- 1School of Electrical Engineering, Shandong University, Jinan, China
- 2National Space Research and Development Agency, Abuja, Nigeria
- 3Department of Electrical Engineering, Tshwane University of Technology, Pretoria, South Africa
- 4Department of Electrical and Electronics Engineering, University of Lagos, Lagos, Nigeria
- 5Department of Electrical and Information Engineering, Covenant University, Ota, Nigeria
- 6Centre for Atmospheric Research, National Space Research and Development Agency (NASRDA), Kogi State University Campus, Anyigba, Nigeria
Sub-Saharan Africa (SSA) is home to 75% of the world’s unelectrified population, and approximately 500 million of these live in rural areas. Off-grid mini-grids are being deployed on a large scale to address the region’s electrification inequalities. This study aims to provide a comprehensive review of the research on the off-grid renewable mini-grids in SSA. The study covers the current status of the level of deployment of off-grid mini-grids. It also reviews multi-criteria decision-making models for optimizing engineering, economics, and management interests in mini-grid siting and design in SSA. The statuses of financing, policy, and tariffs for mini-grids in SSA are also studied. Finally, the current status of energy justice research in respect of mini-grids in SSA is reviewed. The study shows the important role of decentralized renewable technologies in the electrification of SSA’s rural population. Within a decade since 2010, the rural electrification rate of SSA has increased from 17% to 28%, and 11 million mini-grid connections are currently operational. Despite these gains, the literature points to several injustices related to the present model by which SSA’s renewable mini-grids are funded, deployed, and operated. Hence, several recommendations are provided for the effective application of the energy justice framework (EJF) for just and equitable mini-grids in SSA.
1 Introduction
In September 2015, World Leaders ratified the United Nations Sustainable Development Goals (SDG). Among these, SDG7 has a target to “achieve universal access to affordable, reliable and modern energy services by 2030.” This target has a unique alignment with the needs of Sub-Saharan Africa (SSA), where 60% of the population reside in rural areas. According to the SDG7 Tracking Report (Results, 2020), as of 2020, although the global electrification rate is 91%, SSA’s electrification rate is 48% (up from 33% in 2010), which is the lowest among all other regions in the world. SSA’s 2020 rural electrification rate is 28% (up from 17% in 2010), and the urban electrification rate is 78% (up from 86% in 2010). (The SDG7 Tracking Report data are a joint output of the International Energy Agency (IEA), International Renewable Energy Agency (IRENA), United Nations Statistics Division, the World Bank, and World Health Organization.) Apart from the low rural electrification rate, SSA also has a high youth population who form the core of the region’s future human resources. Thus, fair attention needs to be provided to the rural and urban populations. In this regard, mini-grids can help achieve SDG7.
Mini-grids have the potential to electrify off-grid communities while avoiding the high economic burdens associated with extending the grid to locations with low-population density and relatively low electrical energy demand (Come Zebra et al., 2021). Mini-grids are independent electrical networks of electrical loads which are powered by independent electrical sources. They have power ratings up to 10 MW and can flexibly operate in stand-alone and grid-connected modes. Three forms of mini-grids can be identified in the literature: fossil-based mini-grids, renewable-based mini-grids, and hybrid renewable mini-grids (BloombergNEF and SEforALL, 2020). Fossil-based mini-grids are powered by generators fueled mainly by diesel. Renewable-based mini-grids are powered by renewable sources of energy such as solar PV, wind, and small hydro. Hybrid renewable mini-grids are powered by a combination of fossil-fuels and renewable sources as a means to improve their reliability and cost-effectiveness.
In the past decade, significant efforts have been made to electrify rural areas, and 11 million mini-grid connections are now available in SSA (Domegni and Azouma, 2022). As of 2020, an estimated 2,200 mini-grids were operational in SSA, and the majority of these are powered by solar PV (Domegni and Azouma, 2022; USAID, 2022). A total of 32% of globally installed mini-grids operate on diesel, heavy oil, and hydropower (Domegni and Azouma, 2022). Due to the intermittency of generation, solar mini-grids require energy storage. Lead–acid batteries account for 66% of installed mini-grids due to lower capital and installation costs, whereas 32% use lithium–ion batteries (Domegni and Azouma, 2022). The mini-grid market is projected to impact 111 million households by 2030 (Domegni and Azouma, 2022). This requires the appropriate policies to overcome existing challenges with accessing performance and upfront subsidies, lengthy bureaucratic procedures to secure operational licenses, non-cost reflective tariffs, and uncertain grid-arrival regulations.
It is estimated that nearly 60% of Africans reside in rural communities, and approximately 5% of these people have access to clean electricity. One of the major causes of low electrification rates in rural African communities is the proximity to the existing grid infrastructure, sparsely distributed settlements and low population density, and the presence of low socio-economic activities. Critical to extending electricity access to these remote communities is the deployment of mini-grid technologies. As such, electrification policies in Africa are now redirecting focus to the proliferation of mini-grid facilities not only for the rural communities but also for the peri-urban and urban areas that are underserved (Akinyele et al., 2022). Governments, private sectors, and researchers at various levels are now dedicating efforts to unlocking the potentials embedded in mini-grid technologies to accomplish the attainment of SDG7. This is made possible because of the cost-effectiveness of various renewable energy (RE) technologies and the increase in the efficiency of these technologies (Magenta Global, 2021).
It is said that Africa is home to a large number of planned mini-grid initiatives globally. Data available from World Bank indicate that over 4,000 mini-grid facilities are currently in the planning stage on the African continent (Schwerhoff and Sy, 2019). Based on a market survey, the energy sectors of Nigeria and Senegal were identified as the most promising because they account for 40% of the planned mini-grid initiatives on the continent (Schwerhoff and Sy, 2019). Enabling policies and frameworks that can support the integration of mini-grids into the energy sectors in Africa have also been developed in Ghana, Nigeria, Uganda, Kenya, and Tanzania (Schwerhoff and Sy, 2019). This is expected to create an enabling environment for financiers to participate in emerging development.
The literature is replete with studies on techno-economic performance (Feron, 2016; Morstyn et al., 2018) and size optimization of the mini-grid systems (Gambino et al., 2019; Azimoh et al., 2016). Details on these studies are summarized in Table A1. Countries covered include South Africa, Tanzania, Mali, Burkina Faso, Togo, Uganda, Kenya, Namibia, Cameroon, and Ghana. The viability assessment of hybrid mini-grids for the electrification and development of two rural South African communities (Odarno et al., 2017; (Azimoh et al., 2017) provides insights into the cost frameworks of PV/hybrid mini-grid facilities for SSA. Eder et al. (2015) explored the contribution of mini-grids to electricity access in West Africa. Antonanzas-Torres et al. (2021a) analyzed the elements that impact the adoption of renewable electricity in Ugandan households. Pillot et al. (2019) explored the environmental life cycle impact assessment of mini-grids in West Africa. A review of hybrid renewable mini-grids for developing countries was reported by BloombergNEF and SEforALL (2020). Due to its broad global scope, several details on the specific status of mini-grids, challenges, solutions, and policies that affect SSA were left out. A historical review of solar PV systems in SSA was done by Antonanzas-Torres et al. (2021b). However, it focused on the impact of world-energy policy on the failure and successes of mini-grid projects in SSA. In addition, funding agencies have commissioned reports on the global state of mini-grids (Domegni and Azouma, 2022). Nonetheless, these focus on providing investors and funders with insights into the potential profitability of the min-grid market. These studies do not clarify policy status, optimal multi-criteria decision-making, or the recent energy-justice framework for off-grid mini-grids in SSA.
Motivated by these research gaps, this study provides a comprehensive review of the literature on renewable off-grid mini-grids in SSA. The key research questions answered in this study include the following:
• What is the status of the actual deployment of renewable off-grid mini-grids in SSA?
• What multi-criteria decision-making models can be used to optimize engineering, economics, and management interests in mini-grid siting and design in SSA?
• What are the financing and tariff trends for off-grid mini-grids in SSA?
• How is energy justice applied to off-grid mini-grids in SSA?
The current study is organized as follows: Section 2 discusses renewable mini-grid technologies in SSA. Section 3 reviews multi-criteria decision-making models for mini-grids. Section 4 discusses the challenges associated with mini-grid projects in SSA. Section 5 reviews finance and tariff with respect to SSA mini-grids. Section 6 discusses the progress made with policy for off-grid mini-grids across SSA. Section 7 presents cases of mini-grid progress in West, East, and Southern Africa. Section 8 discusses energy-justice themes with respect to SSA’s mini-grids, and Section 9 concludes the article.
2 Renewable Energy sources and technologies for mini-grids in SSA
The local availability of renewable energy sources in SSA has a significant influence on the energy sources deployed in an off-grid community. At present, solar energy is the dominant renewable energy source for off-grid mini-grids in SSA (Paliwal et al., 2014). Among more than 7,000 mini-grids in Asia and Africa, 63% were solar or solar hybrid systems, 21% hydro, 11% diesel/heavy fuel oil, and 3% biomass (Domegni and Azouma, 2022). Hybridization of different energy sources with respect to techno-economical and available resources is essential for sustainable electrification of SSA to attain energy access goals.
Figure 1 shows various RES technologies in different categories (Korkovelos et al., 2020). The electrical-based storages are not generation sources; they provide efficient and continuous utilization of power generated when energy sources are temporarily unavailable. The principles of operation of these technologies are given in Table 1. Energy stored in any storage technology is mainly used when energy sources are unavailable or during high tariff rates. The differences in these storage technologies are their sizes, storage methods, and types of stored energy.
The size of the mini-grid system is dependent on the amount of demanded energy. It usually combines multiple energy sources, including gas, water, solar, biomass, tidal, and geothermal. The renewable types of energy sources often make use of storage systems that are usually incorporated (e.g., batteries, flywheels, and capacitors) to guard against intermittent energy supply associated with renewable energy sources (RES) and also aid the reliable supply of electricity (Pedersen and Nygaard, 2018; Bhattacharyya, 2018; Shrestha et al., 2020). Mini-grid systems can stand alone to supply electricity to rural dwellers or be integrated into national grid systems to serve either as an additional generating unit (hybrid mode of operation) to supply electric power to the national grids or as distribution franchise reselling electric power from national grids to the rural dwellers due to the increase in their load demand. This type of configuration has been explained in several open studies (BloombergNEF and SEforALL, 2020; Korkovelos et al., 2020) but not properly captured, as depicted in Figure 2. By this integration, the power system operation becomes more complex as the mini-grid system should be able to be isolated from the national grid system (which is usually referred to as island mode) in case of faults from any of the systems or networks.
The mini-grid system can serve as a building block for several modern decentralized electricity systems in SSA (Duran and Sahinyazan, 2021). It can serve diverse electricity consumers, including homes, commercial buildings, farms, and industries. The mini-grid’s operation and control are much easier and more flexible than those of the main grid due to lesser interconnectivity. Faults in the system and network can be attended to more easily, and energy theft can easily be identified and curbed. With the present era of power generation company deregulation, different persons or groups can own and manage mini-grid systems (Bhattacharyya, 2018). They can be owned by individuals, state utilities, private companies, or communities.
Mini-grid system technologies are becoming more advanced in terms of reliability and control, making the integration of mini-grids into national grids possible. Likewise, the improvement achieved by manufacturers of these technologies has led to the general acceptance of renewable energy sources for electric power generation by most countries (including SSA) because they are practical, cost-effective, and environmentally pollution-free (Moner-Girona et al., 2016). With these technologies, rural electrification can be easier to implement compared to the main grid extension because extending the main grid system will be more expensive than setting up a mini-grid system (Pedersen, 2016). Moreover, it is important to note that the grid extension approach is a long-term project that spans several decades, considering the level of development in the major towns and cities. This means that without the off-grid power interventions, most communities would remain in the dark even with gas-fired power additions to the central grid.
3 Optimal planning, sizing, and technology selection for mini-grids
In many cases, stakeholders in developing countries adopt top-bottom and bottom-top approaches for electrification purposes. While electrifying the urban and semi-urban through grid extension, mini-grid energy services are being proposed and deployed for rural and remote electricity consumers. However, many of these mini-grids have not been able to attain the standard and scale required to increase electrification considerably due to various reasons related to sustainability. Mini-grids designed for electrification purposes must be reliable (technical), cost-effective (economic), environmentally viable, and socially acceptable, with relevant policies to back them up (Babatunde et al., 2019a). As such, the design, operations, and planning of mini-grids are a multi-criteria problem in which a balance must be attained between conflicting objectives related to engineering, economics, and management (Somefun et al., 2022). To mitigate the multi-criteria challenges related to mini-grid proliferation, stakeholders must choose the right sizing and implementation tools; they must have a robust understanding of the energy needs. Moreover, it is expected that designers should know the optimal location of mini-grids based on the availability of resources and analyze the affordability of the technologies; this is the focus of this section.
3.1 Mini-grid planning tools
The design and sizing of a mini-grid largely depend on the adopted tools and methodologies. If not properly designed and optimally sized, it may lead to oversizing, which would eventually place an economic burden on the consumers, or undersizing, which would precipitate reliability challenges. Therefore, the choice of the right tools or methodologies for the design and sizing of mini-grids is essential (Denwigwe et al., 2021). Traditionally, the pen and paper approach (rule of thumb method) to the sizing and design of mini-grids may be sufficient for a single source system. However, as the complexity of the system increases, this method may produce a sub-optimal result considering various sustainability factors. Therefore, various computer-aided simulation tools have been developed for the design and optimal sizing of mini-grids. Mobile phone applications are also being developed for the sizing of mini-grids (Turcotte et al., 2001). Based on the literature, these sizing and simulation tools have been classified as open architecture research tools, pre-feasibility, simulation, and sizing (Kemausuor et al., 2018). Kemausuor et al. (2018) attempted to classify mini-grid planning tools into the design of production, network design, and territorial planning tools. However, based on another classification, energy system and sizing tools are classified as universal and global sizing tools, island energy-system tools, a single project or local community-based sizing tools, national energy-system tools, and tools that can handle all scales; examples of tools in these categories are given in Table 2 (Ajeigbe et al., 2020).
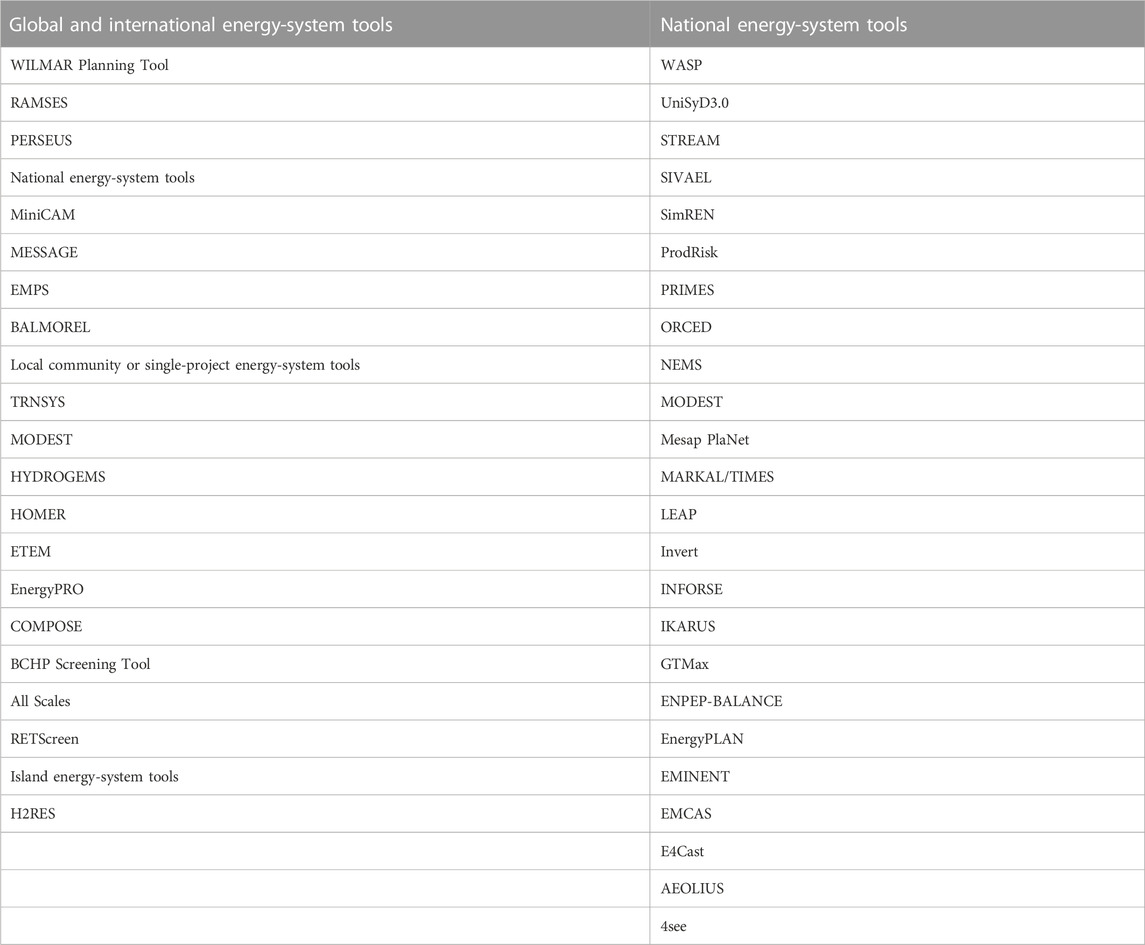
TABLE 2. Classification based on global sizing tools, island energy-system tools, single-project or local community-based sizing tools, national energy-system tools, and tools that can handle all scales (Ajeigbe et al., 2020).
According to the explanation offered by Turcotte et al. (2001), prefeasibility modeling tools are used for the business case and initial assessment of mini-grids. These include technical and financial evaluations. As for the sizing tools, they provide the optimal combination of the sizes of each system component that satisfies the load. They also provide comprehensive information about the energy flow of viable energy systems. While open architecture research software enables users to adjust the communications and algorithms of the individual components of the energy system, simulation tools provide the option of specifying the features of the components to obtain the technical characteristics of the entire system. A summary of some of the prominent tools used for mini-grid design and planning is presented in Table 3. Both classical and meta-heuristic techniques have also been extensively explored for the sizing of mini-grids; more details are discussed in the literature (Dawoud et al., 2018; Baring-Gould et al., 1996).
3.2 Community needs assessment
During the conceptual stages of mini-grid projects, planners and other stakeholders are tasked with the responsibility of identifying the various needs of the communities. These needs are essential to the sustainability of such projects. Community needs appraisal is important to mini-grid developers because it helps them understand the technical, capacity, and energy needs of the community. Based on the role paid by a community, these needs usually vary from one community to another. For instance, ownership can play a role in the needs of a community; a community that does not wish to take ownership or maintain or operate a mini-grid would not need investments in local technical expertise.
3.3 Energy needs assessment
In order to determine the energy needs of a community, stakeholders are typically faced with questions related to the quality of electricity consumed, electricity use, electricity use pattern, and future growth of energy demand. Obtaining the present and expected load growth for the host community is an essential part of energy needs assessment and helps reduce uncertainties (Nsafon et al., 2020). Uncertainty with regard to energy needs can lead to over- or undersizing of the mini-grid, which may eventually lead to dissatisfaction on the part of the customers (Katre et al., 2019). Developers are expected to estimate the amount of energy the community would consume, what the energy would be used for, the pattern of consumption, and the anticipated growth in energy demand. These figures usually depend on the socio-economic status of the residents in the host community (Babatunde et al., 2019a; Ighravwe and Babatunde, 2018a). In cases where grid expansion is expected to reach the host community in the future, the mini-grid facility is cited at the closest proximity to the central grid. Besides elucidating the need for mini-grid or otherwise, energy needs assessment helps developers identify the best power generation technologies, the optimal sizes of the components, the cost of electricity, and the best revenue collection method (N R E C Association and others, 2019). With energy needs assessments, consumers can be classified according to the type of load, which helps in the development of a reflective tariff system.
While developing energy needs assessments, it is important to identify potential economically intensive activities and productive use of electricity that is present or may be put in place in the future. Thus, if the dynamics of the energy demand is known over the planning period, developers and utilities in mini-grid business would be able to set realistic economic and technical targets for the future of the business. A summary of the outputs offered by energy needs assessment is provided in Figure 3.
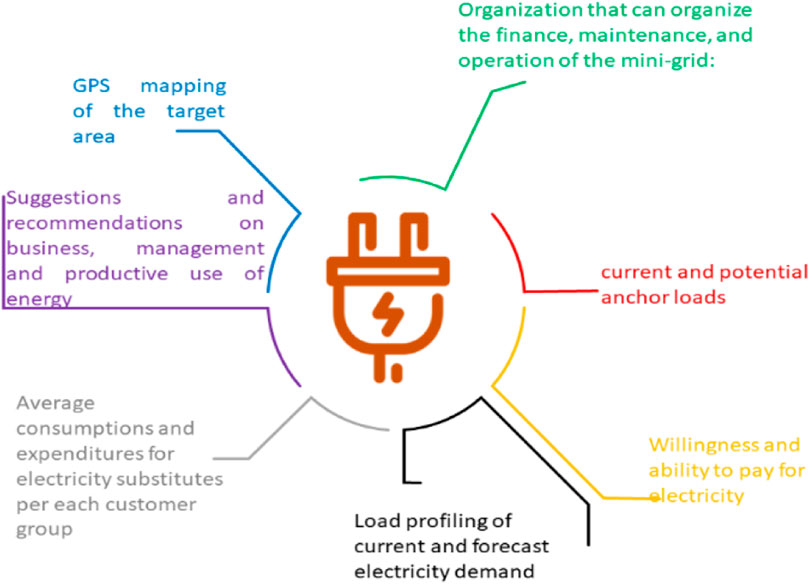
FIGURE 3. A summary of the outputs offered by energy needs assessment (Nsafon et al., 2020).
3.3.1 Energy demand estimation and projection
Typically, apart from residential needs, mini-grids may serve factory loads, commercial loads, schools, agricultural loads, municipal loads, hospitals, and any projected investments in those classifications. To project future demand, developers must access historical data related to population growth, the number of consumers, and the electricity use per consumer. To access future needs, it is also important to project future energy-consuming businesses, emerging technologies that can accelerate energy demand, and the state of infrastructure.
In estimating demand, the first step is to categorize the various consumers, followed by predicting the growth of the consumers based on their classification (Nkiriki and Ustun, 2017). Growth can either be in the form of energy consumption or the number of consumers. Municipal loads, such as public street lighting, are also included in the analysis. In remote communities without electricity access, the energy needs of residential consumers are carried out based on the willingness of the consumers to pay. The willingness to pay is a survey that estimates the present energy consumption (non-electric and electric) (Nkiriki and Ustun, 2017). The two components are then added to arrive at an equivalent electricity consumption. When the categories of consumers and the various energy-consuming equipment are known, the energy demand can be obtained using the following equation (Babatunde et al., 2019a):
where
3.4 Capacity needs assessment
As for the capacity needs, developers must identify the administrative-related needs, technical expertise, financial needs, and institutional requirements that may hamper the construction and smooth operation of the mini-grid facility in the host community. This would help in the sustainability of the project with regard to tariff collection, conflict resolution, facility vandalization, and technical expertise (Niyonteze et al., 2019; Hartvigsson et al., 2021). Other capacity needs that developers must identify are the ones related to the various ways residents use electricity. Some of these include demand-side activities and specific productive use of electricity (Ramakumar et al., 1986). The creation of awareness and sensitization of the community through education workshops are also some of the ways to build community capacity.
3.5 Mini-grid sizing
The sizing of a mini-grid system is typically an optimization problem. It involves sizing various components of the system so that the power generated by the entire system meets the load connected to the system. The mini-grid energy generated by the energy sources must be dispatched such that the reliability of the energy system is not compromised. Depending on the motivation, the sizing of a mini-grid system involves maximizing or minimizing a multi- or single-objective function subject to various constraints (optional or compulsory):
Some of the objective functions, constraints, and types of energy sources adopted in the literature are presented in Table 4. It could be inferred that the most common objective revolves around the economic/financial aspect of the project.
3.6 Multi-criteria perspectives in mini-grid planning
When planning for mini-grid development, certain evaluation metrics are considered. These are meant to ensure the sustainability of the projects. As earlier stated, these metrics assist the stakeholders involved in the planning and implementation of the project to make informed decisions. Depending on the objective of the mini-grid project, performance metrics are usually economic, technical (most especially reliability), environmental, social, policy, and legal (Greco et al., 2016). The economic metrics are related to the profitability and affordability of the project. The social metrics measure how bearable the proposed system is, whereas the environmental metrics measure the influence of the system on the host community. Examples of performance indicators are presented in Table 5.
The boundaries of these indicators usually overlap, thereby making decisions as regards the best mini-grid alternative complex. For instance, it could be cheap to install a fossil-powered generator because of its lower initial cost compared to solar PV. However, from the environmental perspective, more emissions are attributed to the operations of the fossil-powered generator, while none is attributed to that of the PV. Choosing between the two mini-grid options becomes a complex decision. Moreover, in the selection of the most preferred mini-grid alternative, decision-makers are usually faced with quantitative and qualitative indicators. While it is easy to measure the quantitative indicators, defining the extent of the qualitative indicators is usually complex. Since renewable energy-powered mini-grids are typically dependent on meteorological parameters and their availability at any time, decision-makers are faced with the dilemma of identifying the best sites. Mini-grid site locations also depend on proximity to existing infrastructures and land use.
Various multi-criteria decision-making (MCDM) frameworks have been developed to address these types of challenges, including TOPSIS, AHP, VIKOR, PROMETHEE, ARAS, EDAS, SAASPAS, MOORA, COPRAS, DEMATEL, and SWARA (Ighravwe and Babatunde, 2018b). For instance, to improve the sustainability of mini-grids, researchers have explored the use of MCDM in addressing various issues. Researchers have applied CRITIC-TOPSIS with interval type-2 fuzzy sets to prioritize the mini-grid business model that is applicable to the Nigerian energy sector (Pohekar and Ramachandran, 2004). The result from the study indicates that the most preferred model based on the 12 criteria identified by the authors is the private business model. In order to improve the planning process of mini-grids, another study used AHP-VIKOR with a Plan-Do-Check-Act cycle to rank hybrid renewable energy alternatives (Azimoh et al., 2016). A detailed review of the applications of MCDM in mini-grid developments, energy policy, and energy planning has been presented in the literature.
3.7 Mini-grid siting using geospatial analysis
The hybridization of the MCDM and GIS has been identified as a very valuable method to analytically harness available geographical information or meteorological data and to employ criteria importance in specifying the optimal sites for mini-grid implementation. As such, because of its visualization capabilities and high spatial resolution, more recently, the geographical information system (GIS) has been hybridized with MCDM approaches to select the best site for the location of mini-grid projects. Although potential sites for the implementation of mini-grids can be anchored on the factors discussed in Table 5 (social, technical, economic, environmental, and policy), geographical attributes, such as slope and proximity to major infrastructure, are also important factors that must be considered. Consequently, the integration of GIS MCDM tools has become effective in proposing solutions to the multifaceted dilemma of optimal site selection for mini-grid projects. The GIS-MCDM approach has found application in the wind and solar form siting. For instance, Ayodele et al. (2018) proposed a GIS-MCDM approach based on interval type-2 fuzzy analytic hierarchy to assess the most preferred site for the implementation of wind farms across Nigeria. Moreover, Al Garni and Awasthi (2017) applied the GIS-AHP approach to the selection of the best site for a solar PV power plant in Saudi Arabia. Other examples of cases where GIS and MCDM approaches have been deployed for energy planning in Africa are given in Table 6.
4 Challenges with off-grid mini-grid projects in SSA
In this section, challenges associated with mini-grid projects are discussed, as well as solutions proposed in the literature.
4.1 Poor community participation
When a community cannot buy into a social project, such a social venture is set up for failure ab initio. In situations such as this, there will be conflict over issues such as siting of the project, security of the project, and community part-financing the project via contributing free/minimized manual labor for stages such as site clearing. It is vital to engage the support of the entire community as much as possible through agencies of mediation and consultation that can facilitate communal interactions, including community leaders, traditional rulers, community development associations (CDAs), and local government and town hall meetings (Peters et al., 2019).
The question of who owns the mini-grid project must be settled from the point of conception. The research has indicated that the method used by donors and donor agencies to simply install energy generation systems without plans for long-term sustainability does not work in the end. For instance, solar installations that have the potential to last for 20 years do not survive after the third year after installation and commissioning (Babayomi and Okharedia, 2019). Some other challenges associated with community participation include a lack of clearly defined commercial/community ownership structure, infrastructural insecurity, and community illiteracy on sustainable energy practices. Hence, solutions to these challenges include effective community participation, clearly defined commercial/community ownership structure, collaborating with community security groups, and public energy literacy and advocacy campaigns (Peters et al., 2019; AMDA and ECA, 2020).
4.2 Economic challenges
This relates to subsisting poverty in spite of electricity access. The average monthly energy consumed by each connected off-grid mini-grid user in SSA is only 6.1 kWh (Nygaard et al., 2015). To put this in context, this amount of energy can only power a 65 W laptop charger for only 3 h daily. When residents do not have a compelling reason to subscribe to electricity plans, it can limit its impact and even sustainability in the rural area supplied. Other challenges include revenue generation problems, macroeconomic economic instability, exchange rate fluctuations, and high operational and maintenance costs. A lack of government cash guarantees and high import taxes also hamper mini-grid development. Their policy even supports developers with insurance in case of political instability. A tax-free import regime on PV panels, like the practice in Kenya, is more favorable to mini-grid businesses (Ohunakin et al., 2014). Furthermore, for components that government is unwilling to give tax-free classification (probably due to their use by non-mini-grid entities too), lower taxes will be a boon (Peters et al., 2019; AMDA and ECA, 2020).
4.3 Technical challenges
Several remote communities had no prior data on electricity consumption. It is sometimes difficult to carry out energy forecasts, for instance, the village size, prior adoption of mobile phones, how much the mobile phone is used, and the nature of commercial activities in the community. There is a paucity of skilled technical workers and a void of standardization and certifications in the mini-grid and hybrid renewable energy sectors (Peters et al., 2019). A lack of feasibility studies prior to the commencement of the project also leaves the project vulnerable to circumstances and factors that could result in premature failure. These challenges can be overcome by leveraging technology to improve energy forecast accuracies and engage engineers and technologists with a track record of professionalism, bespoke feasibility surveys, regular maintenance procedures, government-led oversight and standardization, and use of quality-certified equipment and components (Peters et al., 2019; AMDA and ECA, 2020).
4.4 Environmental challenges
The lack of detailed mapping of local renewable energy resources that form inputs to the mini-grid system makes cost-minimization difficult. A resource map available at a country level and spread across all the remote communities will enable a correct mapping of energy resources to mini-grid projects. The International Renewable Energy Agency (IRENA) has published data from Geographic Information Systems (GIS) data to prepare a country-by-country mapping of renewable energy technology resources for solar energy, wind energy, and bioenergy production (Morstyn et al., 2018). There is a lack of impact assessment reports on how renewable energy technology sources affect the local environment. For instance, wind energy turbines impact the skyline, and when there are many of them, they cause noise pollution. Furthermore, there could be a need to clear vegetation or a forest to accommodate a large amount of energy equipment such as solar panels or wind turbine farms. The details in the energy impact assessment at each location should form a significant determinant of the type of renewable energy system deployed there (Peters et al., 2019; AMDA and ECA, 2020).
4.5 Policy and political challenges
Traditionally, power investments and developments in SSA have been the prerogative of government electricity agencies or parastatals. Therefore, in climes where there is still heavy government involvement, the focus on traditional large-scale electrification projects to the exclusion of decentralized electrification is rampant. Some of this stems from skills and knowledge gaps at the top echelon of government. The weak political will of the government (Monyei and Akpeji, 2020) stems largely from the poor quality of energy advisers in the government. For instance, due to bureaucratic inefficiencies, it takes an average of 17 months to secure regulatory license approvals to operate mini-grids in SSA (Nygaard et al., 2015). Moreover, a lack of a grid-creep protection policy causes uncertainties about the future fate of rural mini-grids. This apprehension makes major private investments in the industry high risk and, hence, high cost. Policies, including mini-grid or renewable feed-in tariffs, can help in such situations (Pedersen, 2016). Additional related challenges include a lack of tariff-subsidy regulations and ineffective quality control implementation. Some tariff-subsidy policies required to boost the penetration and proliferation of mini-grids in SSA include feed-in tariffs and feed-in premiums (Babayomi and Okharedia, 2019). These also provide mini-grid operators additional income and security when the grid is later extended to their remote locations.
Table 7 summarizes the sustainable mini-grid framework to combat the problem of failed mini-grids in SSA.
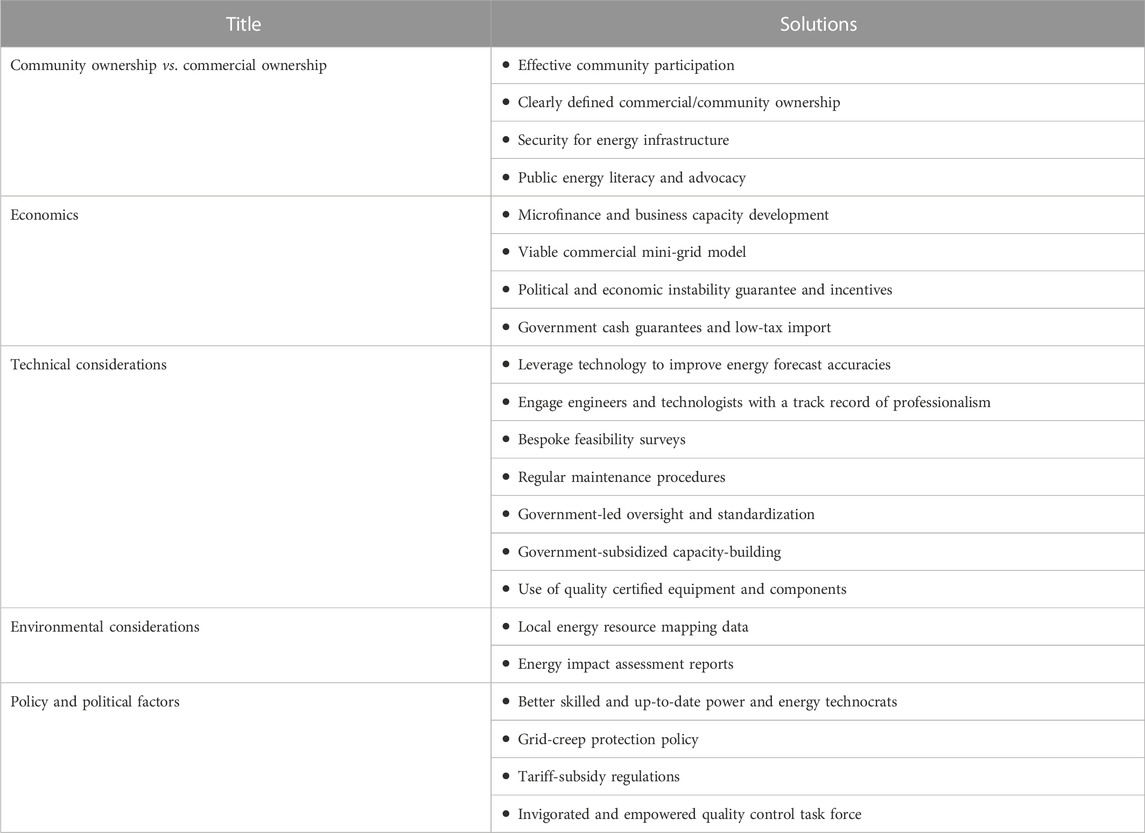
TABLE 7. Challenges to mini-grid developments in Sub-Saharan Africa and corresponding solutions (Peters et al., 2019; Boait, 2014).
5 Finance and tariffs for SSA mini-grids
This section discusses the finance and tariffs of mini-grids in SSA.
5.1 Finance
Mini-grid projects are funded through loans, equities, grants, and subsidies (Aziz et al., 2016). These funds are sourced from corporate entities, foundations, and government agencies.
Selecting financial means and types of investment for funding mini-grids depends on the mini-grid type and the development stage of the project. The earlier the stage, the riskier the project, and the later the stage, the less risky the project (Zhang et al., 2020).
Most mini-grids in SSA heavily rely on grants and subsidies for at least 30% of the cost used for investment. The grants and subsidies are majorly used for piloting the mini-grid project, the initial operational needs of the projects, and investing in capital and technical engagements. They are usually sourced from local agencies, private individuals, international agencies, trust funds, and foundations (von Breitenstein, 1999).
Equity is another means by which mini-grids in SSA are financed. Equity investors are a very good means of financing mini-grid investments. Equity investors include Angel investors and venture capitalists (who provide initial capital for the development and implementation of mini-grid projects), private equity (for expansion of capital investments), impact investors (who decide to provide initial or final capital investment depending on the investor, and the social and financial return of the project), and development financing institutions (DFIs) that provide equity investments directly or through third-party funding streams. DFIs look for potentials in the form of development impact and project viability before making financial commitments (Jonker Klunne, 1020; Nuru et al., 2021; Bukari et al., 2021; Antonanzas-Torres et al., 2021c).
Loans become necessary when grants, subsidies, and equities are unavailable. DFIs are the most common sources of loan provision in SSA. They offer loans that are concessional and lower-priced than other commercial lenders. However, the high transaction cost for DFIs does not suit the operations of small mini-grids. Local commercial banks categorize the business models of mini-grids as very high risks; they either do not lend at all or offer prohibitive lending rate terms and conditions (Jonker Klunne, 1020; Nuru et al., 2021). International lenders avoid mini-grid transactions, too, owing to their relatively small value and foreign exchange risks (Jonker Klunne, 1020; Nuru et al., 2021; Bukari et al., 2021; Antonanzas-Torres et al., 2021c).
Guarantees are used to ensure that financing mini-grids are successful by improving the debit or credit worthiness of the mini-grid project. This serves as an assurance to investors that mini-grids can meet their profit expectations. Two types of financial guarantee exist; loan guarantee, which covers repayment of loans in full and in due time, and risk guarantee, which covers the full or part repayment of loans (Jonker Klunne, 1020; Nuru et al., 2021; Bukari et al., 2021; Antonanzas-Torres et al., 2021c).
Foreign exchange risk is a major challenge faced by developers of mini-grids in SSA. The capital cost of mini-grid projects is usually in foreign currency, whereas the revenues are in local currency. There is usually a currency mismatch between capital investments and revenue whenever the local currency loses its value against the foreign currency. Projects funded in foreign currency, such as dollars, pounds, and euros, are usually affected by this currency mismatch (Pillot et al., 2019; Ihirwe et al., 2021).
In 2008, the Tanzanian government developed a framework to encourage investments in decentralized electrification. This led to an increase in the number of mini-grid investments in the country. Since that period, private businesses and local communities now conduct their activities using mini-grid systems (Booth et al., 2018). The framework of small power producers (SPP) designed by the Tanzanian government in 2008 allowed facilities generating electricity of less than 10 MW from renewable sources to sell power to the country’s main grid as retailers and wholesalers as a means of funding the operations of the facilities. In order to support the development of mini-grids, the Tanzania Rural Electrification Agency (REA) created the Tanzania Energy Development and Access Project (TEDAP) supported by the World Bank, offering grants to develop mini-grid projects in Tanzania. The World Bank also came up with a $23 million loan scheme to support the government-owned Tanzania Investment Bank for the delivery of 15-year loan facilities to local commercial banks, which was to be used for the development of mini-grid projects in Tanzania (Booth et al., 2018). Although the loan scheme has benefited mini-grid projects, many developers have had trouble accessing the funds. Despite funding support received by the Tanzanian government from other international donors in support of activities for the development of mini-grid projects, developers remain concerned about the unknown risks involved in mini-grid investment (Nfah and Ngundam, 2012).
Figure 4 and Table 8 show the number of funds obtained from result-based financing schemes to support mini-grid development in East Africa since 2013.
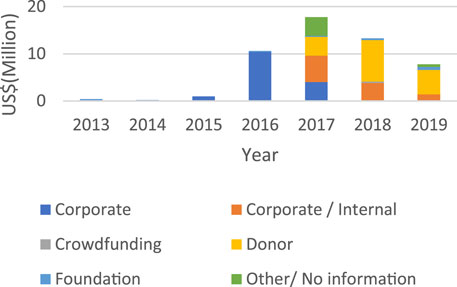
FIGURE 4. Comparison of funding obtained from different funding types (Eales and Unyolo, 2018).
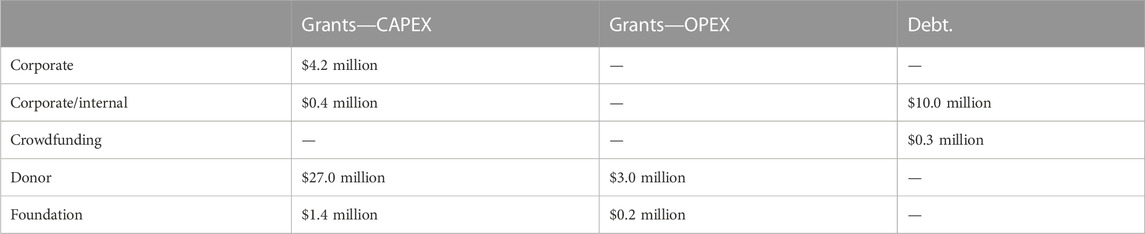
TABLE 8. Funding support received through corporate expenditures and operation expenses (Nygaard et al., 2015).
Figure 4 shows that the year 2017 experienced the highest amount of funds (with more than $17 million) distributed for financing mini-grid projects. Funding for that year came from foundations, donor agencies, crowdfunding sources, and corporate organizations. The years 2013 and 2014 experienced the lowest amounts of funding, which came only from corporate organizations and foundations. Figure 4 shows that funding increased from 2014 to 2017 before experiencing a decrease in 2018. The increase can be linked to good returns on investment, which increases the confidence of funders, whereas the decrease can be linked to poor returns on investment, which could be caused by mismanagement or unfavorable government policies leading to a decrease in the confidence of funders.
In Table 8, grant capital expenditure (CAPEX) refers to grants in the form of capital expenditures (in the form of building sites, provision and installation of panels, generators, meters, and batteries), whereas grant operating expenditure (OPEX) refers to grants in the form of operating costs and expenses (research and development, consultancy, capacity building, and marketing). It can be observed that corporate organizations provide grants only in the form of capital expenditures, whereas donor agencies and foundations provide grants in the form of capital expenditures and operating costs. In terms of capital expenditure, donor agencies provide the highest amount.
Figure 4 and Table 8 show the importance of funding in the development of mini-grid projects and initiatives.
Figure 5 shows the amount of funding received by the different regions in the African continent.
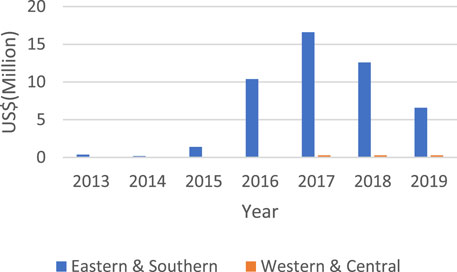
FIGURE 5. Funding obtained by different regions in Africa (Nygaard et al., 2015).
From Figure 5, Western and Central Africa received no funding from 2013 to 2016 while receiving a small amount of funding below $1 million from 2017 to 2019. East and Southern Africa received funding support from 2013 to 2019. Funding for East and Southern Africa increased from 2014 to 2017 and experienced a decrease in 2018 and 2019. The year 2017 showed the highest amount of funding support to regions in Africa.
5.2 Tariffs
Some key drivers influence tariff plans for mini-grids in SSA. For effectiveness and sustainability, any tariff structure must adequately balance the needs of three key groups of stakeholders: governments, developers, and customers (Allee, 2021).
• Government: Composed of ministries of energy, government regulatory agencies, and banks who are responsible for the creation and implementation of energy policy, enforcement of regulations, and provision of financial and funding instruments for implementation of mini-grid projects.
• Developers: Oversee all activities regarding planning, construction, commissioning, operation, management, and revenue collection for the mini-grid project. They are held responsible by financial donors for all expenses made during project implementation. They ensure that all financial requirements put in place by donor agencies are met during project implementation.
• Customers: Typically do not have direct control over tariffs, but their concerns and subsequent decisions influence the considerations of both governments and developers. For example, their perceptions of and willingness to pay tariffs and fees can have political impacts on governments and real impacts on system design, customer connection rates, load growth, and financing considerations.
Creation and regulation of tariff plans can be achieved with the use of different approaches. Some of these approaches include the following:
i) Uniform tariff: In this type of structure, all mini-grid consumers are billed the same way without consideration of the utility type of the grid (which could be rural utility, urban utility, or isolated type of grid) they are connected to. This type of tariff plan ensures fairness and equity in utility distribution amongst customers. The government subsidizes electricity rates to ensure that electricity prices are cheap and affordable (Korzhenevych and Owusu, 2021; Reber and Booth, 2018).
ii) Cost-reflective tariff: In this tariff plan, mini-grid developers freely set their own tariff and payment plans at high levels that enable them to recover their capital and operational expenses directly from the consumers. In several situations, the tariff plans may also be subject to approval by a regulatory body or the government. Cost-reflective tariffs are usually higher than the rates that existing grid customers are charged; however, these charges are decided via negotiations between customers and micro-grid operators where both parties agree on a rate suitable for the customers while allowing the operators to recover all expenses and incurred cost (if this arrangement does not exist, this could lead to a potential failure in the micro-grid development). Most times, the micro-grid developers charge cost-reflective rates, which are lower than the price unconnected customers would pay for energy generation in the form of kerosene, candles, batteries, or other fuels (Antonanzas-Torres et al., 2021c; Akpan et al., 2013; U.S. Agency et al., 2020).
iii) Bid Tariff: In this tariff plan, prices are set at the lowest price by mini-grid developers in a competitive and efficient bidding process involving retailers who push down prices to consumers (Léautier, 2001). Prices generated from bids provide good solutions to the problem of defining the prudent cost of electricity that would be passed to consumers. This is a good strategy that could be used in procurement policies aimed at reforming the power sector of any country (Kalathil et al., 2019).
iv) Willing buyer, willing seller: In this scheme, tariffs are created via an agreement involving mini-grid developers and customers or a network of customers (customers could be retailers or direct consumers). In this type of plan, middlemen can be bypassed, and consumers can directly access the developers (Manetsgruber, 2015).
v) Hybrid approaches: In this tariff plan, elements of the cost-reflective and uniform tariff structures are combined. Using this approach, the government allows the use of cost-reflective tariffs while providing grants or subsidies to offset micro-grid costs to ensure that micro-grid tariffs consistently match those charged to grid-tied customers. In this situation, subsidies are directly provided to micro-grid developers or consumers. However, it is very risky for investors and developers to rely on subsidies from governments that have a reputation for lacking creditworthiness. Investors might require a guarantee that any form of government subsidies will be valid for a long time (at least 7 years and above) to recover their costs and become profit-oriented eventually (Antonanzas-Torres et al., 2021c; Akpan et al., 2013).
Table 9 summarizes the tariff strategies used by different countries in SSA.
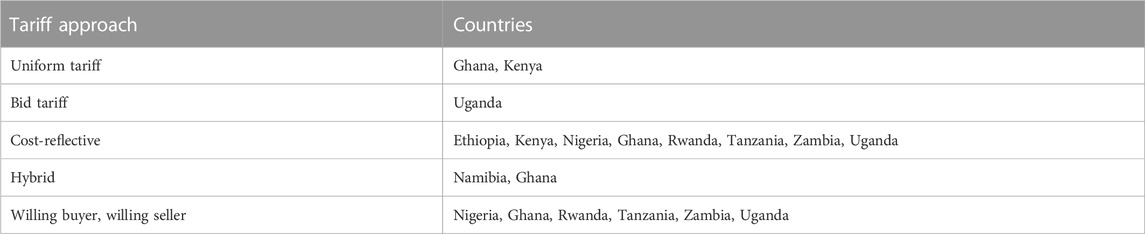
TABLE 9. Tariff strategies in Sub-Saharan Africa (Léautier, 2001).
All governments consider equity and fairness when determining tariff plans for public use. Many African governments have put in place electricity tariffs, which are uniform across their country, and they have ensured that these plans are low and affordable for public consumption based on economic realities. In most cases, the national tariffs are set lower than the charges that must be set by the mini-grid utilities to make up for their cost of operations and capital. While this is favorable for consumers, this is unfavorable for micro-grid developers. In a recent survey conducted for stakeholders in the African power industry, two-thirds of respondents expressed concerns over the difficulties involved in recovering from the high costs of implementing power projects under current tariff schemes. According to them, this is the biggest barrier to rapid power development in SSA (Akpan et al., 2013).
As a result, national governments have created subsidy schemes to make up for the gaps and ensure a continuous supply of utility services. As attractive as the arrangement of subsidy may be, it is potentially able to drain the financial resources of the country and distort capital market operations. Subsidy shortfalls arising from subsidy schemes can discourage utilities from expanding their energy service because their operations have become overly dependent on funding coming from government subsidies. This can lead to utilities losing profits and bring a premature end to their operations. For instance, in 2016, the Tanzania Electric Supply Company Limited (TANESCO) made a request to the Tanzanian government for a hike in the rate of electricity supply by 18.9% to make up for ongoing losses. The government regulator responded by granting a hike of 8.5% rather than the 18.9% requested. The 8.5% hike was later blocked by the Tanzanian president, which led to continuous losses in utility, thereby affecting grid expansion and long-term financial liabilities (Akpan et al., 2013). The provision of subsidies to mini-grid utilities should only be explored in critical times. It is very important to make these utilities economically viable with little support from the government by expanding their capacity to meet growing energy demand and support economic development. To forestall the effects of changing domestic responsibilities and global trends, the governments in SSA avoid signing long-term power purchase agreements (PPAs) that can cause barriers to short-term restructuring, which are immediate responses to unforeseen circumstances.
6 Policy status for off-grid electrification in SSA
Despite the global attention that energy access has received in recent years, at least since the launch of the “Energy for All” in 2012 and the Sustainable Development Goals 2015 (Goal 7), the pace of growth has failed to match the projected level of delivery to the society, especially in rural areas, which has remained slow compared to the population growth. Access to electricity is more problematic and evident in the SSA region and demands an active-driven approach from the national government of each country to enact workable and sustainable energy policies that will attract investors. Such policies will encourage patronage of off-grid mini-grid electrification, which will improve access to electricity in the region, thereby reducing poverty and improving the standard of living. Despite the enormous potential offered by off-grid electrification as alternative access to electricity, some technical and environmental limitations affect it. These challenges include weak regulatory and policy environmental instability, business practicability, affordability, and financial obstacles. In all of these and according to Aglina et al. (2016), who categorized the challenges related to off-grid electrification, their study identified weak regulatory and policy frameworks as the main challenge. Therefore, this section presents the policy status for off-grid electrification in SSA countries.
6.1 Policy status of off-grid electrification in Ghana
In 2016, Ghana’s Ministry of Energy (MoEn) issued a policy statement to regulate the deployment of mini-grid electrification in Ghana. The policy incorporated the public sector model of ownership and installation of mini-grids. The policy highlighted that electricity generation would be the responsibility of the public utility generation and the Volta River Authority (VRA). While the responsibility to distribute power to customers is vested in the distribution utilities: the Electricity Company of Ghana (ECG) and the Northern Electricity Distribution Company of Ghana (NEDCo) (Bukari et al., 2021). Thereafter, Safdar (2017) reported that all utilities have permission to generate and distribute power to customers. He asserted that VRA is allowed to generate and distribute power on the island, whereas NEDCo and ECG shall provide for lakeside communities within their territories. The policy statement further includes the incorporation of mini-grid electrification into the National Electrification Scheme (NES) and the safeguarding of the investment by the government. In addition, the proposition to develop an inclusive investment plan for mini-grids by the Ministry of Energy (MoEn) and the procurement of all mini-grids that allow competitiveness are part of the policy. Furthermore, the application of uniform tariffs to all customers as it is relevant to the central grid and the provision of renewable energy funds that will support the expansion of mini-grids are stated in the regulation. Based on these established facts, all businesses relating to electricity generation in Ghana are expected to acquire licenses that will guide their activities, including mini-grid operations. Currently, there is no record of a sole regulatory policy apart from the incorporated one, which will partially regulate the mini-grid operations in Ghana. Notwithstanding, the authors proclaimed to have sighted a revised version of regulations being drafted by the Energy Commission in 2017, which will address: the scope of applicability, conditions of award of licenses, and technical and commercial regulations.
6.2 Policy status of off-grid electrification in Nigeria
As many African countries are seriously developing interest in the deployment of mini-grid electrification, Nigeria is not exempted. In 2017, the country’s electricity regulatory commission approved inclusive guidelines to encourage mini-grids for both off and grid connections with a cap to regulate tariffs for grids with a distribution capacity of more than 100 kW. In a situation of stand-alone mini-grid energy generation with a capacity ranging from 100 kW to 1 MW, developers are required to obtain permits, whereas those with capabilities to generate up to 100 kW are required to register with the country’s electricity regulatory commission. The provision of subsidized capital cost by the Nigerian regulatory commission has significantly contributed to the attendant of the mini-grid electrification projects the country has recently enjoyed. In some cases, there are hybrid ownerships of the system in which the federal government offers financial resources for the project, whereas the local communities make available land and security of the projects.
6.3 Policy status of off-grid electrification in Mali, Kenya, and Senegal
Over time, diesel-based mini-grid electrification is mostly embraced in Mali, and the establishment of a rural electrification fund for mini-grid development has enhanced the involvement of private investors and developers. The rural electrification fund set aside to offset the feasibility studies and the capital cost of the projects make it more pronounced. Even then, the associated negative consequences of diesel-based mini-grid electrification, such as global warming, have motivated the Malian Agency for the Development of Domestic Energy and Rural Electrification to provide financial aid by relieving the value-added tax (VAT) on all renewable energy equipment imported. The financial aid is to inspire the deployment and incorporation of renewable energy into the existing mini-grid systems, which are more sustainable (Tenenbaum et al., 2018). Like Mali, Kenya’s earlier mini-grid electrification is diesel-based. Thereafter, some non-government organizations, faith-based organizations, and research institutions introduced the deployment of renewable energy mini-grid to the country. This prompted Kenya’s government to institute a program incorporating renewable energy into the diesel-based mini-grid system, which has improved the number of mini-grid systems in the country. Presently, the Kenya Power and Lighting Company is responsible for the operation and maintenance of the systems. With this policy arrangement, the non-government organizations support the community-based mini-grids, whereas the private organizations are free to operate under various business models. To ensure an effective operation, Kenya’s government signed an agreement with the Kenya Power and Lighting Company to keep all profits generated from mini-grids for the operation and maintenance of the system, and it will be responsible for the emanated losses. This arrangement has improved the feasibility of the system in the country (Niyonteze et al., 2019; Tenenbaum et al., 2018).
6.4 Policy status of off-grid electrification in Rwanda, Mozambique, and Tanzania
The first set of mini-grid electrification in Rwanda was for powering a set of rural healthcare clinics funded by international partners (Energy Sector Management Assistance Program, 2017). Recently, in 2015, the Rwanda Utility Regulatory Agency publicized an enthusiastic mini-grid regulation framework that excuses electricity generation below 50 kW from procuring an operating license, but the generation of 50–100 kW needs a simple license. The policy extends olive oil to private operators of mini-grid electrification to have access to grant support of 40%–70% and capital expenditure subsidies up to 100 kW. Further, the suppliers of mini-grids where there is the attendance of the main grid electrification are allowed to either transfer its asset to the utility company or sell electricity based on a fixed tariff as a small electricity generator (Safdar, 2017; Euei, 2014; Walters et al., 2015; Stiles and Murove, 2015). In Mozambique, the earliest mini-grid electrification, mostly installed by the Energy Fund (FUNAE) created by the government, are not functional due to the lack of maintenance (SEI, 2016). Although FUNAE is responsible for the operation of the existing off-grid mini-grids, it handed the management of small electricity generation below 10 kW to the local management committees, which shows that a regulatory framework for an effective operation of mini-grid electrification is needed. Interestingly, the Tanzania government has a mini-grid regulatory framework that exempts payment of VAT and import duty, which are motivations for the deployment of mini-grid electrification (Moner-Girona et al., 2016). Furthermore, the small power producers’ framework released by the Energy and Water Utilities Regulatory Authority (EWURA) has motivated private investors to invest in off-grid and mini-grid electrifications. These have contributed to the rapid growth of the mini-grid electrification in Tanzania, amounting to 0.2 MW (Pedersen, 2016; Bukari et al., 2021; Bank, 2017a). Presently, in Tanzania, private organizations install mini-grids, but the Rural Electrification Agency (REA) provides technical support (Tenenbaum et al., 2018; Euei, 2014).
6.5 Policy status of off-grid electrification in South Africa
There is vast potential for off-grid electrification in South Africa. However, this potential is yet to be fully explored due to the limited and unclear policy framework (Nasir et al., 2018). The success of off-grid electrification in South Africa depends on the right regulation and policy framework, which is lacking. Although the South Africa Government and those of other SSA countries are giving significant attention and encouraging investors to support mini-grid electrification in their regions through the development of environmentally friendly policymaking (Pegels, 2010), the current energy policy in South Africa is primarily intended for grid electrification, which is unhealthy for the utilization of mini-grid applications. This current energy policy is monopolistic in nature, unaligned with mini-grid investment, and limiting its usage. This has driven countries to review their energy policies to accommodate different key energy players who will attract investors and eventually promote the usage of mini-grid electrifications. The adoption and effective utilization of mini-grid electrification are vital for South Africa, particularly the rural settlers, where the lack of grid electrification is more pronounced. Therefore, an environmentally friendly policy framework is essential to provide and improve access to electricity for rural dwellers (Nikolina, 2016).
South Africa has a prospect to enact an effective energy policy framework because of its affiliation with a reputable global institution, the International Renewable Energy Agency (IRENA), which advocates for the use of renewable energy policies as one of its key objectives for its member countries (Winkler et al., 2009). There are three energy policies in South Africa: the white paper on energy policy in 1998, the white paper on renewable energy policy in 2003, and the National Climate Change Response Policy white paper in 2011. These energy policy documents aim to enhance access to low-cost energy services and facilitate operational energy governance. In addition, they aim to secure energy resource diversity, handle the environmental consequences that may ensue, and promote economic growth. Other related existing strategic policy documents are the Integrated Energy Plan (IEP) and Integrated Resources Plan (IRP) for mediating and determining the future of renewable energy usage in South Africa (Boait, 2014; Babatunde et al., 2019c; AMDA and ECA, 2020). The 2016 IEP provides an imperative framework for a sustainable energy environment supporting impending investment in the energy sector (Motjoadi et al., 2020). Unfortunately, this document was not approved, just like those of 2010, 2013, and 2018. Furthermore, the most recent IRP 2019 is yet to be approved. All of these have posited a negative viewpoint on renewable energy policy in South Africa and have eventually held back investment to secure the system’s flexibility and productivity (Pegels, 2010; Justo et al., 2013; Renné et al., 2006).
Table 10 shows the capacity thresholds of mini-grid operation with license exemption by some countries in the Sub-Sahara region. (Domegni and Azouma, 2022).
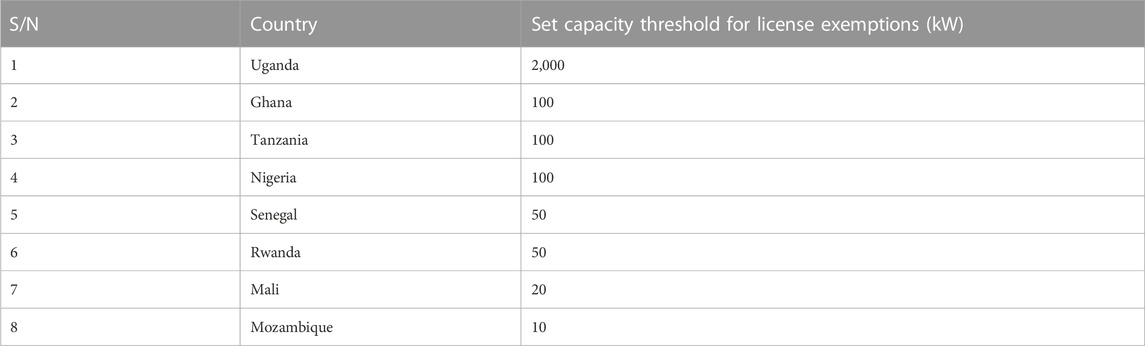
TABLE 10. Capacity thresholds of mini-grid operation with license exemption (Domegni and Azouma, 2022).
7 Rural off-grid mini-grid case studies in SSA
There are three main classes of decentralized solutions in SSA: mini-grids, energy kiosks, and individual systems. Mini-grids are managed by independent developers, usually with hybrid designs composed of renewable energy and storage sources combined together, supplying electricity to end users (small- and medium-scale businesses and households). Energy kiosks provide community-based services, for example, mobile phone charging and on-demand refrigeration in shared commercial refrigerators. Individual systems (solar kits, solar torches, and outdoor solar lanterns) meet basic needs such as lighting and phone charging.
These solutions, which are mostly distributed commercially by external stakeholders and industry players, constitute an autonomous model that provides a pathway to actualizing a vision of an independent and self-sufficient supply of off-grid electricity to solve the problems of energy poverty. In this section, we study off-grid scenarios of mini-grid adoption in East, West, and Southern African countries. Case studies from countries from SSA will be considered to get an in-depth understanding of off-grid solutions for improving access to electricity to reduce energy poverty.
At present, more than 50 mini-grid developers (both non-profit and for-profit organizations) operate across Africa, with the majority of them locally owned. Africa Minigrid Developers Association (AMDA) reports that more than 40 active for-profit developers spread across 19 countries in Africa (Nygaard et al., 2015). Several of these operators are not only focused on rural electrification but also provide renewable electrification solutions to urban entities. It is also reported that most operators do not share details of their projects in the public domain (Nygaard et al., 2015). Therefore, it is difficult to ascertain their market shares based on publicly available data. Table 11 provides a list of for-profit companies that have at least one operational mini-grid that provides 600 h or more of monthly electricity supply.
7.1 East Africa
During 2010–2018, an approximate value of $1.28 billion in equity and debt from venture capital and bonds was invested in SSA companies to improve access to energy in SSA. More than half of the investment was given to off-grid renewable energy companies in East Africa whose mode of operation was the sale of small-scale photovoltaic systems and renewable energy-powered appliances (Acker and Kammen, 1996).
The use of decentralized or off-grid solar energy is now very popular in rural communities across East Africa in the 21st century. It is very common to find solar panels on rooftops of schools, homes, and health centers across rural communities in Tanzania, Kenya, and Uganda. Before the advent of the 21st century, the implementation of solar energy projects in East Africa was undertaken to support the development of national grids. Extensive public programs organized by East African governments (supported by multinational financial organizations such as the World Bank) for national development through rural electrification were majorly focused on building energy infrastructures for the generation of electricity in regional power grids (Sheya and Mushi, 2000; Jacobson, 2007). Before the 21st century, only Kenya had a flourishing market for off-grid solar systems in rural communities, which was fully spearheaded by the efforts of a small number of entrepreneurs (Allela, 2022). In the last 20 years, business operations involving the use of off-grid solar energy systems have become very fruitful across East Africa, largely due to the commitment of privately owned, for-profit companies. Currently, off-grid solar photovoltaics are used as portable lighting and charging systems.
As of 2015, approximately 8.4 million households in Kenya, nearly 32% of the population, were connected to the nation’s electricity grid. While the connected urban population was 60%, the percentage of rural households connected to the grid was only 7% (Bhatia and Angelou, 2015). When there is no electricity supply, most households in rural communities depend on kerosene lamps and devices powered by dry cell batteries for lighting purposes (Wagner et al., 2021). The Kenyan government has made commitments toward ensuring that access to universal energy in rural locations is achieved by 2022; the government accepts the fact that it is important that the use of alternative energy be encouraged in locations with dispersed patterns of settlements requiring huge investments in building infrastructure and connection to the national grid (Hagumimana et al., 2021). The option of using solar energy as a means of generating alternative energy is very prominent as insolation in Kenya occurs all year round. Generally, the Kenyan government supports the use off-grid solutions and has put in place policies such as waivers for VAT and tariffs on solar PV products (Antonanzas-Torres et al., 2021c). Kenya has now become a leading market for off-grid solar products in Africa due to support from favorable government policies and key stakeholders. During 2009–2013, there was an increase in the sale of solar lanterns by over 200% (Hagumimana et al., 2021). Between January 2009 and June 2018, Kenya witnessed the sale of 4.7 million high-powered solar products, and almost 10 million people (approximately 20% of Kenya’s population) used high-powered solar products to meet their basic electricity needs (Antonanzas-Torres et al., 2021c). This prominent growth attributed to sales in the country is largely based on sales in densely populated parts of the country. These solar products are sold using distribution models such as direct sales to customers, pay-as-you-go (PAYG) systems, and loan-based sales through credit systems. Portable solar lights represent 90% of solar products sold in Kenya, thereby dominating its off-grid solar market (Antonanzas-Torres et al., 2021c).
In Rwanda, the off-grid solar electrification strategy encourages the use of solar water pumps, solar lanterns, solar mini-grids, solar water heaters, and SHS (Grimm et al., 2020). Therefore, much progress has been made in Rwanda in improving access to energy, with an increase from 6% in on-grid access in 2000 to 37% in on-grid access in 2019 (Eustache et al., 2019). Despite the progress in on-grid access to electricity, the low percentage of on-grid access is an opportunity for the off-grid sector to experience a boom. Presently, off-grid access to electricity in Rwanda covers 14% of the population. Currently, energy generated from renewable sources makes up 52.4% (113.14 MW) of the total energy generated in Rwanda; 218 MW is the total installed energy capacity from all energy sources in Rwanda (Chang et al., 2021). The Rwandan government has committed to the off-grid sector by targeting 48% of its population for off-grid electricity supply by 2024 (Niyonteze et al., 2020). Currently, SHS, sold using a pay-as-you-go (PAYG) model, continues to dominate the off-grid sector. This situation is very similar to other off-grid markets in East Africa (Bishoge et al., 2019). However, according to results from a survey conducted by the Fifth Integrated Household Living Conditions (EICV5), the consumer’s ability to pay for the off-grid solar product in Rwanda is discouraging. According to the survey, 75% of off-grid households spend less than $1.67 per month on lighting and charging electric devices (Bishoge et al., 2019). Therefore, the Rwandan government has recognized this constraint and is working with development partners to develop an effective subsidy scheme.
In Tanzania, rural electrification is a serious challenge, as only 2% of the rural population can access electricity. Most rural communities are forced to depend on low-quality fuels (such as kerosene for lighting and charcoal) for cooking, which are hazardous and expensive (Marcel et al., 2021). In 2013, Tanzania had a per capita electricity consumption of 89 kWh. At the time, this was one of the lowest rates in the world and only one-quarter of the average rate of electricity consumption in SSA (Kadri et al., 2019). Some of the main challenges faced by the energy sector in Tanzania include rising rural electrification demand and unreliable electric utilities. The high cost of grid extension and grid unreliability in remote areas with dense populations in Tanzania provide an opportunity for off-grid electrification (Kadri et al., 2019). Tanzania is blessed with immense, high-quality renewable energy resources, which are yet to be explored to their full potential. Tanzania has an abundance of sunlight, experiencing a range of 2,800–3,500 h of solar radiation yearly and an average radiation of 4–7 kWh per square meter every day. There is a wealth of solar energy resources in the central region of Tanzania. Tanzania is also known for its high wind power potential averaging 15–23 feet per second. More than 10% of Tanzania, equaling the landmass of Malawi, have high wind power potential. The overall wind potential of Tanzania also exceeds the wind speed potential and coverage of the entire state of California (Marcel et al., 2021). According to an estimation performed by the Rural Electrification Investment Prospectus in 2012, half of the rural populace in Tanzania might have a better experience combining mini-grids and off-grid options rather than using the conventional grid connection (Pillot et al., 2019). It is cheaper to generate off-grid electricity using renewable energy compared to the conventional diesel option. Integrating multiple renewable energy sources, which are highly accessible, ensures that residents of rural communities have renewable energy systems that provide a clean and reliable supply of electricity for rural residents. The optimal configuration and sizing of an integrated system would be specific to a site for the minimization of energy generation costs (Pedersen, 2016).
7.2 West Africa
The status of electrification in West African nations is currently presented to be below 40%, losses in the national grid above 39% with regular disruptions, and electricity prices averaging between $0.35/kWh and $0.66/kWh (Pillot et al., 2019). Accordingly, in the last decade, great attention has been given to off-grid systems (SHS and mini-grids) as an alternative to conventional energy solutions. Currently, over 385 mini-grids operate with a power of approximately 30 MW in West Africa, and 95% of these mini-grids are based on PV (USAID, 2022). Since 2019, different projects aiming to install at least 317 new mini-grids in Togo, 250 in Nigeria, 100 in Burkina Faso, and 2 in Mali using funding obtained from international aid have been implemented. Moreover, the market involving start-ups dealing with mini-grid energy has grown from $19 million in 2013 to $339 million in 2018 (Bamisile et al., 2017).
Nigeria is rich in conventional and renewable energy sources. The economic potential of renewable energy in Nigeria is twice higher than that of oil and natural gas reserves. Solar, biomass, hydro, and wind energy are notable sources of renewable energy in Nigeria. The daily solar radiation capacity of Nigeria is 3.5–7.0 kWm−2 solar radiation, and the wind speed capacity is 2.0–4.0 m−1. An estimated hydro potential of 14,750 MW, including 144 million tons per year of biomass resources, also exists in Nigeria (Liu et al., 2019). Although Nigeria is rich in vast energy resources, there is surprisingly poor coverage in terms of access to electricity. As of 2020, only 55.4% of the entire Nigerian population have access to electricity, and only 24.6% of the rural population have access to electricity (Liu et al., 2019). The nature of the power sector has led to problems and challenges undermining access to a reliable and constant power supply in Nigeria. The national grid is mostly unsuitable for the electrification of several rural populations in Nigeria due to long rural distances from the grid and bad topography, making it uneconomical or difficult to extend such rural populations to the national grid. The Rural Electrification Agency (REA) of Nigeria estimates an annual market opportunity of $10 Bn/year from the use of mini-grids and solar home systems in Nigeria, with a saving potential of $6 Bn/year. The mini-grid market in Nigeria can be increased to 10,000 sites by 2023, covering 14% of the Nigerian population with a capacity of up to 3,000 MW. This will create an estimated investment potential of ∼$20 Bn and an annual revenue opportunity exceeding $3 Bn. Most mini-grid projects in Nigeria can be found in densely populated agrarian communities, with a population of 2,500 people distributed among 300–500 households. Before the advent of mini-grids in Nigeria, people in rural communities used torchlights, kerosene lamps, candles, and generators as lighting sources (Liu et al., 2019). According to the Rural Electrification Agency (REA) of Nigeria, there is an enabling environment for the growth of mini-grid markets. There is developer protection in the form of mini-grid regulations, effective site-selection processes, and procedures to de-risk projects (Bamisile et al., 2017). Some proposals for the use of educational tools in the form of digital applications to improve consumer knowledge of renewable energy opportunities exist in Nigeria. For instance, Turcotte et al. (2001) designed an android application that uses a framework that gives information to consumers about a chosen PV system architecture and provides cost implications of continuing with the investment. The application aims to enlighten household owners about the viability of utilizing solar energy systems for residential energy needs, especially in rural areas.
In Togo, the use of energy systems is a balance between centralized and distributed energy systems. This is a current trend aimed at improving the reliability and independence of energy by ensuring that the electricity supply is stable (Kansongue et al., 2018). Togo depends on the following composition for the generation of energy: biomass energy of 71% (vegetable waste, firewood, charcoal, etc.), petroleum products of 26%, and electric power of 3% (Kansongue et al., 2018). Electricity is currently the main source of energy in Togo. Access to electricity in Togo has increased from 17% in 2000 to 45% in 2018. However, there is a large difference between urban and rural access to electricity, where urban access is 88% and rural access is 8% (Kansongue et al., 2022). The total production of electricity in Togo in 2018 was 385.62 GWh, with losses in transmission and distribution activities accounting for 15.85% of the generated units of electricity (Kitegi et al., 2022). Togo has significant resources of renewable energy such as solar, wind, and hydroelectric power. The renewable energy potential of the country could be developed effectively for a sustainable nationwide energy system. The Togolese government is conscious of this fact and is trying to improve off-grid access to electricity via mini-grids (Kitegi et al., 2022). The Government of Togo aims to deploy more than 300 mini-grids worth $251 million in investments by 2030. The Togolese government is currently focused on mobilizing investments in the private sector by facilitating Public Private Partnerships (PPP). The government is also leveraging the use of digital tools for the rapid and effective increase in rural energy access through the implementation of off-grid energy services. It aims to expand the extension of energy services in rural areas to improve rural access to electricity (Monyei et al., 2018a). Therefore, it has developed a strategy for electrification that uses geospatial modeling to determine the most efficient means of electrifying households in rural communities. Between 2018 and 2030, this strategy aims to cover the deployment of 555,000 SHS, 300 mini-grids (with 55,000 connections), and 400,000 on-grid connections, with the goal of achieving universal electrification by 2030 (Kitegi et al., 2022).
In Mali, there has been a considerable shift in the mix of energy over the years from power generation dominated by hydro sources to a generation nearly split equally between hydro and thermal (fossil) power. Averagely, installed power capacity has increased by 8.1% on a yearly basis during 2005–2015. Despite this growth, an electricity supply deficit of 150 MW remains owing to the rising demand for electricity. Despite uncertainties in the sector, progress has significantly been made in terms of increasing access to electricity in Mali. As of 2017, over 40.5% of Malians had access to modern energy services, with 83% of that access being in urban areas and 17% in rural areas. However, the interaction between on-grid and off-grid access to electricity remains very unclear and actively hinders future electrification plans by preventing the involvement of donors, operators, and government institutions alike. The price difference between mini-grids in urban and rural areas remains very high, with the price charge in rural areas estimated to be $0.44–$0.49/kWh and that charged by the national utility in the urban areas being $0.23/kWh. The electricity system is composed of a national grid owned and operated by Energie du Mali SA (EDM SA). The national grid supplies 35 towns, including the city of Bamako. The Malian Agency for Domestic Energy and Rural Electrification (AMADER) is responsible for the implementation and regulation of rural electrification schemes below 250 kW in accordance with the rural electrification reference framework adopted in the year 2003. Hydro and thermal power stations are the main sources of energy in Mali. Although there has been an increase in the installation of hybrid systems (solar/diesel) and small-scale decentralized photovoltaic (PV) systems since 2011, the use of renewable energy with the exclusion of large hydropower remains low. Mali continues to face major challenges in the power sector. Due to the high costs of energy generation from thermal power stations and excessive losses from technical and non-technical networks, there exist high tariffs in charges when consumption is below 50 kWh per month. According to IRENA, in 2015, using only 2% of the country’s solar PV potential was sufficient to cover the total supply of electricity (Moner-Girona et al., 2016). The Malian National Renewable Energy Action Plan (NREAP) has set ambitious objectives for both on-grid and off-grid systems. A target of 1,416 MW in installed capacity for on-grid renewable energy sources has been set for connected systems to achieve by 2030. For off-grid renewables, installed capacity is targeted to increase from 20 MW in 2010 to over 600 MW by 2030.
7.3 Southern Africa
Southern Africa, just like every other region, has great prospects as regards the potential of renewable energy for achieving complete access to electricity. However, several challenges hindering the exploration of these prospects also exist and are quite similar to those faced by East and West Africa.
In South Africa, non-renewable fossil fuel, which generates more than 95% of the nation’s electricity, has peaked and is now experiencing a decline. This has led to an increase in the cost of electricity generation in the presence of an irreversible increase in electricity demand (Monyei and Adewumi, 2017). Incidentally, the domestic housing sector accounts for 20% of this demand (Nhamo and Mukonza, 2016). The national grid of South Africa provides electricity access to 85% of South Africans. However, it is greatly challenged by capacity and connection constraints, which hinder the development of renewable energy in the country. Some of the capacity constraints faced by the national grid include aging infrastructure and vast geography, leading to grid congestion. This hinders potential consumers, especially in rural areas, from connecting to the national grid. As of 2013, the rate of access to electricity in rural areas of South Africa was 77%. However, the need to improve the off-grid generation of electricity remains, as the South African National Energy Development Institute (SANEDI) estimated that an excess of three million people still live without electricity in rural areas of South Africa (Winkler, 2005). In addition, kerosene and paraffin are still dominantly used for lighting in rural areas amongst the poorest populations, as 17–20 million South Africans (accounting for 40% of the population) use kerosene daily (Oyewo et al., 2019). To improve rural electrification in South Africa, the Government of South Africa, through the Department of Energy, came up with New Household Electrification Strategy in 2011. The strategy aims to reach 90% on-grid electrification and 10% off-grid electrification (Temudo et al., 2019).
In Angola, access to clean, modern, and reliable energy remains low: 33% nationwide, 69% in urban areas, and 6% in rural areas (Puati Zau and Daniel Chowdhury B, 2018). The Government of Angola aims to increase access to electricity by 60% by 2025 as part of its long-term development strategy. It also aims to make renewable energy (RE) contribute 70% of the country’s installed capacity by 2025. The potential of hydropower is huge, with a current estimation of 18.2 GW, out of which only 20% is currently exploited (Garcia and Raji, 2021). So far, the Government of Angola has identified 100 locations with the potential to cumulatively generate 600 MW from mini-hydropower resources. Angola has an annual global horizontal radiation of 1.350–2.070 kWh/m2/year (Temudo et al., 2020). The Angolan government has also targeted the installation of 100 MW of solar resources by 2025, with 30 MW planned for off-grid electricity generation. In addition to solar energy, a substantial opportunity also exists to develop wind energy with the presence of wind farms in Southern Angola (Lyambai, 2018). Exploring the renewable energy potential in Angola needs significant investments from the private sector in collaboration with technical assistance from relevant institutions in charge of promoting the implementation of renewable energy projects. Putting in place effective regulatory frameworks to bring in credible independent power producers (IPPs) is very important in meeting the targets of the Angolan Government. The Government of Angola has already begun conducting reforms to improve the country’s investment climate in the renewable energy sector. They have a) adopted a new renewable energy strategy, b) revised the electricity tariff regime and enacted a reform on energy subsidy, and c) adopted new laws on procurement and distribution of electricity to support independent power generation. To support its achievements and challenges, the Government of Angola has formally requested the assistance of The Sustainable Energy Fund for Africa (SEFA) to develop and implement a regulatory and institutional framework to encourage private sector investment in renewable energy generation aimed at reducing the dependence of the country on fossil fuel resources that are depleting. The support from SEFA will assist the Angolan Government in achieving commitments in the form of investments in the renewable energy project. A one-stop shop unit called the Energy Project Implementation Support Unit (EPISU) was established to achieve this. The technical assistance provided by SEFA includes a) providing legal frameworks, policy statements, and fiscal incentives for renewable energy to ensure an enabling environment for IPP/PPP projects and improve the economic viability of renewable energy projects and b) resolving issues related to capacity building, procurement, implementation, and monitoring of projects (Temudo et al., 2020).
In Zambia, a wide range of energy resources exist, including coal, woodlands, hydropower, sand forests, and renewable sources of energy. However, the electrification rate remains low because only 31.2% of the Zambian population are connected to the grid (Stritzke and Jain, 2021). As of 2019, 69% (accounting for approximately 11 million Zambians) are without access to electricity. Nearly 67% of the access to electricity is in urban areas, whereas only 4% is in rural areas. As of 2016, the installed electricity generation capacity was 2,800 MW, with 2,380 MW (85%) generated by hydropower plants and 405 MW (15%) generated by non-hydropower plants. Independent power producers exist to support the generation of electricity across the country (Heffron and McCauley, 2017). Although the Zambian Government aims to reach 90% urban electrification and 51% rural electrification by 2030, it has slowly invested in the solar and hydro energy sector, not up to 30% of the available 6,000 MW potential of hydro resources in Zambia has been used, and the installation of solar PV only exists in approximately 400 households, few schools, and Chief’s Palaces across the country (Stritzke and Jain, 2021). At least five mini-grids exist in Zambia, and the government has put in place frameworks and strategies to ensure that the development of the off-grid generation of electricity is effective.
8 Energy justice in off-grid mini-grids in SSA
Energy justice is a framework that addresses the energy trilemma of politics, economics, and the environment, with a just and equitable approach (Villavicencio Calzadilla and Mauger, 2017). It comprises three key tenets: distributional justice, recognition justice, and procedural justice. Distributive justice is concerned with the fair distribution of the burdens and gains of energy production to all social and spatial strata. Recognition justice identifies those affected by injustices and pays attention to fair representation of all socio-political groups. Procedural justice ensures that energy-related decision-making processes are performed transparently and impartially (Sovacool et al., 2017). Figure 6 shows that these tenets can be applied to the entire energy life cycle through principles including availability, affordability, due process, transparency/accountability, sustainability, intra- and inter-generational equity, and responsibility (Villavicencio Calzadilla and Mauger, 2017).
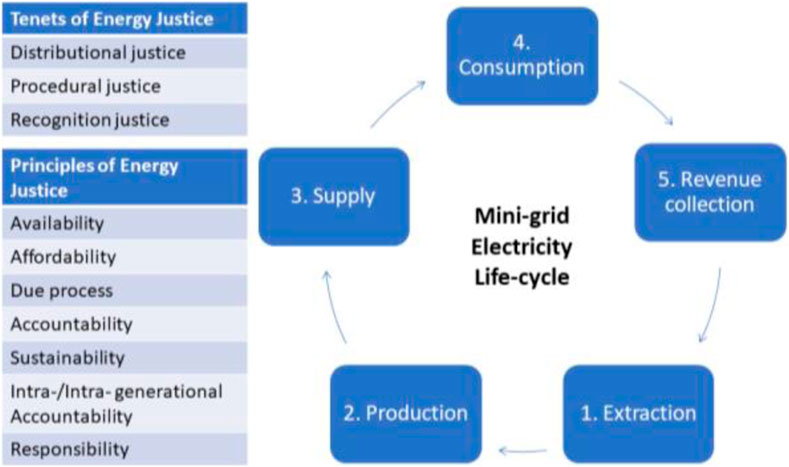
FIGURE 6. The energy justice framework applied to mini-grid electricity life-cycle (Villavicencio Calzadilla and Mauger, 2017).
Researchers consider decentralized renewable universal energy access as a just means of closing the energy gap in developing countries because “they can provide safe, reliable, affordable and sustainable energy to local communities in developing countries” (Sovacool et al., 2017). Nonetheless, without the application of the energy justice framework (EJF) tenets and principles, it will be unclear where influential stakeholders have taken advantage of the poor and marginalized people and communities they were meant to assist. EJF also helps assess the intentions and activities of the global community under the lenses of justice and equity, providing guidelines for restorative justice where necessary. The implementation of energy justice in SSA’s off-grid micro-grid development will facilitate a paradigm shift from the prevalent norm (i.e., rural electrification for the mere sake of participating in the clean transition or mainly as a means of only improving human development by universal energy access). Under the EJF, rural off-grid electrification should achieve affordability, energy reliability, sufficiency, environmental sustainability, economic growth, human development, intra- and inter-generational equity, and freedom to call out and resist perceived energy injustices (Monyei et al., 2018b).
Researchers from the Global South have pointed out the gap in understanding that some Global North researchers have in respect of the needs of the Global South, resulting in models and policies that cause failed projects (Monyei and Adewumi, 2017; Lacey-Barnacle et al., 2020; Gladkykh et al., 2021). This poor understanding is reflected by Boamah (2020), who applied “energy justice” to SSA’s energy access. The developed model is intended to achieve energy sufficiency for households with 100%-renewables as a priority. It resulted in a levelized cost of energy (LCOE) six times higher than the 25%-renewable scenario. Several limitations can be observed within the work. First, it ignored the place of economic activities that will enable the energy-poor communities to fund the proposed high-cost sustainable electricity without perpetual recourse to grants and charity donations. Second, it defines energy sufficiency from the viewpoint of having electricity to meet “basic” household needs (i.e., lighting, charging mobile phones, fan-based cooling, and radio). These household devices are insufficient to facilitate skilling up and active social participation in the information age. The researchers ignore the low utility of the proposed solar PV electricity for heating and cooling, cooking, and medium-scale commercial activities. Thus, residents still have to pay for additional solid biomass for cooking and heating. Hence, for the EJF to be effective for SSA’s off-grid mini-grids, it is important for researchers to deeply understand the peculiarities and practical needs of the region.
8.1 Identified challenges with solar energy-focused policy
• Solar PV provides restrictive utility because SHS and solar mini-grids are unaffordable for heating, cooking, heavy machine/motor operation, and medium/large-scale agro-processing (Monyei and Adewumi, 2017).
• Solar PV systems require energy storage due to the intermittency of solar energy availability. This necessitates battery storage technologies, and the literature has a consensus on their unaffordability for rural communities in developing SSA. For instance, battery storage accounts for 40% or more (depending on the kind of battery technology) of total capital expenditure for installing decentralized building solar systems in SSA. Declining solar PV prices are yet to be matched by a commensurate fall in energy storage technologies (Babayomi and Dahoro, 2021).
• Local innovation and maintenance are difficult, leading the bulk of indirect economic impacts of solar electrification systems to be directed to already-developed and prosperous countries. The key technologies in solar systems, including solar panels, inverters, and battery storage, are relatively new and advanced, which increases dependence on foreign expertise (Babayomi et al., 2020).
• AC mini-grids are relatively inefficient and increase energy losses due to higher conversion stages from DC/AC along the path from generation to consumption. Solar energy is generated directly in DC form, whereas most rural electrical appliances and equipment utilize a form of AC power. If DC appliances are sold to rural SSA communities, inverters will become unnecessary, and the capital cost and (financial and technical) inconveniences caused by faulty inverters can be eliminated. This will further improve equity in rural electrification. Thus, DC mini-grids need to be better promoted (Kyriakarakos et al., 2020).
8.2 Recommendations for just and equitable mini-grids in SSA
• Several studies advocate for the adoption of hybrid energy systems which accommodate renewable and fossil-based (particularly diesel and gasoline) energy sources. Hybrid electricity systems provide lower LCOE and energy sufficiency, enabling households to meet diverse energy needs from lighting to cooking and heating (Akpan et al., 2013; Lacey-Barnacle et al., 2020). Hybrid systems also provide energy mobility, easing the ability of users to increase energy asset acquisition as their demand increases without incurring financial losses (Allela, 2022). Energy resilience, as measured via sufficiency and mobility, should be given a prime place in the planning and deployment of off-grid electricity projects. Moreover, hybrid electricity systems permit a paced transition for SSA rather than the immediate clean transition, which widens the energy poverty gap, and exacerbates energy injustice.
• Productive use of energy is an oft-overlooked form of energy justice. When mini-grid suppliers provide affordable electricity to power profitable commercial ventures in rural agricultural/pastoral settlements, they further empower the consumers to pay for electricity to improve their economic activities and standard of living (USAID, 2022; Reber et al., 2018). Catering to commercial energy needs also spreads energy use throughout the day (instead of only at night-time for households), leading to a higher load factor and a lower tariff (Akpan et al., 2013; Reber and Booth, 2018). Therefore, metrics that facilitate the productive use of energy, including affordable tariff (that facilitates competitive production of goods and services), quantity, quality, and reliability of electricity, must be integrated into off-grid mini-grid planning, financing, and business models (USAID, 2022). Customized solutions to catalyze commercial agriculture and livestock and the agro-processing industry should be provided by mini-grid developers.
• Babayomi et al. (2020) explored the theme of renewable energy access for economic growth in developing countries, advocating for balancing rural policies between human development and economic growth. Interventions that do not prioritize local economic growth will be unsustainable in the long term and will remain ever dependent on grants and hand-outs from charities. The study pointed out that the present technologies and market models of decentralized rural electrification result in tariffs up to 37 times the main grid tariff (most SSA grid tariffs are not cost-reflective). As a result, rural agro-industries and allied industries in SSA struggle to sell produce in the cities due to cheaper imported alternatives, which, paradoxically, are produced in developed countries operating on mainly fossil-powered grids. Therefore, the entire solar PV off-grid mini-grid value chain, from the promoted technologies in SSA to the market models in its present form, does not support low-cost rural energy access. Until these challenges are solved, SSA policymakers may need to adopt hybrid forms of decentralized electrification, which are more affordable, and catalyze competitive rural markets.
• Off-grid mini-grids should be designed beyond only providing basic, reliable access to catalyzing community members to realize multi-faceted personal potentials and impact the larger society politically (Lacey-Barnacle et al., 2020).
• Improved funding for the competitiveness of decentralized solar PV systems over the centralized grid and financing mechanisms that substantially reduce the prices of battery storage systems where utilizing PV is unavoidable are recommended (Boamah, 2020).
• Special agreements can be made with mini-grid equipment vendors for more affordable and reliable off-grid DC-based technologies. As earlier explained, the prevalent AC-based inverter systems are more expensive and less efficient and suffer lower reliability than DC systems (Babayomi and Dahoro, 2021; Babayomi et al., 2020).
• Policy uncertainties about the grid extension to the off-grid locations where mini-grid projects will be sited discourage private participation. As grid tariffs are usually better subsidized than mini-grid tariffs, electricity users are likely to prefer grid supply over the prior existing mini-grid, causing a loss of income for investors. Therefore, clear policies that protect mini-grid investments can further improve private investment in off-grid mini-grids in SSA.
9 Conclusion
This study reviewed the developments in the renewable off-grid mini-grids of SSA over the past 2 decades. This work covered the present state of research and developments in technologies, policy tariffs, design techniques, and energy justice in SSA’s off-grid mini-grids. The review indicates that solar PV is the most common and easiest renewable to deploy for mini-grids in SSA. In order to reduce the spate of oversized mini-grids installed across SSA, modern multi-criteria design techniques need to be better engaged during the feasibility study and design stages. This would also lead to the efficient deployment of financial and social resources toward the goal of providing electricity access for all. Key challenges include weak regulatory and policy frameworks, which increase the time for approvals, and financial requirements to operate off-grid mini-grids. The energy justice body of work asserts that enabling the ease of deploying hybrid mini-grids and catalyzing the profitability and growth of rural agro-markets will improve energy justice in off-grid mini-grid developments of SSA (EnergyPLAN, 2022; Long and Steinberger, 2018; Elkadeem et al., 2021).
Author contributions
OB: conceptualization, introduction, and conclusion. BO: optimal planning, sizing, and technology selection for mini-grids. ID: rural off-grid mini-grid case studies in SSA. TS: review of different renewable technologies. OA: challenges with off-grid mini-grid projects in SSA. CS: policy states for off-grid electrification in SSA. KO: rural off-grid mini-grid case studies in SSA. AA: energy justice in off-grid mini-grids in SSA.
Acknowledgments
The authors would like to express special thanks to Covenant University Management for financial support through CUCRID.
Conflict of interest
The authors declare that the research was conducted in the absence of any commercial or financial relationships that could be construed as a potential conflict of interest.
Publisher’s note
All claims expressed in this article are solely those of the authors and do not necessarily represent those of their affiliated organizations or those of the publisher, the editors, and the reviewers. Any product that may be evaluated in this article, or claim that may be made by its manufacturer, is not guaranteed or endorsed by the publisher.
References
Acker, R. H., and Kammen, D. M. (1996). The quiet (energy) revolution: Analysing the dissemination of photovoltaic power systems in Kenya. Energy Policy 24 (1), 81–111. doi:10.1016/0301-4215(95)00112-3
Aglina, M. K., Agbejule, A., and Nyamuame, G. Y. (2016). Policy framework on energy access and key development indicators: ECOWAS interventions and the case of Ghana. Energy Policy 97, 332–342. doi:10.1016/j.enpol.2016.07.012
Ajeigbe, O. A., Munda, J. L., and Hamam, Y. (2020). Towards maximising the integration of renewable energy hybrid distributed generations for small signal stability enhancement: A review. Int. J. Energy Res. 44 (4), 2379–2425. doi:10.1002/er.4864
Akinyele, D., Belikov, J., and Levron, Y. (2018). Challenges of microgrids in remote communities: A steep model application. Energies 11, 432. doi:10.3390/EN11020432
Akinyele, D., Okakwu, I., Olabode, E., Blanchard, R., Ajewole, T., and Monyei, C. (2022). Integrated TEEP approach to microgrid design and planning with small hydro/solar/diesel resources for standalone application. e-Prime-Advances Electr. Eng. Electron. Energy, 100091.
Akpan, U. S., Isihak, S. R., and Udoakah, Y. O. N. (2013). “Electricity access in Nigeria: Viability of off-grid photovoltaic system,” in Proceeding of the IEEE AFRICON Conf, Pointe aux Piments, Mauritius, September 2013 (IEEE). doi:10.1109/AFRCON.2013.6757778
Al Garni, H. Z., and Awasthi, A. (2017). Solar PV power plant site selection using a GIS-AHP based approach with application in Saudi Arabia. Appl. Energy 206, 1225–1240. doi:10.1016/j.apenergy.2017.10.024
Allela, T. (2022). A 2020 desk review of Power Africa. J. Int. Dev. 34, 334–348. doi:10.1002/jid.3595
Aly, A., Jensen, S. S., and Pedersen, A. B. (2017). Solar power potential of Tanzania: Identifying CSP and PV hot spots through a GIS multicriteria decision making analysis. Renew. energy 113, 159–175. doi:10.1016/j.renene.2017.05.077
AMDA and ECA (2020). Benchmarking africa’s minigrids, 1–59. Africa Minigrid Developers Association and Economic Consulting Associates.
Antonanzas-Torres, F., Antonanzas, J., and Blanco-Fernandez, J. (2021). State-of-the-art of mini grids for rural electrification in West Africa. Energies 14 (4), 990. doi:10.3390/en14040990
Antonanzas-Torres, F., Antonanzas, J., and Blanco-Fernandez, J. (2021). State-of-the-Art of mini grids for rural electrification in West Africa. Energies 14, 990. doi:10.3390/EN14040990
Antonanzas-Torres, F., Antonanzas, J., and Blanco-Fernandez, J. (2021). Environmental life cycle impact of off-grid rural electrification with mini grids in West Africa. Sustain. Energy Technol. Assessments 47, 101471. doi:10.1016/j.seta.2021.101471
Aydin, N. Y., Kentel, E., and Duzgun, H. S. (2013). GIS-based site selection methodology for hybrid renewable energy systems: A case study from Western Turkey. Energy Convers. Manag. 70, 90–106. doi:10.1016/j.enconman.2013.02.004
Ayodele, T. R., Ogunjuyigbe, A. S. O., Odigie, O., and Munda, J. L. (2018). A multi-criteria GIS based model for wind farm site selection using interval type-2 fuzzy analytic hierarchy process: The case study of Nigeria. Appl. Energy 228, 1853–1869. doi:10.1016/j.apenergy.2018.07.051
Azimoh, C. L., Klintenberg, P., Wallin, F., Karlsson, B., and Mbohwa, C. (2016). Electricity for development: Mini-grid solution for rural electrification in South Africa. Energy Convers. Manag. 110, 268–277. doi:10.1016/j.enconman.2015.12.015
Azimoh, C. L., Klintenberg, P., Mbohwa, C., and Wallin, F. (2017). Replicability and scalability of mini-grid solution to rural electrification programs in sub-Saharan Africa. Renew. energy 106, 222–231. doi:10.1016/j.renene.2017.01.017
Aziz, S., Chowdhury, S. A., and Groh, S. (2016). The success of solar diesel minigrids in Bangladesh: A case study of sandwip island. Energy Procedia 103, 316–321. doi:10.1016/J.EGYPRO.2016.11.292
Babatunde, O. M., Munda, J. L., and Hamam, Y. (2019). A comprehensive state-of-the-art survey on power generation expansion planning with intermittent renewable energy source and energy storage. Int. J. Energy Res. 43, 6078–6107. doi:10.1002/er.4388
Babatunde, O. M., Munda, J. L., and Hamam, Y. (2019). How can a low-income household procure small-scale hybrid renewable energy system? A techno-economic and acquisition analysis. Int. J. Energy Sect. Manag. 13, 1149–1172. doi:10.1108/IJESM-03-2019-0003
Babatunde, O. M., Munda, J. L., and Hamam, Y. (2019). Selection of a hybrid renewable energy systems for a low-income household. Sustainability 11 (16), 4282. doi:10.3390/su11164282
Babatunde, O. M., Munda, J. L., and Hamam, Y. (2020). A comprehensive state-of-the-art survey on hybrid renewable energy system operations and planning. IEEE Access 8, 75313–75346. doi:10.1109/access.2020.2988397
Babayomi, O., and Dahoro, D. (2021). “Energy access vs. Energy for prosperity: A reassessment of africa’s strategies and priorities,” in Proceeding of the 2021 IEEE PES/IAS PowerAfrica, Nairobi, Kenya, August 2021 (IEEE), 1–5. doi:10.1109/PowerAfrica52236.2021.9543177
Babayomi, O. O., Dahoro, D. A., and Zhang, Z. (2022). Affordable clean energy transition in developing countries: Pathways and technologies. iScience 25 (5), 104178. doi:10.1016/J.ISCI.2022.104178
Babayomi, O., and Okharedia, T. (2019). “Challenges to sub-saharan africa's renewable microgrid expansion - a cetep solution model,” in Proceeding of the 2019 IEEE PES/IAS PowerAfrica, Abuja, Nigeria, August 2019 (IEEE). doi:10.1109/PowerAfrica.2019.8928865
Babayomi, O., Shomefun, T., and Zhang, Z. (2020). “Energy efficiency of sustainable renewable microgrids for off-grid electrification,” in Proceeding of the 2020 IEEE PES/IAS PowerAfrica, Nairobi, Kenya, August 2020 (IEEE). doi:10.1109/PowerAfrica49420.2020.9219958
Balamurugan, P., Ashok, S., and Jose, T. L. (2009). Optimal operation of biomass/wind/PV hybrid energy system for rural areas. Int. Jouran Green Energy 6 (1), 104–116. doi:10.1080/15435070802701892
Bamisile, O., Dagbasi, M., Babatunde, A., and Ayodele, O. (2017). A review of renewable energy potential in Nigeria; solar power development over the years. Eng. Appl. Sci. Res. 44 (4), 242–248. Accessed: Jun. 25, 2022. [Online]. Available: https://ph01-ohno.tci-thaijo.org/index.php/easr/article/view/77226.
Bank, W. (2017). State of electricity access report 2017. Washington, DC, United States: World Bank.
Baring-Gould, E. I., Green, H. J., van Dijk, V. A. P., and Manwell, J. F. (1996). Hybrid2: The hybrid power system simulation model. Golden, Colorado, United States: National Renewable Energy Laboratory.
Bhatia, M., and Angelou, N. (2015). Beyond connections : Energy access redefined. ESMAP Technical Report;008/15. Available: https://openknowledge.worldbank.org/handle/10986/24368 (accessed Jan. 17, 2022).
Bhattacharyya, S. C. (2015). Mini-grid based electrification in Bangladesh: Technical configuration and business analysis. Renew. Energy 75, 745–761. doi:10.1016/j.renene.2014.10.034
Bhattacharyya, S. C. (2018). Mini-grids for the base of the pyramid market: A critical review. Energies 11, 813. doi:10.3390/EN11040813
Bishoge, O. K., Zhang, L., and Mushi, W. G. (2019). The potential renewable energy for sustainable development in Tanzania: A review. Clean. Technol. 1, 70–88. doi:10.3390/CLEANTECHNOL1010006
BloombergNEF and SEforALL (2020). State of the global mini-grids market report 2020: Trends of renewable energy hybrid mini-grids in sub-saharan africa, asia and island nations. United Kingdom: BloombergNEF and Sustainable Energy for All. [Online]. Available: https://www.seforall.org/system/files/2020-06/MGP-2020-SEforALL.pdf.
Boait, P. J. (2014). Mini-grids for rural electrification of developing countries: Technical aspects of mini-grids for rural electrification. Springer Cham. doi:10.1007/978-3-319-04816-1
Boamah, F. (2020). Desirable or debatable? Putting africa’s decentralised solar energy futures in context. Energy Res. Soc. Sci. 62, 101390. doi:10.1016/J.ERSS.2019.101390
Booth, S., Li, X., Baring-Gould, I., Kollanyi, D., Bharadwaj, A., and Weston, P. (2018). Productive use of energy in african micro-grids: Technical and business considerations. doi:10.2172/1465661
Bukari, D., Kemausuor, F., Quansah, D. A., and Adaramola, M. S. (2021). Towards accelerating the deployment of decentralised renewable energy mini-grids in Ghana: Review and analysis of barriers. Renew. Sustain. Energy Rev. 135, 110408. doi:10.1016/J.RSER.2020.110408
Bukari, D., Quansah, D. A., Kemausuor, F., and Adaramola, M. S. (2022). Ex-post design, operations and financial cost-benefit analysis of mini-grids in Ghana: What can we learn? Energy sustain. Dev. 68, 390–409. doi:10.1016/j.esd.2022.04.009
Bullich-Massagué, E., D\’\iaz-González, F., Aragüés-Peñalba, M., Girbau-Llistuella, F., Olivella-Rosell, P., and Sumper, A. (2018). Microgrid clustering architectures. Appl. Energy 212, 340–361. doi:10.1016/j.apenergy.2017.12.048
Chang, K. C., Hagumimana, N., Zheng, J., Asemota, G. N. O., Niyonteze, J. D. D., Nsengiyumva, W., et al. (2021). Standalone and minigrid-connected solar energy systems for rural application in Rwanda: An in situ study. Int. J. Photoenergy 2021, 2021–2022. doi:10.1155/2021/1211953
Come Zebra, E. I., van der Windt, H. J., Nhumaio, G., and Faaij, A. P. C. (2021). A review of hybrid renewable energy systems in mini-grids for off-grid electrification in developing countries. Renew. Sustain. Energy Rev. 144, 111036. doi:10.1016/j.rser.2021.111036
Dawoud, S. M., Lin, X., and Okba, M. I. (2018). Hybrid renewable microgrid optimization techniques: A review. Renew. Sustain. Energy Rev. 82, 2039–2052. doi:10.1016/j.rser.2017.08.007
de Energia, F., and Funae, M. (2015). Solar photovoltaic systems for social infrastructure and village electrification in Mozambique: Study of existing systems in two provinces.
Denwigwe, I. H., Akinde-Peters, J. D., Babatunde, O. M., Samson Adedoja, O., Taiwo, I. A., and Adedoja, T. B. (2021). An Android-based mobile platform for understanding Residential PV system sizing. Afr. J. Sci. Technol. Innov. Dev., 1–14. doi:10.1080/20421338.2021.1959280
Department of Energy (2015). Department of energy south Africa. Energy Advocacy Newsl. Accessed, no. 29th April 2022.
Diemuodeke, E. O., Hamilton, S., and Addo, A. (2016). Multi-criteria assessment of hybrid renewable energy systems for Nigeria’s coastline communities. Energy. sustain. Soc. 6 (1), 26. doi:10.1186/s13705-016-0092-x
Domegni, K. M. S., and Azouma, Y. O. (2022). Productive uses of energy: A solution for promoting energy justice in rural areas in West Africa. Renew. Sustain. Energy Rev. 160, 112298. doi:10.1016/J.RSER.2022.112298
Duran, A. S., and Sahinyazan, F. G. (2021). An analysis of renewable mini-grid projects for rural electrification. Socioecon. Plann. Sci. 77, 100999. doi:10.1016/J.SEPS.2020.100999
Eales, A., and Unyolo, B. (2018). Renewable energy mini-grids in Malawi: Status, barriers and opportunities. Scottish: University of Strathclyde.
Eder, J. M., Mutsaerts, C. F., and Sriwannawit, P. (2015). Mini-grids and renewable energy in rural Africa: How diffusion theory explains adoption of electricity in Uganda. Energy Res. Soc. Sci. 5, 45–54. doi:10.1016/j.erss.2014.12.014
Elkadeem, M. R., Younes, A., Sharshir, S. W., Campana, P. E., and Wang, S. (2021). Sustainable siting and design optimization of hybrid renewable energy system: A geospatial multi-criteria analysis. Appl. Energy 295, 117071. doi:10.1016/j.apenergy.2021.117071
Energy Sector Management Assistance Program (2017). Mini grids in Kenya: A case study of a market at a turning point. Kenya: World Bank.
Euei (2014). Mini-grid policy toolkit. Rep. From: Http//Www.Euei-Pdf.Org.
Eustache, H., Sandoval, D., Wali, U. G., and Venant, K. (2019). Current status of renewable energy technologies for electricity generation in Rwanda and their estimated potentials. Energy Environ. Eng. 6 (1), 8–15. doi:10.13189/eee.2019.060102
Feron, S. (2016). Sustainability of off-grid photovoltaic systems for rural electrification in developing countries: A review. Sustain 8, 1326. doi:10.3390/SU8121326
Fowlie, M., Khaitan, Y., Wolfram, C., and Wolfson, D. (2018). Solar microgrids and remote energy access: How weak incentives can undermine smart technology. California: Energy Institute AT HAAS.
Franz, M., Peterschmidt, N., Rohrer, M., and Kondev, B. (2014). “Mini-grid policy toolkit,” in Alliance rural electrif. Eschborn, tech. Rep.
Gambino, V., Del Citto, R., Cherubini, P., Tacconelli, C., Micangeli, A., and Giglioli, R. (2019). Methodology for the energy need assessment to effectively design and deploy mini-grids for rural electrification. Energies 12 (3), 574. doi:10.3390/en12030574
Garcia, F. P. K., and Raji, A. K. (2021). “Potential and reliability assessment of renewable power: The case of Angola,” in Proceeding of the 2021 IEEE PES/IAS PowerAfrica, PowerAfrica, Nairobi, Kenya, August 2021 (IEEE). doi:10.1109/POWERAFRICA52236.2021.9543099
Gladkykh, G., Davíðsdóttir, B., and Diemer, A. (2021). When justice narratives meet energy system models: Exploring energy sufficiency, sustainability, and universal access in Sub-Saharan Africa. Energy Res. Soc. Sci. 79, 102075. doi:10.1016/J.ERSS.2021.102075
Graber, S., Narayanan, T., Alfaro, J., and Palit, D. (2018). Solar microgrids in rural India: Consumers’ willingness to pay for attributes of electricity. Energy sustain. Dev. 42, 32–43. doi:10.1016/j.esd.2017.10.002
Grimm, M., Lenz, L., Peters, J., and Sievert, M. (2020). Demand for off-grid solar electricity: Experimental evidence from Rwanda. J. Assoc. Environ. Resour. Econ. 7 (3), 417–454. doi:10.1086/707384
Hagumimana, N., Zheng, J., Asemota, G. N. O., Niyonteze, J. D. D., Nsengiyumva, W., Nduwamungu, A., et al. (2021). Concentrated solar power and photovoltaic systems: A new approach to boost sustainable energy for all (Se4all) in Rwanda. Int. J. Photoenergy 2021, 1–32. doi:10.1155/2021/5515513
Hakimi, S. M., and Moghaddas-Tafreshi, S. M. (2009). Optimal sizing of a stand-alone hybrid power system via particle swarm optimization for Kahnouj area in south-east of Iran. Renew. energy 34 (7), 1855–1862. doi:10.1016/j.renene.2008.11.022
Hansen, K. (2019). Decision-making based on energy costs: Comparing levelized cost of energy and energy system costs. Energy Strateg. Rev. 24, 68–82. doi:10.1016/j.esr.2019.02.003
Hartvigsson, E., Ehnberg, J., Ahlgren, E. O., and Molander, S. (2021). Linking household and productive use of electricity with mini-grid dimensioning and operation. Energy sustain. Dev. 60, 82–89. doi:10.1016/j.esd.2020.12.004
Heffron, R. J., and McCauley, D. (2017). The concept of energy justice across the disciplines. Energy Policy 105, 658–667. doi:10.1016/J.ENPOL.2017.03.018
Hennet, J. C., and Samarakou, M. T. (1986). Optimization of a combined wind and solar power plant. Int. J. energy Res. 10 (2), 181–188. doi:10.1002/er.4440100208
Ighravwe, D., and Babatunde, M. (2018). Selection of a mini-grid business model for developing countries using CRITIC-TOPSIS with interval type-2 fuzzy sets. Decis. Sci. Lett. 7 (4), 427–442. doi:10.5267/j.dsl.2018.1.004
Ighravwe, D. E., and Babatunde, M. O. (2018). Determination of a suitable renewable energy source for mini-grid business: A risk-based multicriteria approach. J. Renew. Energy 2018, 1–20. doi:10.1155/2018/2163262
Ihirwe, J. P., Li, Z., Sun, K., Bimenyimana, S., Wang, C., Asemota, G. N. O., et al. (2021). Solar PV minigrid technology: Peak shaving analysis in the East African community countries. Int. J. Photoenergy 2021, 1–40. doi:10.1155/2021/5580264
Iniyan, S., and Sumathy, K. (2000). An optimal renewable energy model for various end-uses. Energy 25 (6), 563–575. doi:10.1016/s0360-5442(99)00090-0
Inversin, A. R. (2017). “Mini-grid design manual-ESMAP technical paper 007,” in Energy sect. Manag. Assist. Program. (Washington, DC: ESMAP), 17. viewed.
Irena, I. (2018). Renewable power generation costs in 2017. Report. Abu Dhabi: Int. Renew. Energy Agency.
Jacobson, A. (2007). Connective power: Solar electrification and social change in Kenya. World Dev. 35 (1), 144–162. doi:10.1016/J.WORLDDEV.2006.10.001
Jonker Klunne, W. Current status and future developments of small and micro hydro in southern Africa. Wroclaw, Poland: Hydroenergia. Available: http://researchspace.csir.co.za/dspace/handle/10204/6032 (accessed Jun. 25, 2022).
Justo, J. J., Mwasilu, F., Lee, J., and Jung, J.-W. (2013). AC-microgrids versus DC-microgrids with distributed energy resources: A review. Renew. Sustain. energy Rev. 24, 387–405. doi:10.1016/j.rser.2013.03.067
Kadri, S. M., Bagre, A. O., Camara, M. B., Dakyo, B., and Coulibaly, Y. (2019). “Electrical power distribution status in West Africa: Assessment and perspective overview,” in Proceeding of the 8th Int. Conf. Renew. Energy Res. Appl. ICRERA, Brasov, Romania, Nov. 2019 (IEEE), 511–515. doi:10.1109/ICRERA47325.2019.8997112
Kalathil, D., Wu, C., Poolla, K., and Varaiya, P. (2019). The sharing economy for the electricity storage. IEEE Trans. Smart Grid 10 (1), 556–567. doi:10.1109/TSG.2017.2748519
Kansongue, N., Njuguna, J., and Vertigans, S. (2022). An assessment of renewable energy development in energy mix for Togo. Int. J. Sustain. Energy 41. doi:10.1080/14786451.2021.2023150
Kansongue, N., Njuguna, J., and Vertigans, S. (2018). “Sustainable energy for emerging nations development-A case study on Togo renewable energy,” in Proceeding of the 2018 IEEE PES/IAS PowerAfrica, PowerAfrica, Cape Town, South Africa, June 2018 (IEEE), 137–141. doi:10.1109/POWERAFRICA.2018.8521054
Katre, A., Tozzi, A., and Bhattacharyya, S. (2019). Sustainability of community-owned mini-grids: Evidence from India. Energy. sustain. Soc. 9 (1), 2–17. doi:10.1186/s13705-018-0185-9
Katsigiannis, Y. A., Georgilakis, P. S., and Karapidakis, E. S. (2012). Hybrid simulated annealing--tabu search method for optimal sizing of autonomous power systems with renewables. IEEE Trans. Sustain. Energy 3 (3), 330–338. doi:10.1109/tste.2012.2184840
Kaya, I., Çolak, M., and Terzi, F. (2018). Use of MCDM techniques for energy policy and decision-making problems: A review. Int. J. Energy Res. 42 (7), 2344–2372. doi:10.1002/er.4016
Kemausuor, F., Sedzro, M. D., and Osei, I. (2018). Decentralised energy systems in africa: Coordination and integration of off-grid and grid power systems—review of planning tools to identify renewable energy deployment options for rural electrification in africa. Curr. Sustain. Energy Rep. 5 (4), 214–223. doi:10.1007/s40518-018-0118-4
Kempener, R., Lavagne, O., Saygin, D., Skeer, J., Vinci, S., and Gielen, D. (2015). Off-grid renewable energy systems: Status and methodological issues. Abu Dhabi, United Arab Emirates: Int. Renew. Energy Agency.
Kitegi, M. S. P., Lare, Y., Coulibaly, O., Kitegi, M. S. P., Lare, Y., and Coulibaly, O. (2022). Potential for green hydrogen production from biomass, solar and wind in Togo. Smart Grid Renew. Energy 13 (2), 17–27. doi:10.4236/SGRE.2022.132002
Korkovelos, A., Zerri, H., Howells, M., Bazilian, M., Rogner, H. H., and Nerini, F. F. (2020). A retrospective analysis of energy access with a focus on the role of mini-grids. Sustain 12, 1793. doi:10.3390/SU12051793
Korzhenevych, A., and Owusu, C. K. (2021). Renewable minigrid electrification in off-grid rural Ghana: Exploring households willingness to pay. Sustain 13 (21), 11711. doi:10.3390/SU132111711
Kumar, A., Sah, B., Singh, A. R., Deng, Y., He, X., Kumar, P., et al. (2017). A review of multi criteria decision making (MCDM) towards sustainable renewable energy development. Renew. Sustain. Energy Rev. 69, 596–609. doi:10.1016/j.rser.2016.11.191
Kyriakarakos, G., Balafoutis, A. T., and Bochtis, D. (2020). Proposing a paradigm shift in rural electrification investments in sub-saharan africa through agriculture. Sustain 12 (8), 3096. doi:10.3390/SU12083096
Lacey-Barnacle, M., Robison, R., and Foulds, C. (2020). Energy justice in the developing world: A review of theoretical frameworks, key research themes and policy implications. Energy sustain. Dev. 55, 122–138. doi:10.1016/J.ESD.2020.01.010
Lambert, T., Gilman, P., and Lilienthal, P. (2006). “Micropower system modeling with HOMER,” in Integration of alternative sources of energy, 379–418.
Léautier, T. O. (2001). Electricity auctions. J. Econ. Manag. Strategy 10 (3), 331–358. doi:10.1111/j.1430-9134.2001.00331.x
Lee, T.-Y., and Chen, C.-L. (2009). Wind-photovoltaic capacity coordination for a time-of-use rate industrial user. IET Renew. Power Gener. 3 (2), 152–167. doi:10.1049/iet-rpg:20070068
Lena, G. (2013). “Rural electrification with PV hybrid systems: Overview and recommendations for further deployment,” in Int. Energy agency photovolt. Power syst. Program. Club african natl. Agencies struct. Charg. Rural electrif.
Li, L., Yao, Z., You, S., Wang, C.-H., Chong, C., and Wang, X. (2019). Optimal design of negative emission hybrid renewable energy systems with biochar production. Appl. Energy 243, 233–249. doi:10.1016/j.apenergy.2019.03.183
Liu, W. H., Ho, W. S., Lee, M. Y., Hashim, H., Lim, J. S., Klemes, J. J., et al. (2019). Development and optimization of an integrated energy network with centralized and decentralized energy systems using mathematical modelling approach. Energy 183, 617–629. doi:10.1016/J.ENERGY.2019.06.158
Long, N., and Steinberger, K. (2018). Renewable energy is key to fighting climate change. Nat. Resour. Def. Counc. Accessed, no. 29th April 2022.
Lyambai, M. (2018). Accelerating energy access through public-private partnership investment in Zambia. Tlemcen Algeria: SSRN Electron. J. doi:10.2139/SSRN.3211256
Manetsgruber, D. (2015). Risk management for mini-grids: A new approach to guide mini-grid deployment.
Marcel, E. T., Mutale, J., and Mushi, A. T. (2021). Optimal design of hybrid renewable energy for Tanzania rural communities. Tanzan. J. Sci. 47 (5), 1716–1727. doi:10.4314/tjs.v47i5.19
Messaoudi, D., Settou, N., Negrou, B., and Settou, B. (2019). GIS based multi-criteria decision making for solar hydrogen production sites selection in Algeria. Int. J. Hydrogen Energy 44 (60), 31808–31831. doi:10.1016/j.ijhydene.2019.10.099
Micangeli, A., Del Citto, R., Kiva, I., Santori, S., Gambino, V., Kiplagat, J., et al. (2017). Energy production analysis and optimization of mini-grid in remote areas: The case study of habaswein, Kenya. Energies 10 (12), 2041. doi:10.3390/en10122041
Mokveld, K., and Von Eije, S. (2018). Final energy report Mozambique. Netherlands: Netherlands Enterp. Agency Hague.
Moner-Girona, M., Ghanadan, R., Solano-Peralta, M., Kougias, I., Bodis, K., Huld, T., et al. (2016). Adaptation of feed-in tariff for remote mini-grids: Tanzania as an illustrative case. Renew. Sustain. Energy Rev. 53, 306–318. doi:10.1016/j.rser.2015.08.055
Moner-Girona, M., Solano-Peralta, M., Lazopoulou, M., Ackom, E. K., Vallve, X., and Szabó, S. (2018). Electrification of Sub-Saharan Africa through PV/hybrid mini-grids: Reducing the gap between current business models and on-site experience. Renew. Sustain. Energy Rev. 91, 1148–1161. doi:10.1016/J.RSER.2018.04.018
Monyei, C. G., and Adewumi, A. O. (2017). Demand Side Management potentials for mitigating energy poverty in South Africa. Energy Policy 111, 298–311. doi:10.1016/J.ENPOL.2017.09.039
Monyei, C. G., and Akpeji, K. O. (2020). Repurposing electricity access research for the Global South: A tale of many disconnects. Joule 4 (2), 278–281. doi:10.1016/J.JOULE.2019.11.013
Monyei, C. G., Adewumi, A. O., and Jenkins, K. E. H. (2018). Energy (in)justice in off-grid rural electrification policy: South Africa in focus. Energy Res. Soc. Sci. 44, 152–171. doi:10.1016/J.ERSS.2018.05.002
Monyei, C. G., Jenkins, K., Serestina, V., and Adewumi, A. O. (2018). Examining energy sufficiency and energy mobility in the global south through the energy justice framework. Energy Policy 119, 68–76. doi:10.1016/J.ENPOL.2018.04.026
Morstyn, T., Savkin, A. V., Hredzak, B., and Tuan, H. D. (2018). Scalable energy management for low voltage microgrids using multi-agent storage system aggregation. IEEE Trans. Power Syst. 33 (2), 1614–1623. doi:10.1109/TPWRS.2017.2734850
Motjoadi, V., Bokoro, P. N., and Onibonoje, M. O. (2020). A review of microgrid-based approach to rural electrification in South Africa: Architecture and policy framework. Energies 13 (9), 2193. doi:10.3390/en13092193
N R E C Association and others (2019). Guides for electric cooperative development and rural electrification. United States: NRECA Int. Ltd., Arlingt.
Nagabhushana, A. C., Jyoti, R., and Raju, A. B. (2011). “Economic analysis and comparison of proposed HRES for stand-alone applications at various places in Karnataka state,” in Proceeding of the ISGT2011-India, Kollam, India, December 2011 (IEEE), 380–385.
Nasir, M., Jin, Z., Khan, H. A., Zaffar, N. A., Vasquez, J. C., and Guerrero, J. M. (2018). A decentralized control architecture applied to DC nanogrid clusters for rural electrification in developing regions. IEEE Trans. Power Electron. 34 (2), 1773–1785. doi:10.1109/tpel.2018.2828538
Nfah, E. M., and Ngundam, J. M. (2012). Identification of stakeholders for sustainable renewable energy applications in Cameroon. Renew. Sustain. Energy Rev. 16 (7), 4661–4666. doi:10.1016/J.RSER.2012.05.019
Nhamo, G., and Mukonza, C. (2016). Policy, institutional and programme readiness for solar energy uptake in South Africa. Afr. Insight 45 (4), 69–90. doi:10.4314/ai.v45i4
Niyonteze, J. D. D., Zou, F., Asemota, G. N. O., and Bimenyimana, S. (2019). Solar-powered mini-grids and smart metering systems, the solution to Rwanda energy crisis. J. Phys. Conf. Ser. 1311 (1), 012002. doi:10.1088/1742-6596/1311/1/012002
Niyonteze, J. D. D., Zou, F., Norense Osarumwense Asemota, G., Bimenyimana, S., and Shyirambere, G. (2020). Key technology development needs and applicability analysis of renewable energy hybrid technologies in off-grid areas for the Rwanda power sector. Heliyon 6 (1), e03300. doi:10.1016/J.HELIYON.2020.E03300
Nkiriki, J., and Ustun, T. S. (2017). “Mini-grid policy directions for decentralized smart energy models in Sub-Saharan Africa,” in Proceeding of the 2017 IEEE PES innovative smart grid technologies conference europe (ISGT-Europe), Turin, Italy, September 2017 (IEEE), 1–6.
Nsafon, B. E. K., Butu, H. M., Owolabi, A. B., Roh, J. W., Suh, D., and Huh, J.-S. (2020). Integrating multi-criteria analysis with PDCA cycle for sustainable energy planning in Africa: Application to hybrid mini-grid system in Cameroon. Sustain. energy Technol. assessments 37, 100628. doi:10.1016/j.seta.2020.100628
Nuru, J. T., Rhoades, J. L., and Gruber, J. S. (2021). The socio-technical barriers and strategies for overcoming the barriers to deploying solar mini-grids in rural islands: Evidence from Ghana. Technol. Soc. 65, 101586. doi:10.1016/J.TECHSOC.2021.101586
Nygaard, I., Hansen, U. E., Mackenzie, G., and Pedersen, M. B. (2015). Measures for diffusion of solar PV in selected African countries. Int. J. Sustain. Energy 36 (7), 707–721. doi:10.1080/14786451.2015.1086768
Odarno, L., Sawe, E., Swai, M., Katyega, M. J. J., and Lee, A. (2017). Accelerating mini-grid deployment in sub-Saharan Africa: Lessons from Tanzania. United States: World Resources Institute.
Odoi-Yorke, F., Owusu, J. J., and Atepor, L. (2022). Composite decision-making algorithms for optimisation of hybrid renewable energy systems: Port of Takoradi as a case study. Energy Rep. 8, 2131–2150. doi:10.1016/j.egyr.2022.01.118
Ohunakin, O. S., Adaramola, M. S., Oyewola, O. M., and Fagbenle, R. O. (2014). Solar energy applications and development in Nigeria: Drivers and barriers. Renew. Sustain. Energy Rev. 32, 294–301. doi:10.1016/J.RSER.2014.01.014
Ortega-Arriaga, P., Babacan, O., Nelson, J., and Gambhir, A. (2021). Grid versus off-grid electricity access options: A review on the economic and environmental impacts. Renew. Sustain. Energy Rev. 143, 110864. doi:10.1016/j.rser.2021.110864
Oyewo, A. S., Aghahosseini, A., Ram, M., Lohrmann, A., and Breyer, C. (2019). Pathway towards achieving 100% renewable electricity by 2050 for South Africa. Sol. Energy 191, 549–565. doi:10.1016/J.SOLENER.2019.09.039
Paliwal, P., Patidar, N. P., and Nema, R. K. (2014). Planning of grid integrated distributed generators: A review of technology, objectives and techniques. Renew. Sustain. Energy Rev. 40, 557–570. doi:10.1016/J.RSER.2014.07.200
Patel, H., and Chowdhury, S. (2015). “Review of technical and economic challenges for implementing rural microgrids in South Africa,” in Proceeding of the 2015 IEEE Eindhoven PowerTech, Eindhoven, Netherlands, September 2015 (IEEE), 1–6.
Pedersen, M. B. (2016). Deconstructing the concept of renewable energy-based mini-grids for rural electrification in East Africa. Wiley Interdiscip. Rev. Energy Environ. 5 (5), 570–587. doi:10.1002/wene.205
Pedersen, M. B., and Nygaard, I. (2018). System building in the Kenyan electrification regime: The case of private solar mini-grid development. Energy Res. Soc. Sci. 42, 211–223. doi:10.1016/J.ERSS.2018.03.010
Pegels, A. (2010). Renewable energy in South Africa: Potentials, barriers and options for support. Energy Policy 38 (9), 4945–4954. doi:10.1016/j.enpol.2010.03.077
Peters, J., Sievert, M., and Toman, M. A. (2019). Rural electrification through mini-grids: Challenges ahead. Energy Policy 132, 27–31. doi:10.1016/J.ENPOL.2019.05.016
Pillot, B., Muselli, M., Poggi, P., and Dias, J. B. (2019). Historical trends in global energy policy and renewable power system issues in Sub-Saharan Africa: The case of solar PV. Energy Policy 127, 113–124. doi:10.1016/J.ENPOL.2018.11.049
Pohekar, S. D., and Ramachandran, M. (2004). Application of multi-criteria decision making to sustainable energy planning-a review. Renew. Sustain. energy Rev. 8 (4), 365–381. doi:10.1016/j.rser.2003.12.007
Psomopoulos, C. S., Ioannidis, G. C., Kaminaris, S. D., Mardikis, K. D., and Katsikas, N. G. (2015). A comparative evaluation of photovoltaic electricity production assessment software (PVGIS, PVWatts and RETScreen). Environ. Process. 2 (1), 175–189. doi:10.1007/s40710-015-0092-4
Puati Zau, A. T., and Daniel Chowdhury B, S. P. (2018). “Design of photo voltaic (PV) solar power plant to supply electricity and to pump water to chele community,” in Proceeding of the 2018 IEEE PES/IAS PowerAfrica, PowerAfrica, Cape Town, South Africa, June 2018 (IEEE), 821–826. doi:10.1109/POWERAFRICA.2018.8521177
Pueyo, A., and DeMartino, S. (2018). The impact of solar mini-grids on Kenya’s rural enterprises. Energy sustain. Dev. 45, 28–37. doi:10.1016/j.esd.2018.04.002
Ramakumar, R., Shetty, P. S., and Ashenayi, K. (1986). A linear programming approach to the design of integrated renewable energy systems for developing countries. IEEE Trans. Energy Convers. 4, 18–24. doi:10.1109/tec.1986.4765768
Reber, T., Booth, S., Cutler, D., Li, X., and Salasovich, J. (2018). Tariff considerations for micro-grids IN SUB-saharan africa. Accessed: Jun. 25, 2022. [Online]. Available: www.nrel.gov/publications.
Reber, T., and Booth, S. (2018). Tariff structures to encourage micro-grid deployment in sub-saharan africa: Review and recent trends. Curr. Sustain. Energy Rep. 53 (3), 199–204. doi:10.1007/S40518-018-0115-7
Renné, D., Kelly, M., Lilienthal, P., Gilman, P., Baring-Gould, I., Heimiller, D., et al. (2006). A strategic approach for promoting access to clean energy development. Int. Sol. Energy Conf. 2006.
Results (2020). Results | tracking SDG 7. Available at: https://trackingsdg7.esmap.org/results?p=Access_to_Electricity&i=Electricity_access_rate,_Total_(%25). accessed Jun. 25, 2022.
Rezk, H., Mukhametzyanov, I. Z., Al-Dhaifallah, M., and Zeiden, H. A. (2021). Optimal selection of hybrid renewable energy system using multi-criteria decision-making algorithms. Comput. Mat. \& Contin. 68 (2), 2001–2027. doi:10.32604/cmc.2021.015895
Safdar, T. (2017). Business models for mini-grids: Technical report 9. 2017. Cambridge, UK: Smart Villages Initiat. c/o Trinity Coll.
Scholtz, L., Muluadzi, K., Kritzinger, K., Mabaso, M., and Forder, S. (2017). Renewable Energy: Facts and Futures the energy future we want. Cape Town: Cape T.
Schwarzbözl, P., Eiden, U., Pitz-Paal, R., Zentrum, D., and Jones, S. (2006). A TRNSYS model library for solar thermal electric components (STEC) reference manual release 3.0.
Schwerhoff, G., and Sy, M. (2019). Developing Africa’s energy mix. Clim. Policy 19 (1), 108–124. doi:10.1080/14693062.2018.1459293
SEI (2016). Renewable energy mini-grids: An alternative approach to energy access in southern africa. Accessed, no. 28th April 2022.
Shao, M., Han, Z., Sun, J., Zhang, S., Zhao, Y., and Shao, Z. (2020). “A GIS-MCDM combination for the site assessment of wave energy power station in qingdao, eastern China,” in Preoceeding of the 30th International Ocean and Polar Engineering Conference, Virtual, October 2020 (IEEE).
Sheya, M. S., and Mushi, S. J. S. (2000). The state of renewable energy harnessing in Tanzania. Appl. Energy 65 (1–4), 257–271. doi:10.1016/S0306-2619(99)00116-6
Shimray, B. A., Singh, K. M., and Mehta, R. K. (2017). A survey of multi-criteria decision making technique used in renewable energy planning. Int. J. Comput. 4523, 124–140.
Shrestha, P., Shrestha, A., Shrestha, N. T., Papadakis, A., and Maskey, R. K. (2020). Assessment on scaling-up of mini-grid initiative: Case study of mini-grid in rural Nepal. Int. J. Precis. Eng. Manuf. Technol. 8 (1), 217–231. doi:10.1007/S40684-020-00190-X
Siddaiah, R., and Saini, R. P. (2016). A review on planning, configurations, modeling and optimization techniques of hybrid renewable energy systems for off grid applications. Renew. Sustain. Energy Rev. 58, 376–396. doi:10.1016/j.rser.2015.12.281
Sinha, S., and Chandel, S. S. (2014). Review of software tools for hybrid renewable energy systems. Renew. Sustain. Energy Rev. 32, 192–205. doi:10.1016/j.rser.2014.01.035
Somefun, T., Popoola, O., Abdulkareem, A., and Awelewa, A. (2022). Review of different methods for siting and sizing distributed generator. Int. J. Energy Econ. Policy 12 (3), 16–31. doi:10.32479/ijeep.12803
Sovacool, B. K., Burke, M., Baker, L., Kotikalapudi, C. K., and Wlokas, H. (2017). New frontiers and conceptual frameworks for energy justice. Energy Policy 105, 677–691. doi:10.1016/J.ENPOL.2017.03.005
Stritzke, S., and Jain, P. (2021). The sustainability of decentralised renewable energy projects in developing countries: Learning lessons from Zambia. Energies 14 (13), 3757. doi:10.3390/EN14133757
Temudo, M. P., Cabral, A. I. R., and Talhinhas, P. (2019). Petro-Landscapes: Urban expansion and energy consumption in mbanza kongo city, northern Angola. Hum. Ecol. 47 (4), 565–575. doi:10.1007/S10745-019-00088-6
Temudo, M. P., Cabral, A. I. R., and Talhinhas, P. (2020). Urban and rural household energy consumption and deforestation patterns in zaire province, northern Angola: A landscape approach. Appl. Geogr. 119, 102207. doi:10.1016/J.APGEOG.2020.102207
Tenenbaum, B., Greacen, C., Siyambalapitiya, T., and Knuckles, J. (2014). From the bottom up: How small power producers and mini-grids can deliver electrification and renewable energy in africa. Washington, DC: World Bank Publications.
Turcotte, D., Ross, M., and Sheriff, F. (2001). “Photovoltaic hybrid system sizing and simulation tools: Status and needs,” in PV horizon: Workshop on photovoltaic hybrid systems, 1–10.
Unwin, J. (2020). Solar power in South Africa: A look at the country’s solar Plants.2020. Sol. Energy Newsl. S. Afr. vol. Accessed, no. 28th April 2022.
Upadhyay, S., and Sharma, M. P. (2014). A review on configurations, control and sizing methodologies of hybrid energy systems. Renew. Sustain. Energy Rev. 38, 47–63. doi:10.1016/j.rser.2014.05.057
U. S. Agency, I. Development, and R. U. Commissioners (2020). Exploring africa ’ S mini-grid tariff exploring africa ’ S mini-grid tariff.
van Bracht, N., Grote, F., Fehler, A., and Moser, A. (2016). “Incorporating long-term uncertainties in generation expansion planning,” in Preoceeding of the 2016 13th International Conference on the European Energy Market (EEM), Porto, Portugal, June 2016 (IEEE), 1–5.
Villavicencio Calzadilla, P., and Mauger, R. (2017). The UN’s new sustainable development agenda and renewable energy: The challenge to reach SDG7 while achieving energy justice. J. Energy & Nat. Resour. Law 36 (2), 233–254. doi:10.1080/02646811.2017.1377951
von Breitenstein, A. (1999). ‘Enterprises of trust’ - sustainable development through local utilities for renewable energies in rural areas of Tanzania. Sustain. Dev. through Renew. Energies Tanzan.
Wagemann, B., and Manetsgruber, D. (2016). Risk management for mini-grid deployment in rural areas. Energy Procedia 103, 106–110. doi:10.1016/j.egypro.2016.11.257
Wagner, N., Rieger, M., Bedi, A. S., Vermeulen, J., and Demena, B. A. (2021). The impact of off-grid solar home systems in Kenya on energy consumption and expenditures. Energy Econ. 99, 105314. doi:10.1016/J.ENECO.2021.105314
Walters, T., Rai, N., Esterly, S., Cox, S., Reber, T., Muzammil, M., et al. (2015). Policies to spur energy access. Executive summary; volume 1, engaging the private sector in expanding access to electricity; volume 2, case studies to public-private models to finance decentralized electricity access. United States.
Winkler, H., Hughes, A., and Haw, M. (2009). Technology learning for renewable energy: Implications for South Africa’s long-term mitigation scenarios. Energy Policy 37 (11), 4987–4996. doi:10.1016/j.enpol.2009.06.062
Winkler, H. (2005). Renewable energy policy in South Africa: Policy options for renewable electricity. Energy Policy 33 (1), 27–38. doi:10.1016/S0301-4215(03)00195-2
Wolsink, M. (2020). Distributed energy systems as common goods: Socio-political acceptance of renewables in intelligent microgrids. Renew. Sustain. Energy Rev. 127, 109841. doi:10.1016/j.rser.2020.109841
Yusuf, S. S., and Mustafi, N. N. (2018). Design and simulation of an optimal mini-grid solar-diesel hybrid power generation system in a remote Bangladesh. Int. J. Smart Grids, ijSmartGrid 2 (1), 27–33.
Zeng, J., Li, M., Liu, J. F., Wu, J., and Ngan, H. W. (2010). “Operational optimization of a stand-alone hybrid renewable energy generation system based on an improved genetic algorithm,” in Proceeding of the IEEE PES general meeting, Minneapolis, MN, USA, July 2010 (IEEE), 1–6.
Zerhouni, F. Z., Zerhouni, M. H., Zegrar, M., Benmessaoud, M. T., Stambouli, A. B., and Midoun, A. (2010). Proposed methods to increase the output efficiency of a photovoltaic (PV) system. Acta Polytech. Hung. 7 (2), 55–70.
Zhang, X., Yang, J., Wang, W., Jing, T., and Zhang, M. (2020). Construction and application of evaluation indexes for rural micro-energy-grid. Nongye Gongcheng Xuebao/Transactions Chin. Soc. Agric. Eng. 36 (6). doi:10.11975/j.issn.1002-6819.2020.06.023
Glossary
Appendix
Keywords: off-grid mini-grids, renewable mini-grids, energy access, rural electrification, energy poverty, energy justice, Sub-Saharan Africa
Citation: Babayomi OO, Olubayo B, Denwigwe IH, Somefun TE, Adedoja OS, Somefun CT, Olukayode K and Attah A (2023) A review of renewable off-grid mini-grids in Sub-Saharan Africa. Front. Energy Res. 10:1089025. doi: 10.3389/fenrg.2022.1089025
Received: 03 November 2022; Accepted: 15 December 2022;
Published: 16 January 2023.
Edited by:
Fidelis Ibiang Abam, Michael Okpara University of Agriculture, NigeriaReviewed by:
Macmanus Ndukwu, Michael Okpara University of Agriculture, NigeriaOgheneruona E. Diemuodeke, University of Port Harcourt, Nigeria
Copyright © 2023 Babayomi, Olubayo, Denwigwe, Somefun, Adedoja, Somefun, Olukayode and Attah. This is an open-access article distributed under the terms of the Creative Commons Attribution License (CC BY). The use, distribution or reproduction in other forums is permitted, provided the original author(s) and the copyright owner(s) are credited and that the original publication in this journal is cited, in accordance with accepted academic practice. No use, distribution or reproduction is permitted which does not comply with these terms.
*Correspondence: Tobiloba E. Somefun, tobi.somefun@covenantuniversity.edu.ng