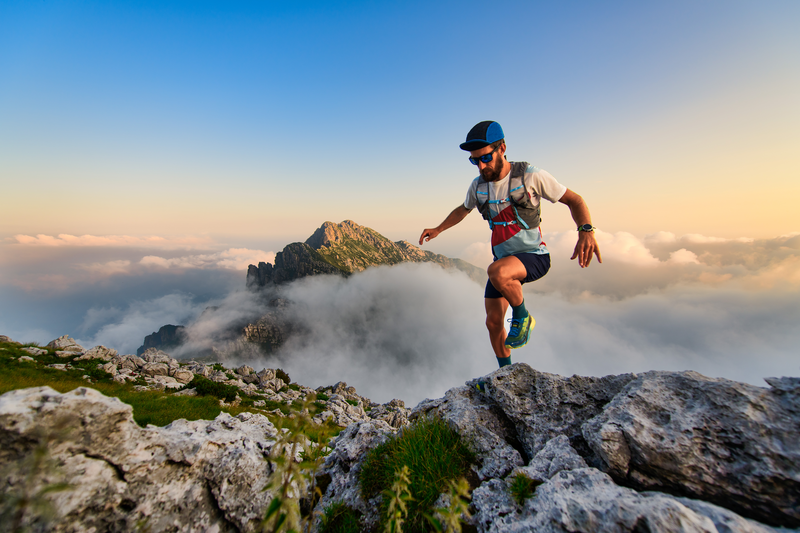
94% of researchers rate our articles as excellent or good
Learn more about the work of our research integrity team to safeguard the quality of each article we publish.
Find out more
OPINION article
Front. Energy Res. , 25 February 2022
Sec. Advanced Clean Fuel Technologies
Volume 10 - 2022 | https://doi.org/10.3389/fenrg.2022.860591
This article is part of the Research Topic Recent Advances in High-efficiency Development of Conventional/Unconventional Gas Reservoirs and CCUS Technologies View all 51 articles
As an unconventional energy source, natural gas hydrate is a polyhedral cage crystal formed by hydrogen bonding between methane and a small amount of non-hydrocarbon gases and water molecules under high pressure and low temperature (Sloan et al., 2007). Natural gas hydrates are widely distributed in permafrost and deep-water areas. The main methods of mining natural gas hydrate are depressurization method, heating method, inhibitor method, CO2 replacement method and solid exploitation method. The depressurization method and heating method are commonly used in the production of natural gas hydrate. The basic principle is to change the temperature and pressure of the environment where the hydrate is located, destroy the hydrate equilibrium state, and promote the decomposition of the hydrate into natural gas and water. Natural gas hydrate has attracted extensive attention of the state and industry due to its huge resource potential. It is known as one of the most promising strategic alternative energy sources for commercial development in the 21st century (Makogon et al., 2007). More than 30 countries around the world have successively formulated national key development strategies for natural gas hydrate and actively carried out hydrate exploration and development research (Yushan, 2012). Five countries including Japan, the United States, Canada, South Korea and China have carried out gas hydrate production (Le and He, 2021). On 17 February2020, the second round of pilot production was carried out in the water depth of 1,225 m. After 1 month of pilot production, the total gas production was 861400 m3 and the average daily gas production was 28700 m3, which was 2.8 times of the total gas production in the first round of 60 days (Li et al., 2018). China has become the world’s first country to use horizontal well drilling technology to test natural gas hydrate in the sea area (Ye and Qin, 2020). However, due to immature development technology, high cost, and environmental concerns, commercial exploitation of natural gas hydrate has not yet been realized.
Patent analysis has more reference significance for judging the Frontier, practicability and economic attributes of technology. Therefore, based on the INNOGRAPHY, this paper retrieves all patents published in 105 countries around the world by 5 January 2020. After manual screening and simple congregation processing, 3,993 patents related to natural gas hydrate development technology was obtained. The hydrate development technology analyzed in this paper refers to the development technology after drilling and completion, including laboratory experiments, numerical simulation, injection and production, flow simulation, new production methods and development technology.
As shown in Figure 1, in the last decade, the number of patents about natural gas hydrate applications has increased rapidly, and the development technology of natural gas hydrate is in a period of accelerated development. There is total 3,993 patents and 1,568 Chinese patents, accounting for 39.3% of the total patents. The United States, Japan and China have become hot spots for patent applications in turn. Due to the success of two trial production, China’s current patent application growth is the most prominent under the support of national policy.
Laboratory experimental research started early and developed rapidly. At present, it still accounts for a high proportion, indicating that the technical research in this field is still concentrated on basic theoretical research and laboratory production simulation. This is consistent with the fact that the exploitation of natural gas hydrates worldwide is still in the stage of basic research and short-term production, and the commercial exploitation is not really realized. It is worth noting that the number of patent applications for increasing production and lifting, development technology and equipment, which are closely related to the field exploitation of natural gas hydrate, has increased rapidly in recent years and has gradually become a technical hotspot.
The field test of natural gas hydrate is difficult, so the physical simulation experiment in laboratory has become the main measures to study the accumulation, production simulation and physical parameters of natural gas hydrate. The United States, South Korea, Germany, Japan and other countries have carried out research and development of natural gas hydrate physical simulation devices and experimental research (Phelps et al., 2001). In order to reduce the scale effect of the model, the physical simulation experiment devices are gradually large-scale. Guangzhou Institute of Energy Confusion, Chinese Academy of Sciences has developed a large-scale three-dimensional comprehensive experimental device for production wells with an internal volume of 2585 L. This device is the largest hydrate device that can be simulated internationally (Li and Chen, 2020). At the same time, the function of large-scale physical simulation experiment system is more comprehensive, which meets the needs of basic theoretical research in geology-development-engineering (Li and Wang, 2020). The application of acoustics, electrical method, time domain reflection technology (Ren et al., 2010), X-CT (Wang et al., 2018) and nuclear magnetic resonance spectroscopy (Meng and Liu, 2012) makes the experimental device of natural gas hydrate develop towards visualization and refinement, which provides basic knowledge and data for fine characterization and development technology of natural gas hydrate reservoirs.
At present, depressurization development is the most likely method for commercial development of natural gas hydrate. The latest research points out that the minimum critical production required for commercialization of natural gas hydrate is 160,000–250,000 m3 per day for a well (Chen et al., 2022). Compared with other unconventional oil and gas, commercial development of natural gas hydrate still depends on further technological breakthroughs, especially in terms of increasing production. Therefore, the development of natural gas hydrate development technology at this stage is in the direction of increasing production and reducing cost. In order to increase hydrate production, it is necessary to study the mechanism of efficient decomposition, flow simulation and heat transfer of natural gas hydrate in the target area (Zhao et al., 2014). From the perspective of expanding the decomposition area and improving the heat transfer capacity (Nair et al., 2018; Chandrasekharan Nair et al., 2019; Jian and Er-meng, 2021), various development methods such as comprehensive utilization of depressurization and heat injection, complex well types (Gao and Chen, 2021), well pattern development (Yu et al., 2020; Zhao and Feng, 2020) and reservoir stimulation to increase permeability (Too et al., 2018; Zhou and Xie 2021) were proposed. More and more researchers combine the above methods to achieve synergy and increase production (Feng et al., 2019; Lei and Lei, 2021).
The efficient development of natural gas hydrate needs to break through the development approach of the conventional oil and gas, and innovatively solve the problems of low permeability, weak cementation and complex hydrate occurrence types of gas hydrate sediments in deep-sea. For example, solid-state fluidization (Zhou and Li, 2014), using heat pipe and solar thermal chemical energy storage to realize heat injection (Lang and Cheng, 2021), using geothermal energy (Hou and Liu, 2019), flue gas heat (Song and Zheng, 2021) as heat source to inject into natural gas hydrate reservoir for development. Taking renewable energy as energy source, it is expected to achieve low-carbon development of natural gas hydrate in the future. In the future, the development of natural gas hydrate will take green, efficient and safe development as the ultimate goal, and will move towards the direction of new methods, new technologies, new processes and new equipment, such as physical simulation technology, numerical simulation technology, lifting technology, high-efficiency string and comprehensive monitoring system equipment for natural gas hydrate development based on fine characterization of natural gas hydrate reservoirs. By means of experimental simulation, numerical simulation and field application, innovative production methods, technical adaptability evaluation and technical optimization are carried out to support long-term production of natural gas hydrate and commercial development in the near future.
(1) This paper collects and analyzes global patent related to natural gas hydrate exploitation technology. Natural gas hydrate development technology is in a period of accelerated development. The United States, Japan and China have become the hot spots of patent applications.
(2) Due to the success of two trials, China’s current patent application growth under the support of national policy is the most prominent, and is most likely to become the first country to achieve commercial development in the future.
(3) As the basic measures of hydrate research, laboratory experimental research started early and developed rapidly, and still accounts for a high proportion at this stage. The physical simulation experiment device for natural gas hydrate development is developing towards the direction of large-scale, integration, diversification and visualization of test methods.
(4) At present, the development of natural gas hydrate development technology focuses on improving production and reducing costs. Therefore, efficient, green and safe production-increasing methods and technologies are the focus of attention in this field.
(5) In order to improve the production of natural gas hydrate, we should start from efficient decomposition, enhanced seepage and heat transfer, and innovate development ideas. If renewable energy is used as energy source, it is expected to achieve low-carbon development of natural gas hydrate in the future.
HW: Conceptualization, Methodology, Software LZ: Conceptualization, Methodology, Software, Investigation JH: Visualization, Project administration, Data Curation TZ: Supervision, Funding acquisition.
This work was supported by the Sinopec Ministry of Science and Technology project (Grant No. P20025, No. P20040-4). We also thank Key Special Project for Introduced Talents Team of Southern Marine Science and Engineering Guangdong Laboratory (Guangzhou) (GML2019ZD0102).
The authors declare that the research was conducted in the absence of any commercial or financial relationships that could be construed as a potential conflict of interest.
All claims expressed in this article are solely those of the authors and do not necessarily represent those of their affiliated organizations, or those of the publisher, the editors and the reviewers. Any product that may be evaluated in this article, or claim that may be made by its manufacturer, is not guaranteed or endorsed by the publisher.
Chandrasekharan Nair, V., Gupta, P., and Sangwai, J. S. (2019). Natural Gas Production from a Marine Clayey Hydrate Reservoir Formed in Seawater Using Depressurization at Constant Pressure, Depressurization by Constant Rate Gas Release, Thermal Stimulation, and Their Implications for Real Field Applications. Energy Fuels. 33 (APR), 3108–3122. doi:10.1021/acs.energyfuels.9b00187
Chen, X., Lu, H., Gu, L., Shang, S., Zhang, Y., Huang, X., et al. (2022). Preliminary Evaluation of the Economic Potential of the Technologies for Gas Hydrate Exploitation. Energy. 243, 123007. doi:10.1016/j.energy.2021.123007
Feng, Y., Chen, L., Suzuki, A., Kogawa, T., Okajima, J., Komiya, A., et al. (2019). Enhancement of Gas Production from Methane Hydrate Reservoirs by the Combination of Hydraulic Fracturing and Depressurization Method. Energ. Convers. Manag. 184, 194–204. doi:10.1016/j.enconman.2019.01.050
Gao, Y., and Chen, Y. (2021). Fish Bone Type Well Structure and Method for Exploiting Natural Gas Hydrate. CN108999604A. Qingdao, Shandong: China University of Petroleum (Huadong).
Hou, J., and Liu, Y. (2019). A Method of Exploiting Natural Gas Hydrate Reservoir Using Fluid Circulation Mode Using Geothermal Energy. CN107130944A. Qingdao, Shandong: China University of Petroleum (Huadong).
Jian, H., and Er-meng, Z. (2021). A marine Hydrate Mining Method for Assisting Low-Frequency Electric Field Heating by Sea Water Injection. CN113669041A. Guangzhou, Guangdong: South China University of Technology.
Lang, X., and Cheng, J. (2021). Natural Gas Hydrate Mining Method and Device Based on Heat Pipe Technology. CN113586014A.
Le, Z., and He, J. (2021). Research Progress of Numerical Simulation Technology of Natural Gas Hydrates Production. Sci. Technology Eng. 21 (28), 11891–11899. doi:10.3969/j.issn.1671-1815.2021.28.001
Lei, Y., and Lei, X. (2021). Synergistic Mining Equipment and Method of Horizontal Well Fracturing Filling Natural Gas Hydrate. CN111411922A. Dalian, Liaoning: Dalian University of Technology.
Li, J.-f., Ye, J., Ye, J.-l., Qin, X.-w., Qiu, H.-j., Wu, N.-y., et al. (2018). The First Offshore Natural Gas Hydrate Production Test in South China Sea. China Geology. 1 (1), 5–16. doi:10.31035/cg2018003
Li, X., and Chen, C. (2020). Full-size Natural Gas Hydrate Production Simulation Well Device and Experimental Method Thereof. CN111997568A. Guangzhou, Guangdong: Guangzhou Institute of Energy Conversion Chinese Academy of Sciences.
Li, X., and Wang, Y. (2020). Three-dimensional Comprehensive Test Mining System for Large-Scale Full-Size Mining Well. CN112031714A. Guangzhou, Guangdong: Guangzhou Institute of Energy Conversion Chinese Academy of Sciences.
Makogon, Y. F., Holditch, S. A., and Makogon, T. Y. (2007). Natural Gas-Hydrates - A Potential Energy Source for the 21st Century. J. Pet. Sci. Eng. 56 (1-3), 14–31. doi:10.1016/j.petrol.2005.10.009
Meng, Q., and Liu, C. (2012). In Situ Monitoring Ice Melting and Tetrahydrofuran Hydrates Dissociation with Magnetic Resonance Imaging. J. Basic Sci. Eng. 20 (1), 11–20. doi:10.3969/j.issn.1005-0930.2012.01.002
Nair, V. C., Prasad, S. K., Kumar, R., and Sangwai, J. S. (2018). Energy Recovery from Simulated Clayey Gas Hydrate Reservoir Using Depressurization by Constant Rate Gas Release, thermal Stimulation and Their Combinations. Appl. Energ. 225, 755–768. doi:10.1016/j.apenergy.2018.05.028
Phelps, T. J., Peters, D. J., Marshall, S. L., West, O. R., Liang, L., Blencoe, J. G., et al. (2001). A New Experimental Facility for Investigating the Formation and Properties of Gas Hydrates under Simulated Seafloor Conditions. Rev. Sci. Instrum. 72 (2), 1514–1521. doi:10.1063/1.1334628
Ren, S. R., Liu, Y., Liu, Y., and Zhang, W. (2010). Acoustic Velocity and Electrical Resistance of Hydrate Bearing Sediments. J. Pet. Sci. Eng. 70 (1-2), 52–56. doi:10.1016/j.petrol.2009.09.001
Sloan, E. D., and Koh, C. A. (2007). Clathrate Hydrates of Natural Gases. Boca Raton, Florida: CRC Press
Song, Y., and Zheng, J. (2021). A thermal Shock Natural Gas Hydrate Exploiting Device and Method Based on Smoke Waste Heat Absorbing Type Heat Pump. CN111852408B. Dalian, Liaoning: Dalian University of Technology.
Too, J. L., Cheng, A., Khoo, B. C., Palmer, A., and Linga, P. (2018). Hydraulic Fracturing in a Penny-Shaped Crack. Part II: Testing the Frackability of Methane Hydrate-Bearing Sand. J. Nat. gas Sci. Eng. 52, 619–628. doi:10.1016/j.jngse.2018.01.046
Wang, D., Wang, C., Li, C., Liu, C., Lu, H., Wu, N., et al. (2018). Effect of Gas Hydrate Formation and Decomposition on Flow Properties of fine-grained Quartz Sand Sediments Using X-ray CT Based Pore Network Model Simulation. Fuel. 226, 516–526. doi:10.1016/j.fuel.2018.04.042
Ye, J., and Qin, X. (2020). Main Progress of the Second Gas Hydrate Trial Production in the South China Sea. Geology. China. 47 (3), 557–568. doi:10.12029/gc20200301
Yu, T., Guan, G., Abudula, A., and Wang, D. (2020). 3D Investigation of the Effects of Multiple-Well Systems on Methane Hydrate Production in a Low-Permeability Reservoir. J. Nat. Gas Sci. Eng. 76, 103213. doi:10.1016/j.jngse.2020.103213
Yushan, L. (2012). New Trend of Exploration and Exploitation of Marinenatural Gas Hydrate (Ⅱ). Mineral. Deposits. 31 (1), 176–177. doi:10.16111/j.0258-7106.2012.01.014
Zhao, J., Liu, D., Yang, M., and Song, Y. (2014). Analysis of Heat Transfer Effects on Gas Production from Methane Hydrate by Depressurization. Int. J. Heat Mass Transfer. 77, 529–541. doi:10.1016/j.ijheatmasstransfer.2014.05.034
Zhao, T., and Feng, J. (2020). A Well Production System and Production Method of Natural Gas Hydrate. CN108412467B. Beijing: China University of Petroleum-Beijing.
Zhou, J., and Xie, W. (2021). Method and Device for Increasing and Improving Freezing and Fracturing of Reservoir of Natural Gas Hydrate. CN113586022A. Guangzhou, Guangdong: Guangzhou Marine Geological Survey.
Keywords: natural gas hydrate, exploitation technology, patents, renewable energy, commercial development
Citation: Wang H, Zhang L, He J and Zhou T (2022) The Development of Natural Gas Hydrate Exploitation Technology From Perspective of Patents. Front. Energy Res. 10:860591. doi: 10.3389/fenrg.2022.860591
Received: 23 January 2022; Accepted: 08 February 2022;
Published: 25 February 2022.
Edited by:
Xun Zhong, Yangtze University, ChinaReviewed by:
Yongge Liu, China University of Petroleum (East China), ChinaCopyright © 2022 Wang, Zhang, He and Zhou. This is an open-access article distributed under the terms of the Creative Commons Attribution License (CC BY). The use, distribution or reproduction in other forums is permitted, provided the original author(s) and the copyright owner(s) are credited and that the original publication in this journal is cited, in accordance with accepted academic practice. No use, distribution or reproduction is permitted which does not comply with these terms.
*Correspondence: Tong Zhou, dG9uZ196aG91MTk4NkAxNjMuY29t
Disclaimer: All claims expressed in this article are solely those of the authors and do not necessarily represent those of their affiliated organizations, or those of the publisher, the editors and the reviewers. Any product that may be evaluated in this article or claim that may be made by its manufacturer is not guaranteed or endorsed by the publisher.
Research integrity at Frontiers
Learn more about the work of our research integrity team to safeguard the quality of each article we publish.