- 1Key Laboratory of Geological Safety of Coastal Urban Underground Space, Ministry of Natural Resources, Qingdao, China
- 2Qingdao Geo-Engineering Surveying Institute (Qingdao Geological Exploration Development Bureau), Qingdao, China
- 3School of Environment and Municipal Engineering, Qingdao University of Technology, Qingdao, China
Shallow geothermal energy (SGE) is a part of geothermal resources and is mainly used through ground source heat pumps (GSHP). However, the potential of SGE varies from region to region due to different geological conditions. There is a lack of regulations and codes for assessing SGE, which makes the design and planning of GSHP restricted. In this study, an evaluation system of the suitability of GSHP in a region of Qingdao by using Analytic Hierarchy Process (AHP) is proposed, and the test area is divided into three suitability levels based on suitability scores. The evaluation system contains property indicators, elemental indicators, and their weights. The result shows that the highly suitable area for the application of GSHP in the test area is 110.04 km2, accounting for 41.8% of the whole test area. The area of moderately suitable area is 65.02 km2, accounting for 24.7%, and GSHP should be developed and utilized on the basis of full demonstration in this level. The unsuitable area for GSHP is 88.19 km2, accounting for 33.5%. The indicator weights in this article may only be applicable to the Qingdao area and cities with similar geological conditions to Qingdao. However, the indicators within this evaluation system can be applied to the vast majority of locations where GSHP are to be developed, as it provides a method of assessment in terms of geological conditions, groundwater conditions, construction conditions, and ecological aspects.
1 Introduction
Shallow geothermal energy (SGE) is a part of geothermal resources, which generally refers to the thermal energy resources in the interior of the earth with a temperature of less than 25°C from the thermostatic zone to 200 m burial depth, and has the value of development and utilization at present. In China, it has been proven that the SGE resources available within 1.69 × 105 km2 are equivalent to 7 × 1012 kg of coal (Xu et al., 2020). In 2021, 58.8% of geothermal energy resources was extracted through ground source heat pumps (GSHP) (Lund et al., 2021), which provide energy by the borehole heat exchanger (BHE) (Sarbu et al., 2014). According to the current GSHP code in China (GB 50366-2009, 2009), the ground temperature and thermal conductivity obtained from thermal response tests are essential parameters. However, these two parameters cannot represent the development potential of SGE and efficiency of GSHP in detail (Luo et al., 2016). Moreover, BHE is usually deeper than 100 m, which means the initial investment is costly. Therefore, before designing GSHP, the local geological environment, thermophysical properties of the soil, groundwater conditions, and construction costs need to be evaluated to ensure the efficiency of GSHP (Casasso et al., 2017).
However, these influencing factors do not have a clear description of their contribution. Firstly, the geological, climatic, and hydrological conditions vary from region to region and are difficult to generalize by the same criteria. Secondly, the factors are not independent of each other but interact with each other. For example, lithology will largely determine the ability of heat transfer (He et al., 2017; Kai-Qi et al., 2020), as well as the microscopic particle shape (Wang et al., 2019), porosity (Dong et al., 2015), saturation (Tong et al., 2009), and groundwater conditions (Chen, 2008; Zhang et al., 2015) of the rock can also contribute to changes in thermal conductivity (Dong et al., 2021). In engineering, lithology and rock thickness can also affect construction difficulty and drilling costs. In fact, one of the most important factors of concern for engineering is construction costs. Construction methods vary in different geological conditions, which lead to the differences in construction costs. Thirdly, the safety and sustainability of GSHP also need to be considered, which makes the evaluation of SGE a task that requires multidisciplinary and multisectoral cooperation. At present, the development of SGE is still in the exploration stage, and there is a lack of systematic technical codes for evaluation and calculation methods of SGE (Wang et al., 2020).
The Analytic Hierarchy Process (AHP), developed by Saaty (1988), quantifies the empirical judgment of decision makers and is suitable for problems that are difficult to analyze completely quantitatively, such as evaluating SGE. AHP uses various types of indicators to form an evaluation system (Tinti et al., 2018). This system generally includes the influencing factors from the above literature, but the contribution of each indicator will be different. Therefore, the evaluation system cannot be applied to every region due to the different geological conditions. This article establishes an evaluation system applicable to the Qingdao area based on the geographic conditions, urban characteristics, and geological conditions. The evaluation system consists of four attribute indicators and 11 element indicators, and their weights are analyzed and calculated. Finally, this article uses the geographic information system (GIS) to classify the evaluation area and obtain an effective SGE suitability index. The research results can provide guidance to the development of GSHP.
2 Materials and Methods
2.1 Analytic Hierarchy Process
AHP is essentially the formalization of our intuitive understanding of a complex problem using a hierarchical structure (Saaty, 1994). The AHP is a systematic analysis method that integrates qualitative and quantitative analyses. It quantifies the empirical decision of policymakers and is applicable to problems that are difficult to analyze completely quantitatively. It is applied to multiple fields (Vaidya et al., 2006). The structure at least has three levels: the focus or the overall goal of the problem on the top level, multiple criteria that define alternatives in the middle level, and competing alternatives in the bottom level. The main application of SGE is GSHP, thus the AHP system in this study will be built on all factors that affect GSHP. In this article, there are three steps in AHP modelling:
1) The influencing factors are analyzed and a hierarchy is created. The details are shown in Section 2.2.
2) The judgment matrices for each level are constructed. The property indicators in the second layer are compared pairwise, and then the elemental indicators under each property indicator are also compared pairwise. Two indicators,
where
3) The consistency of each judgment matrix is checked. First, the consistency indicator
where
Third, when the consistency ratio
2.2 Establishing the Evaluation System
2.2.1 Evaluation Indicators
In the AHP, the evaluation indicators are divided into two levels. The first level of indicators is property indicators, which provides a general description of all the indicators that affect the decision. In this study, since the water quality and quantity in the test area are not suitable for hybrid GSHP, the closed-loop GSHP with vertical borehole is the subject. According to the code for the evaluation of SGE in Shandong Province (DB37/T 4308-2021, 2021), the selection of property indicators includes geological and hydrogeological conditions, engineering geological conditions, thermophysical conditions, and geological environmental conditions. The second level of indicators is the elemental indicators, which are elements that can describe property indicators. These elemental indicators may be interacting (DZ/T 0225-2009, 2009), for example, lithology and groundwater conditions will affect thermal conductivity and specific heat capacity. The hierarchical analysis can effectively resolve these ambiguities.
The evaluation system shown in Figure 1 is established. In order to ensure the long-term operational efficiency of GSHP and to protect the local ecology (Hähnlein et al., 2013), the ecological protection area and the metro line are used as the restricted area in this study, which are directly labeled as poor suitability areas.
1) Geological and hydrogeological conditions
This index includes rock thickness, groundwater depth, aquifer thickness, and gushing volume. Underground rock thickness affects the difficulty of BHE construction and the initial investment of engineering. During the construction of BHE, if there is a lot of gravel and sand, a casing will be required when drilling. The best drilling conditions are achieved when the thickness of the rock is greater than the length of the BHE. In addition, the more complex the underground stratification, the higher the difficulty and cost during the construction of the BHE.
The groundwater depth affects the heat transfer of the BHE. The heat and cold load released by the BHE are carried away by the groundwater and then energy transfers quickly between the groundwater and soil (Zhang et al., 2020). This makes the accumulation of underground cold and heat loads less likely to form, thus enabling a more durable and stable provision of energy. The aquifer thickness and gushing volume determine the effect of underground heat transfer and heat exchange conditions of the soil. Although the permeability coefficient is a representative of groundwater transport, there are strips of aquifer rock structure in the test area (Figure 2C). The aquifer thickness and gushing volume would be more representative of the groundwater conditions in the test area.
2) Engineering geological conditions
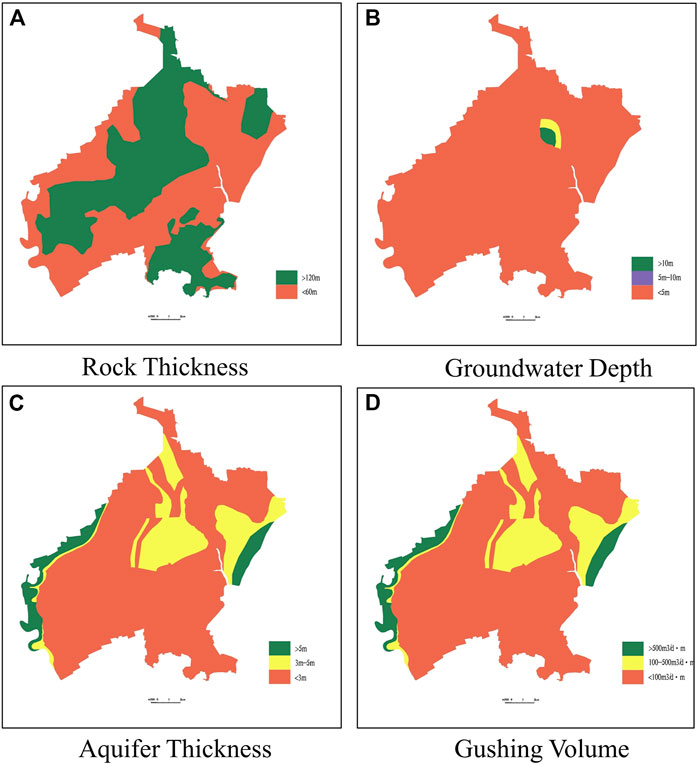
FIGURE 2. The map of geological and hydrological conditions. (A) Rock thickness, (B) groundwater depth, (C) aquifer thickness, and (D) gushing volume.
This index includes the lithology and the alluvial thickness. The lithology affects the heat transfer capacity of BHE. Generally, bedrock such as granite has higher thermal conductivity and higher heat transfer efficiency (VDI 4640/1, 2010). The alluvial thickness mainly considers the difficulty of hole formation during the drilling operation. If the thickness of alluvial is too large, it is not only difficult to drill but also prone to collapse after lifting the drill, which makes it difficult to build a borehole.
3) Thermophysical conditions
This index includes thermal conductivity, specific heat capacity, and ground temperature. Thermal conductivity reflects energy transfer, which directly determines the level of heat transfer capacity of the BHE. The specific heat capacity represents the heat absorbed or released when the temperature of the soil changes and can indicate the amount of SGE contained in the soil. The ground temperature indicates the gradient of the earth's temperature and the earth's heat flow; the higher the temperature, the more abundant the SGE (Zhang et al., 2019).
4) Geological environmental conditions
Geological environmental conditions include topography and geological hazards, which are mainly concerned on the construction and operation of GSHP. The smoother the topography and lesser the geological hazards, the better it is for organizing the construction and later the operation.
2.2.2 Quantification of Indicators
The indicators mentioned above use different forms and scales of data. In order to compare and calculate different data within the same evaluation system, it is necessary to standardize the data before evaluation. In this study, the standardization of data is done by dividing the range of each element into three levels of criteria, i.e., 9, 5, and 1, based on whether the GSHP is suitable for construction. In Table 2, the scoring used here is shown, i.e., 1∼9 intended to increase the variation in the final score. Due to the large number of elemental indicators, the weights do not differ significantly in value. If smaller scores are used, the range of the final scores would be so small that it would be difficult to assign a suitability level. The more favorable the GSHP, the higher the score obtained, thus transforming all data into dimensionless values that can be compared with each other. The quantitative grading of each indicator is based on both the geological environment conditions and the operability of the system in the process of quantification. The quantification of each indicator is shown in Table 2. After quantification, the maps of each indicator are drawn in the GIS, and the results are shown in Figures 2–5.
The classification of the range of each indicator is based on the Chinese code (DZ/T 0225-2009, 2009; DB37/T 4308-2021, 2021) and on real GSHP applications. It is important to emphasize that the lithology of the strata in the test area can be divided into two categories: bedrock zone and loose layer. In the bedrock zone, the rock thickness is all greater than 120 m, which is the usual length of the buried pipe. In the loose layers, where the rock thickness is less than 60 m, gravel and sandy soils lead to increased construction costs and therefore score lower. In addition, the groundwater in the test area is barren and unsuitable for hybrid GSHP. However, the transport of groundwater will significantly enhance the heat transfer capacity of the BHE. In order to demonstrate the influence of groundwater, three groundwater-related indicators, namely, the groundwater depth, aquifer thickness, and gushing volume, are partitioned according to the results of the recharge experiment.
2.2.3 The Weights of Indicator
In this study, the AHP was used to determine the indicators' weights. On the basis of the hierarchical relationship of the evaluation system, the importance of each indicator in the property layer and the element layer is compared separately using the 1–9 scale method to form a comparison matrix. The consistency of the comparison matrix is checked by calculation. If necessary, the comparison matrix is modified to finally reach an acceptable consistency and finally the weights of each indicator is determined.
The calculation and results of the weights of the property indicators are presented in Table 3 and are analyzed by consistency analysis. Among the four property indicators, the thermophysical condition is the most important because it reflects the heat exchange capacity of the GSHP. The geological and hydrogeological conditions reflect the SGE and the main factors affecting heat exchange.
Among the four indicators of geological and hydrogeological conditions, the rock thickness has the greatest weight and aquifer thickness has the least weight. The aquifer thickness is related to the type of geology in the test area, which is less water-rich (Figure 2). Therefore, the aquifer thickness has little influence. The two hydrogeological indicators, groundwater depth and gushing volume, mainly represent their influence on the heat transfer, both of which are slightly more important than the aquifer thickness. The calculation and results of the weights of geological and hydrogeological conditions are presented in Table 4.
The engineering geological conditions include two indicators: lithology and alluvial thickness, with lithology being given more weight than alluvial thickness. The lithology represents the difference of the physical and thermal physical characteristics of the soil, which has an important influence on the suitability zoning and resource calculation. The lithology is more important than the alluvial thickness because the distribution of the floodplain is less and is not representative (Figure 3). The calculation and results of the weights of engineering geological conditions are presented in Table 5.
In the design and utilization of GSHP, the thermophysical properties, as an extremely important aspect, influence the calculation of suitability zoning and resource volume (Figure 4). Among them, the thermal conductivity is directly involved in the calculation of heat transfer as an indicator. Therefore, the thermal conductivity is the most important indicator. The specific heat capacity and ground temperature represent the magnitude of the SGE and also have an influence on the suitability zoning. The calculation and results of the weights of thermophysical conditions are presented in Table 6.
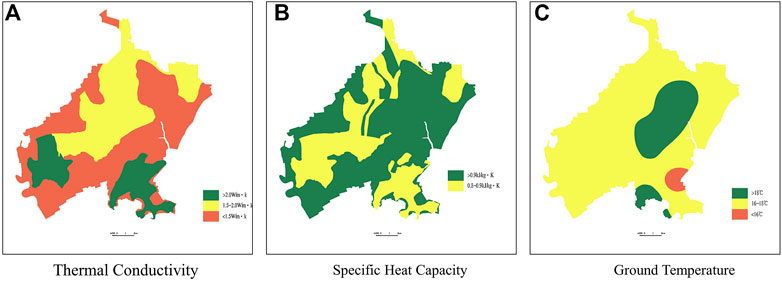
FIGURE 4. The map of thermophysical conditions. (A) Thermal conductivity, (B) specific heat capacity, and (C) ground temperature.
Geological environment conditions include two indicators: terrain slope and geological hazards (Figure 5). The terrain slope affects the difficulty of GSHP construction, while geological hazards are directly responsible for whether GSHP can be implemented. Therefore, the weight of the geological hazards is higher than the terrain slope. The calculation and results of the weights of the thermophysical conditions are presented in Table 7.
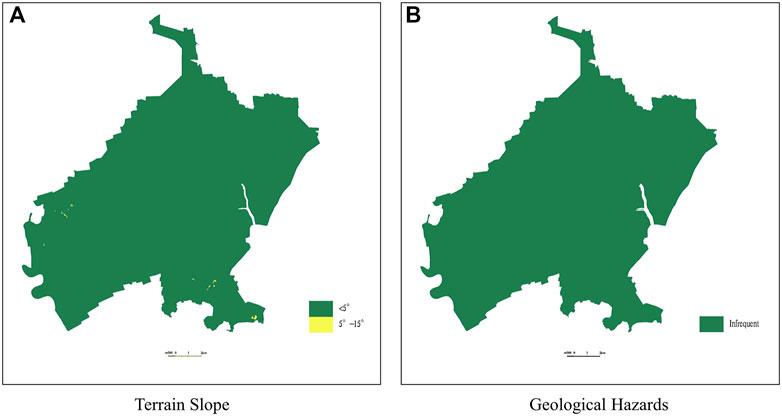
FIGURE 5. The map of geological environmental conditions. (A) Terrian slope. (B) Geological hazards.
The weights of all indicators are presented in Table 8. The thermophysical conditions are the property indicators with the highest weight, which lead to the thermal conductivity becoming the highest elementary indicator. The importance of the geological and hydrogeological conditions is also high.
3 Result and Discussion
According to the above weights, each indicator is assigned a value in the GIS multiplied by its corresponding weight, and then summed up to derive the score on each point. In this article, according to the scores, the areas with scores greater than 5 are set as high suitability areas (A), 3–5 as medium suitability areas (B), and less than 3 as low suitability areas (C). The results are shown in Figure 6.
In Figure 6, area A covers an area of 110.04 km2, accounting for 41.8% of the test area. It is mainly distributed in the northeast, central, southwest, and southeast of the working area. Area A is a bedrock area (Figure 3A), and the lithology is mainly basalt, coarse andesite, andesite, breccia, etc. The thickness of the rock is greater than 120 m (Figure 2A), and the construction conditions are moderate. The thermal conductivity is greater than 2.0 w/(mK) in the southwestern region and the south-eastern region (Figure 4A), with good heat transfer conditions. The lithology of the central region is basalt, and the thickness of the aquifer is moderate. The specific heat capacity is greater than 0.9 kJ/(kgK) (Figure 4B), which means that the heat transfer capacity is strong. It is suitable for the development and utilization of GSHP in area A.
Area B covers 65.02 km2, accounting for 24.7%. It is mainly located in the central and north-eastern bedrock areas, and the lithology is mainly basalt, coarse andesite, andesite, breccia, etc. (Figure 3A). The thickness of the rock is more than 120 m (Figure 2A), which means the drilling conditions are suitable. The thermal conductivity of the central and north-eastern part is 1.5–2.0 w/(mK) (Figure 4A). Thus, the comprehensive heat transfer capacity of the geotechnical layer is strong. In addition, the ground temperature conditions are good. The GSHP should be developed and utilized on the basis of full demonstration in area B.
Area C covers 88.19 km2, accounting for 33.5%. Area C is not only the area with a score less than 3 but also that which includes the ecological protection zone and metro area. It is mainly located in the loose layer (Figure 3A) with rock thickness less than 60 m (Figure 2A). The thermal conductivity is less than 1.5 w/(mK) (Figure 4A), and the heat transfer capacity is poor. It is not suitable for the development of GSHP.
4 Conclusion
This study establishes an evaluation system based on the geographic conditions, urban characteristics, and geological conditions in Qingdao. After the analysis and calculation of AHP, the weights of the elemental indicators are calculated. Then, the suitability zones are mapped through GIS. The following conclusions are drawn:
1) In the evaluation system, although the thermal conductivity is the most important indicator, geological conditions and hydrogeological conditions are also of high importance. The thermal conductivity alone cannot be considered when designing GSHP.
2) The AHP-based evaluation system can provide an effective solution of suitability evaluation for SGE exploration in the GSHP application in the Qingdao region. This system can be extended to places with similar geological conditions in Qingdao and provide a reference for evaluation of SGE in other regions.
3) In the test area, the area of high suitability (area A) is 110.04 km2, accounting for 41.8%. The area of medium suitability (area B) covers 65.02 km2, accounting for 24.7%. The area of low suitability (area C) covers 88.19 km2, accounting for 33.5%.
4) Due to geological differences, the weights used in this article may not be applicable to all regions. In addition, although the AHP is already a very mature evaluation method, its subjectivity is inevitable. It is recommended that a more objective evaluation method be used for subsequent use as a basis for development.
Data Availability Statement
The original contributions presented in the study are included in the article/Supplementary Material, further inquiries can be directed to the corresponding author.
Author Contributions
JD conducted the experiments and wrote the manuscript. PH and HoL have corrected and edited the manuscript. YG and HaL supported the project technically. WX and JrD were responsible for software data curation.
Conflict of Interest
The authors declare that the research was conducted in the absence of any commercial or financial relationships that could be construed as a potential conflict of interest.
Publisher’s Note
All claims expressed in this article are solely those of the authors and do not necessarily represent those of their affiliated organizations, or those of the publisher, the editors, and the reviewers. Any product that may be evaluated in this article, or claim that may be made by its manufacturer, is not guaranteed or endorsed by the publisher.
Abbreviations
AHP, Analytic Hierarchy Process; BHE, borehole heat exchanger; GSHP, ground source heat pumps; SGE, shallow geothermal energy;
References
Casasso, A., and Sethi, R. (2017). Assessment and Mapping of the Shallow Geothermal Potential in the Province of Cuneo (Piedmont, NW Italy). Renew. Energ. 102, 306–315. doi:10.1016/j.renene.2016.10.045
Chen, S. X. (2008). Thermal Conductivity of Sands. Heat Mass. Transfer 44 (10), 1241–1246. doi:10.1007/s00231-007-0357-1
DB37/T 4308-2021 (2021). Specification for Investigation and Evaluation of Regional Shallow Geothermal Energy. Beijing, China: Shandong Administration for Market Regulation.
Dong, J., Li, X., Han, B., Tian, R., and Yu, H. (2022). A Regional Study of In-Situ thermal Conductivity of Soil Based on Artificial Neural Network Model. Energy and Buildings 257, 111785. doi:10.1016/j.enbuild.2021.111785
Dong, Y., McCartney, J. S., and Lu, N. (2015). Critical Review of Thermal Conductivity Models for Unsaturated Soils. Geotech Geol. Eng. 33, 207–221. doi:10.1007/s10706-015-9843-2
DZ/T 0225-2009 (2009). Specification for Shallow Geothermal Energy Investigation and Evaluation. Beijing, China: Ministry of Land and Resources of the People's Republic of China.
Hähnlein, S., Bayer, P., Ferguson, G., and Blum, P. (2013). Sustainability and Policy for the thermal Use of Shallow Geothermal Energy. Energy Policy 59, 914–925. doi:10.1016/j.enpol.2013.04.040
He, H., Zhao, Y., Dyck, M. F., Si, B., Jin, H., Lv, J., et al. (2017). A Modified Normalized Model for Predicting Effective Soil thermal Conductivity. Acta Geotech. 12, 1281–1300. doi:10.1007/s11440-017-0563-z
Li, K.-Q., Li, D.-Q., and Liu, Y. (2020). Meso-scale Investigations on the Effective thermal Conductivity of Multi-phase Materials Using the Finite Element Method. Int. J. Heat Mass Transfer 151, 119383. doi:10.1016/j.ijheatmasstransfer.2020.119383
Lund, J. W., and Toth, A. N. (2021). Direct Utilization of Geothermal Energy 2020 Worldwide Review. Geothermics 90, 101915. doi:10.1016/j.geothermics.2020.101915
Luo, J., Rohn, J., Xiang, W., Bertermann, D., and Blum, P. (2016). A Review of Ground Investigations for Ground Source Heat Pump (GSHP) Systems. Energy and Buildings 117, 160–175. doi:10.1016/j.enbuild.2016.02.038
Saaty, T. L. (1994). How to Make a Decision: the Analytic Hierarchy Process. Interfaces 24 (6), 19–43. doi:10.1287/inte.24.6.19
Saaty, T. L. (1988). “What Is the Analytic Hierarchy Process,” in Mathematical Models for Decision Support (Berlin, Heidelberg: Springer), 109–121. doi:10.1007/978-3-642-83555-1_5
Saaty, T. L. (1990). Decision Making for Leaders: The Analytic Hierarchy Process for Decisions in a Complex World (RWS Publications).
Sarbu, I., and Sebarchievici, C. (2014). General Review of Ground-Source Heat Pump Systems for Heating and Cooling of Buildings. Energy and buildings 70, 441–454. doi:10.1016/j.enbuild.2013.11.068
Tinti, F., Kasmaee, S., Elkarmoty, M., Bonduà, S., and Bortolotti, V. (2018). Suitability Evaluation of Specific Shallow Geothermal Technologies Using a GIS-Based Multi Criteria Decision Analysis Implementing the Analytic Hierarchic Process. Energies 11 (2), 457. doi:10.3390/en11020457
Tong, F., Jing, L., and Zimmerman, R. W. (2009). An Effective thermal Conductivity Model of Geological Porous media for Coupled Thermo-Hydro-Mechanical Systems with Multiphase Flow. Int. J. Rock Mech. Mining Sci. 46 (8), 1358–1369. doi:10.1016/j.ijrmms.2009.04.010
Vaidya, O. S., and Kumar, S. (2006). Analytic Hierarchy Process: An Overview of Applications. Eur. J. Oper. Res. 169 (1), 1–29. doi:10.1016/j.ejor.2004.04.028
Wang, C., Lai, Y., Zhang, M., and Li, S. (2019). A Generalized thermal Conductivity Model of Geomaterials Based on Micro-structures. Acta Geotech. 14, 1423–1436. doi:10.1007/s11440-018-0728-4
Wang, G., Liu, Y., Zhu, X., and Zhang, W. (2020). The Status and Development Trend of Geothermal Resources in China. EARTH SCIENCE FRONTIERS 27 (01), 312–321. doi:10.13745/j.esf.2020.1.1
Xu, Y.-S., Wang, X.-W., Shen, S.-L., and Zhou, A. (2020). Distribution Characteristics and Utilization of Shallow Geothermal Energy in China. Energy and Buildings 229, 110479. doi:10.1016/j.enbuild.2020.110479
Zhang, B., Gu, K., Shi, B., Liu, C., Bayer, P., Wei, G., et al. (2020). Actively Heated Fiber Optics Based thermal Response Test: A Field Demonstration. Renew. Sustain. Energ. Rev. 134, 110336. doi:10.1016/j.rser.2020.110336
Zhang, C., Song, W., Liu, Y., Kong, X., and Wang, Q. (2019). Effect of Vertical Ground Temperature Distribution on Parameter Estimation of In-Situ thermal Response Test with Unstable Heat Rate. Renew. Energ. 136, 264–274. doi:10.1016/j.renene.2018.12.112
Keywords: shallow geothermal energy (SGE), ground source heat pump (GSHP), Analytic Hierarchy Process (AHP), evaluation system, suitability zoning
Citation: Dong J, He P, Liu H, Guan Y, Liu H, Xia W and Dong J (2022) AHP-Based Evaluation of the Suitability of Shallow Geothermal Energy Utilization in GSHP System. Front. Energy Res. 10:859454. doi: 10.3389/fenrg.2022.859454
Received: 21 January 2022; Accepted: 08 February 2022;
Published: 03 March 2022.
Edited by:
Huaqing Xie, Northeastern University, ChinaReviewed by:
Bo Han, Heriot-Watt University, United KingdomFujiao Tang, Harbin Institute of Technology, China
Wei Song, North China University of Technology, China
Copyright © 2022 Dong, He, Liu, Guan, Liu, Xia and Dong. This is an open-access article distributed under the terms of the Creative Commons Attribution License (CC BY). The use, distribution or reproduction in other forums is permitted, provided the original author(s) and the copyright owner(s) are credited and that the original publication in this journal is cited, in accordance with accepted academic practice. No use, distribution or reproduction is permitted which does not comply with these terms.
*Correspondence: Honghua Liu, majorhp0207@163.com