- 1Department of Mechanical Engineering, College of Engineering, Landmark University, Omu Aran, Nigeria
- 2Department of Agricultural and Biosystems Engineering, College of Engineering, Landmark University, Omu Aran, Nigeria
Energy need for cooking in both the rural and urban areas all over the world is increasing every day as a result of an increase in population. The consequence of global warming due to the usage of fuels such as fossil fuel, firewood, and other biomass products for cooking necessitates innovative techniques that will improve the standard of living of people. In this study, the design, fabrication, and thermal evaluation of a solar cooking system integrated with an Arduino-based tracking device and sensible heat storage (SHS) materials was investigated. During the water boiling trials with black oil sensible material (BOSHSM), the obtained maximum temperatures for water, cooking box, and sensible heat storage material at 14:00 h when the solar radiation attained its peak value of 881.2 W/m2 were 64,52, and 54°C, respectively, while at 14:00 h with Black coated granite sensible heat storage material (BCGSHSM) at the solar radiation peak value of 890.4 W/m2, the maximum temperatures for water, cooking box, and sensible heat storage material were 73.5, 76, and 59°C, respectively. The maximum cooking power and thermal efficiency obtained from the water boiling trials were 48.4 and 56.4 W, and 31.6 and 35.8% respectively. Also, the results from the cooking of edibles revealed that the cooking power values ranged between 42.5 and 58.2, while that of efficiency ranged between 34.5 and 40.3% respectively. The maximum solar radiation during the cooking trial period was 986, 975, 956, and 953 W/m2. In general, from the results, the developed solar cooking system is a viable alternative to cooking with traditional/open burning of wood or other biomass products that pose a serious environmental and health-related threat to the people living in developing countries.
1 Introduction
Cooking is one of the major domestic activities that require energy for human sustenance. All over the world, energy for cooking is being sourced for using different types of cooking fuels such as fossil fuels, firewood, charcoal, etc. are in use. Due to the increase in the cost of cooking gas, fossil fuel, and also the epileptic nature of power supplies in developing countries, the majority of people in the rural and urban areas still use firewood for cooking (Komolafe and Awogbemi, 2010). Burning of firewood, charcoal, or crop residue, slash and burn agriculture, and deforestation account for 5–20% of all carbon emissions worldwide (Smith et al., 1993). Energy experts all over the world have recently become more conscious of the adverse effects of global warming as a result of the usage of non-environmental and eco-friendly fuels such as fossil fuel, firewood, charcoal, etc. Harnessing the abundant potentials in the freely given renewable energy source (Sun) which is clean, safe, and readily available for cooking has become a burning issue and most attractive alternative. History made it clear that the idea of using solar energy for cooking started in 1,650 as a result of fuel shortages and rationing arising from the second world war (Wentzel and Pouris, 2007). Using solar cooking would not only eliminate or at least reduce the respiratory illness arising from the exposure to smoke but could also be used to defeat another scourge of the developing world, contaminated water (Tucker, 1999). Solar cookers are classified broadly into two categories, the box, and the concentrating type. The box-type permits solar radiation directly through the glass window for cooking while the concentrating type uses parabolic or spherical, linear fresnel reflectors and a central receiver tower with the cooking pot positioned at the focal point where the Sun rays are focussed (Kumaresan et al., 2016; Noman et al., 2019).
Several studies on various configurations of the box and concentrating types of solar cookers have been reported (Kumar et al., 2008; Akoy and Ahmed, 2015; Hafez et al., 2016; Noman et al., 2019). However, in an attempt to increase the performance of solar cooking systems, researchers have suggested the use of thermal storage materials (sensible, latent heat, and thermochemical). Sensible and latent thermal energy storage has become a critical feature of energy management, with prominence in the effective use and reuse of waste heat and solar energy not only in manufacturing and buildings but also for cooking (Goldstein et al., 2006). The use of thermal energy storage is crucial whenever there is a mismatch between the supply and demand for energy. Based on the cost of the three identified types of thermal storage materials, sensible thermal storage materials is cheaper and readily available for people in rural areas and some urban areas of developing countries like Nigeria. Table 1 presents the summary of studies on solar cooker with thermal storage materials (Hussein et al., 2008; Saxena et al., 2013a; Saxena et al., 2013b; Kumaresan et al., 2016; Saxena and Karakilcik, 2017; Yadav et al., 2017; Bhave and Thakare, 2018; Coccia et al., 2018; Saxena and Agarwal, 2018; Keith et al., 2019; Khallaf et al., 2020; Omara et al., 2020; Saxena et al., 2020; Hosseinzadeh et al., 2021; Kanyowa et al., 2021). Some authors have reported integration of tracking device (Sun tracker) (Roth et al., 2005; Regin et al., 2008; Skouri et al., 2016; Herez et al., 2018; Babu et al., 2019). (Roth et al., 2005; Regin et al., 2008; Skouri et al., 2016; Herez et al., 2018; Babu et al., 2019). However, heating of the cooking pot/vessel through the parabolic dish and cooking box at the same time has not been reported.
In this study, therefore, design, fabrication, and thermal evaluation of a solar cooking system integrated with a photovoltaic controlled Arduino-based data logging cum tracking device and sensible heat storage materials (Black coated granite and used engine oil) for both the rural and urban areas are presented.
2 Materials and Methods
2.1 Material Selection
Locally sourced and eco-friendly materials such as aluminum sheet, angle iron, mild steel pipes, plywood, reflective glass, Rockwool, aluminum foil, etc. were selected for the fabrication solar cooking system based on design consideration, preliminary investigation, etc. Also, black coated granite and used black engine oil (black) were used as sensible heat storage materials. Fresh and clean samples of sweet potato and plantain purchased from the neighbouring market were used for the performance evaluation of the cooking system. The measuring instruments and thermophysical properties of SHS are shown in Tables 2–4 respectively.
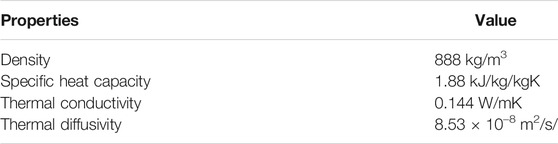
TABLE 3. Thermo-physical properties of engine oil (Sharma et al., 2009).
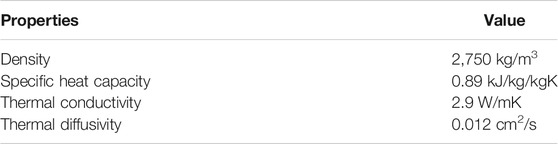
TABLE 4. Thermo-physical properties of granite (Bejan and Kraus, 2003).
2.2 Description of the Parabolic Solar Cooker
The experimental set-up of the fabricated solar cooking system is presented schematically and pictorially as shown in Figures 1, 2 respectively. It comprises mainly four components namely: the parabolic solar reflector, cooking box, data acquisition and tracking device and supporting frame.
(a) Parabolic reflector: The reflector was fabricated from an aluminum sheet. It is 110 mm in diameter and 150 mm deep. It was cut and glued to the parabolic reflector’s surface.
(b) Cooking box: The cooking box has an overall dimension of 1,376 × 1203 × 1,203 mm. It has three of its sides made of wood (lagged with Rockwool for insulation) and the other three reflective glass. The three glasses were located at the top, bottom, and one of the sides of the box to allow the Sun rays into the cooking pot. Inside the cooking box, were a cooking pot made of aluminum sheet and a compartment for heat storage material as shown in Figure 3.
(c) Data acquisition and tracking device: The data acquisition and tracking device consists of eight major components namely; micro-controller, Bluetooth module, SD card, real-time clock, temperature sensors (DS18B20), Humidity sensor (DHT22), battery, solar panel, and stepper motor.
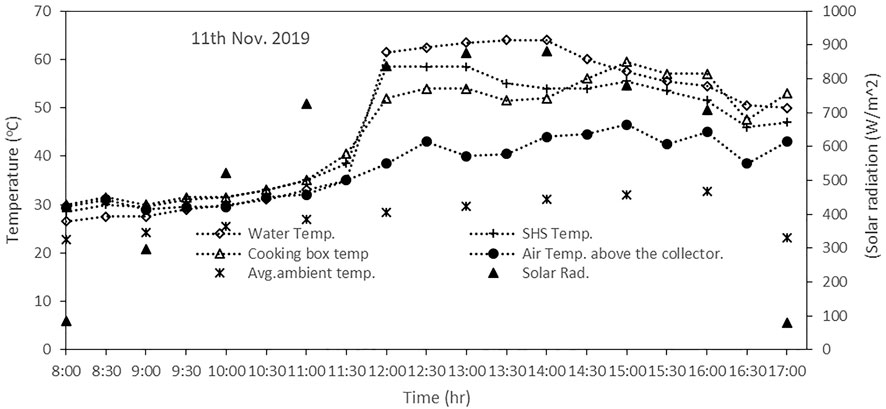
FIGURE 3. The plot of solar radiation and the temperature of the water, cooking box, SHS material, and air against time.
2.3 Experimental Procedure
Water boiling and cooking tests were carried out in November 2019 between 8:00 and 17:00 h to evaluate the performance of the tracking device integrated cooking system using black-coated gravel and used engine oil. The experimentation site was Teaching and Research Farm, Landmark University, Omu Aran, Nigeria which is located at latitude 8.8oN, longitude 5.5oE. Prior to the cooking tests, water boiling trials were conducted in order to ascertain the thermal response of the system with SHS materials. Thereafter, two edibles (Rice and plantain) were used for the cooking tests. The time, date, relative humidity and temperature of water in the pot and other locations within the cooking system data were programmed to be collected by the micro-controller (Atmeg) from the real-time clock chip (DS1307) and stores it in the SD card at intervals of 10 min. The stored temperature solar radiation, data were retrieved from the solar cooker via the Bluetooth connection without any physical contact with the SD card or device. Data for solar radiation, relative humidity, wind speed and ambient temperature were also monitored and recorded for comparison with readings from the installed Campbell Scientific Ltd. made metrological station at Landmark University Teaching and Research Farm which is just a few centimetres away from where the solar cooking system was positioned. The solar tracking device tracks the Sun with the help of two light sensors and a stepper motor. The two LDR (Light dependent renters) were used to monitor the light rays at opposite sides of the parabolic dish. The microcontroller was also configured and programmed to monitor the direction of light rays and to move the parabolic collector with the aid of a stepper motor towards the direction of the sensor with higher light rays. However, when the light ray sensors on both sides sense an equal ray of light, the stepper motor adjusts and position the parabolic reflector at the centre. Specification of measuring device is shown in Table 1.
2.4 Theory and Analysis
2.4.1 Parabolic Concentrator
Various components of the solar cooking system were designed using the following parameters (Stine and Diver, 1994; Sup et al., 2015; Hafez et al., 2016; González-Avilés et al., 2018; Babu et al., 2019; Noman et al., 2019; Ahmed et al., 2020).
Parabolic concentrator’s aperture area.
The concentrator aperture area, which according to Affandi et al. (Affandi et al., 2014) is defined as the area that receives the solar radiation is given by (Hafez et al., 2016; Yahuza et al., 2016)
Receiver’s aperture area.
The aperture area of the receiver is given by Eq. 2 (Hafez et al., 2016) as:
Area concentration ratio.
The area concentration ratio is defined as the ratio of concentrator aperture area to the receiver aperture area (Affandi et al., 2014)
Parabolic dish surface area.
The surface area of the parabolic dish can be determined by Eq. 4 (El Ouederni et al., 2009)
Parabolic dish focal length.
The focal length of the focal point from the parabolic dish concentrator can be expressed as Eqs 5, 6 (Hafez et al., 2016)
Rim angle.
The rim angle according to Stine (Stine and Diver, 1994), is the angle measured at the focus from the axis to the rim of the solar parabolic truncated. It can be determined using [7]
2.4.2 Design Theory for Solar Radiation
Total solar radiation on tilted surface.
The total incident solar radiation on the tilted surface
The instantaneous beam radiation on the surface per unit area can be calculated as:
The angle of incidence
where
The surface diffuse radiation
The ground reflected solar radiation can be calculated by multiplying the total solar radiation from the total solar incident on the horizontal surface by the ground reflectance
2.4.3 Thermal Modelling
The available energy is absorbed by the cooking fluid while the unavailable is lost to the surrounding by convection and radiation. The energy balance equations for various components of the cooking system namely; the parabolic reflector, Vessel (cooking pot), vessel fluid, energy storage material, cooking box glass cover and the enclosed air cover were written (Mbodji and Hajji, 2017; Yettou et al., 2019; Khallaf et al., 2020; Bhavani et al., 2021). The following assumptions were made:
a. Thermo-physical properties of air, glass, and reflector/absorber remain constant within the cooker temperature range.
b. Proper thermal contact between cooking pot and reflector/absorber surface of the cooker.
c. Negligible heat transfer by reflection between the sidewalls and cooking pot.
d. Exchange of heat as a result of air within the lid covered pot not considered.
Energy balance for the parabolic reflector:
Energy balance for the vessel (cooking pot):
Energy balance for fluid in the vessel:
Energy balance for the storage material:
Energy balance for the cooking box glass cover
Energy balance for the air inside the cooking box
where
Substituting the heat transfer flux, the energy balance model Eqs 13–18 can be written as follows:
- For glass cover
- For the air inside the cooking box
- For Parabolic reflector:
- For the vessel (cooking pot):
- For the vessel fluid:
- For the storage material
The coefficient of heat transfer for cooker can be expressed as (Duffie et al., 2020):
Assume the side losses are negligible, the overall heat loss can be obtained as (Channiwala and Doshi, 1989):
2.4.4 Standard Cooking Power
The amount of heat that enters the container of the solar cooking system to raise the temperature of a given quantity of water in a certain time interval is known as standard cooking power
where m is the mass of water (kg);
2.4.5 Thermal Efficiency
Thermal efficiency is obtained as (Komolafe and Waheed, 2018; Komolafe et al., 2019; Komolafe et al., 2021):
2.5 Uncertainty Analysis
Implementation of the uncertainty analysis is necessary in order to investigate the reliability of the results. The total uncertainty for a measured parameter (P) can be calculated using the following equation (Zamani et al., 2015; Hosseinzadeh et al., 2020): Some measured parameters are shown in Table 5.
where
The uncertainty function
3 Results and Discussion
Figure 3 shows the plot of solar radiation and the temperature of the water, cooking box, sensible heat storage material, and air against time with used engine oil as sensible heat storage material. It is evident from the figure that the temperature of the water and that of other locations within the cooking system were almost the same in the early hour of the experiment and began to vary from 11:00 h. The observed temperature profile follows the same pattern with the solar radiation. Thus, an increase in solar radiation resulted in to increase in temperature. The obtained maximum temperatures for water, cooking box, and sensible heat storage material at 14:00 h when the solar radiation attained its peak value of 881.2 W/m2 were 64,52, and 54°C, respectively. The figure further revealed that at 17:00 h when the lowest solar radiation value of 80 W/m2 was attained, the temperatures of water and the cooking box were 50 and 53°C, respectively. This could be attributed to the incorporation of insulating and heat storage materials into the cooking box.
The variation of relative humidity and the temperature of the water, cooking box, sensible heat storage material, and air against time with used engine oil (black) as heat storage material is shown in Figure 4. It can be seen that the relative humidity decreased with time as the temperatures increased. The relative humidity minimum and maximum values of 57.2 and 88.6% were attained at 15:00 and 17:00 h respectively. When compared with Figure 3, solar radiation and temperatures decreased as the relative humidity increased and vice versa.
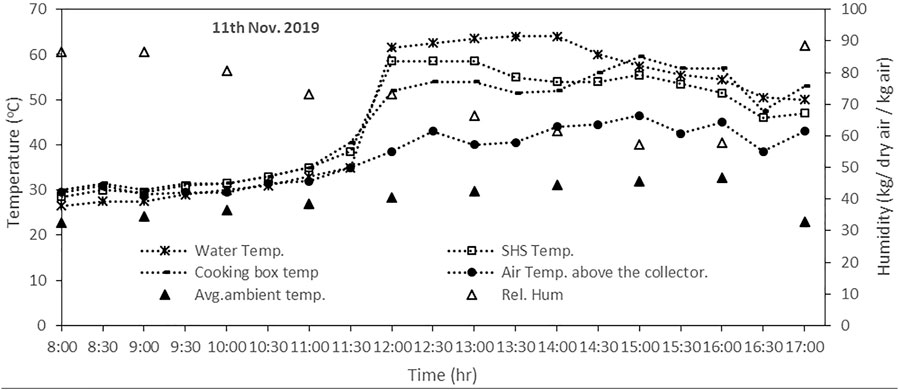
FIGURE 4. The plot of humidity and the temperature of the water, cooking box, SHS material, and air against time.
Figure 5 depicts the variation of solar radiation and the temperature of the water, cooking box, sensible heat storage material, and air with time. From the graph, it is evident that the temperature profile follows the same pattern with the solar radiation in a similar manner to Figure 3. However, the maximum temperatures for water, cooking box, and sensible heat storage material at 14:00 h when the solar radiation attained its peak value of 890.4 W/m2 were 73.5, 76, and 59°C, respectively. The difference in these temperature values and those reported in Figure 3 could be attributed to the irregular intensity of solar radiation or the thermal conductivity of the sensible heat storage materials used. Similar reports have been presented by (Komolafe and Waheed, 2018).
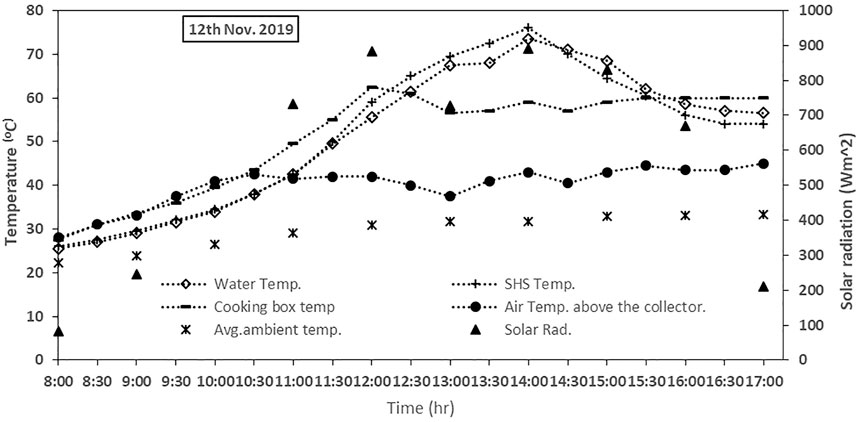
FIGURE 5. The plot of solar radiation and the temperature of the water, cooking box, SHS material, and air against time.
Figure 6 presents the plot of humidity and the temperature of the water, cooking box, sensible heat storage material, and air against time using black coated gravel as heat storage material. It can be seen that the relative humidity decreased with time as the temperatures increased. The relative humidity minimum and maximum values of 49.8 and 94.6% were attained at 17:00 and 8:00 h respectively. When compared with Figure 5, solar radiation and temperatures decreased as the relative humidity increased and vice versa.
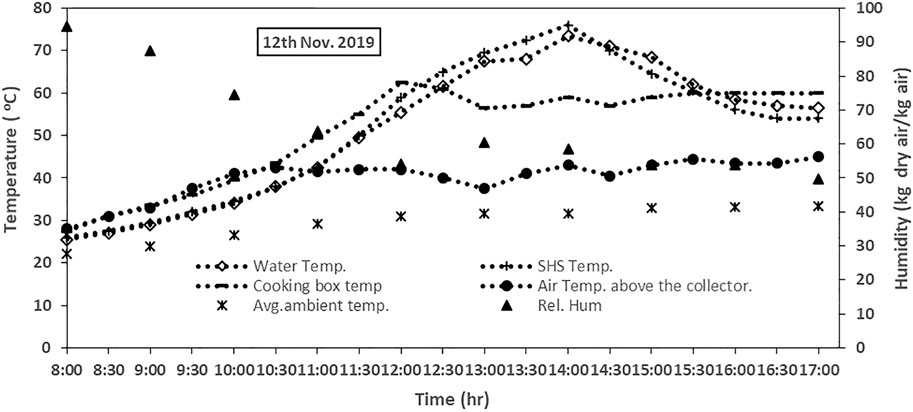
FIGURE 6. The plot of humidity and the temperature of the water, cooking box, SHS material, and air against time.
Figure 7 represents the plots of temperature and solar radiation versus cooking duration of rice using black coated granite as SHS material. With water as cooking fluid, 0.8 kg of rice was boiled between 18 and 20th of November 2019. Each experiment was conducted between 10:30 and 13:30 h. From the graph, the average minimum and maximum temperature of water and ambient which occurred at 10: 30 and 13:00 h were 49.7 and 102°C respectively. The average solar radiation at these periods were 600 and 986 W/m2 respectively.
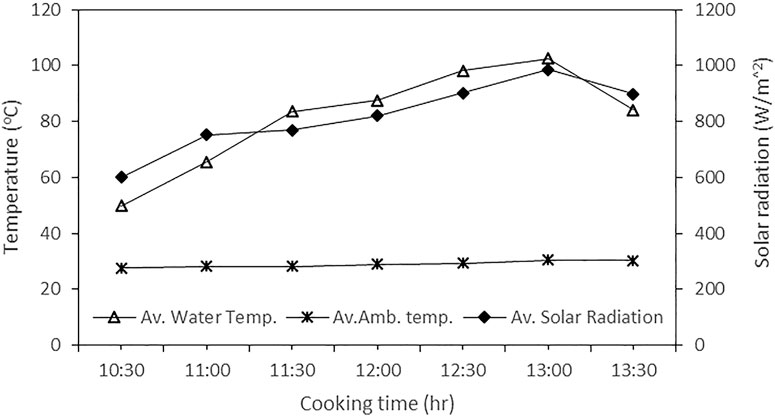
FIGURE 7. The graph of temperature and solar radiation versus cooking duration of rice using granite as SHS.
Figure 8 shows the plots of temperature and solar radiation versus cooking duration of rice using as granite as SHS material. With water as cooking fluid, 0.8 kg of rice was boiled between 21st–23rd of November 2019. Each experiment commenced at 10:30 h and terminated at 13:30 h. It was observed that the average minimum and maximum temperature of water and ambient which occurred at 10: 30 and 13:00 h were 55.5 and 101.5°C respectively, while the average solar radiation at these periods were 680 and 975 W/m2 respectively.
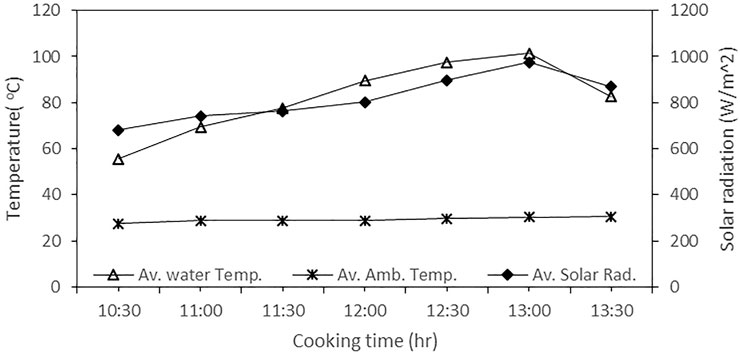
FIGURE 8. The graph of temperature and solar radiation versus cooking duration of rice using engine oil as SHS material.
Figure 9 reveals the plots of temperature and solar radiation versus cooking duration of rice using black engine oil as SHS material. With water as cooking fluid, 0.95 kg of plantain was boiled between 24th–26th of November 2019. Each experiment was conducted between 10:30 and 13:30 h. It was observed that the average minimum and maximum temperature of water and ambient which occurred at 10:30 and 13:00 h were 54 and 100.8°C respectively, while the average solar radiation at these periods were 635 and 956 W/m2 respectively.
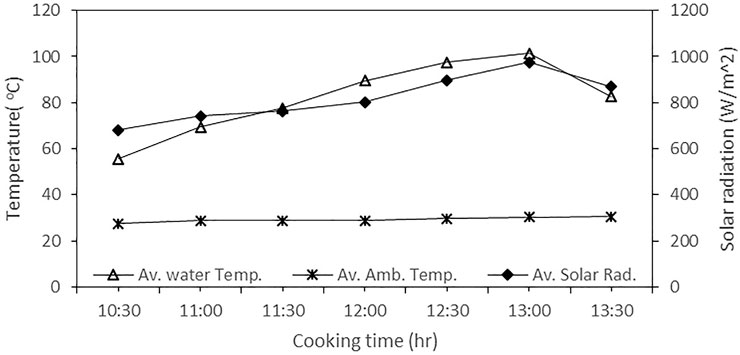
FIGURE 9. The graph of temperature and solar radiation versus cooking duration Plantain using engine oil as SHS material.
Figure 10 depicts the plots of temperature and solar radiation versus cooking duration of rice using black engine oil as SHS material. With water as cooking fluid, 0.95 kg of plantain was boiled between 27th–30th of November 2019. Each experiment was conducted between 10:30 and 13:30 h. It was observed that the average minimum and maximum temperature of water and ambient which occurred at 10:30 and 13:00 h were 51.5 and 99.5°C respectively, while the average solar radiation at these periods were 635 and 953 W/m2 respectively. Generally from the graphs, it can be seen that the increase in solar radiation resulted to increase in temperature.
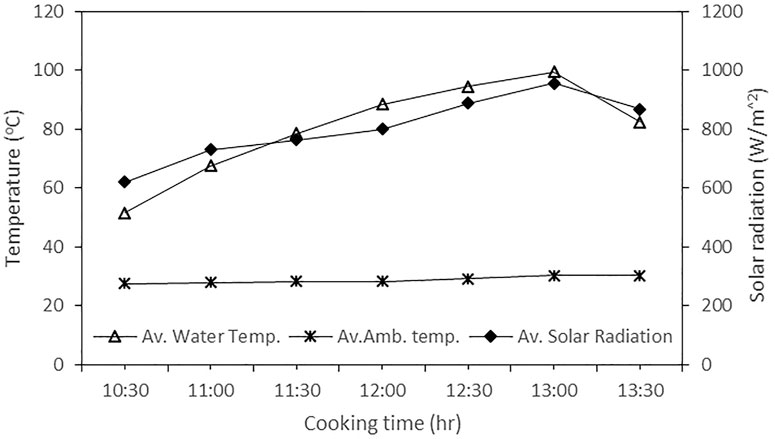
FIGURE 10. The graph of temperature and solar radiation versus cooking duration of plantain using engine oil as SHS material.
3.1 Cooking Duration
The cooking time, heat capacity, and efficiency obtained from cooking trials are shown in Table 6.
3.2 Cooking Power and Thermal Efficiency
The maximum cooking power and thermal efficiency for the water boiling tests under solar cooking system integrated with both black engine oil and black coated gravel were 48.4 and 56.4 W, and 31.6 and 35.8% respectively. However, for the edibles cooking, the cooking power values ranged between 42.5 and 58.2, while that of efficiency ranged between 34.5 and 40.3% respectively.
4 Conclusion
In the current study, the design, fabrication, and thermal evaluation of a solar cooking system integrated with tracking device and sensible heat storage materials (granite and engine oil) has been presented. The objective was to address majorly health challenge that is predominant among the people living in the rural area who in most cases use firewood and other biomass product for cooking. Locally sourced materials were used to fabricate the cooker. Thereafter, water boiling and cooking trials were adopted to evaluate the performance of the cooking system. From the results, the following major conclusions were drawn:
1. The obtained maximum solar radiation and water temperature during the water boiling tests with black engine oil as sensible heat storage material at 14:00 h were 881.2 W/m2 and 64 respectively, while with black coated gravel at this period were 890.4 W/m2 and 73.5°C.
2. The average cooking duration of rice and plantain with cooking system ranged between 144 and 151 min using black coated granite and black engine oil SHS materials respectively.
3. The maximum cooking power and thermal efficiency obtained from the cooking trials of rice and plantain were 58.2 W and 40.3% respectively.
4. The developed cooker is expected to perform better when the solar intensity is higher
5. Adoption of the developed solar cooking system will reduce environmental pollution that occur when firewood, fossil fuel etc. are used.
6. With minor design modifications, there will be an improvement on the performance of the developed cooking system.
Data Availability Statement
The original contributions presented in the study are included in the article/Supplementary Material, further inquiries can be directed to the corresponding author.
Author Contributions
CK: Conceived and design the experiment, performed the experiment, analysed and interpreted the data, wrote the paper. CO: Performed the experiment, wrote the paper.
Conflict of Interest
The authors declare that the research was conducted in the absence of any commercial or financial relationships that could be construed as a potential conflict of interest.
Publisher’s Note
All claims expressed in this article are solely those of the authors and do not necessarily represent those of their affiliated organizations, or those of the publisher, the editors and the reviewers. Any product that may be evaluated in this article, or claim that may be made by its manufacturer, is not guaranteed or endorsed by the publisher.
References
Affandi, R., Gan, C. K., Ruddin, M., and ghani, A. (2014). Development of Design Parameters for the Concentrator of Parabolic Dish (Pd) Based Concentrating Solar Power (Csp) under malaysia Environment. J. Appl. Sci. Agric. 9, 42–48.
Ahmed, S. M. M., Al-Amin, M. R., Ahammed, S., Ahmed, F., Saleque, A. M., and Abdur Rahman, M. (2020). Design, Construction and Testing of Parabolic Solar Cooker for Rural Households and Refugee Camp. Solar Energy 205, 230–240. doi:10.1016/j.solener.2020.05.007
Akoy, E. O., and Ahmed, A. I. (2015). Design, Construction and Performance Evaluation of Solar Cookers. J. Agric. Sci. Eng. 1, 75–82.
Babu, M., Raj, S. S., and Valan Arasu, A. (2019). Experimental Analysis on Linear Fresnel Reflector Solar Concentrating Hot Water System with Varying Width Reflectors. Case Stud. Therm. Eng. 14, 100444. doi:10.1016/j.csite.2019.100444
Bhavani, S., Shanmugan, S., Chithambaram, V., Essa, F. A. E., Kabeel, A.-E., and Selvaraju, P. (2021). Simulation Study on thermal Performance of a Solar Box Cooker Using Nanocomposite for Natural Food Invention. Environ. Sci. Pollut. Res. 1, 1–19. doi:10.1007/s11356-021-14194-w
Bhave, A. G., and Thakare, K. A. (2018). Development of a Solar thermal Storage Cum Cooking Device Using Salt Hydrate. Solar Energy 171, 784–789. doi:10.1016/j.solener.2018.07.018
Channiwala, S. A., and Doshi, N. I. (1989). Heat Loss Coefficients for Box-type Solar Cookers. Solar Energy 42, 495–501. doi:10.1016/0038-092x(89)90050-9
Coccia, G., Di Nicola, G., Tomassetti, S., Pierantozzi, M., Chieruzzi, M., and Torre, L. (2018). Experimental Validation of a High-Temperature Solar Box Cooker with a Solar-Salt-Based Thermal Storage Unit. Solar Energy 170, 1016–1025. doi:10.1016/j.solener.2018.06.021
Duffie, J. A., Beckman, W. A., and Blair, N. (2020). Solar Engineering of thermal Processes, Photovoltaics and Wind. John Wiley & Sons.
El Ouederni, A., Salah, M. B., Askri, F., Nasrallah, M. B., and Aloui, F. (2009). Experimental Study of a Parabolic Solar Concentrator. Revue des Energies Renouvelables 12, 395–404.
Goldstein, R. J., Ibele, W. E., Patankar, S. V., Simon, T. W., Kuehn, T. H., Strykowski, P. J., et al. (2006). Heat Transfer-A Review of 2003 Literature. Int. J. Heat Mass Transfer 49, 451–534. doi:10.1016/j.ijheatmasstransfer.2005.11.001
González-Avilés, M., Urrieta, O. R., Ruiz, I., and Cerutti, O. M. (2018). Design, Manufacturing, thermal Characterization of a Solar Cooker with Compound Parabolic Concentrator and Assessment of an Integrated Stove Use Monitoring Mechanism. Energ. Sustainable Development 45, 135–141.
Goswami, D. Y., Kreith, F., and Kreider, J. F. (2000). Principles of Solar Engineering. Phildelphia, PA: CRC Press.
Hafez, A. Z., Soliman, A., El-Metwally, K. A., and Ismail, I. M. (2016). Solar Parabolic Dish Stirling Engine System Design, Simulation, and thermal Analysis. Energ. Convers. Manag. 126, 60–75. doi:10.1016/j.enconman.2016.07.067
Herez, A., Ramadan, M., and Khaled, M. (2018). Review on Solar Cooker Systems: Economic and Environmental Study for Different Lebanese Scenarios. Renew. Sustainable Energ. Rev. 81, 421–432. doi:10.1016/j.rser.2017.08.021
Hosseinzadeh, M., Faezian, A., Mirzababaee, S. M., and Zamani, H. (2020). Parametric Analysis and Optimization of a Portable Evacuated Tube Solar Cooker. Energy 194, 116816. doi:10.1016/j.energy.2019.116816
Hosseinzadeh, M., Sadeghirad, R., Zamani, H., Kianifar, A., and Mirzababaee, S. M. (2021). The Performance Improvement of an Indirect Solar Cooker Using Multi-Walled Carbon Nanotube-Oil Nanofluid: An Experimental Study with Thermodynamic Analysis. Renew. Energ. 165, 14–24. doi:10.1016/j.renene.2020.10.078
Hussein, H. M. S., El-Ghetany, H. H., and Nada, S. A. (2008). Experimental Investigation of Novel Indirect Solar Cooker with Indoor PCM thermal Storage and Cooking Unit. Energ. Convers. Manag. 49, 2237–2246. doi:10.1016/j.enconman.2008.01.026
Kanyowa, T., Victor Nyakujara, G., Ndala, E., and Das, S. (2021). Performance Analysis of Scheffler Dish Type Solar thermal Cooking System Cooking 6000 Meals Per Day. Solar Energy 218, 563–570. doi:10.1016/j.solener.2021.03.019
Keith, A., Brown, N. J., and Zhou, J. L. (2019). The Feasibility of a Collapsible Parabolic Solar Cooker Incorporating Phase Change Materials. Renew. Energ. Focus 30, 58–70. doi:10.1016/j.ref.2019.03.005
Khallaf, A. M., Tawfik, M. A., El-Sebaii, A. A., and Sagade, A. A. (2020). Mathematical Modeling and Experimental Validation of the thermal Performance of a Novel Design Solar Cooker. Solar Energy 207, 40–50. doi:10.1016/j.solener.2020.06.069
Komolafe, C. A., Oluwaleye, I. O., Awogbemi, O., and Osueke, C. O. (2019). Experimental Investigation and thermal Analysis of Solar Air Heater Having Rectangular Rib Roughness on the Absorber Plate. Case Stud. Therm. Eng. 14, 100442. doi:10.1016/j.csite.2019.100442
Komolafe, C. A., and Waheed, M. A. (2018). Design and Fabrication of a Forced Convection Solar Dryer Integrated with Heat Storage Materials. ACSM 1, 23–39. doi:10.3166/acsm.42.22-39
Komolafe, C. A., Waheed, M. A., Kuye, S. I., Adewumi, B. A., and Daniel Adejumo, A. O. (2021). Thermodynamic Analysis of Forced Convective Solar Drying of cocoa with Black Coated Sensible thermal Storage Material. Case Stud. Therm. Eng. 26, 101140. doi:10.1016/j.csite.2021.101140
Komolafe, C., and Awogbemi, O. (2010). Fabrication and Performance Evaluation of an Improved Charcoal Cooking Stove. Pac. J. Sci. Technology 11, 51–58.
Kumar, N., Agravat, S., Chavda, T., and Mistry, H. N. (2008). Design and Development of Efficient Multipurpose Domestic Solar Cookers/dryers. Renew. Energ. 33, 2207–2211. doi:10.1016/j.renene.2008.01.010
Kumaresan, G., Vigneswaran, V. S., Esakkimuthu, S., and Velraj, R. (2016). Performance Assessment of a Solar Domestic Cooking Unit Integrated with thermal Energy Storage System. J. Energ. Storage 6, 70–79. doi:10.1016/j.est.2016.03.002
Mbodji, N., and Hajji, A. (2017). Modeling, Testing, and Parametric Analysis of a Parabolic Solar Cooking System with Heat Storage for Indoor Cooking. Energ. Sustainability Soc. 7, 1–16. doi:10.1186/s13705-017-0134-z
Noman, M., Wasim, A., Ali, M., Jahanzaib, M., Hussain, S., Ali, H. M. K., et al. (2019). An Investigation of a Solar Cooker with Parabolic Trough Concentrator. Case Stud. Therm. Eng. 14, 100436. doi:10.1016/j.csite.2019.100436
Omara, A. A. M., Abuelnuor, A. A. A., Mohammed, H. A., Habibi, D., and Younis, O. (2020). Improving Solar Cooker Performance Using Phase Change Materials: A Comprehensive Review. Solar Energy 207, 539–563. doi:10.1016/j.solener.2020.07.015
Regin, A. F., Solanki, S. C., and Saini, J. S. (2008). Heat Transfer Characteristics of thermal Energy Storage System Using PCM Capsules: a Review. Renew. Sustainable Energ. Rev. 12, 2438–2458. doi:10.1016/j.rser.2007.06.009
Roth, P., Georgiev, A., and Boudinov, H. (2005). Cheap Two axis Sun Following Device. Energ. Convers. Manag. 46, 1179–1192. doi:10.1016/j.enconman.2004.06.015
Saxena, A., and Agarwal, N. (2018). Performance Characteristics of a New Hybrid Solar Cooker with Air Duct. Solar Energy 159, 628–637. doi:10.1016/j.solener.2017.11.043
Saxena, A., Cuce, E., Tiwari, G. N., and Kumar, A. (2020). Design and thermal Performance Investigation of a Box Cooker with Flexible Solar Collector Tubes: An Experimental Research. Energy 206, 118144. doi:10.1016/j.energy.2020.118144
Saxena, A., and Karakilcik, M. (2017). Performance Evaluation of a Solar Cooker with Low Cost Heat Storage Material. Ijsge 6, 57–63. doi:10.11648/j.ijrse.20170604.12
Saxena, A., Lath, S., and Tirth, V. (2013a). Solar Cooking by Using PCM as a thermal Heat Storage. MIT Int. J. Mech. Eng. 3, 91–95.
Saxena, A., Lath, S., and Tirth, V. (2013b). Solar Cooking by Using PCM as a thermal Heat Storage. MIT Int. J. Mech. Eng. 3, 91–95.
Sharma, A., Tyagi, V. V., Chen, C. R., and Buddhi, D. (2009). Review on thermal Energy Storage with Phase Change Materials and Applications. Renew. Sustainable Energ. Rev. 13, 318–345. doi:10.1016/j.rser.2007.10.005
Skouri, S., Ben Haj Ali, A., Bouadila, S., Ben Salah, M., and Ben Nasrallah, S. (2016). Design and Construction of Sun Tracking Systems for Solar Parabolic Concentrator Displacement. Renew. Sustainable Energ. Rev. 60, 1419–1429. doi:10.1016/j.rser.2016.03.006
Smith, K. R., Khalil, M. A. K., Rasmussen, R. A., Thorneloe, S. A., Manegdeg, F., and Apte, M. (1993). Greenhouse Gases from Biomass and Fossil Fuel Stoves in Developing Countries: a Manila Pilot Study. Chemosphere 26, 479–505. doi:10.1016/0045-6535(93)90440-g
Stine, W. B., and Diver, R. B. (1994). A Compendium of Solar Dish/Stirling Technology. Albuquerque, NM (United States): Sandia National Labs.
Sup, B. A., Zainudin, M. F., Ali, T. Z. S., Bakar, R. A., and Ming, G. L. (2015). Effect of Rim Angle to the Flux Distribution Diameter in Solar Parabolic Dish Collector. Energ. Proced. 68, 45–52. doi:10.1016/j.egypro.2015.03.231
Tucker, M. (1999). Can Solar Cooking Save the Forests? Ecol. Econ. 31, 77–89. doi:10.1016/s0921-8009(99)00038-5
Wentzel, M., and Pouris, A. (2007). The Development Impact of Solar Cookers: a Review of Solar Cooking Impact Research in South Africa. Energy policy 35, 1909–1919. doi:10.1016/j.enpol.2006.06.002
Yadav, V., Kumar, Y., Agrawal, H., and Yadav, A. (2017). Thermal Performance Evaluation of Solar Cooker with Latent and Sensible Heat Storage Unit for Evening Cooking. Aust. J. Mech. Eng. 15, 93–102. doi:10.1080/14484846.2015.1093260
Yahuza, I., Rufai, Y., and Tanimu, L. (2016). Design, Construction and Testing of Parabolic Solar Oven. J. Appl. Mech. Eng. 5.
Yettou, F., Gama, A., Azoui, B., Malek, A., and Panwar, N. L. (2019). Experimental Investigation and thermal Modelling of Box and Parabolic Type Solar Cookers for Temperature Mapping. J. Therm. Anal. Calorim. 136, 1347–1364. doi:10.1007/s10973-018-7811-9
Zamani, H., Moghiman, M., and Kianifar, A. (2015). Optimization of the Parabolic Mirror Position in a Solar Cooker Using the Response Surface Method (RSM). Renew. Energ. 81, 753–759. doi:10.1016/j.renene.2015.03.064
Nomenclature
A Area (m2)
C Concentration ratio
D Diameter (mm)
f focal length (mm)
hc,i−gls convective heat transfer coefficient from air to glass (W/m2 K)
hc,gls−a convective heat transfer coefficient from glass to ambient (W/m2 K)
hc,p−i convective heat transfer coefficient from cooking pot to enclosed air (W/m2 K)
hc,v−i convective heat transfer coefficient from the cooking vessel to enclosed air (W/m2 K)
hr,gls−s radiative heat transfer coefficient from glass to the pot surface (W/m2 K)
hr,pot−gls radiative heat transfer coefficient from cooking pot to glass (W/m2 K)
hr,v−gls radiative heat transfer coefficient from the cooking vessel to glass (W/m2 K)
hc,ref−a radiative heat transfer coefficient from the reflector to ambient (W/m2 K)
hc,pot−a convective heat transfer coefficient from the cooking pot to ambient (W/m2 K)
hc,pot−wat convective heat transfer coefficient from the cooking pot to water (W/m2 K)
hr,pot−ref radiative heat transfer coefficient from air to glass (W/m2 K)
hr,pot−s radiative heat transfer coefficient from the pot to the cover surface (W/m2 K)
hc,ref−s convective heat transfer coefficient from the reflector to the pot surface (W/m2 K)
hr,ref−s radiative heat transfer coefficient from the reflector to the pot surface (W/m2 K)
Keywords: design, cooking, cooking power, thermal efficiency, heat storage materials, modelling, energy
Citation: Komolafe CA and Okonkwo CE (2022) Design, Fabrication, and Thermal Evaluation of a Solar Cooking System Integrated With Tracking Device and Sensible Heat Storage Materials. Front. Energy Res. 10:821098. doi: 10.3389/fenrg.2022.821098
Received: 23 November 2021; Accepted: 07 February 2022;
Published: 07 March 2022.
Edited by:
K Sudhakar, Universiti Malaysia Pahang, MalaysiaReviewed by:
Abhishek Saxena, Moradabad Institute of Technology, IndiaHafiz Muhammad Ali, King Fahd University of Petroleum and Minerals, Saudi Arabia
Copyright © 2022 Komolafe and Okonkwo. This is an open-access article distributed under the terms of the Creative Commons Attribution License (CC BY). The use, distribution or reproduction in other forums is permitted, provided the original author(s) and the copyright owner(s) are credited and that the original publication in this journal is cited, in accordance with accepted academic practice. No use, distribution or reproduction is permitted which does not comply with these terms.
*Correspondence: Clement A. Komolafe, Y2xlbWt1bmxlQHlhaG9vLmNvLnVr