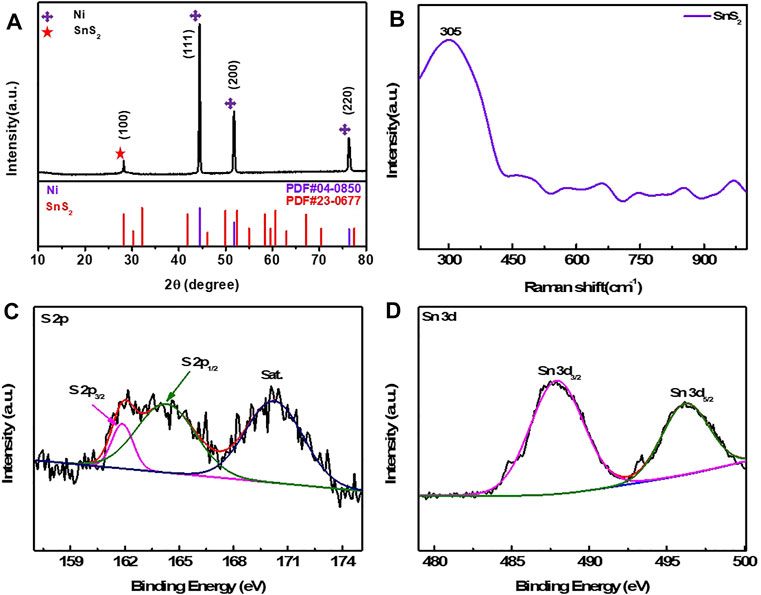
FIGURE 1. (A) XRD pattern of the Ni@SnS2 nanosheet arrays; (B) Raman spectrum; XPS survey spectra of (C) S 2p and (D) Sn 3d.
Figures 2A–D display the morphological features of the Ni@SnS2 nanosheet arrays by SEM. In the low-magnification SEM image (Figure 2A), a homogeneous and complete SnS2 nanosheet arrays coated the skeleton of the Ni foam. Figures 2B–D show the high-magnification SEM images, revealing that the vertically aligned SnS2 nanosheets are highly packed on the Ni foam and are interconnected to form numerous porous structures. A single Ni@SnS2 nanosheet array was further observed by TEM. Figures 2E,I show images of the intersected SnS2 nanosheet structures, which are consistent with the SEM analysis. From the high-resolution TEM (HRTEM) image, Figures 2F,G shows a lattice fringe of 0.21 nm, corresponding to the (102) plane of SnS2. The selected area electron diffraction (SAED) pattern is shown in Figure 2H, implying that the polycrystalline rings can be indexed to the (101), (100) and (102) planes of SnS2 (Liu et al., 2021). Furthermore, elemental mappings (Figures 2I–M) were measured to demonstrate the porous structure and homogeneous distribution of Sn, and S supported on Ni foam.
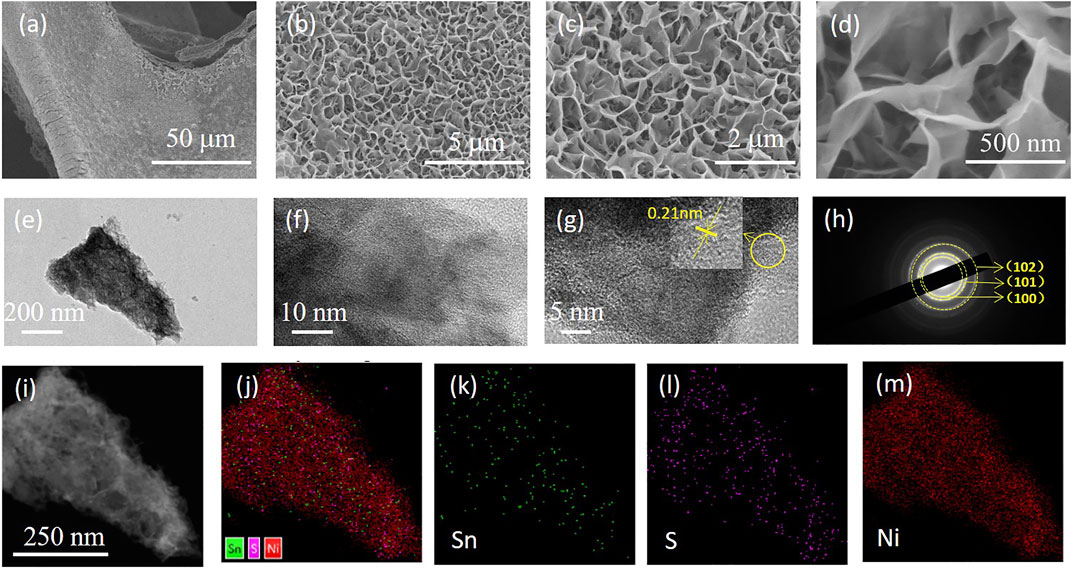
FIGURE 2. (A–D) SEM images at different magnifications of the Ni@SnS2 nanosheet arrays; (E–G) TEM images at different magnifications; (H) SAED pattern; (I–M) Elemental mappings.
Electrochemical Properties
Figure 3A shows a typical Ni@SnS2 electrode with a pair of redox peaks at a scan rate of 5 mV s−1. With increasing scan rates, the shapes of these CV curves nearly retain their initial state when the scan rate is as high as 100 mV s−1. As shown in Figure 3B, the GCD curves of the samples display almost symmetric shapes at all current densities from 1 to 100 mA cm−2, suggesting ideal pseudocapacitance properties (Li et al., 2021).
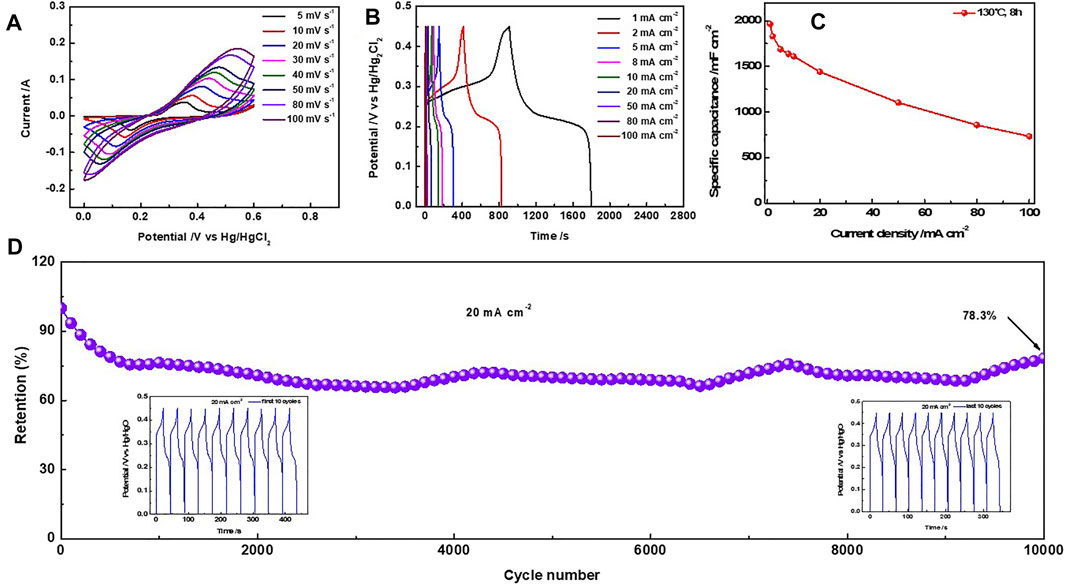
FIGURE 3. (A) CV curves of the Ni@SnS2 nanosheet arrays at different scan rates; (B) GCD curves; (C) Rate performance; (D) Cycle stability at 20 mA cm−2 (inset: the lower left corner shows the GCD curves of the first 10 cycles, and the lower right corner shows the GCD curves of the last 10 cycles).
When current density was increased from 1 to 100 mA cm−2 (Figure 3C), the specific capacitance decreased from 1965.56 to 733.78 mF cm−2. Furthermore, 75% of the initial specific capacitance was maintained even at a high current density of 20 mA cm−2. Here, the specific capacitances of bare Ni foam measured in Supplementary Figure S5 show much less than to the values of Ni@SnS2 electrode, suggesting the ignorable influence. The following relationship (Bian et al., 2022) is established between the peak current i) and scan rate (v):
Conclusion
Porous Ni@SnS2 nanosheet arrays were successfully prepared by a facile solvothermal method using Ni foam as the 3D framework. Due to the unique structure and crystal composition, the Ni@SnS2 electrode exhibited remarkable supercapacitive performance with high specific capacity and good cycling stability.
Data Availability Statement
The original contributions presented in the study are included in the article/Supplementary Material, further inquiries can be directed to the corresponding author.
Author Contributions
HS: Conceptualization, Supervision SL: Data curation, Writing—original draft WZ and CL: Data analysis CZ and ZJ: Visualization, Investigation SL: Methodology WX: review and editing.
Funding
This work was supported by the National Natural Science Foundation of China (11874317), Key Scientific Research Projects of Colleges and Universities in Henan Province (22A140009), XYNU Excellent MD Students Major Foundation (2021KYJJ07) and Nanhu Scholars Program for Young Scholar of XYNU. This work made use of instruments in analysis testing center of XYNU.
Conflict of Interest
The authors declare that the research was conducted in the absence of any commercial or financial relationships that could be construed as a potential conflict of interest.
Publisher’s Note
All claims expressed in this article are solely those of the authors and do not necessarily represent those of their affiliated organizations, or those of the publisher, the editors and the reviewers. Any product that may be evaluated in this article, or claim that may be made by its manufacturer, is not guaranteed or endorsed by the publisher.
Supplementary Material
The Supplementary Material for this article can be found online at: https://www.frontiersin.org/articles/10.3389/fenrg.2022.870329/full#supplementary-material
References
Bai, X., Tong, X., Gao, Y., Zhu, W., Fu, C., Ma, J., et al. (2018). Hierarchical Multidimensional MnO2 via Hydrothermal Synthesis for High Performance Supercapacitors. Electrochimica Acta 281, 525–533. doi:10.1016/j.electacta.2018.06.003
Bian, Z., Zhang, Z., Zhao, K., Li, K., Xiao, Q., Li, K., et al. (2022). 3D Net Carbon-Based Cobalt Sulfides for High-Performance Supercapacitors by a Simple, green and Convenient Method. Mater. Lett. 311, 131539. doi:10.1016/j.matlet.2021.131539
Cao, K., Wang, S., Jia, Y., Xu, D., Liu, H., Huang, K.-J., et al. (2021). Promoting K Ion Storage Property of SnS2 Anode by Structure Engineering. Chem. Eng. J. 406, 126902. doi:10.1016/j.cej.2020.126902
Fu, J., Jiang, X., Han, W., and Cao, Z. (2021). Enhancing the Cycling Stability of Transition-Metal-Oxide-Based Electrochemical Electrode via Pourbaix Diagram Engineering. Energ. Storage Mater. 42, 252–258. doi:10.1016/j.ensm.2021.07.037
Javed, M. S., Lei, H., Wang, Z., Liu, B.-t., Cai, X., and Mai, W. (2020). 2D V2O5 Nanosheets as a Binder-free High-Energy Cathode for Ultrafast Aqueous and Flexible Zn-Ion Batteries. Nano Energy 70, 104573. doi:10.1016/j.nanoen.2020.104573
Javed, M. S., Shah, S. S. A., Najam, T., Siyal, S. H., Hussain, S., Saleem, M., et al. (2020). Achieving High-Energy Density and superior Cyclic Stability in Flexible and Lightweight Pseudocapacitor through Synergic Effects of Binder-free CoCa2O4 2D-Hexagonal Nanoplates. Nano Energy 77, 105276. doi:10.1016/j.nanoen.2020.105276
Javed, M. S., Shaheen, N., Hussain, S., Li, J., Shah, S. S. A., Abbas, Y., et al. (2019). An Ultra-high Energy Density Flexible Asymmetric Supercapacitor Based on Hierarchical Fabric Decorated with 2D Bimetallic Oxide Nanosheets and MOF-Derived Porous Carbon Polyhedra. J. Mater. Chem. A. 7, 946–957. doi:10.1039/c8ta08816k
Jiang, X., Yang, X., Zhu, Y., Shen, J., Fan, K., and Li, C. (2013). In Situ assembly of Graphene Sheets-Supported SnS2 Nanoplates into 3D Macroporous Aerogels for High-Performance Lithium Ion Batteries. J. Power Sourc. 237, 178–186. doi:10.1016/j.jpowsour.2013.03.048
Li, X., Zhao, Y., Yao, Q., and Guan, L. (2018). Encapsulating SnS2 Nanosheets into Hollow Carbon Sphere: A Yolk-Shell SnS2@C Composite with Enhanced Sodium Storage Performance. Electrochimica Acta 270, 1–8. doi:10.1016/j.electacta.2018.03.080
Li, Z., Guo, D., Wang, D., Sun, M., and Sun, H. (2021). Exploration of Metal/Ti3C2 MXene-Derived Composites as Anode for High-Performance Zinc-Ion Supercapacitor. J. Power Sourc. 506, 230197. doi:10.1016/j.jpowsour.2021.230197
Liu, J. D., Chang, Y. F., Chen, C., Guo, P. Q., Sun, K., Cao, D. L., et al. (2021). Sandwich-like SnS2/graphene Multilayers for Efficient Lithium/sodium Storage. Dalton Trans. 50, 14884–14890. doi:10.1039/d1dt00781e
Parveen, N., Ansari, S. A., Alamri, H. R., Ansari, M. O., Khan, Z., and Cho, M. H. (2018). Facile Synthesis of SnS2 Nanostructures with Different Morphologies for High-Performance Supercapacitor Applications. ACS Omega 3, 1581–1588. doi:10.1021/acsomega.7b01939
Qu, B., Ma, C., Ji, G., Xu, C., Xu, J., Meng, Y. S., et al. (2014). Layered SnS2-Reduced Graphene Oxide Composite - A High-Capacity, High-Rate, and Long-Cycle Life Sodium-Ion Battery Anode Material. Adv. Mater. 26, 3854–3859. doi:10.1002/adma.201306314
Sun, Y., Li, G., Xu, J., Lei, B., Feng, H., and Sun, Z. (2019). Impacts of Graphene Sheets on Photoelectric and Photocatalytic Activities of SnS2 Nanoparticles. Mater. Chem. Phys. 229, 92–97. doi:10.1016/j.matchemphys.2019.02.070
Wang, C., Qu, H., Peng, T., Mei, K., Qiu, Y., Lu, Y., et al. (2016). Large Scale α-Co(OH)2 Needle Arrays Grown on Carbon Nanotube Foams as Free Standing Electrodes for Supercapacitors. Electrochimica Acta 191, 133–141. doi:10.1016/j.electacta.2016.01.057
Xie, W., Xu, Z., Zheng, W., Wang, W., Wang, C., Zhang, C., et al. (2021). Fabrication of Ni3S2 Nanoneedle-Sheets Electrode Material with Ultrahigh Areal Capacity of over 15 mAh Cm−2. Mater. Lett. 282, 128716. doi:10.1016/j.matlet.2020.128716
Keywords: SnS2 nanosheet arrays, crystal growth, self-supported, pseudocapacitors, energy storage and conversion
Citation: Sun H, Liang S, Zheng W, Liu C, Zhang C, Ji Z, Liu S and Xie W (2022) Supported SnS2 Nanosheet Arrays on Ni Foam for Supercapacitors. Front. Energy Res. 10:870329. doi: 10.3389/fenrg.2022.870329
Received: 06 February 2022; Accepted: 21 March 2022;
Published: 26 April 2022.
Edited by:
Vivekanand Shukla, Chalmers University of Technology, SwedenCopyright © 2022 Sun, Liang, Zheng, Liu, Zhang, Ji, Liu and Xie. This is an open-access article distributed under the terms of the Creative Commons Attribution License (CC BY). The use, distribution or reproduction in other forums is permitted, provided the original author(s) and the copyright owner(s) are credited and that the original publication in this journal is cited, in accordance with accepted academic practice. No use, distribution or reproduction is permitted which does not comply with these terms.
*Correspondence: Haibin Sun, c3VuaGFpYmluQHh5bnUuZWR1LmNu
†These authors have contributed equally to this work