- 1Southwest Petroleum University, Chengdu, China
- 2Second Gas Production Plant, Sinopec Southwest Oil and Gas Company, Langzhong, China
- 3Tarim Southwest Exploration and Development Company of PetroChina Tarim Oilfield Company, Korla, China
- 4Petrochina Xinjiang Oilfield Company, Karamay, China
Introduction
For a carbonate gas reservoir, there are mainly three porous types: pores, natural fractures, and vugs. According to the different combinations of diverse pore types, in fact, the carbonate gas reservoirs usually have three formation types: fractured-porous formation, porous-vuggy formation, and fractured-vuggy formation.
Through the evaluation of activity for edge water, a fractured-porous gas reservoir can be divided into two types: a fractured-porous gas reservoir with inactive edge water and a fractured-porous gas reservoir with active edge water for some areas. In this kind of reservoir, pores are the main gas storage space, and fractures are the main seepage channels. Although this kind of gas reservoir has a low permeable porous medium, it is connected for every place, and the whole gas reservoir is a unified hydrodynamic system. However, due to the non-uniform distribution of fractures, the reservoir shows strong heterogeneity. In a place with rich natural fractures and a broad connected area, the gas production rate is usually very high and can remain stable for a long time. In general, the natural fractures are abundant in the structural axis and steep zones, and there are few fractures in the gentle wing. Therefore, the gas production for wells located in in the wing area is low and cannot be stable. In the original state, there is a unified gas–water interface in the reservoir, and after the water breakthrough into the bottom of gas wells, the edge water will intrude along the stripes with rich fractures (Liu et al., 2021; Guo et al., 2020).
For a porous-vuggy gas reservoir with active bottom water, the pores are the dominant storage space, and there are a few vugs and fractures. Pores, caves, and natural fractures interpenetrate with each other, and the reservoir has strong heterogeneity. Fractures are the main seepage channels for gas and water, and the bottom water well intrudes along the fractures from horizontal and vertical directions. Due to the heterogeneity of gas reservoirs and the irregular intrusion of bottom water in the longitudinal and horizontal directions, each well has a certain control volume, such as the Dengying formation, Sinian reservoir, and Weiyuan gas field.
For a fractured-vuggy gas reservoir with active bottom or edge water, there are multiple natural fracture systems in a gas field, which are disconnected and well-trapped. In these natural fracture systems, generally the formation water surrounding the gas reservoir formation is active. As the natural fractures are dispersed, and the distribution range is complex, the heterogeneity of the production layer is very strong indeed. Therefore, for the same gas reservoir in a gas field, there is no uniform gas–water interface. The distribution of water is controlled by the natural fracture system, and even for the same fracture system, the distribution of gas and water is quite complex indeed. The primary patterns for gas reservoir and formation water can be summarized into four types: edge water, bottom water, gas isolation, and water isolation (Meng et al., 2018; Meng et al., 2020; Wang et al., 2020).
At present, most of developed gas reservoirs in the world are typical water-drive gas reservoirs with different aquifer potentials, and the gas reservoirs with active bottom or edge water can account for 40–50% in practice. Particularly for the Sichuan Basin, the gas reserves for water-bearing gas reservoirs account for more than 80% of the total reserves. Due to the serious influences of edge and bottom water invasion on the gas recovery, the mechanisms, dynamics, and influences for water influx are always the research hotspots in the field of natural gas exploitation. After the occurrence of water breakthrough, the gas flowing resistance will increase significantly, which leads to the decrease of gas well productivity. Meanwhile, influenced by the accumulation of fluids in the wellbore, the ability of blowing for gas wells is weakened, and in some cases, the gas production will stop due to serious liquid loading, which seriously affects the recovery of gas reservoirs (Meng, et al., 2021; Yan, et al., 2021).
Currently, the J2 gas reservoir of the HB gas field is in the early stage of development, and the issue of water influx is greatly serious, which significantly affects the gas production rate. However, the recognitions of water influx characteristics for this gas reservoir are insufficient, and it is difficult to guide the future development of gas reservoirs. Therefore, it is urgent to carry out the research on the water influx mode of gas reservoirs, which can provide some guidance for the strategies of water control measures on gas reservoirs or single wells in the later period.
The area of the J2 gas reservoir is about 66.41 km2, and the gas reserve is 1.405 billion cubic meters through the calculation by the volumetric method. The reserves controlled by the Heba 101 well and the surrounding wells are 50.3 billion cubic meters. This gas reservoir was put into production in 2010. After that, four gas wells began to produce water successively. For these wells, the water production is large, and the decline rate of gas production is diverse, which has great impacts on future gas production. Therefore, it is essential to study the water production performance of gas wells from the aspects of gas–water distribution, well logging interpretation, structural position, natural fracture locations, and water source tracing. The obtained results are very important for proposing the reasonable strategies to deal with water breakthrough and ensuring the stable production of gas wells (Wu, et al., 2019; Tabatabaei, et al., 2012; Kolawole et al., 2008).
Classical Water Influx Mode of Gas Reservoirs
(1) Typical issues for carbonate gas reservoirs with edge water
The J2 gas reservoir was firstly found in 2005 after the testing of the HJ 1 well in the J2 layer. In 2010, the HJ 203H well was put into production, and three wells (HJ201H, HJ202H, HJ206H) began to produce after 2020. At present, there are four production wells in total, and three of them remain in an open state. The daily gas and water production for these wells is 240,000 and 113 cubic meters per day, respectively, and the cumulative gas production is 296 million cubic meters. The decline rate for gas production is about 24%.
The gas bearing property for the J2 gas reservoir is dominated by the structure and beach body distribution. This gas reservoir has no uniform gas–water interface, and the structure is low, which causes that partial wells produce gas and water simultaneously, and the gas–water differentiation is incomplete indeed. Micro-fractures are rich in HJ201H, HJ202H, and HJ206H wells.
Formation distribution for the J2 gas reservoir is stable, and there is no water zone in the vertical direction. Through the preliminary analysis, the formation water may come from the areas in the edge or intermediate of beach that are located in the lower part of the ancient structure. Dynamically, there are obvious characteristics that the water is produced from natural fractures, and the comprehensive analysis results show that the water is more likely to come from the lower part of the south wing structure.
In the process of development, the gas reservoir exhibits several problems: rapid decline of gas well production, oil pressure, and water production and reduction of dominant reserves.
(2) Classification of water influx modes
Based on the investigation and analysis of more than 100 water-producing gas wells for water-bearing gas reservoirs in the Sichuan Basin, and the references on water influx patterns for water-bearing gas reservoirs, according to the production dynamic characteristics of water-producing gas wells, the recognition technology for water influx patterns in the J2 gas reservoir is proposed (Ruan et al., 2020). According to the curve shapes in the presented diagram, the influx modes for a reservoir with edge water can be divided into four types:
(1) Weak tongue water influx: The water–gas ratio rises slowly, and the water–gas ratio is relatively low, while the value is very stable.
(2) Strong tongue water influx: The water–gas ratio rises drastically, and the initial water–gas ratio is low, whereas it will increase faster in the later period.
(3) Water channeling along fractures: The water–gas ratio increases rapidly, and the time of water breakthrough for gas wells is earlier.
(4) Water channeling along high-conductivity fractures: The water–gas ratio rises dramatically, and the water–gas ratio rises faster after water breakthrough.
To show the aforementioned four water influx types clearly, the recognition diagram for water influx modes and the schematic for a geological section are presented in Figure 1.
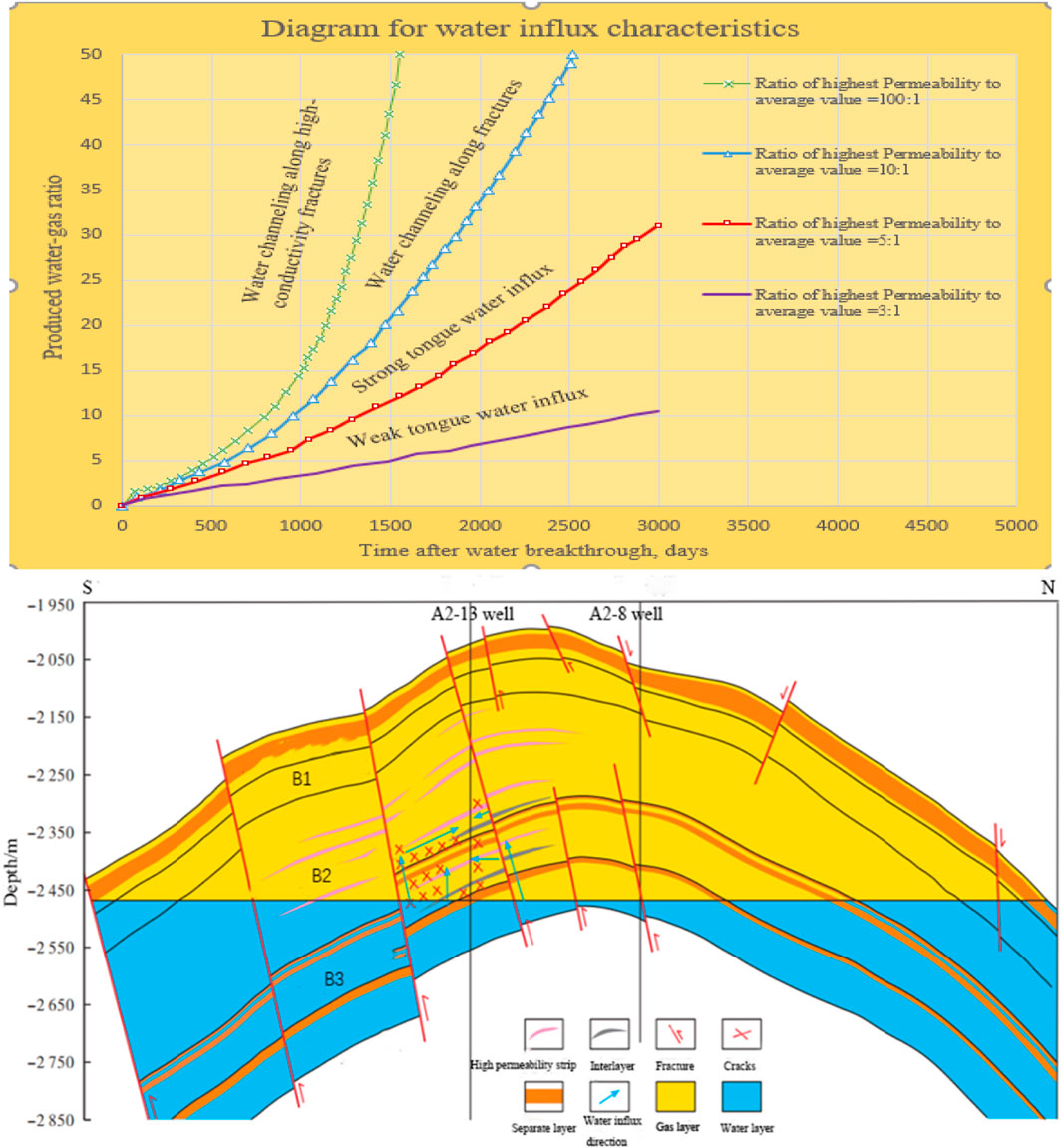
FIGURE 1. Recognition diagram for water influx modes and schematic for a geological section in a typical gas reservoir.
Conclusion
(1) According to different combinations of diverse pore types, the traditional carbonate gas reservoir can be divided into three types: fractured-porous formation, porous-vuggy formation, and fractured-vuggy formation.
(2) The J2 gas reservoir has rich fractures, and there is no unified gas–water interface. Comprehensive analysis results show that the water comes from the lower edge of the southern structure.
(3) There are four classical water invasion modes: weak tongue water influx, strong tongue water influx, water channeling along fractures, and water channeling along high-conductivity fractures.
Author Contributions
SH, JL, JS, and MG contributed equally to this work.
Conflict of Interest
SH was employed by Second Gas Production Plant, Sinopec Southwest Oil and Gas Company. JS was employed by Tarim Southwest Exploration and Development Company of PetroChina Tarim Oilfield Company. MG was employed by Petrochina Xinjiang Oilfield Company.
The remaining authors declare that the research was conducted in the absence of any commercial or financial relationships that could be construed as a potential conflict of interest.
Publisher’s Note
All claims expressed in this article are solely those of the authors and do not necessarily represent those of their affiliated organizations, or those of the publisher, the editors, and the reviewers. Any product that may be evaluated in this article, or claim that may be made by its manufacturer, is not guaranteed or endorsed by the publisher.
References
Guo, J., Meng, F., Jia, A., Dong, S., Yan, H., Zhang, H., et al. (2020). Production Behavior Evaluation on Multilayer Commingled Stress-Sensitive Carbonate Gas Reservoir. Energy Exploration & Exploitation 39 (1), 86–107. doi:10.1177/0144598720964155
Kolawole, A., Wattenbarger, R. A., and Maggard, J. B. (2008). Estimating Water Production Behavior from Edge-Water Drive in a Monocline Reservoir. CIPC/SPE Gas Technology Symposium, Paper SPE-114593-MS. doi:10.2118/114593-MS
Liu, X., Meng, F., Li, Q., Guo, Z., Shen, W., and Zhang, C. (2021). Absolute Open Flow (AOF) Potential Evaluation for Watered-Out Gas wells in Water-Drive Gas Reservoir. Pet. Sci. Tech. 39 (7-8), 249–269. doi:10.1080/10916466.2021.1895837
Meng, F., He, D., Yan, H., Zhao, H., Zhang, H., and Li, C. (2021). Production Performance Analysis for Slanted Well in Multilayer Commingled Carbonate Gas Reservoir. J. Pet. Sci. Eng. 204 (2021), 108769. doi:10.1016/j.petrol.2021.108769
Meng, F., Lei, Q., He, D., Yan, H., Jia, A., Deng, H., et al. (2018). Production Performance Analysis for Deviated wells in Composite Carbonate Gas Reservoirs. J. Nat. Gas Sci. Eng. 56, 333–343. doi:10.1016/j.jngse.2018.06.014
Meng, F., Su, Y., Wang, W., Lei, Q., and He, D. (2020). Semi-analytical Evaluation for water-alternating-CO2 Injectivity in Tight Oil Reservoirs. Ijogct 24 (1), 62–84. doi:10.1504/IJOGCT.2020.106704
Tabatabaei, M., Ghalambor, A., and Guo, B. (2012). An Analytical Solution for Water Coning in Vertical wells. SPE Prod. Operations 27 (2), 195–204. doi:10.2118/113106-PA
Wang, W., Meng, F., Su, Y., Hou, L., Geng, X., Hao, Y., et al. (2020). A Simplified Capillary Bundle Model for CO2-Alternating-Water Injection Using an Equivalent Resistance Method. Geofluids 2020, 1–14. doi:10.1155/2020/8836287
Wu, Y., Yang, M., Li, M., Sun, Y., Zhang, Y., and Wang, H. (2019). Laws and Models of Water Invasion in Kela 2 Gas Field. Xinjiang Pet. Geology. 40 (6), 302–306. doi:10.1155/2021/6631006
Keywords: invasion modes, water invasion, gas reservoirs, wellbore effusion, pore type
Citation: Huang S, Liu J, Sun J and Geng M (2022) Water Invasion Mode of Carbonate Gas Reservoirs Controlled by Edge Water: Three Invasion Modes. Front. Energy Res. 10:860527. doi: 10.3389/fenrg.2022.860527
Received: 23 January 2022; Accepted: 03 March 2022;
Published: 07 April 2022.
Edited by:
Xun Zhong, Yangtze University, ChinaReviewed by:
Yuhui Zhou, Yangtze University, ChinaXiang Zuping, Fisheries Research Institute of Zhao Qing, China
Copyright © 2022 Huang, Liu, Sun and Geng. This is an open-access article distributed under the terms of the Creative Commons Attribution License (CC BY). The use, distribution or reproduction in other forums is permitted, provided the original author(s) and the copyright owner(s) are credited and that the original publication in this journal is cited, in accordance with accepted academic practice. No use, distribution or reproduction is permitted which does not comply with these terms.
*Correspondence: Shilin Huang, ZGt3MTk5ODA4MDlAc2luYS5jb20=