- 1State Key Laboratory of Enhanced Oil Recovery, PetroChina, Beijing, China
- 2Research Institute of Petroleum Exploration and Development, PetroChina, Beijing, China
- 3College of Engineering, Polytechnic Institute, Hebei Normal University, Shijiazhuang, China
With the gradually deep development of oil field, the remaining oil in reservoir become more scattered, to exploit them within the high water-cut pay become extremely difficult. Based on the characteristics of extremely high water-cut reservoir, three large scale physical models with different injection-production well patterns are designed. Through the analysis of oil production rate and the data of Computed Tomography (CT) scanning, the change law of fluid content within the core are obtained; The new calculation method of sweep efficiency for waterflooding within non-homogeneous models are set up in accordance with the CT number at different waterflooding stages. Furthermore, the development results of different plans are compared with each other, both injection and production with horizontal wells at low-permeability formation have the best recovery, because the displacement fluid can reach the places in reservoir where conventional waterflooding cannot. Therefore, this well pattern is the optimum plan for the positive rhythm model in this experiment, and the development strategy optimization is realized for high water-cut thick oil formation.
1 Introduction
With the increase of the world’s population, human demand for fossil energy such as oil and gas has been increasing (Tang, 20222022; Wang et al., 2022). However, due to the impact of the epidemic and geopolitical factors, oil and gas companies are facing a series of challenges, such as large fluctuations in oil prices, increasingly complex exploration environment, continuous increase in environmental regulation and increasing exploitation costs (Cao and Liu, 2021; Dang et al., 2022; Dou et al., 2022; Li et al., 2022). Therefore, how to continuously improve oil recovery has always been of great significance to oil and gas companies (Chen, 2021; Wang et al., 2021).
Reservoir heterogeneity is one of the important factors affecting oilfield development, which not only reduces vertical sweep efficiency of the reservoir, but also has a greater impact on the oil displacement efficiency in the water injection layer system, and reduces the ultimate recovery (Tang et al., 2006; Li et al., 2018; He et al., 2021). For example, in the typical case of Daqing Lasaxing Oilfield, 100% of the main oil layers with thickness larger than 1 m have water breakthrough, and the remaining oil is mainly distributed in the water washing layer with 74.4% of the remaining geological reserves, and 45.7% of the remaining geological reserves are controlled by the oil layers with thickness larger than 2 m. The “in-layer contradiction” of thick oil reservoirs has become the main contradiction in the high water cut stage (Wu et al., 2014; Du et al., 2019; Shi and Wu, 2019). Understanding reservoir heterogeneity and remaining oil distribution is the basis of improving oilfield development effect. Seismic, logging and other means can be used to depict the geological model of the reservoir scale and characterize its macroscopic heterogeneity, which lays a solid foundation for the water injection development of the oilfield. Taking the Badaowan Formation reservoir in the Chong-18 well block of Fengcheng Oilfield as an example, He et al. established a geological model of combined facies types and combined facies. Then the sequential Gaussian algorithm is used to establish the reservoir property model, and the reliability of the model is verified. The study can provide reference for reservoir dynamic management and numerical simulation (He et al., 2017). Wen et al. proposed 14 main parameters affecting reservoir heterogeneity and established a three-dimensional reservoir comprehensive index model. Then the model is applied to the reservoir characterization of gas reservoir in Su 53 block, and useful results are obtained (Wen and Sun, 2011). In the study of the heterogeneity of the fan-delta reservoir in the Tan-Lu fault zone, Huang et al. synthesized a variety of modeling methods and theories, and established its sedimentary microfacies model, physical parameter model, fractal geometric model, etc. Furthermore, the advantages and disadvantages of various models are compared (Huang et al., 2005). However, with the deepening of development, the distribution of remaining oil tends to be scattered, and the understanding of reservoir micro-heterogeneity needs to be strengthened. How to excavate the remaining oil in the water layer will be an unavoidable problem for continuous improvement of oil recovery (Zhang et al., 2008; Li et al., 2010; Liu et al., 2015). In order to improve the recovery factor of low permeability reservoir in high water cut stage, Hou et al. carried out the core displacement experimental study in the laboratory and obtained the mechanism of deep profile control to improve the recovery factor (Hou et al., 2020). Lin et al. used the parallel core water drive technology to obtain the main reasons for the low efficiency of water injection development in the late stage of high water cut. This study provides a reasonable basis for the formulation of development measures in the later stage of high water cut (Lin and Zhang, 2010). Zhao et al. confirmed that cyclic water injection can improve the development effect of heterogeneous sandstone reservoirs through indoor physical simulation experiments, which provides experimental basis for technical decision-making of oilfield development (Zhao et al., 2019). Zhuge used the strip heterogeneous simulation microscopic model to carry out the experimental study of water flooding, and defined the microscopic oil displacement mechanism for improving the water flooding effect in high water cut period (Zhuge, 2010). Guo combined laboratory oil-water phase permeability and nuclear magnetic resonance (NMR) to study the percolation characteristics of reservoirs in high water cut stage, and studied the percolation characteristics of reservoirs in high water cut stage from a microscopic point of view, which is of great significance to the adjustment of old oilfield plans (Guo, 2022). Zhou et al. obtained the influencing factors of remaining oil distribution in the process of water flooding through the analysis of nuclear magnetic resonance imaging core micro-displacement experiment (Zhou et al., 2021). Wu et al. carried out T2 spectrum test and slice imaging detection on each stage of Bailey core high multiple water flooding experiment by using nuclear magnetic resonance instrument, and obtained the specific correlation between oil displacement efficiency and injection multiple (Wu et al., 2019). Based on the established three-dimensional physical model and combined with nuclear magnetic resonance, Xiao et al. defined the remaining oil producing mechanism under the dominant channel. Specific strategies for remaining oil recovery are proposed (Xiao et al., 2017).
As early as the 1980s, Computed Tomography (CT) scanning technology has been applied to the study of oil and gas reservoirs in China, and has gradually become an important tool for studying the characteristics of formation rocks. In the displacement experiment, CT scanning technology can be used to dynamically observe the fluid seepage process and saturation distribution characteristics in the core during different displacement processes without changing the experimental device as well as the external shape and internal structure of the core. Gao et al. uses CT scanner to scan the core displacement process axially to obtain the oil saturation and oil displacement characteristic curve of each section. By comparing with the measured oil saturation obtained by the displacement method, it is found that the CT method has higher accuracy (Gao et al., 2009); Cao found that CT scanning is an intuitive and effective technology to study remaining oil through CT scanning of water flooding, polymer flooding and subsequent water flooding experiments, and comparing the remaining oil distribution during the experiment according to the scanning results, which can quantitatively depict the distribution of remaining oil in different displacement processes and at different displacement times (Cao, 2015; Cao and Liu, 2015); By comparing the water saturation distribution obtained by the oil-water metering method and the X-ray scanning method in the water-flooding CT scanning experiment, Sun et al. found that CT scanning can not only obtain the dynamic change of the water-flooding front and the real-time water saturation distribution, but also more accurately describe the distribution of water saturation at different sections (Sun et al., 2017). Compared with the oil saturation measured by oil-water measurement method and displacement experiment method, the CT scanning method has higher accuracy and can make up for the shortcomings of the first two methods. Although the application of CT scanning technology to study the distribution of remaining oil in the core has been relatively mature, there is still room of improvement for the application of CT scanning displacement experiment into large-scale physical models. In this experiment, the calculation method of sweep efficiency is built and the application scope of CT scanning is enlarged in the study of oil field development. According to the reservoir characteristics of ultra-high water cut stage, three large-scale physical models with different types of injection-production wells are designed to simulate oil displacement experiments, study the oil-water distribution and seepage characteristics of each model in the process of water flooding, analyze the development effect of different injection-production schemes, and finally realize the optimization of development strategy for thick reservoirs in high water cut stage.
2 Experimental system
A physical modelling experimental device based on CT scanning system is established to study the mechanism of development adjustment strategy for heterogeneous thick reservoir. Its schematic diagram is shown in Figure 1. It is mainly composed of CT scanning system, displacement system, experimental core and measurement system. The core is designed by layering, embedded in the well pipe according to the experimental requirements, and covered by epoxy resin material, which can be used for CT scanning and can be measured by laryer. The experimental flow setup and the large-scale physical model are shown in Figures 2, 3.
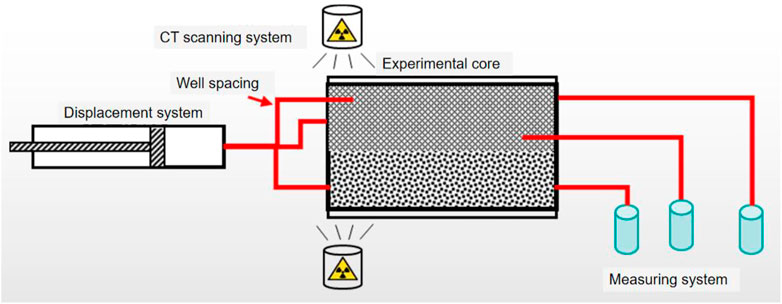
FIGURE 1. Schematic diagram of physical modelling platform for mechanism study on development adjustment strategy of heterogeneous thick reservoir.
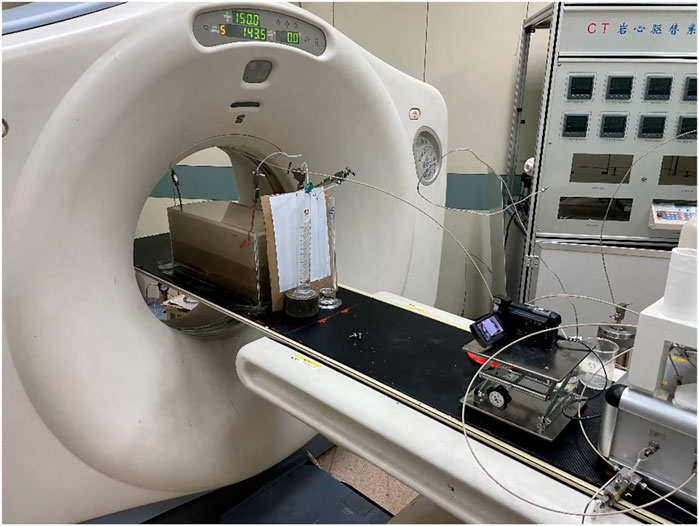
FIGURE 2. Physical modelling platform for mechanism study on development adjustment strategy of heterogeneous thick reservoir.
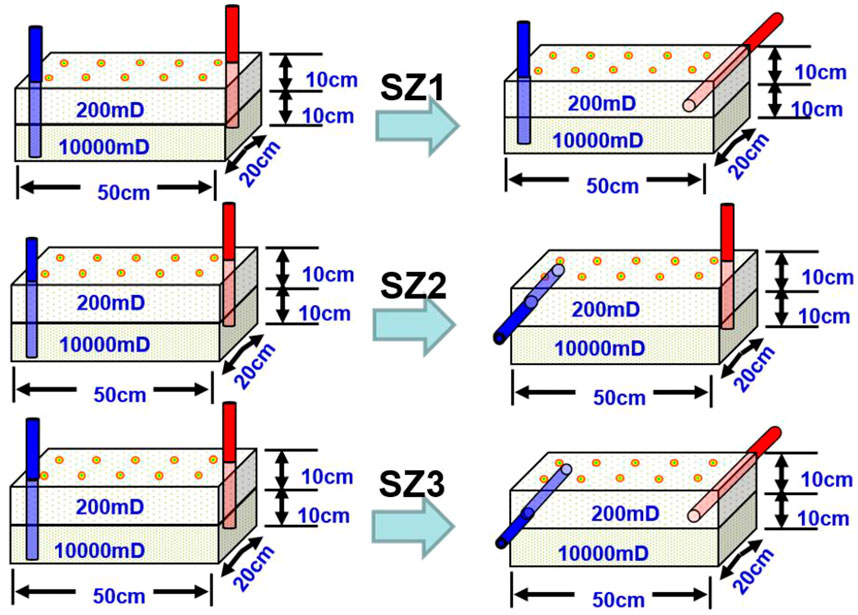
FIGURE 3. Three large-scale physical models for mechanism study on development adjustment strategy of heterogeneous thick reservoir.
3 Experimental method
In this study, three groups of water flooding experiments with positive rhythmic intraformational heterogeneity were carried out to analyze the water flooding recovery percent and oil-water movement law of the positive rhythmic intraformational heterogeneity model under different water injection schemes.
As shown in Figure 3, from top to bottom, the models are SZ1, SZ2 and SZ3 respectively. The physical experimental model is a positive rhythm heterogeneous core of epoxy resin material with different well spacing schemes. Experimental oil used is the mixed oil of 15# white oil with aviation kerosene. At temperature 23 °C, its viscosity is 11.93 mPa s and its density is .8292 g/cm3 10% content NaBr saline is used as formation water in the experiment. Parameters and schemes of models are shown in Table 1.
Firstly, the basic parameters of each model, such as porosity and permeability, were measured. The permeability is as shown in Figure 3 and the porosity for each model is 25%. The model, simulated oil and 10% NaBr saline were scanned by CT. The core is then evacuated to saturate the simulated oil, and the saturated wet model is CT scanned. In the first stage, 10% NaBr saline is used for displacement at the rate of 6 ml/min, and the amount of oil and water produced at the outlet is measured separately (stratified measurement method). In the early stage of water flooding, CT is used to scan once every 25 min, and in the late stage of water flooding, the time interval of CT scanning is increased. At the end of the experiment, the software was used to analyze the CT scanning data and calculate the sweep efficiency and water saturation of the model at each scanning time.
For the calculation of sweep efficiency, there are actually three steps. First of all, through the oil and water production and CT scanning data, the digital core analysis software is used to analyze and obtain the internal fluid changes in models. Secondly, according to the distribution characteristics of oil and water in models of the experiment, the change of CT number in each stage of water flooding is calculated on the basis of establishing the data matrix and visual image of oil and water distribution. Finally, the water flooding sweep efficiency of the heterogeneous model is calculated.
4 Results and discussion
4.1 Overall injection-production displacement effect of vertical and horizontal wells
Through the online CT scanning of the water flooding process of the heterogeneous model, the oil-water distribution of three groups of positive rhythm heterogeneous models at each water injection stage can be obtained.
In the process of water flooding experiment, after the injection and production of vertical wells, the injection and production of horizontal wells were used, and the water cut of the produced fluid decreased in the three groups of positive rhythm models, the total recovery degree was further improved, and the upwarping water flooding characteristic curve turned downward, as shown in Figure 4, Figures 5, 6. It shows that in the high water cut stage, the above measures can effectively improve the oil displacement efficiency and improve the recovery percent of the reservoir. Specifically, Figure 4 is about the water-flooding characteristic curves of different positive rhythm models SZ1, SZ2, and SZ3. Figure 5 is about the relation between recovery degree and injection pore volume of different models SZ1, SZ2, and SZ3. Figure 6 is about the relation between water cut and injection pore volume of different models SZ1, SZ2, and SZ3.
4.2 Overall water saturation distribution in conventional water flooding stage by vertical wells
As shown in Figures 7, 8, in the injection-production stage of the vertical well, due to the permeability difference, the displacement fluid first enters the lower part of the high permeability layer, forming a dominant channel for water flow. The displacement fluid entering the middle part of the low permeability layer and the middle and upper part of the high permeability layer become less, and the two parts have a low degree of production, resulting in a low degree of recovery.
4.3 Water saturation distribution of each layer at production stage by horizontal well in SZ1 model
As shown in Figure 9, the recovery percent of the SZ1 model is 22% at the injection and production stage by vertical wells. It is switched to the mode of injection by vertical well and production by horizontal well in the low permeability layer at the later stage. At this time, a large amount of displacement fluid enters the high permeability layer through the vertical well, and gradually moves up into the low permeability layer in the flow process, and finally flows into the horizontal well in the low permeability layer. The water saturation in the near wellbore area of the horizontal well increases significantly. In this process, the oil in the middle and upper part of the high permeability layer is swept by the displacement liquid and enters the horizontal well in the low permeability layer, while the oil in the lower and rear part of the low permeability layer is also produced, and the final recovery degree reaches 59.4%.
In terms of sweep efficiency, when the final recovery degree reaches 59.4%, the sweep efficiency of low permeable layer in SZ1 model increases from 25.1% to 42.3% in the conventional water flooding stage, with an increase of 17.2%. The sweep efficiency of high permeable layer in SZ1 model increases from 51.2% to 83.3%, with an increase of 32.1%. It can be seen that the vertical well injection and horizontal well recovery scheme in low permeability layer can obviously improve the sweep efficiency of high permeability layer, and the recovery percent is mainly contributed by high permeability layer, and also has a certain improvement effect on low permeability layer.
4.4 Water saturation distribution of each layer at injection stage by horizontal well in SZ2 model
As shown in Figure 10, the recovery percent of the SZ2 model is 25.7% at the injection and production stage of the vertical wells, and it is switched to the horizontal well injection and vertical well production mode in the low permeability layer at the later stage. The horizontal well increases the vertical sweep range, and the displacement fluid flows through the upper part of the low permeability layer or through the front part of the low permeability layer to the upper part of the high permeability layer, and the producing degree of these positions is obviously improved, while the lower part of low permeability layer has little change. The final recovery percent is 49.8%.
The sweep efficiency can also reflect the flow of the displacement fluid. When the final recovery of 49.8% is reached, the sweep efficiency of SZ2 model increases from 22.2% during the conventional water flooding stage to 43. 3% in the low permeable layer with an increase of 21.1%. The sweep efficiency increases from 53.6% to 65% in the high permeable layer with an increase of 11.4%. This also shows that the scheme of horizontal well injection and vertical well production in low permeability layers mainly improves the sweep efficiency of low permeability layers, and has little effect on the sweep efficiency of high permeability layers, and the overall recovery percent of the model is mainly contributed by the low permeability layer.
4.5 Water saturation distribution of each layer at injection/production stage by horizontal well in SZ3 model
As shown in Figure 11, the recovery percent of the SZ3 model is 26.2% at the conventional injection and production stage of the vertical wells, and it is switched to the horizontal well injection and horizontal well production mode in the low permeability layer at the later stage. Because the injection and production horizontal wells are located in the low permeability layer, more displacement fluid keeps flowing in the low permeability layer and enters the middle and upper part of the high permeability layer at the same time, and the recovery degree of the high and low permeability layers is obviously improved. When the ultimate recovery of 65.4% is reached, the sweep efficiency of SZ3 model increases from 23% to 41.4% in the low permeable layer and from 56.8% to 88.6% in the high permeable layer, with an increase of 18.4% and 31.8%, respectively. It shows that the effect of horizontal well injection and horizontal well production in low permeability layer is similar to that of vertical well injection and horizontal well production in low permeability layer, and the improvement of sweep efficiency in high permeability layer is obvious, and it also has a certain improvement effect on low permeability layer.
4.6 Effect comparison of three models
The experimental data of recovery percent and sweep efficiency concerning the three models are shown in Table 2. Through the comparison of SZ1 model and SZ3 model, as shown in Figure 12, in the injection and production stage of horizontal wells, under the condition of retaining vertical wells for oil production in low permeability layers, the displacement volume is about 1 pore volume (PV). Comparing SZ1 (vertical well injection, horizontal well production) with SZ3 (horizontal well injection, horizontal well production), the recovery degree of SZ1 is 48.3%, which is 26.3% higher than that before taking potential stimulating measures. The recovery percent of SZ3 is 55.1%, increased by 28.9% than before. The sweep efficiency of SZ1 in high and low permeable layers are increased by 31.1% and 2.1% respectively. Compared with the sweep efficiency of SZ3 in high and low permeable layers which are increased by 27.5% and 12.7% respectively, the sweep effect of SZ1 scheme on low permeable layer is poor, while that of high permeable layer is slightly better. The difference is due to the different flow channels of the two models. The horizontal well in SZ3 makes all the displacement fluid injected into the low-permeability layer, and the sweep range near the injection end of the low-permeability layer is larger, so the sweep efficiency of the low-permeability layers is greatly improved, while the displacement fluid in SZ1 model enters the high-permeability layers in large quantities, so the sweep efficiency of the high-permeability layers is greatly improved.
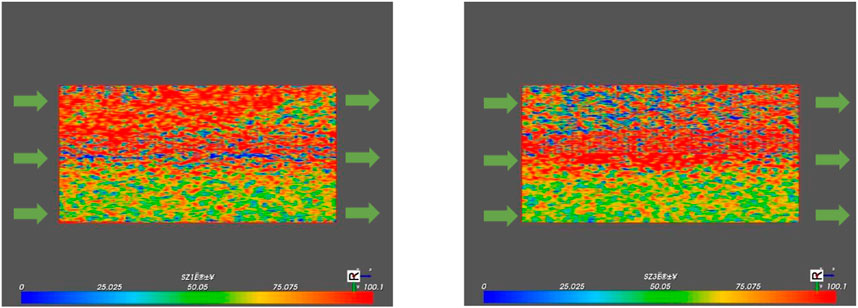
FIGURE 12. Distribution of oil-water saturation in SZ1 (left image) and SZ3 (right image) model respectively.
Continue to close the vertical wells for oil production in low permeability layers until the overall water cut reaches 98%. The final recovery degree of SZ1 (vertical well injection and horizontal well production) is 59.4%, which is 37.4% higher than that before potential stimulating measures are taken. The final recovery degree of SZ3 (horizontal well injection and horizontal well production) is 65.4%, which is 39.2% higher than that before potential stimulating measures are taken. The final increase rates of sweep efficiency of high and low permeable layers in SZ1 are 32.1% and 17.2% respectively, while the final increase rates of sweep efficiency of high and low permeable layers in SZ3 are 31.8% and 18.4% respectively. It can be seen that there is little difference between the two schemes, the sweep efficiency in high permeable layer of SZ1 is slightly better than that in SZ3, and the sweep efficiency in low permeable layer of SZ3 is slightly better than that in SZ1. On the whole, the results in SZ3 is slightly better than that in SZ1. As the vertical well in the low permeability layer is closed, the sweep efficiency of the SZ1 low permeability layer is further significantly improved, and the gap between the two schemes is finally leveled (as shown in Figure 13).
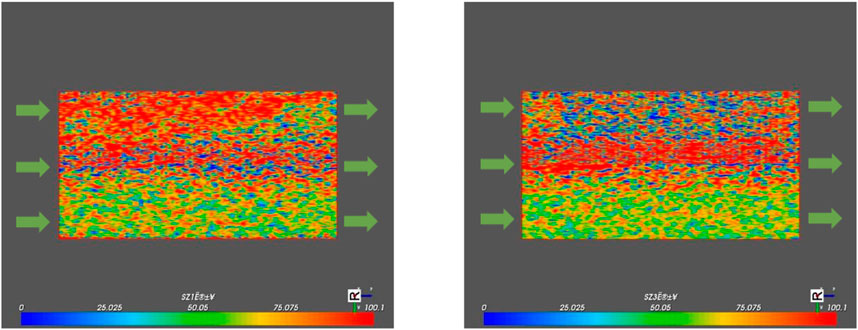
FIGURE 13. Final oil-water saturation distribution of SZ1 (left image) and SZ3 (right image) model respectively.
As shown in Figure 14, by comparing the SZ2 model with the SZ3 model, it is found that in the case of retaining the vertical wells with high and low permeability layers in SZ2 and retaining the vertical wells of low permeability layer in SZ3, comparisons are firstly made when the displacement volume in SZ2 is 1.3 PV, and the displacement volume in SZ3 is 0.9 PV. The recovery percent of SZ2 is 35.6%, which is 9.9% higher than that before potential stimulating measures are taken, and the recovery percent of SZ3 is 55.1%, which is 28.9% higher. The increase of sweep efficiency in SZ2 high and low permeability layers is 2.5% and 8.6% respectively, which is much lower than that in SZ3 high and low permeability layers by 27.5% and 12.7%. The reason is that in the SZ2 model, although the horizontal well injection can improve the sweep efficiency of the low permeability layer, a large amount of displacement fluid enters the vertical well through the water flow channel at the lower part of the high permeability layer, and the ineffective circulation is more serious, so the recovery degree is less improved. The overall recovery percent of reserves of SZ2 is only 40.5% even when the water cut of high-permeability vertical wells is 98%.
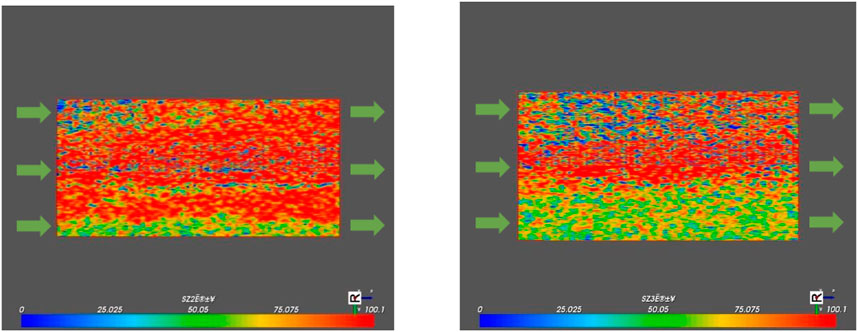
FIGURE 14. Distribution of oil-water saturation in SZ2 (left image) and SZ3 (right image) model respectively.
With the experiment going on, the vertical oil well in high permeability layer is closed for model SZ2, and the vertical oil well in low permeability layer is closed for model SZ3. After that, continue to displace the oil until the total water cut of the produced fluid reached 98%. The final recovery degree of SZ2 (horizontal well injection, vertical well production) was 49.8%, which was 24.1% higher than that before potential stimulating measures were taken, and 39.2% lower than that of SZ3. The final increase of sweep efficiency in SZ2 high permeability layer is 11.4%, which is also much lower than 31.8% of SZ3. The final increase of sweep efficiency in SZ2 low permeability layer is 21.1%, which is slightly higher than 18.4% of SZ3. In general, the recovery degree of SZ3 model is significantly higher than that of SZ2 model, and the horizontal well production scheme is still better than the vertical well production scheme (Figure 15).
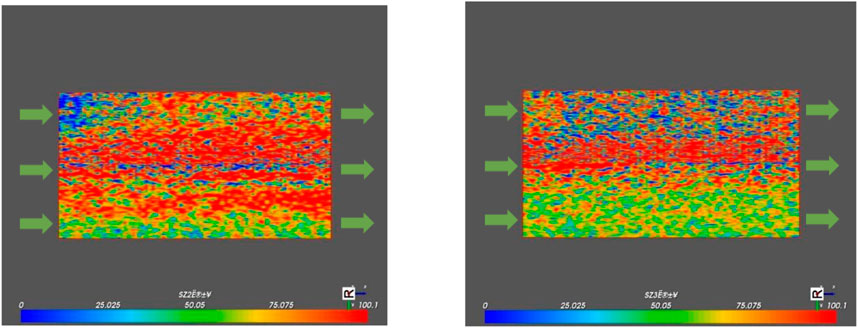
FIGURE 15. Final oil-water saturation distribution of SZ2 (left image) and SZ3 (right image) model respectively.
Therefore, based on the analysis of the displacement experiment results of three groups of positive rhythm heterogeneous models with different water injection schemes, it can be seen that the recovery of horizontal well injection-production scheme is the best, the recovery of vertical well injection and horizontal well production scheme is in the middle and the recovery of horizontal well injection and vertical well production scheme is the smallest. Reasonable utilization of horizontal well injection-production program can change the internal flow channel of displacement fluid in the ultra-high water cut stage reservoir, improve the sweep efficiency in different layers, reduce the inefficient and ineffective circulation, and further improve the recovery of the reservoir.
5 Conclusion
By analyzing the heterogeneous water flooding experiment and according to the variation of CT number in each waterflooding stage, a new method for calculating the waterflooding sweep efficiency of heterogeneous model is established. According to the reservoir characteristics of ultra-high water cut stage, the physical simulation platform and experimental method for the study of the development adjustment strategy of the heterogeneous thick oil reservoir are established. Experiments show that the mode where the horizontal well is used for water injection and oil production in the low permeability layer, which can most effectively make the displacement fluid enter the position that cannot be swept by the conventional water flooding in the reservoir, and the recovery percent is most obviously improved. Therefore, the positive rhythm model is the best solution for this experiment. Although the two schemes of horizontal well and vertical well (model SZ1 and SZ2) can optimize the flow of displacement fluid to a certain extent, they are limited by the small area of vertical well in the horizontal direction, which is easy to generate dead angle for displacement. At the same time, displacement fluid crossing different layers leads to the fast generation of dominant channels for water flows in high permeability layers, so the effect is not as good as that of model SZ3 (injection and production with horizontal wells at the same time). Through large-scale physical modelling experiment, the mechanism of development adjustment strategy for heterogeneous thick reservoir is recognized, the oil-water distribution law and seepage characteristics of different strategies in the process of water flooding are revealed, the development effect is evaluated, and the development strategy of thick reservoir in high water cut stage is optimized.
Data availability statement
The original contributions presented in the study are included in the article/supplementary material, further inquiries can be directed to the corresponding author.
Author contributions
All authors listed have made a substantial, direct, and intellectual contribution to the work and approved it for publication.
Funding
This work is supported by Science and Technology Management Department of PetroChina with funding grant number 2019B-1207 and is also supported by the Open Fund of State Key Laboratory of Enhanced Oil Recovery, PetroChina with funding grant number 2022-KFKT-29 and RIPED-2022-JS-2156.
Acknowledgments
The authors acknowledge the contributions of Hebei Normal University, State Key Laboratory of Enhanced Oil Recovery and Research Institute of Petroleum Exploration and Development, PetroChina that aided the efforts of the authors.
Conflict of interest
Authors XC, ZZ, QL, LS, HX, and JG were employed by PetroChina.
The remaining authors declare that the research was conducted in the absence of any commercial or financial relationships that could be construed as a potential conflict of interest.
The authors declare this study received funding from State Key Laboratory of Enhanced Oil Recovery, PetroChina. The funder had the following involvement with the study: study design, data analysis and preparation of the manuscript.
Publisher’s note
All claims expressed in this article are solely those of the authors and do not necessarily represent those of their affiliated organizations, or those of the publisher, the editors and the reviewers. Any product that may be evaluated in this article, or claim that may be made by its manufacturer, is not guaranteed or endorsed by the publisher.
References
Cao, D., and Liu, S. (2021). The lagging response of U.S. Shale oil production to international oil price change —phenomena, causes and future prospects. Int. Pet. Econ. 29 (12), 24–33. doi:10.3969/j.issn.1004-7298.2021.12.004
Cao, Y., and Liu, X. M. (2015). Application CT scanning technology analysis micro-flooding experiments and the residual oil. Comput. Tomogr. Theory Appl. 24 (1), 47–52. doi:10.1007/s12020-014-0354-3
Cao, Y. (2015). Study of microscopic flooding process using CT scanning technique. Sci. Technol. Eng. 15 (6), 64–68, 77. doi:10.3969/j.issn.1671-1815.2015.06.013
Chen, X. (2021). Digital management transformation of distributed CO2-EOR project: Exploration and practice. Reserv. Eval. Dev. 11 (4), 635–642, 658. doi:10.13809/j.cnki.cn32-1825/te.2021.04.021
Dang, L., He, J., Liu, M., Huang, M., and Kong, B. (2022). Innovative development of laboratory in oil and gas companies: An example from PetroChina Southwest Oil & Gasfield Company. Nat. Gas. Res. Technol. Econ. 16 (3), 71–77. doi:10.3969/j.issn.2095-1132.2022.03.011
Dou, L., Wen, Z., Wang, J., Wang, Z., He, Z., Liu, X., et al. (2022). Analysis of the world oil and gas exploration situation in 2021. Pet. Explor. Dev. 49 (5), 1195–1209. doi:10.1016/s1876-3804(22)60343-4
Du, Q., Song, B., Zhu, L., Jiang, Y., and Zhao, G. (2019). Challenges and countermeasures of the waterflooding development for Lasaxing Oilfields during extra-high watercut period. Pet. Geol. Oilfield Dev. Daqing 38 (5), 189–194. doi:10.19597/J.ISSN.1000-3754.201907004
Gao, J., Han, D., Wang, J., Wu, K., and Liu, L. (2009). Application of CT scanning image technique to study of oil saturation distribution in core displacement test. Xinjiang Pet. Geol. 30 (2), 269–271.
Guo, X. (2022). The application of relative permeability with NMR on percolation characteristics in high water cut period—taking minghuazhen reservoir of sealed coring wells in D oilfield. Petrochem. Ind. Technol. 29 (1), 172156–172173. doi:10.3969/j.issn.1006-0235.2022.01.078
He, W., Yang, T., Fei, L., Huang, X., Bao, H., and Yang, Y. (2017). 3D geological modeling for heterogeneous glutenite reservoir with viscous oil: A case of Jurassic Badaowan formation reservoir in Zhong 18 well block in Fengcheng oilfield. Complex Hydrocarb. Reserv. 10 (1), 21–26. doi:10.16181/j.cnki.fzyqc.2017.01.004
He, Z., Guo, Y., Guo, B., Wang, Y., and Bai, H. (2021). Heterogeneity of yan 91 reservoir in yang 66 wellblock of jing'an oilfield, ordos basin. J. Xi'an Shiyou Univ. Nat. Sci.) 36 (1), 37–44. doi:10.3969/j.issn.1673-064X.2021.01.005
Hou, S., Wang, Y., Rao, Z., and Zhang, C. (2020). Experimental study on deep profile control and oil displacement mechanism of high water cut reservoir. Sci. Technol. Eng. 20 (32), 13163–13167. doi:10.3969/j.issn.1671-1815.2020.32.012
Huang, J., Peng, S., and Huang, J. (2005). Application of stochastic simulation of reservoir parameters on heterogeneity in fan delta reservoir. Pet. Explor. Dev. 32 (6), 52–55. doi:10.3321/j.issn:1000-0747.2005.06.012
Li, H., Wang, C., Zhang, Y., Xu, J., Zheng, H., Zhang, E., et al. (2022). Safety and environmental risks and control measures in the oil and gas production for oilfield in beach-shallow sea. Environ. Prot. Oil Gas. Fields 32 (3), 58–62. doi:10.3969/j.issn.1005-3158.2022.03.012
Li, J., Liu, Y., Gao, Y., Cheng, B., Meng, F., and Xu, H. (2018). Effects of microscopic pore structure heterogeneity on the distribution and morphology of remaining oil. Pet. Explor. Dev. 45 (6), 1112–1122. doi:10.1016/s1876-3804(18)30114-9
Li, M., Gao, J., Zhang, L., and Wang, K. (2010). The influence of medium heterogeneity on the residual oil of reservoirs in the period of high water content and high dispersion. Prog. Geophys 25 (1), 85–89. doi:10.3969/j.issn.1004-2903.2010.01.014
Lin, Y., and Zhang, J. (2010). Laboratory experiment on flow characteristics of heterogeneous reservoir at late stage of high water cut. Pet. Geol. Oilfield Dev. Daqing 29 (5), 90–95. doi:10.3969/J.ISSN.1000-3754.2010.05.018
Liu, B., Zhang, Y., Zhang, W., and Deng, J. (2015). The vertical heterogeneity affecting of residual oil distribution in heavy oil reservoir. Sci. Technol. Eng. 15 (26), 161–164. doi:10.3969/j.issn.1671-1815.2015.26.028
Shi, C., and Wu, X. (2019). Development modes and evolution trend of Lasaxing Oilfields. Pet. Geol. Oilfield Dev. Daqing 38 (5), 45–50. doi:10.19597/J.ISSN.1000-3754.201907084
Sun, L., Wang, Z., and Pan, Y. (2017). Comparison of X-CT scanning technique and Berkeley-Villewater water-flooding theory fluid saturation distribution. Sci. Technol. Eng. 17 (32), 87–92. doi:10.3969/j.issn.1671-1815.2017.32.014
Tang, J., Jia, A., He, D., Wang, W., Fan, L., Bai, Q., et al. (2006). Development technologies for the Sulige gas field with low permeability and strong heterogeneity. Pet. Explor. Dev. 33 (1), 107–110. doi:10.3321/j.issn:1000-0747.2006.01.025
Tang, J. (2022). The energy writing in the anthropocene-on margaret Atwood's MaddAddam trilogy. J. China Univ. Pet. Soc. Sci.) 38 (3), 39–45. doi:10.13216/j.cnki.upcjess.2022.03.0006
Wang, B., Wu, Y., Liu, P., and Chen, S. (2022). Regional heterogeneity governing renewable energy development —from the perspective of industrial chain of production, transportation and consumption. J. Beijing Inst. Technol. Soc. Sci.) 24 (1), 39–50. doi:10.15918/j.jbitss1009-3370.2022.5679
Wang, G., Wang, F., Zhao, B., Sun, G., Meng, Q., Wang, Y., et al. (2021). Exploration and development situation and development strategy of Daqing Oilfield Company. China Pet. explor. 26 (1), 55–73. doi:10.3969/j.issn.1672-7703.2021.01.005
Wen, H., and Sun, N. (2011). A new quantitative description method of gas reservoir heterogeneity. Spec. Oil Gas. Reserv. 18 (1), 51–53. doi:10.3969/j.issn.1006-6535.2011.01.013
Wu, X., Wang, B., Liang, X., and Hao, Q. (2014). Produced recoveries of Lasaxing Oilfields at extra-high watercut period. Pet. Geol. Oilfield Dev. Daqing 33 (6), 52–56. doi:10.3969/J.ISSN.1000-3754.2014.06.010
Wu, Y., Wang, C., Kan, L., Ao, W., Tian, J., Fu, Y., et al. (2019). Study on the characteristics of high multiple water drive by NMR. Petrochem. Ind. Appl. 38 (12), 15–19, 35. doi:10.3969/j.issn.1673-5285.2019.12.005
Xiao, K., Mu, L., Jiang, H., Shen, J., and Zhang, P. (2017). Mechanisms of remaining oil production by plugging dominant flowing path and the application and strategies. Oil Gas Geol. 38 (6), 1180–1186. doi:10.11743/ogg20170619
Zhang, Z., Shen, X., Jones, B. H., Xu, B., Herr, J. C., and Strauss, J. F. (2008). Phosphorylation of mouse sperm axoneme central apparatus protein SPAG16L by a testis-specific kinase, TSSK2. Inn. Mong. Petrochem. Ind. 34 (8), 75–83. doi:10.1095/biolreprod.107.066308
Zhao, J., Weng, D., Chen, P., Zheng, J., Zhang, Q., and Hu, X. (2019). Experimental study on the cyclic water flooding of heterogeneous reservoir in a high water cut stage. Appl. Sci. Technol. 46 (6), 92–95. doi:10.11991/yykj.201611016
Zhou, Z., Zhang, F., Liu, B., and Liu, J. (2021). Microscopic residual oil seepage mechanism and pore throat producing characteristics in high water-cut reservoirs. Xinjiang Geol. 39 (2), 275–279. doi:10.3969/j.issn.1000-8845.2021.02.012
Keywords: non-homogeneous, development adjustment, high water-cut, physical modeling, computerized tomography
Citation: Chen X, Zhang Z, Liu Q, Sun L, Xiao H, Gao J and Kang H (2023) Physical modeling of development adjustment mechanism for heterogeneous thick oil reservoir. Front. Energy Res. 10:1094697. doi: 10.3389/fenrg.2022.1094697
Received: 10 November 2022; Accepted: 27 December 2022;
Published: 09 January 2023.
Edited by:
Xiukun Wang, China University of Petroleum, Beijing, ChinaCopyright © 2023 Chen, Zhang, Liu, Sun, Xiao, Gao and Kang. This is an open-access article distributed under the terms of the Creative Commons Attribution License (CC BY). The use, distribution or reproduction in other forums is permitted, provided the original author(s) and the copyright owner(s) are credited and that the original publication in this journal is cited, in accordance with accepted academic practice. No use, distribution or reproduction is permitted which does not comply with these terms.
*Correspondence: Hao Kang, haokang@hebtu.edu.cn