- 1Engineering Technology Research Institute, Xinjiang Oilfield Company, PetroChina, Karamay, China
- 2College of Petroleum Engineering, China University of Petroleum, Beijing, China
- 3Baikouquan Oil Production Plant, Xinjiang Oilfield Company, PetroChina, Karamay, China
Mahu Oilfield, the largest conglomerate oilfield around the world, is facing the severe problems of rapid production decline and low recovery ratio. Re-fracturing of horizontal wells is an effective method to improve this situation. The study of horizontal well re-fracturing has increased popularity recently, but the optimization of re-fracturing parameters has not been thoroughly investigated, especially for the conglomerate reservoir. The optimal selection of re-fracturing parameters such as length and conductivity is unclear, which significantly influences re-fracturing treatment and economic cost. In order to fill this gap, a series of numerical models are established based on filed data to predict horizontal well production after re-fracturing. The parameters of primary hydraulic fractures are calculated by net pressure matching and are imported into the numerical models. A production matching is conducted to confirm the reliability of numerical models. The cumulative productions of different re-fracturing stimulation methods, including lengthening old fractures and perforating new fractures, are simulated, and the corresponding re-fracturing parameters are optimized. The results have shown that the length of primary fractures is smaller than designed, which means lengthening old fractures is necessary. Lengthening old fractures and perforating new fractures between primary clusters can increase production. On the condition of the same fracture length, the increasing ranges of production corresponding to perforating new fractures are larger than that of lengthening old fractures. Lengthening old fractures is particularly suitable to the situation that the length of primary fractures is smaller than designed, and the optimal value in lengthening half-length of old fractures is 160 m. By contrast, perforating new fractures between old clusters applies to the case that the cluster spacing of primary hydraulic fracturing is larger than 35 m, and the optimal fracture half-length is 140 m.
Introduction
For the horizontal wells of the unconventional reservoir, after a long period of production, the problems of rapid production decline and low recovery ratio happen due to the geological characteristics of low porosity, low permeability, and failure of hydraulic fractures (Zou et al., 2015; US Energy Information Administration, 2018; US Energy Information Administration, 2019; Li and Zhu, 2020). Currently, re-fracturing technology, such as restoring the conductivity of the primary fracturing fracture, increasing the fracture length, and generating new fractures, can reactivate the productivity capacity of oil and gas wells. Therefore, re-fracturing treatment has become an effective way for unconventional reservoirs to restore production and enhance oil recovery (Fleming, 1992; Potapenko et al., 2009; Wei and Tang, 2014; Tian et al., 2016; Yang et al., 2019).
Much research related to the re-fracturing technology of horizontal wells, including well selection and re-fracturing time optimization, has been conducted in the literature (Li et al., 2005; French et al., 2014; Xu J. et al., 2019). However, there are few studies and reports on the optimization of re-fracturing parameters of horizontal wells. The impacts of pressure depletion and the simultaneous production of new and old fractures after re-fracturing must be considered when the re-fracturing parameters are optimized. The conventional optimization methods for primary fracturing are no longer applicable to re-fracturing treatment. Guo et al. optimized the timing of re-fracturing for tight oil reservoir in Songliao Basin and studied the impact of different re-stimulation methods, including perforating new fractures and lengthening old fractures, on oil production. However, they did not continue to optimize the corresponding fracture parameters (Guo et al., 2019). Ren et al. optimized the re-fracturing parameters of horizontal wells under the condition of perforating new fractures in the Daqing tight reservoir. However, they did not consider the situation of lengthening old fractures (Ren et al., 2020). For tight reservoirs of Xinjiang in Northwest China, Fang et al. used the numerical simulation method to establish the productivity prediction model of re-fractured horizontal wells and comprehensively studied the impact of re-fracturing methods, fracture half-length, and fracture conductivity on production after re-fracturing. However, they did not quantify the optimal fracture parameters (Fang et al., 2017). Because the basic physical properties are highly heterogeneous, the aforementioned results based on other types of tight reservoirs are not directly applicable to the Mahu conglomerate reservoir in the Junggar Basin. Therefore, the influence of re-fracturing treatment on the oil production of the Mahu tight conglomerate reservoir and the corresponding optimal fracture parameters are still not clear. Taking a horizontal well in Ma131 Block of Mahu Oil Field as an example, the fracture parameters of the primary fracturing are firstly inversed through net pressure matching, and a numerical model is then established based on the geological model. Production history matching is conducted to verify the reliability of the established model. Considering the fracture-cluster spacing and fracture length of the primary fracturing, the re-fracturing stimulation measures and the corresponding optimal fracture parameters are determined.
The simulation results show a valuable guide to many other fields that rely on hydraulic fracturing, such as the development of geothermal and natural gas. In addition, it is helpful to use the carbon dioxide fracturing fluid to achieve carbon neutrality. Based on our optimization results, how much fluid should be injected into the well and how much liquid will be produced from the well can be calculated to make the development design. In summary, this study not only is very important for traditional oil exploitation but also has reference significance for the development of clean energy.
Reservoir Information
Description of Geological Parameters
The reservoir of Baikouquan Formation (Kuang et al., 2014; Xu T. et al., 2019; Li et al., 2020) in Ma131 Block is composed of gray sandy conglomerate, gravelly coarse sandstone, sandy conglomerate, medium-coarse conglomerate, and calcareous sandy conglomerate. The formation thickness evenly distributes with a range of 112.4–175.2 m and an average of 130.2 m. Baikouquan Formation is divided into three sections, including T1b1, T1b2, and T1b3, from bottom to top. The oil layers are mainly distributed in T1b3 and T1b2 Formation. According to the porosity and permeability obtained by core analysis, the porosity of T1b3 varies from 5.00% to 14.30%, with an average of 8.26%, and the permeability varies from 0.02 to 17.20 mD, with an average of 0.89 mD. The porosity of T1b2 varies from 5.00% to 13.90%, with an average of 7.63%, and the permeability varies from 0.02 to 19.40 mD, with an average of 1.33 mD.
Production History After Primary Fracturing
The bridge-plugging perforation combined with the staged fracturing process was employed for the primary fracturing of well MaHW1321. There are 15 stages consisting of 40 clusters, with an average interval of 38 m. The primary fracturing stimulation was completed on 7 September 2016, and the production data were being recorded from 28 October. During the initial production stage, natural flow exploitation was adopted, and the maximum daily oil production could reach 52 m3/d. After about 6 months, the production began to decline. After about 22 months of production, the well was repaired and converted to pumping. Rod pump was used, and the maximum daily oil production increased to 50 m3/d. However, about 4 months after the well was converted to pumping, the production decreased rapidly again, as shown in Figure 1.
Parameter Inversion of Primary Fractures
In order to select the optimized re-fracturing stimulation measure, the fracture parameters of the primary fracturing are required as the basis of a numerical model for predicting productivity. The difference of the maximum and minimum horizontal principal stress in the target layer is about 12–15 MPa, which is too large for creating a complex fracture network. In addition, the average minimum horizontal principal stress of the fracturing target layer is about 52.4 MPa, the elastic modulus is about 29,500 MPa, and Poisson’s ratio is 0.27. According to the formula of horizontal stress difference coefficient,
where
The horizontal stress difference coefficient reflects the possibility of forming a complex fracture network. The larger the horizontal stress difference coefficient is, the less likely the complex network will be formed. In this case, the calculated horizontal stress difference coefficient is 0.47, larger than 0.3. Zhao (2021) stated that it is difficult to create a complex fracture network when Kh is larger than 0.3. In addition, according to the observation of 17 coring wells in the Ma131 Block, natural fractures are not developed in the two main oil layers. The interpretation data of imaging logs show that a small number of drilling-induced fractures is developed in Baikouquan Formation. Microseismic monitoring results also show that the shape of primary hydraulic fractures is simple generally. During the primary fracturing in the Ma131 Block, a complex fracture network can barely be created. Because the cluster spacing of primary fractures is large (e.g., generally more than 20 m), the interference among primary fractures can be totally ignored. Based on the conditions above, the parameter inversion of primary fractures considered as single fractures is carried out to provide a data basis for the numerical model.
During the process of primary fracturing of well MaHW1321, an adjacent vertical well is selected as the monitor to place the microseismic instrument and analyze the fracture parameters, including the length and height of the first seven stages. As shown in Table 1, comparing the available microseismic monitoring results of the first seven stages with the inverted fracture parameters, the errors of fracture length and height are 19% and 20%, respectively, confirming the accuracy of the net pressure matching results. The fracture parameters of the whole well inversed by net pressure matching are shown in Table 2.
Numerical Model
Based on the commercial reservoir simulation software (CMG), the numerical model was established by combining the actual geological model and the inverted fracture parameters as inputs. As shown in Figure 2, to consider the influence of adjacent wells and avoid the problem of boundary effects, the size of the numerical model is 2 × 2 km with a grid size of 10 × 10 × 10 m. The physical properties of reservoir model parameters are shown in Table 3.
Because the flow pressure is always higher than the bubble point, the oil-water two-phase flow model is considered in the simulation. The relative permeability curves are shown in Figure 3, and the capillary pressure is shown in Figure 4.
The history production data was used to match the daily production under the construction of monitor pressure. As shown in Figure 5, the matching degree between the observed daily oil production and the simulated daily oil production is very high, which confirms the reliability of the numerical model we established.
Results and Discussion
Based on the numerical model after history match, combining the fracture cluster spacing and half-length of the primary fractures, two kinds of re-fracturing stimulation measures, including perforating new fractures between primary fractures and lengthening old fractures, are investigated. Perforating new fractures means a new open perforation will be added between two existing perforations, and a new fracture will propagate during re-fracturing. On the contrary, lengthening old fractures means fluid of re-fracturing will flow into the existing fractures. There are no new fractures that will be formed during re-fracturing. The oil production after re-fracturing is calculated to optimize fracture parameters and provide theoretical guidance for field construction.
Perforating New Fractures
According to the oil saturation and pressure distribution after history match, as shown in Figure 6, the average oil saturation is about 49%, and the reservoir pressure is about 32 MPa, which shows sufficient potential to perforate new fractures to improve the oil production.
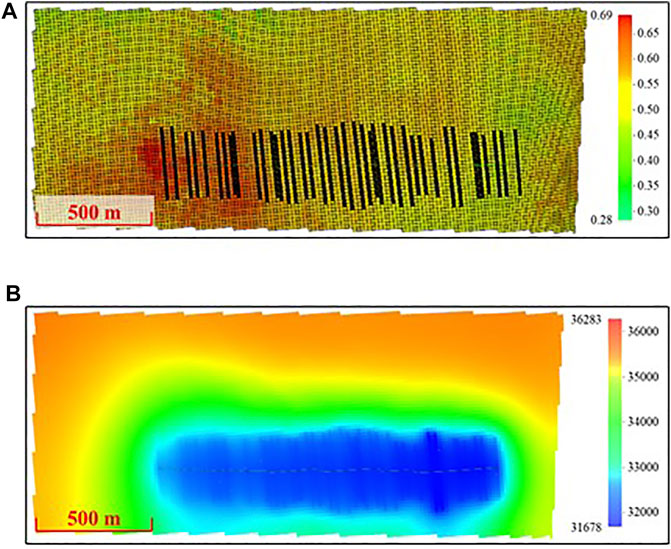
FIGURE 6. (A) Oil distribution after primary fracturing. (B) Pressure distribution after primary fracturing (KPa).
First, the cluster spacing most suitable for perforating new fractures is determined. Under the same length of horizontal section, the cluster spacing is set as 25, 35, 45, and 55 m, respectively. A new perforation is added between the two clusters to study the effect of perforating new fractures. It can be seen from Figure 7 that the cumulative oil production of the well after re-fracturing can increase compared to wells without re-fracturing under different cluster spacing. However, when the fracture cluster spacing is greater than 35 m, the cumulative oil production after re-fracturing can be greatly improved by perforating new fractures. Therefore, when the fracture clusters spacing of the primary fracturing is greater than 35 m, the positive effects of perforating new fractures on oil production are more significant.
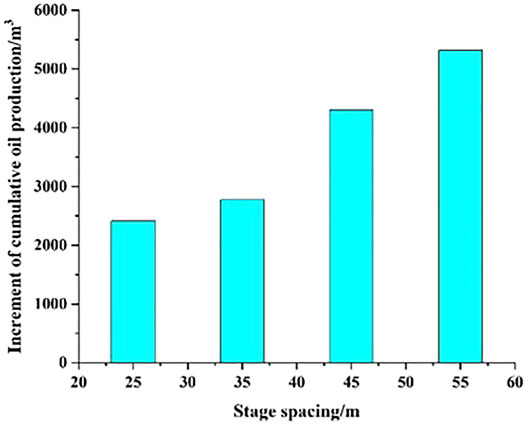
FIGURE 7. Increment of cumulative oil production after re-fracturing under different cluster spacing.
As shown in Figure 8, the cluster spacing is different between old fractures in the primary fracturing of well MaHW1321. One new fracture is added between clusters whose spacing is larger than 35 m, including clusters 1 and 2, 2 and 3, 4 and 5, 5 and 6, 11 and 12, 18 and 19, 20 and 21, 21 and 22, 26 and 27, 32 and 33, 34 and 35, and 39 and 40. A total of 12 new fractures were added for re-fracturing. In addition, four new fracture magnitudes of 100, 120, 140, and 160 m are set to optimize the half-length of the new fracture.
As shown in Figure 9, after a period of production, the measure of perforating new fractures can effectively improve the daily oil production. During the production after primary fracturing, the maximum daily oil production is 42.5 m3/d. During the initial stage of production after re-fracturing, the daily oil production returns to the same level as the maximum daily oil production before re-fracturing. For example, when the fracture half-length is 160 m, the peak of daily oil production is 40.3 m3, which is 95% of the maximum daily oil production before re-fracturing. When the fracture half-length is 100 m, the peak of daily oil production is 38.5 m3, which is 91% of the maximum value before re-fracturing.
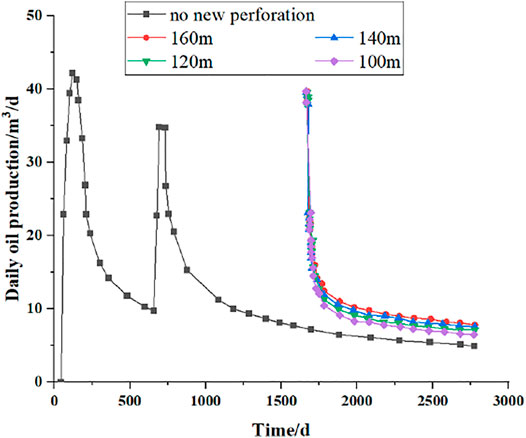
FIGURE 9. Daily oil production after perforating new fractures under different fracture half lengths.
As shown in Figures 10, 11, the cumulative oil production after re-fracturing also gradually increases as the length of new fractures increases. Without perforating new fractures, the cumulative oil production of 3,000 days is about 29800 m3. When the fracture half-length is 160 m, the cumulative oil production of 3,000 days increases to 35300 m3, with an increasing amount of 5500 m3 and an increasing ratio of 18.4%. When the fracture half-length is 100 m, the cumulative oil production at 3,000 days is 33900 m3, with an increasing amount of 4100 m3 and an increasing ratio of 13.7%. When the fracture half-length is greater than 140 m, the growth of cumulative oil production after re-fracturing slows down obviously. In this study, the most suitable half-length for perforating new fractures is 140 m.
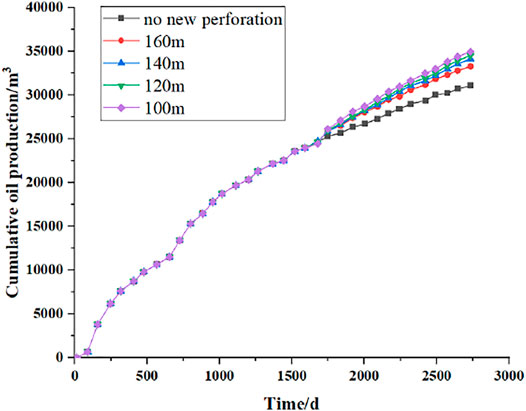
FIGURE 10. Evolution of cumulative oil production after perforating new fractures under different fracture half lengths.
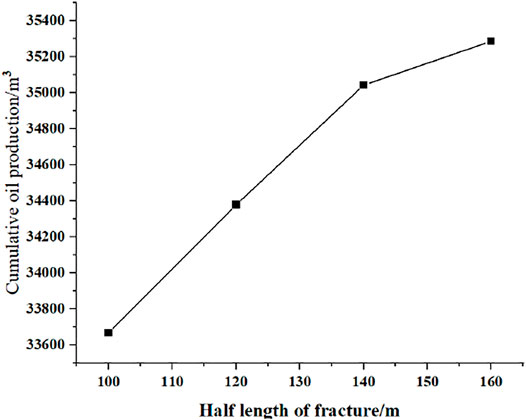
FIGURE 11. Cumulative oil production at 3,000 days after perforating new fractures under different fracture half lengths.
Lengthening Old Fractures
As shown in Figure 12, only the 22nd, 23rd, 26th, 28th, 29th, 33rd, and 34th clusters of old fractures meet the design requirements because the designed half-length of the primary fracturing is 140 m. Therefore, all old fractures are lengthened.
Lengthening the old fractures to 140, 150, 160, and 170 m, respectively, is designed to investigate the effect of re-fracturing under different lengthening magnitudes on oil production. As shown in Figure 13, with the increase of fracture lengthening magnitude, the daily oil production after re-fracturing increases correspondingly. When the half-length of the old fracture increases to 170, 160, and 140 m, the maximum daily oil production after re-fracturing reaches 32, 30.5, 28.9 m3, respectively, which are separately 79%, 75%, and 68% of the maximum daily oil production, (i.e., 40.3 m3) before re-fracturing.
Figures 14, 15 show the evolution of cumulative oil production with respect to the old fracture half-length. When the half-length of old fractures is lengthened to 170, 160, and 140 m, the final cumulative oil production at 3,000 days is about 33,300, 32,650, and 33,200 m3, respectively, which are separately 3,500, 3,400, and 2,850 m3 more than that without increasing the old fractures' length. In addition, when the lengthened half-lengths of old fractures and perforated new fractures are 160 m, the final cumulative oil production at 3,000 days of the former is lower than the latter. When the half-length increases to 160 m, the increasing ratio of production slows down, and the optimal magnitude of the lengthened old fractures is 160 m in this study.
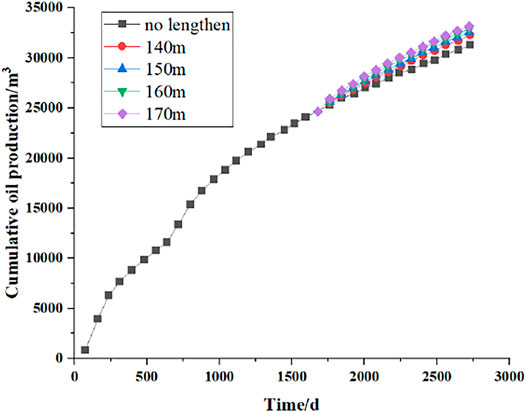
FIGURE 14. Time-serial cumulative oil production under different lengthening magnitude of old fractures.
Conclusion
1. Compared with the microseismic monitoring parameters, the error of the fracture parameters obtained by net-pressure matching is about 20%, which meets the accuracy requirement.
2. When the spacing between the primary fracture clusters is greater than 35 m, perforating new fractures is suitable for re-fracturing the wells.
3. Perforating new fractures can significantly improve the oil production after re-fracturing, and the optimal half-length of new fractures is 140 m.
4. Lengthening old fractures can improve the oil production after re-fracturing, and the optimal half-length of lengthened old fracture is 160 m.
5. At the same fracture length, the effect of perforating new fractures is more obvious than lengthening old fractures on increasing the production.
Data Availability Statement
The original contributions presented in the study are included in the article/Supplementary Material, Further inquiries can be directed to the corresponding author.
Author Contributions
YY and LW: thesis ideas and research methods. JL and SC: numerical model and results analysis. HT and GT: paper writing.
Funding
This work was supported by the Research Foundation of the CNPC Strategic Cooperation Science and Technology Project (ZLZX 2020-01-04-01). This work is supported by Foundation of Key Technologies of Mahu Conglomerate Reservoir (ZLZX2020-01-04).
Conflict of Interest
Authors YY, JL, SC, HT, GT are employed by China National Petroleum Corporation (CNPC).
The remaining author declares that the research was conducted in the absence of any commercial or financial relationships that could be construed as a potential conflict of interest.
Publisher’s Note
All claims expressed in this article are solely those of the authors and do not necessarily represent those of their affiliated organizations or those of the publisher, the editors, and the reviewers. Any product that may be evaluated in this article, or claim that may be made by its manufacturer, is not guaranteed or endorsed by the publisher.
Acknowledgments
The authors would also like to thank the Computer Modeling Group (CMG) for their simulation software.
References
Fang, P., Ran, Q., Liu, L., Ju, B., and Wang, H. (2017). Re-fracturing Mode and Fracture Parameter Optimization for Stripper Well in Tight Oil Reservoir[J]. Sci. Techn. Eng. 17 (24), 32–37. doi:10.15530/AP-URTEC-2019-198207
Fleming, M. (1992). Successful Refracturing in the North Westbrook Unit[C]. Midland, TX: Society of Petroleum Engineers.
French, S., Rodgerson, J., and Feik, C. (2014). Re-fracturing Horizontal Shale Wells: Case History of a Woodford Shale Pilot Project[C]. Woodland, TX: Society of Petroleum Engineers.
Guo, J., Tao, L., and Zeng, F. (2019). Optimization of Refracturing Timing for Horizontal Wells in Tight Oil Reservoirs: A Case Study of Cretaceous Qingshankou Formation, Songliao Basin, NE China[J]. Pet. Exploration Develop. 46 (1), 146–154. doi:10.1016/s1876-3804(19)30015-1
Kuang, L., Tang, Y., Lei, D., Wu, T., and Qu, J. (2014). Exploration of Fan-Controlled Large-Area Lithologic Oil Reservoirs of Triassic Baikouquan Formation in Slope Zone of Mahu Depression in Junggar Basin [J]. China Pet. Exploration 019 (006), 14–23. doi:10.3969/j.issn.1672-7703.2014.06.002
Li, G., Qin, J., Xian, C., Fan, X., Zhang, J., and Ding, Y. (2020). Theoretical Understandings, Key Technologies and Practices of Tight Conglomerate Oilfield Efficient Development: A Case Study of the Mahu Oilfield, Junggar Basin, NW China[J]. Pet. Exploration Develop. 47 (6), 1185–1197. doi:10.1016/s1876-3804(20)60135-0
Li, G., and Zhu, K. (2020). Progress, Challenges and Key Issues of Unconventional Oil and Gas Development of CNPC[J]. China Pet. Exploration 25 (2), 1–13. doi:10.3969/j.issn.1672-7703.2020.02.001
Li, Y., Yao, F., Weng, D., Yin, X., Yu, Y., and Jiang, X. (2005). Development and Prediction of Re-frac Technology[J]. J. Oil Gas Technology(J JPI) s5, 789–791.
Potapenko, D., Tinkham, S., Lecerf, B., Fredd, C., Samuelson, M., and Gillard, M. (2009). Barnett Shale Refracture Stimulations Using a Novel Diversion Technique[C]. Woodland, TX: Society of Petroleum Engineers.
Ren, J., Wang, X., Zhang, X., and Wang, W. (2020). Refracturing and Fracture Parameters Optimization Simulation for Horizontal Well in Daqing Tight Oil Reservoir[J]. Fault- Block Oil & Gas Field 27 (5), 638–642. doi:10.6056/dkyqt202005018
Tian, H., Lv, J., Li, W., Yang, J., Guo, X., Sun, N., et al. (2016). Main Practice of Reducing Drilling and Completion Cost in North America under Low Oil Price and its Enlightenment[J]. Int. Pet. Econ. 24 (9), 36–43. doi:10.3969/j.issn.1004-7298.2016.09.006
US Energy Information Administration (2019). Annual Energy Outlook 2019 with Projections to 2050[R]. Washington: US Energy Information Administration.
US Energy Information Administration (2018). International Energy Outlook 2018[R]. Washington: US Energy Information Administration.
Wei, X., and Tang, J. (2014). Technical Current Status and Development Direction of Horizontal Well Staged Fracturing Technology[J]. Pet. Geology. Oilfield Develop. Daqing 33 (06), 104–111. doi:10.3969/J.ISSN.1000-3754.2014.06.020
Xu, J., Li, J., Wu, Y., Ding, K., and Jiang, H. (2019). Exploration and Practice of Volume Fracturing Technology in Horizontal Well of Mahu Tight Conglomerate Reservoirs [J]. China Pet. Exploration 024 (002), 241–249. doi:10.3969/j.issn.1672-7703.2019.02.013
Xu, T., Lindsay, G., Zheng, W., Baihly, J., Ejofodomi, E., Malpani, R., et al. (2019). Proposed Refracturing-Modeling Methodology in the Haynesville Shale, a US Unconventional Basin[C]. San Antonio, TX: Society of Petroleum Engineers
Yang, Z., Li, Y., Li, X., and Li, C. (2019). Key Technology Progress and Enlightenment in Refracturing of Shale Gas Horizontal Wells[J]. J. Southwest Pet. Univ. (Science Techn. Edition) 41 (6), 75–86. doi:10.11885/j.issn.1674-5086.2019.10.15.05
Zhao, X. (2021). Evaluation Method of Shale Reservoirs Fracability Based on Logging Data and its Application[J]. Complex Hydrocarbon Reservoirs 14 (02), 32–35. doi:10.16181/j.cnki.fzyqc.2021.02.006
Keywords: re-fracturing, parameters optimization, stimulation method, lengthening old fractures, perforating new fractures
Citation: Yi Y, Wang L, Li J, Chen S, Tian H and Tian G (2022) Optimization of Re-Fracturing Method and Fracture Parameters for Horizontal Well in Mahu Conglomerate Oil Reservoir. Front. Energy Res. 10:856524. doi: 10.3389/fenrg.2022.856524
Received: 17 January 2022; Accepted: 14 March 2022;
Published: 11 April 2022.
Edited by:
Xun Zhong, Yangtze University, ChinaReviewed by:
Hun Lin, Chongqing University of Science and Technology, ChinaTiankui Guo, China University of Petroleum Huadong, China
Copyright © 2022 Yi, Wang, Li, Chen, Tian and Tian. This is an open-access article distributed under the terms of the Creative Commons Attribution License (CC BY). The use, distribution or reproduction in other forums is permitted, provided the original author(s) and the copyright owner(s) are credited and that the original publication in this journal is cited, in accordance with accepted academic practice. No use, distribution or reproduction is permitted which does not comply with these terms.
*Correspondence: Lei Wang, bGVnZW5kLXdsQDE2My5jb20=