- 1Research Institute of Petroleum Exploration and Development, CNPC, Beijing, China
- 2China University of Geosciences, Department of Petroleum Engineering, Wuhan, China
- 3Arusha Technical College, Arusha, Tanzania
1 Introduction
Tight gas reservoirs are non-conventional rock reservoirs with matrix permeability of less than 0.1mD and porosity of less than 15% (Kalam et al., 2021). The ultimate gas recovery rates in these reservoirs are very low due to the limited permeability and unfavourable reservoir characteristics which undermine the production of hydrocarbons (Syah et al., 2021). The recovery factor may be greatly elevated by using horizontal drilling and multi-stage hydraulic fracturing (Syed, Muther, Van, Dahaghi, & Negahban, 2022). Due to water shortages and contamination of subsurface water, fracturing fluids commonly used for water-sensitive formations face significant challenges (Shen et al., 2021). Therefore, a viable option is to combine carbon capture, utilization, and storage (CCUS) technology with enhanced gas recovery (EGR) technology using CO2. This technique provides additional economic and environmental value because it uses existing infrastructure to increase gas recovery and permanently store CO2 in depleted reservoirs (Ren et al., 2023).
2 Comprehensive analysis of CO2-assisted technologies
2.1 CO2 fracturing
The failure of water-based fracturing fluids in unconventional reservoirs, especially those with high clay content, led to the development of CO2 fracturing technology (Zhao et al., 2021). With CO2 fracturing, the reservoir rock and formation fluids undergo a variety of physical and chemical reactions. As a result of these reactions, reservoirs may become more porous and permeable, and the flow of natural gas commences. Gas recovery factor is enhanced and CO2 is permanently stored in the geological formations (Tao et al., 2021). Tight reservoirs and other unconventionals are characterized by high temperature especially when the depth exceeds 1000 m where the temperature and pressure are above the critical point of CO2 (31.1 C, 7.38 MPa, respectively). These formation conditions make CO2 attain the supercritical state exhibiting superior properties such as low viscosity, strong diffusion, much higher density than gas, and almost no surface tension (He et al., 2022). CO2 fracturing is significantly superior as it lowers the pressure required to initiate a fracture, connect micro-fractures, and create intricate fracture networks appropriate for rock formations with low pressure, low permeability, and high-water sensitivity. Additionally, liquid CO2 can be used as a fracturing fluid and has several benefits over others, including quick well cleanup, removal of formation damage, and low cost. However, the widespread use of CO2 fracturing fluids has been constrained by inadequate proppant transfer, significant friction loss, and high pump displacement associated with low fluid viscosity (Middleton et al., 2015).
2.2 CO2-responsive gel blockage
Due to CO2 poor mobility control, phase segregation, and extremely low viscosity, gas has the propensity to finger and break through into production wells easily bypassing unswept oil zones (Dai et al., 2017). To address these challenges and improve sweep efficiency, CO2-responsive gels were developed to plug and divert CO2 into oil zones. These chemicals have special functional groups (amines, amidines, guanidines, and carboxylic acids) on either surfactant or polymer chains that activate their responses based on the pH change caused by the presence of CO2 (Yang, He, Sui, He, & Li, 2019). The interaction of CO2 and water in the formation produces carbonic acid leading to protonation of the responsive tertiary amines, electrostatic repulsion among the polymer particles, and an increase in the particle size (Du D. et al., 2022). The injection of N2 induces a reversible reaction by changing the pH of the system leading to CO2 release and gel particle shrinkage (Y. Liu & Liu, 2022).
(Shen et al., 2021) investigated CO2-responsive worm-like micelles (WLMs) called N, N-dimethyl erucamide tertiary amine (DMETA) as a novel plugging agent where a recovery factor was enhanced by 21.7%. Furthermore (Wang et al., 2021), reported the ternary system comprising of cetyltrimethylammonium bromide (CTAB), sodium salicylate (NaSal), and N, N-dimethylcyclohexylamine (DMCA) as plugging agent with improved gas recovery. Their mechanism involved protonating DMCA by CO2 injection to induce a structural change from spherical micelles to WLM, reverting to a spherical state upon addition of NaOH Although this technology has been successful, its effectiveness is hindered by surfactant/polymer loss caused by surface adsorption, retention, thermal degradation, and precipitation in a high-temperature and saline environment (Massarweh & Abushaikha, 2022).
2.3 CO2-CH4 competitive adsorption
In tight reservoirs, CH4 is found adsorbed to organic matter and clay minerals, in a free state in fractures and pores, and trace amounts as a dissolved gas in the liquid phase. Initially, quick gas is produced from free-state gas while the remaining gas (85%) is accessed by other advanced EGR techniques, like a gas CO2 injection (S. Liu, Sun, Xu, Li, & Wang, 2020). For technical and economic reasons, CO2 injection for enhancing gas recovery is coupled with CO2 sequestration in geological formations. Geological sequestration of CO2 in depleted reservoirs is widely considered as one of the most effective techniques to reduce greenhouse gas emissions (Du X. et al., 2022). This is due to the fact that kerogen and formation minerals have higher adsorption capacity to CO2 (Ma, Yue, Li, Xu, & Niu, 2019). The adsorption capacity of CO2 and CH4 can be estimated by using experimental and simulation results and then fitted in various isothermal models (Langmuir) (Bemani, Baghban, Mohammadi, & Andersen, 2020). Most findings indicated that more than 60% of the injected CO2 was adsorbed and the pre-adsorbed CH4 ejected as shown in Figure 1B. However, the efficacy of CO2-CH4 competitive adsorption is still limited by both the reservoir and surrounding environments such as the amount of TOC, kerogen, pressure, and high temperature Adsorption increases with increasing pressure to the optimum level and a higher amount of kerogen since it contains more surface adsorption sites (Kang, Zhang, Kang, Guo, & Zhao, 2020).
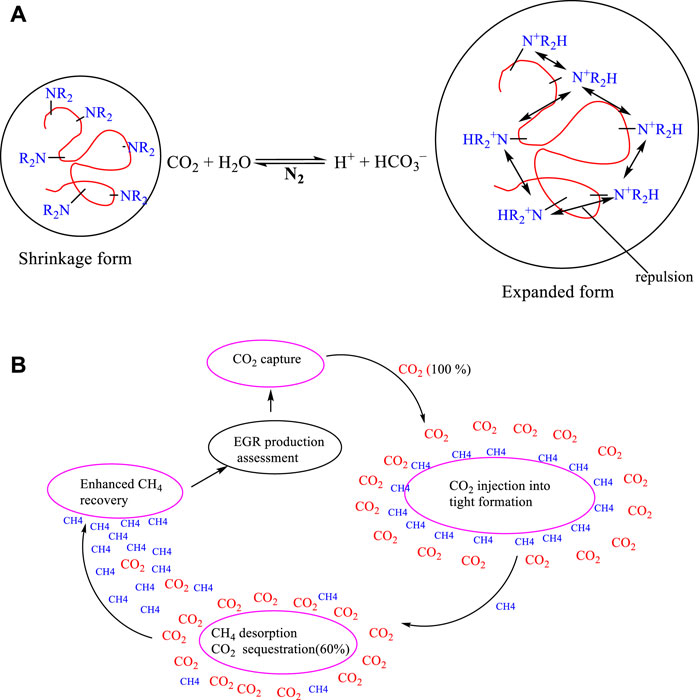
FIGURE 1. (A) Polymer CO2 responsive gel expansion mechanism (Wu et al., 2023), (B) CO2-CH4 competitive adsorption.
3 Conclusion
To extract gas from tight reservoirs, a variety of CO2-assisted systems have been developed. CO2 as a fracturing fluid has many advantages over water. These include suitability for complex rock formations with low pressure, low permeability, and strong water sensitivity.
Even though CO2-fracturing fluid has shown better performance as reported elsewhere, it still needs more research to determine how nanoparticles could improve its viscosity and thereby minimize friction losses, higher pumping pressures, and proppant carrying failures.
CO2-responsive gel blockage has been successfully applied in the US, China, and other countries due to the careful selection of surfactants/polymers that are compatible with reservoir conditions and optimized formulation ratios. Certain setbacks must be addressed as well, such as unexpected retention, temperature-induced instabilities, or phase separation in the reservoir. Field-scale modeling and simulation of the phase behavior of ternary systems are advocated.
CCUS technology and CO2-EGR are required to lower CO2 emissions while enhancing natural gas output in order to make it commercially feasible. The viability of coupled approaches, in which CO2-CH4 competitive adsorption plays a key role, is assured by an increase in global gas prices.
Author contributions
XZ: investigation and research, writing manuscript draft; KX: resources and conceptualization; CW: modify analysis; XL: typesetting; AOM: supervision.
Funding
This work is supported by PetroChina “Fourteenth Five Year” Significant Programs (No. 2021DJ3203).
Conflict of interest
The authors declare that the research was conducted in the absence of any commercial or financial relationships that could be construed as a potential conflict of interest.
Publisher’s note
All claims expressed in this article are solely those of the authors and do not necessarily represent those of their affiliated organizations, or those of the publisher, the editors and the reviewers. Any product that may be evaluated in this article, or claim that may be made by its manufacturer, is not guaranteed or endorsed by the publisher.
References
Bemani, A., Baghban, A., Mohammadi, A. H., and Andersen, P. Ø. (2020). Estimation of adsorption capacity of CO2, CH4, and their binary mixtures in Quidam shale using LSSVM: Application in CO2 enhanced shale gas recovery and CO2 storage. J. Nat. Gas Sci. Eng. 76, 103204. doi:10.1016/j.jngse.2020.103204
Dai, Z., Viswanathan, H., Xiao, T., Middleton, R., Pan, F., Ampomah, W., et al. (2017). CO2 sequestration and enhanced oil recovery at depleted oil/gas reservoirs. Energy Procedia 114, 6957–6967. doi:10.1016/j.egypro.2017.08.034
Du, D., Chen, B., Pu, W., Zhou, X., Liu, R., and Jin, F. (2022). Colloids and Surfaces A : Physicochemical and Engineering Aspects CO 2 -responsive gel particles and wormlike micelles coupling system for controlling CO 2 breakthrough in ultra-low permeability reservoirs. Colloids Surfaces A Physicochem. Eng. Aspects 650 129546. doi:10.1016/j.colsurfa.2022.129546
Du, X., Pang, D., Zhao, Y., Hou, Z., Wang, H., and Cheng, Y. (2022). Investigation into the adsorption of CO 2 , N 2 and CH 4 on kaolinite clay. Arabian J. Chem. 15 (3), 103665. doi:10.1016/j.arabjc.2021.103665
He, J., Zhang, Z., Li, G., Huo, J., Li, S., and Li, X. (2022). Modeling study on supercritical CO2 fracturing applicability and capacity to stimulate reservoirs with different permeabilities. J. Petroleum Sci. Eng. 213, 110427. doi:10.1016/j.petrol.2022.110427
Kalam, S., Afagwu, C., Al Jaberi, J., Siddig, O. M., Tariq, Z., Mahmoud, M., et al. (2021). A review on non-aqueous fracturing techniques in unconventional reservoirs. J. Nat. Gas Sci. Eng. 95, 104223. doi:10.1016/j.jngse.2021.104223
Kang, G., Zhang, B., Kang, T., Guo, J., and Zhao, G. (2020). Effect of pressure and temperature on co2/ch4 competitive adsorption on kaolinite by Monte Carlo simulations. Materials 13 (12), 2851–2912. doi:10.3390/ma13122851
Liu, S., Sun, B., Xu, J., Li, H., and Wang, X. (2020). Study on competitive adsorption and displacing properties of CO2Enhanced shale gas recovery: Advances and challenges. Geofluids 2020 6657995, doi:10.1155/2020/6657995
Liu, Y., and Liu, Q. (2022). Review of gel systems for CO2 geological storage leakage and conformance control for enhanced oil recovery: Mechanisms, recent advances, and future perspectives. J. Petroleum Sci. Eng. 219, 111110. doi:10.1016/j.petrol.2022.111110
Ma, Y., Yue, C., Li, S., Xu, X., and Niu, Y. (2019). Study of CH4 and CO2 competitive adsorption on shale in yibin, sichuan province of China. Carbon Resour. Convers. 2 (1), 35–42. doi:10.1016/j.crcon.2018.11.005
Massarweh, O., and Abushaikha, A. S. (2022). A review of recent developments in CO 2 mobility control in enhanced oil recovery. Petroleum 8 (3), 291–317. doi:10.1016/j.petlm.2021.05.002
Middleton, R. S., Carey, J. W., Currier, R. P., Hyman, J. D., Kang, Q., Karra, S., et al. (2015). Shale gas and non-aqueous fracturing fluids : Opportunities and challenges for supercritical CO 2. Appl. Energy 147, 500–509. doi:10.1016/j.apenergy.2015.03.023
Ren, D., Wang, X., Kou, Z., Wang, S., Wang, H., Wang, X., et al. (2023). Feasibility evaluation of CO2 eor and storage in tight oil reservoirs: A demonstration project in the ordos basin. Fuel 331 (P2), 125652. doi:10.1016/j.fuel.2022.125652
Shen, H., Yang, Z., Li, X., Peng, Y., Lin, M., Zhang, J., et al. (2021). CO2-responsive agent for restraining gas channeling during CO2 flooding in low permeability reservoirs. Fuel 292, 120306. doi:10.1016/j.fuel.2021.120306
Syah, R., Alizadeh, S. M., Nurgalieva, K. S., Guerrero, J. W. G., Nasution, M. K. M., Davarpanah, A., et al. (2021). A laboratory approach to measure enhanced gas recovery from a tight gas reservoir during supercritical carbon dioxide injection. Sustain. Switz. 13 (21), 11606. doi:10.3390/su132111606
Syed, F. I., Muther, T., Van, V. P., Dahaghi, A. K., and Negahban, S. (2022). Numerical trend analysis for factors affecting EOR performance and CO2 storage in tight oil reservoirs. Fuel 316, 123370. doi:10.1016/j.fuel.2022.123370
Tao, J., Meng, S., Jin, X., Xu, J., Yang, Q., Wang, X., et al. (2021). Stimulation and Sequestration Mechanism of CO 2 Waterless Fracturing for Continental Tight Oil Reservoirs, ACS Omega 32 2–11. doi:10.1021/acsomega.1c01059
Wang, J., Huang, Z., Wu, Y., Sun, L., Zheng, C., and Sengupta, A. (2021). Understanding the mechanism of the CO2 responsive viscoelastic fluids obtained from cetyltrimethylammonium bromide, sodium salicylate and N, N-dimethylcyclohexylamine. Chem. Phys. Lett. 777, 138734. doi:10.1016/j.cplett.2021.138734
Wu, Y., Liu, Q., Liu, D., Cao, X. P., Yuan, B., and Zhao, M. (2023). CO2 responsive expansion hydrogels with programmable swelling for in-depth CO2 conformance control in porous media. Fuel 332 (P1), 126047. doi:10.1016/j.fuel.2022.126047
Yang, Z., He, C., Sui, H., He, L., and Li, X. (2019). Recent advances of CO 2 -responsive materials in separations. J. CO2 Util. 30, 79–99. doi:10.1016/j.jcou.2019.01.004
Keywords: foam fluid, fracturing fluid, tsight gas reservoir, gas chanelling, CCUS
Citation: Zhang X, Xiao K, Wang C, Li X and Mmbuji AO (2023) CO2-assisted technologies for the development of tight gas reservoirs: The implication on CCUS. Front. Energy Res. 10:1102774. doi: 10.3389/fenrg.2022.1102774
Received: 19 November 2022; Accepted: 28 November 2022;
Published: 19 January 2023.
Edited by:
Xun Zhong, Yangtze University, ChinaReviewed by:
Yibo Li, Southwest Petroleum University, ChinaYining Wu, China University of Petroleum (Huadong), China
Copyright © 2023 Zhang, Xiao, Wang, Li and Mmbuji. This is an open-access article distributed under the terms of the Creative Commons Attribution License (CC BY). The use, distribution or reproduction in other forums is permitted, provided the original author(s) and the copyright owner(s) are credited and that the original publication in this journal is cited, in accordance with accepted academic practice. No use, distribution or reproduction is permitted which does not comply with these terms.
*Correspondence: Athumani Omari Mmbuji, bW1idWppX2N1Z0BzaW5hLmNvbQ==