- 1Mechanical Engineering Department, Quaid-e-Awam University Engineering, Science and Technology (QUEST), Nawabshah, Pakistan
- 2Department of Mechanical Engineering, Mehran University of Engineering and Technology, Jamshoro, Pakistan
- 3Department of Mathematics, Quaid-e-Awam University Engineering, Science and Technology, Larkana, Pakistan
In this research, biodiesel fuel is produced using potassium hydroxide (KOH) as a catalyst and methanol as a co-solvent through the transesterification process. The analysis of particulate matter emissions from a compression ignition (CI) engine with pure diesel fuel (D100), biodiesel-mixed (B30), and clove oil (CL3000 ppm) was carried out. The findings obtained revealed 5.27% reduction of particulate matter in biodiesel-blended fuel, and furthermore, when clove was added to the biodiesel-blended fuel, 11.61% reduction of particulate matter was observed as compared to pure diesel. It may be due to the higher oxygen ratio present in clove oil. The engine was run for 100 h on each fuel sample, and the data were taken and tested per 25 h for elemental analysis. The results manifest that the metal concentration found different elements in lubricant oil, i.e., lead (51.84), (24.89%), and (23.255%); copper (47.41%), (28.71%), and (23.86%); nickel (37.88%), (32.32), and (29.78%); and cadmium (46.12), (29.87%), and (24.01%) in diesel, biodiesel-blended fuel, and biodiesel blended with clove oil, respectively. This study found better results for engine life, which shows the lower metal concentration in biodiesel-blended fuel and clove oil than diesel. Furthermore, the fuels investigated in this research can reduce the wear of engine parts because biodiesel and clove oil contain a high oxygen ratio. Moreover, it has been found that biodiesel and clove oil as an antioxidant have a positive impact on noise emission levels for the CI engine.
1 Introduction
The transportation industry, as a largest consumer of fossil fuels, requires a transition to renewable energy and environmentally favorable sources (Isa and Ganda, 2018). Biodiesel is easy to use, biodegradable, non-toxic, and virtually sulfur- and aromatic-free. It contains more oxygen than petroleum diesel and is utilized in diesel engines, which profound less emission in result of the particles of hydrocarbons, carbon monoxide, smoke, toxic substances, and noise, from diesel-powered cars. Furthermore, because vegetable oil-based fuel is derived from agricultural products, it does not contribute to net CO2 levels in the environment (Subramani et al., 2018). Renewable energies are reducing the negative impact of conventional fuels. They bring excellent benefits, which are the conventional fuel, sustainability, cleanliness, and environmental friendliness. The use of biodiesel in diesel engines has lately gained traction in this setting (Devi et al., 2019).
The biodiesel is a fatty acid methyl ester made by combining different seeds’ oil, waste oil, the animal’s fat, or wasted oil with catalysts, and alcohol. The capacity and efficiency of biodiesel synthesis are affected by the degree of saturation of these oils (Liu et al., 2019; Mirnezami et al., 2020). Unsaturated fatty acid methyl esters make up a significant percentage of biodiesel. An overload of methyl esters of unsaturated fatty acids (F.A.M.E) produces a micro-environment that is prone to autoxidation (Sundus et al., 2017). The organic acids, with the family of alkenes, alkynes, alkanes, aldehydes, ketones, and various polymers are among the oxidation products. The corrosion blockages, engine power imbalances, and other filtration difficulties are all caused by these chemicals. Consequently, biodiesel’s oxidative stability is critical for preservation and engine performance (Yang et al., 2017). The process of biodiesel production from edible animal and vegetable triglycerides is commonly used in practice. Using these sources for BD production, however, will compete with the food vs. fuel debate, raising the cost of BD manufacturing (Salaheldeen et al., 2015). Biodiesel hydrocarbons produce fewer emissions than diesel fuel, such as carbon dioxide and carbon monoxide (Dincer, 2008). Furthermore, biofuel has received a lot of interest as an alternative energy source because of its biodegradability, nontoxic nature, minimal harmful emissions, sustainability, feasibility, and good quality lubricity (Navas et al., 2020; Gouran et al., 2021). The transesterification of mustard oil into biodiesel is the subject of this article. Using 2.5% alkali methoxide as the catalyst, the maximum biodiesel yield was 84% (Navas et al., 2020).
Particulate matter is made up of very small particles (varying from nanometer to micrometer in size), which make it easier for them to penetrate into the lungs and trigger inflammation at the sites of deposition, making them dangerous (Ahmad et al., 2012; Ashraful et al., 2015). Alternative fuels in internal combustion (IC) engines have sparked interest in recent years due to the possibility for decreased emissions, such as particulate matter (PM) emissions (Rakopoulos et al., 2018a). The incomplete combustion in diesel engines, carbon monoxide (CO), hydrocarbons (HCs), nitrogen oxides (NO2), and particulate matter (PM) is all controlled. Unregulated emissions include formaldehyde, acetaldehyde, 1,3-butadiene, ethene, ethyne, propylene, and benzene, toluene, and xylene (BTX) (Cheung et al., 2009; Vaughan et al., 2015). Researchers are creating strategies to reduce particle emissions utilizing diverse ways such as fuel modification and after-treatment devices; therefore, PM characterization and toxicity analysis are still evolving. To address these issues, this work provides an overview of PM characteristics and health impacts, as well as the impact of various fuels (biodiesel, alcohol fuels, and oxygenated additives) on diesel engine PM emissions (Stevanovic et al., 2017; Rakopoulos et al., 2018b). The experimental results show reduction in CO, 5.1% HC, 4.6% NOx, and 3.00 k% of B100 smoke emissions when using 20% DTBP as an alternative fuel for a CI engine without modifications of the changes (Zhang et al., 2020). In the hybrid diesel engines, the use of liquid boron as an additive for engine lubrication oil in combination with vegetable oil was tested. The research focused on the engine performance, which includes power, torque, fuel consumption, and the specific fuel consumptions, and the exhaust emissions of O2, CO2, SO, CO2, HC, and NOx (Ganesan et al., 2019). The presence of oxygen in the biodiesel decreases HC and CO emissions while increasing NOx emissions, when using diesel engines (Öğüt et al., 2020). The effects of EHN cetane improvers on fuel quality and engine characteristics on diesel were observed; however, same efficiencies were examined without changing fuel properties on hazelnut oil and higher carbon alcohols such as n-butanol and 1-pentanol blends (Dhinesh and Annamalai, 2018).
Antioxidants reduce NOx emissions by reducing OH radical generation, which decreases the combustion temperature and suppresses the formation of peroxyl radicals (HO2) (Atmanli, 2016). Biodiesel is made up of methyl esters of fatty acids generated through the reaction of different seeds’ oil, waste oil, animal fats, or the oils with catalysts, and alcohol. Capacity and efficiency in biodiesel production are affected through the degree of saturation in oils and fats (Mirnezami et al., 2020). Among the most significant parts of biodiesel quality control is oxidation durability. For its high viscosity, water content, acidity, and peroxide degree, biodiesel oxidation is a difficult process. The oxidation stability of biodiesel is influenced by moisture and metal concentrations in the fuel, as well as heat and light exposure (Uğuz et al., 2019; Mastoi et al., 2022a). On double bond isomerization in the structure of free fatty acids, oxidation products have saturated structures. The density and viscosity of biodiesel are increased by these saturated molecules having a high molecular weight; the various fuels or hybrid oils taken as randomly finite grids through SM’s method can be implied (Schirmann et al., 2019; Mastoi et al., 2022b). The chemicals that degrade the oxidation stability can be prevented or limited by adding additives to biodiesel. For inhibiting the oxidation process, antioxidants can be used (Kreivaitis et al., 2013). Antioxidants are widely utilized as deoxidizers as a result of their actions. The most active and widely utilized antioxidants are phenolic groups. The anti-oxidants are not specifically provided the electrons and hydrogen; their stable radicals are also prevented fatty acid oxidation in bio-diesel structures (Bharti and Singh, 2020). The use of graphene nanoparticles in DSOME fuel mixes results in a considerable decrease in combustion duration, ignition delay time, improvement in the maximum pressure, and heat release rate at full load (Soudagar et al., 2019).
The use of aluminum oxide nanoparticles in HOME (B20) fuel improved the performance of the engine (Soudagar et al., 2020). When compared to other evaluated samples, B30 + DMC displayed a considerable improvement in engine performance (BTE) and carbon emissions. B30 + TiO2 also significantly improved engine characteristics (Mujtaba et al., 2021). When nanoparticles blend with biodiesel, it may increase the performance and reduce the emission of engine (Mujtaba et al., 2020; Yaqoob et al., 2022; Ahmed et al., 2023). Antioxidants (AHs) are an effective way to prevent oxidation of biodiesel without affecting fuel properties. The addition of antioxidants significantly slows the degradation of biodiesel (Balaji and Cheralathan, 2015). The addition of the clove extract antioxidant at various concentrations in biodiesel-blended fuel reduced NOX levels in the engine at full load (Jeyakumar and Narayanasamy, 2019). Clove oil has been found to be a more effective antioxidant than synthetic antioxidants such as BHA and BHT in lowering lipid oxidation (Gülçin et al., 2012).
The literature reviewed previously reflects that significant studies have been carried out to investigate the engine performance and emission using variety of fuels. However, to the best of our knowledge, a few studies are reported investigating the compression ignition engine parameters using clove oil.
Therefore, this study was designed with the aim to examine the metal concentration of the lubricant oil of the CI engine using diesel, biodiesel, and clove oil as fuel samples. The long term investigation of lubricant oil was performed as the main focus of the metal concentrations of lubricant oil. The analysis of particulate matter and noise emission was also performed as a secondary objective in this study.
2 Materials and methodology
2.1 Biodiesel
In this work, the renewable and biodegradable fuel was manufactured from mustard oil through transesterification, analyzing the qualities with ASTM standards. The work is initiated by the oil, which is extracted from mustard seeds. Through this transesterification process, the fatty acids from this acid content of the mustard oil are the source to produce biodiesel. The parameters are set within permissible ranges and also described in Figure 1.
The methodology is described step by step using the selection of feedstock and the conversion process, which is from seed to crude oil; the primary focus is the filtration process and heating. The process is stepped ahead to transesterification and purified through washing. The detailed steps are briefly described in Table 1. The steps are briefly explained for further process.
2.1.1 Selection of feedstock
The survey and selection of feedstock, as well as the processing of seed into crude oil, are described in this section. The feedstock availability and selection survey was carried out by visiting various agricultural farms in the city of Nawabshah. Then, based on the oil content and availability, the mustard seed was chosen as the feedstock. Mustard oil is an excellent feedstock for manufacturing, and because it is non-edible, it avoids the food vs. fuel debate.
2.1.2 Conversion of seed into crude oil
Mustard seeds yield varying amounts of crude oil and press cake, according to research. Table 2 shows the percentages of yield and crude oil. Heating in the compression region resulted in some losses. The temperature of the oil at the outlet was found to be in the range of 35–50°C, and the temperature of the press cake at the outlet was found to be in the range of 40–95°C. In the table, the oil yield and losses during crude oil extraction from mustard seed are presented. Mustard seeds produced the highest oil production of 18.9 kg (18.5 L).
2.1.3 Filtration and heating
The transesterification of biodiesel has been carried out for the manufacturing of biodiesel using various catalysts. In this study, sodium hydroxide was mixed with methanol to produce sodium methoxide, which was then employed as a catalyst for the transesterification reaction. Magnetic stirring was applied to stir in the mustard oil for 45 min at 60 rpm at 60°C.
2.2 Transesterification
The transesterification process was utilized in this study to produce biodiesel from mustard oil because the quality of the fuel is excellent; the transesterification process is the most favored method for producing biodiesel from a variety of feedstock shown Figure 2. Different alkali catalysts were employed to transesterify mustard oil for the manufacture of methyl esters. In this approach, a known amount of sodium hydroxide (NaOH) was dissolved in methanol (CH3OH) to generate sodium methoxide, which was utilized as a catalyst for the transesterification reaction. The catalyst was added to the mustard oil at 60°C and swirled with a magnetic stirrer for 45 min at 60 rpm.
After stirring was performed, the reaction mixture was set aside at room temperature for 10–12 h to settle down. The bottom layer of glycerol, the upper thin layer of soap, and the middle layer of fatty acid methyl esters are all different layers.
2.3 Washing and purification
After settling and separation, the final production of biodiesel was rinsed in pure water to eliminate impurities and suspended particles from the biodiesel and to prevent fungal activity. After this procedure, the moisture from pure biodiesel was removed by a heat treatment process shown in Figure 3.
The final process is to obtain biodiesel after purifying with the help of filtering and eliminating the impurities and suspended particles from biodiesel to prevent fungal activity shown in Figure 4.
3 Analysis of the exhaust particulate matter emission
The results of PM (particulate matter) emissions from diesel engines while running on diesel fuel and biodiesel-blended fuels were collected for this goal. The amount of pollution produced by an engine is determined by its speed, load, and fuel quality. Using diesel fuel and biodiesel-blended fuels at the same speed and under varied load conditions, four particle sizes (pm 1.0, pm 2.5, pm 7.0, and pm 10) were determined. The characterized PM in diameter of microns showed four particle sizes of PM (pm 1.0, pm 2.5, pm 7.0, and pm 10.)
Aerocet 531S is a small, handheld, battery-operated, and completely portable unit, as shown in Figure 5. This unit provides particle counts or mass PM measurements as stored data logged values, real-time networked data, or printed results. Four important mass size ranges (PM1, PM2.5, PM7, and PM10) are displayed in the mass mode and four popular cumulative particulate sizes (>0.5, 5.0, and 10.0 microns), in the count mode. These four mass ranges results were obtained at the location, four feet from the source. The tests were made upon all the blends and various loads and found according to the specification as desired shown Table 3.
3.1 Elemental analysis of lubricant oil
Endurance testing was completed for 100 h on diesel and biodiesel-blended fuels. A sliding contact between metallic components of any mechanical system inevitably results in wear, which produces minute metal particles. The piston, piston ring, cylinder liner, bearing, crankshaft, cam, tappet, and valves are all susceptible to wear and strain in diesel engines. In a lubrication system, wear particles remain suspended in oil. The engine was run at constant load and constant rpm. The engine has been run for 25 h for each fuel sample. After the completion of 25 h, lubricant oil samples were collected from the engine. Analysis of lubricant oil properties has been tested through different methods (multi elemental analysis).
3.2 Engine sound pressure level
Here, the noise levels (sound pressure level) of the CI engine have been analyzed. The engine specification shown in Table 4. Two fuel samples have been tested for sound pressure levels at different loading conditions and constant speed. The sound pressure level was measured from three directions such as front, back, and left. Three microphones have been installed at 1-m distance from the test bed at three different locations. The sound pressure level was measured using a dB meter. Detailed specifications are given in Table 5.
4 Result and discussions
4.1 Physico-chemical properties of mustard oil biodiesel
In this study, the basic parameters or fuel parameters of biodiesel, such as density, viscosity, moisture content, pour points, acid value, calorific value, sulfur, cetane number, and flash point, are concluded using the standard method, the evident following the manufacture of biodiesel from mustard oil through transesterification, as shown in Table 6.
4.2 Density
Diesel fuel has a lower density than biodiesel fuel, which is mostly determined by the fatty acid content and purity of biodiesel fuel, as biodiesel density is heavily influenced by contamination. The biodiesel fuel density has a significant impact on several engine fuel properties such as live viscosity, certain number, and combustion. According to the ASTM standard D-1298, the density of mustard biodiesel was calculated and determined to be 0.859.
4.3 Viscosity
The viscosity of gasoline is defined as the resistance of the relative moment of oil. Viscosity has a significant influence on engine start-up, fuel atomization, and fuel–air mixture combustion quality. Mustard oil biodiesel has a viscosity of 5.93 cP, which is within the typical range of 1.9–6.0 cP. In this study, the proportion of less and high viscosities on their extreme values was found to be insufficient combustion which is the result of blue and black smoke. In the studies of biodiesel, it should be specific viscosity that gets the proper droplet size (PDZ) for the complete combustion. The viscosity should be low with respect to temperature, as the ratio is, and if we decrease the temperature, then the viscosity of the bio-diesel is low as it will become more viscous at lower temperatures, causing further operational issues.
4.4 Pour point
Biodiesel has a greater pour point, making it useful in unfavorable situations. Diesel fuel starts at the pour point at a low temperature (decrease by a degree), which may no longer be in use, whereas diesel fuel has a lower pour point and can no longer be used. The pour points are mostly determined by the nature of the oil and feedstock. They determined to use the biodiesel production process and followed the standard suggested range for the bio-diesel. The pour points of the biodiesel lowest temperature at which it can be used have been observed, and when it reaches the pour point, the biodiesel is solidified and no longer for use.
4.5 Calorific value
When the biodiesel starts to burn, then the combustion products are going to be cooled down. Which is the initial temperature of the combustible mixture, the heating value, or the calorific value is the energy per quantity of the biodiesel. The heating (calorific) value of the synthesized biodiesel is determined and set at 40.1 MJ/g using an oxygen bomb calorimeter, which is within the permitted range for smooth engine operation.
4.6 The acid value
In this study, the acid value of mustard oil biodiesel synthesis is 0.78 mg KOH/g, where the value is in the biodiesel standard range. It is mostly dictated by the biodiesel purification method and FFA content. Because excessive levels of FFA complicate biodiesel recovery and harm engine components, the FFA concentration in biodiesel should be kept to a minimum. It has been discovered that using hot distilled water for washing and purifying raises the acid value.
4.7 The cetane number
It is governed by its molecular structure and the parent ester concentration, so for biodiesel, it increases with the increasing number of carbon atoms. The cetane number of biodiesel is higher than that of parent vegetable oil, and biodiesel will initiate ignition easily in the diesel engine while lowering noise as well. In biodiesel, the optimal cetane number range is in between 46 and 52, while in regular diesel, the range is between 40 and 55. Mustard oil biodiesel has a cetane value of 51, which is within the biodiesel standard range.
4.8 Particulate matter emission
The purpose of this research was to see how different fueling alternatives affected particle matter, such as diesel alone, biodiesel mixed, and biodiesel blended with clove oil Figure 6A. PM1.0 displays the findings of average percentages of particulate matter emission as D100 (42.61%), B30 (30.96%), and B30 + CL3000 ppm (26.42%), indicating that PM1.0 is greater in diesel fuel than in biodiesel mixed with clove oil. Figure 6B depicts PM2.5 outcomes such as (38%), (33.20%), and (29.61%), respectively, lower values in biodiesel and antioxidants. Figure 6C shows PM7 results (37.93%), (33.29%), and (26.77%) for D100, B300, and B30 + CL3000 ppm, respectively, and Figure 6D shows PM10 results of average percentages of particulate matter emission such as (38.03%), (34.62), and (27.30%); from the results shown in Figures 6A–D, the particulate matter emission levels of PM1, PM2.5, PM7, and PM10 were higher in diesel fuel. Therefore, biodiesel may be utilized as a diesel fuel alternative to meet energy demands, minimize reliance on fossil fuels, and reduce engine exhaust pollutants (Mofijur et al., 2013). The addition of clove oil in biodiesel was the reduction in particulate matter emissions (Rusli et al., 2022). Furthermore, adding clove oil to biodiesel as an additive improves the oxidative stability of the biodiesel. In comparison to biodiesel-mixed diesel, when antioxidants are added to biodiesel blends, the percentage of particulate matter emissions is lowered because antioxidant properties trap the free radical efficiently in the combustion process; hence, particulate matter emissions are considerably decreased.
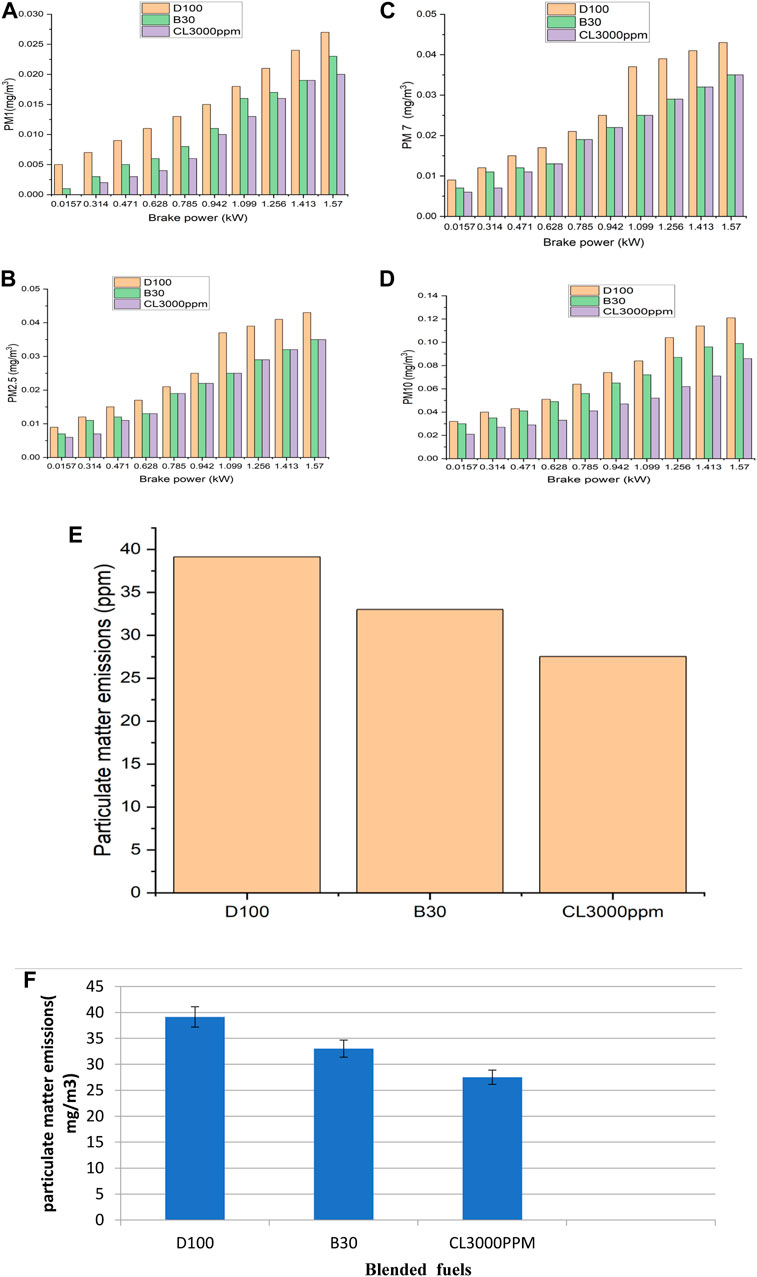
FIGURE 6. (A–D): Particulate emission matter vs. brake power. (E) Comparative results of different blended fuels. (F) Average error of particulate matter emission.
The average errors of particulate matter emissions from diesel, biodiesel, and clove-blended fuel samples are shown in Figure 6F and shown in Table 7. The figure clearly shows reduced average error of particulate matter emissions for biodiesel and clove oil compared to diesel fuel. This shows that biodiesel and clove oil fuels are more reliable for the diesel engine compared to diesel fuel.
4.9 Elemental analysis of lubricant oil
In this study, the analysis of lubricant oil for metal concentration was performed using diesel fuel (D100), biodiesel-blended fuel (B30), and biodiesel-blended fuel with clove oil (B30 + CL3000 ppm). The engine runs for 100 h on each fuel sample. After 25 h, lubricant oil samples were collected for analysis. According to the results in Figure 7A, the most likely source of lead (Pb) wear in debris is the wear of bearing paints and grease addition. The results showed that the lead content is higher when used in diesel than that in biodiesel and biodiesel blended with clove oil as an antioxidant. Copper (Cu) wear in engine oil samples is one of the most serious issues for biodiesel engines, as shown in Figure 7B. The concentration could be obtained because of the wear of the bearings, bronze, and bushings. Figure 7B shows the copper concentration in engine oil samples. It was lower in biodiesel and clove blended than in diesel fuel. Figure 7C shows that the source of nickel (NI) bearing piston nickel concentration is higher in diesel fuel than in biodiesel-mixed fuel. Figure 7D depicts bushes, cylinder linear shafts, and other CI engine components as the most common (Cd) source of wear in lubrication oil. The results are greater in diesel fuel than in B100 and clove oil additives. The average percentage of metal concentration is lower than diesel than that in biodiesel-blended fuel and biodiesel blended with clove oil. It has observed that reduction may occur using biodiesel and clove oil-blended fuel sample. Developed reduction in cylinder the internal pressure in the engine which consequently reduce the frictional forces acting on the moving parts may cause reducing the wear in engine moving parts. In other words, biodiesel improves the fuel lubricity and raises the cetane number of fuel. It happens due to its properties which provide sufficient lubrication and, in contrast, reduces wear to a larger extent. The average errors of the noise emission level from diesel, biodiesel, and clove-blended fuel samples are shown in Figure 6F. The figure shows reduced average error of the noise emission level of compression ignition engine reduction in case of biodiesel and clove oil-blended fuels.
4.10 Noise emission level
Figures 8–10 results illustrated the noise emission level of the CI engine at various load conditions and constant rpm 1,500 by using diesel fuel D100, biodiesel blend B30, and biodiesel-blended fuel with clove oil (3,000 ppm) as an antioxidant. Three positions were selected for the analysis of the noise emission level. Front, back, and left sides were chosen at 1 m distance from the piston head. All comparative results demonstrated that the engine using the diesel fuel D100 has high noise emissions than biodiesel B30 and CL3000 ppm. The outcome clearly shows that owing to biodiesel blend B30, clove oil has better oxygen contents than diesel fuel D100 which declines the noise emission. A higher oxygen content in clove oil is the reason why proper combustion takes place inside the engine. Proper combustion inside the engine may cause reduced noise levels when using clove oil compared with diesel and blended biodiesel.
4.11 Error analysis and standard deviation
In this section, error analysis and standard deviation are employed to demonstrate the approval of the proposed models.
The systematics set of procedure in the experimental data used to obtain the errors is known as uncertainty analysis. In this systematic procedure, we follow the various types of methods to reduce the uncertainty shown in Figure 11.
In this research, the inaccuracies are arising because of the various components, like electronic and mechanical, environmental, and the human miscalculations. The information is gathered at standard conditions, and the specifications and details of all the components are already available. Measurement errors which come from several different sources are classified into bias and precision errors; throughout the experiment, the bias errors remain constant. Furthermore, the uncertainty is the proportion of uncertainty, the testing parameter with the ratio of uncertainty emission, and the evaluated parameters. The measurement uncertainty is estimated as the experiments are carried out. The uncertainty measurements using standard parameters are explained in this section. The propagation of uncertainty for parameters that have been determined on the factors depending on the given independent parameters is determined using the following equations.
where
N = total particulate matter emission of fuel.Standard deviation of D100 = 0.00326.
Standard deviation of B30 = 0.00461.
Standard deviation of CL3000 ppm = 0.00522.
6 Conclusion
The goal of this study was to make biodiesel from mustard oil by transesterification and analyze the qualities of biodiesel according to ASTM standards. The oil was extracted from mustard seeds using a mechanical expeller, and the total amount of the extruded oil yielded 47% and 48% press cake, respectively, with 5% losses. Through the transesterification process, the fatty acid content of the mustard oil was lowered, and the final biodiesel was produced.
• All parameters of mustard biodiesel fuel were attained within permissible ranges.
• The particulate matter emission of the C.I. engine was analyzed, and the results found 5.27% reduction in biodiesel fuel and 11.6% reduction in clove oil-blended fuel as compared to diesel fuel.
• The metal concentration of lubricant oil has been investigated, and different elements were found such as lead (51.84), (24.89%), and (23.255%); copper (47.41%), (28.71%), and (23.86%); nickel (37.88%), (32.32), and (29.78%); and cadmium (46.12), (29.87%), and (24.01%); it was observed that the metal concentration was lower in biodiesel and clove oil-blended fuel.
• The noise emission of the B30 and clove oil was obtained lower than the D100. This happened due to the physical properties and richness of oxygen contents in biodiesel fuel and clove oil-blended fuel.
Finally, we suggested that clove oil as a fuel additive is more feasible due to its better results. The clove oil as an antioxidant showed a significant reduction in particulate matter and noise emission of the engine, reducing the wear of the engine as compared to diesel fuel.
In the future, a long endurance test should be conducted to investigate the effect of fuel additives and deposition formation on the diesel engine components.
Data availability statement
The raw data supporting the conclusion of this article will be made available by the authors, without undue reservation.
Author contributions
SB: conceptualization, data curation, formal analysis, investigation, methodology, visualization, and writing—original draft. LM: conceptualization, formal analysis, writing—review and editing, supervision, and project administration. ML: conceptualization, formal analysis, writing—review and editing, supervision, and project administration. LK: conceptualization, formal analysis, writing—review and editing, supervision, and project administration. UR: formal analysis, visualization, and writing—review and editing. SM: formal analysis, visualization, and writing—review and editing.
Acknowledgments
The authors specially thank QUEST Pakistan, for support throughout in this research work.
Conflict of interest
The authors declare that the research was conducted in the absence of any commercial or financial relationships that could be construed as a potential conflict of interest.
Publisher’s note
All claims expressed in this article are solely those of the authors and do not necessarily represent those of their affiliated organizations, or those of the publisher, the editors, and the reviewers. Any product that may be evaluated in this article, or claim that may be made by its manufacturer, is not guaranteed or endorsed by the publisher.
References
Ahmad, M., Sadia, H., Zafar, M., Sultana, S., Khan, M. A., and Khan, Z. (2012). The production and quality assessment of mustard oil biodiesel: A cultivated potential oil seed crop. Energy Sources, Part A Recovery, Util. Environ. Eff. 34, 1480–1490. doi:10.1080/15567036.2011.596907
Ahmed, S. A., Soudagar, M. E. M., Rahamathullah, I., Basha, J. S., Khan, T. M. Y., Syed, J., et al. (2023). Investigation of ternary blends of animal fat biodiesel-diethyl ether-diesel fuel on CMFIS-CI engine characteristics. Fuel 332, 126200. doi:10.1016/j.fuel.2022.126200
Ashraful, A. M., Masjuki, H. H., and Kalam, M. A. (2015). Particulate matter, carbon emissions and elemental compositions from a diesel engine exhaust fuelled with diesel–biodiesel blends. Atmos. Environ. 120, 463–474. doi:10.1016/j.atmosenv.2015.09.028
Atmanli, A. (2016). Effects of a cetane improver on fuel properties and engine characteristics of a diesel engine fueled with the blends of diesel, hazelnut oil and higher carbon alcohol. Fuel 172, 209–217. doi:10.1016/j.fuel.2016.01.013
Balaji, G., and Cheralathan, M. (2015). Experimental investigation of antioxidant effect on oxidation stability and emissions in a methyl ester of neem oil fueled DI diesel engine. Renew. Energy 74, 910–916. doi:10.1016/j.renene.2014.09.019
Bharti, R., and Singh, B. (2020). Green tea (Camellia assamica) extract as an antioxidant additive to enhance the oxidation stability of biodiesel synthesized from waste cooking oil. Fuel 262, 116658. doi:10.1016/j.fuel.2019.116658
Cheung, K. L., Polidori, A., Ntziachristos, L., Tzamkiozis, T., Samaras, Z., Cassee, F. R., et al. (2009). Chemical characteristics and oxidative potential of particulate matter emissions from gasoline, diesel, and biodiesel cars. Environ. Sci. Technol. 43 (16), 6334–6340. doi:10.1021/es900819t
Devi, A., Das, V. K., and Deka, D. (2019). A green approach for enhancing oxidation stability including long storage periods of biodiesel via Thujaoreantalis L. as an antioxidant additive. Fuel 253, 1264–1273. doi:10.1016/j.fuel.2019.05.127
Dhinesh, B., and Annamalai, M. (2018). A study on performance, combustion and emission behaviour of diesel engine powered by novel nanonerium oleander biofuel. J. Clean. Prod. 196, 74–83. doi:10.1016/j.jclepro.2018.06.002
Dincer, K. (2008). Lower emissions from biodiesel combustion. Energy Sources Part A Recovery Util. Environ. Eff. 30, 963–968. doi:10.1080/15567030601082753
Ganesan, S., Rathinam, S., Sajin, J. B., Subbiah, G., and Devarajan, Y. (2019). Performance and emission study on the effect of oxygenated additive in neat biodiesel fueled diesel engine. Energy Sources A Recovery, Util. Environ. Eff. 41, 2017–2027. doi:10.1080/15567036.2018.1549148
Gouran, A., Aghel, B., and Nasirmanesh, F. (2021). Biodiesel production from waste cooking oil using wheat bran ash as a sustainable biomass. Fuel 295 (2021), 120542. doi:10.1016/j.fuel.2021.120542
Gülçin, İ., Elmastaş, M., and Aboul-Enein, H. Y. (2012). Antioxidant activity of Clove oil–A powerful antioxidant source. Arabian J. Chem. 5 (4), 489–499. doi:10.1016/j.arabjc.2010.09.016
Isa, Y. M., and Ganda, Y. M. (2018). Bio-oil as a potential source of petroleum range fuels. Renew. Sustain. Energy Rev. 81, 69–75. doi:10.1016/j.rser.2017.07.036
Jeyakumar, N., and Narayanasamy, B. (2019). Clove as antioxidant additive in diesel–biodiesel fuel blends in diesel engines. Int. J. Green Energy 16 (4), 284–292. doi:10.1080/15435075.2018.1562931
Kreivaitis, R., Gumbytė, M., Kazancev, K., Padgurskas, J., and Makarevičienė, V. (2013). A comparison of pure and natural antioxidant modified rapeseed oil storage properties. Industrial Crops Prod. 43, 511–516. doi:10.1016/j.indcrop.2012.07.071
Liu, W., Lu, G., Yang, G., and Bi, Y. (2019). Improving oxidative stability of biodiesel by cis-trans isomerization of carbon-carbon double bonds in unsaturated fatty acid methyl esters. Fuel 242, 133–139. doi:10.1016/j.fuel.2018.12.132
Mastoi, S., Ganie, A. H., Saeed, A. M., Ali, U., Rajput, U. A., and Othman, W. A. (2022). Numerical solution for two-dimensional partial differential equations using SM’s method. Open Phys. 20 (1), 142–154. doi:10.1515/phys-2022-0015
Mastoi, S., Kalhoro, N. B., Mugheri, A. B., Rajput, S. A., Mior Othman, W. A., and &Nallasamy, K. (2022). A simple introduction to the SM’s method. Sci. 3 (2), 41–56.
Mirnezami, S. A., Zahedi, A., and Nejad, A. S. (2020). Thermal optimization of a novel solar/hydro/biomass hybrid renewable system for production of low-cost, high-yield, and environmental-friendly biodiesel. Energy 202, 117562. doi:10.1016/j.energy.2020.117562
Mofijur, M., Atabani, A. E., al Masjuki, H. H., Kalam, M. A., and Masum, B. M. (2013). A study on the effects of promising edible and non-edible biodiesel feedstocks on engine performance and emissions production: A comparative evaluation. Renew. Sustain. energy Rev. 23, 391–404. doi:10.1016/j.rser.2013.03.009
Mujtaba, M. A., Kalam, M. A., Masjuki, H. H., Gul, M., Soudagar, M. E. M., Ong, H., et al. (2020). Comparative study of nanoparticles and alcoholic fuel additives-biodiesel-diesel blend for performance and emission improvements. Fuel 279, 118434. doi:10.1016/j.fuel.2020.118434
Mujtaba, M. A., Cho, H. M., Masjuki, H. H., Farooq, M. A. K, M., Soudagar, M., Gul, M., et al. (2021). Effect of alcoholic and nano-particles additives on tribological properties of diesel–palm–sesame–biodiesel blends. Energy Rep. 7 (2021), 1162–1171. doi:10.1016/j.egyr.2020.12.009
Navas, M. B., Ruggera, J. F., Lick, I. D., and Casella, M. L. (2020). A sustainable process for biodiesel production using Zn/Mg oxidic species as active, selective and reusable heterogeneous catalysts. Bioresour. Bioprocess. 7 (1), 4–13. doi:10.1186/s40643-019-0291-3
Öğüt, H., Oğuz, H., Aydın, F., Ciniviz, M., and Deveci, H. (2020). The effects of the use of vegetable oil based as engine lubrication oil on engine performance and emissions in diesel engines. Energy Sources, Part A Recovery, Util. Environ. Eff. 42, 2381–2396. doi:10.1080/15567036.2019.1668507
Rakopoulos, D. C., Rakopoulos, C. D., Giakoumis, E. G., and Papagiannakis, R. G. (2018). Evaluating oxygenated fuel’s influence on combustion and emissions in diesel engines using a two-zone combustion model. J. Energy Eng. 144 (4), 04018046. doi:10.1061/(asce)ey.1943-7897.0000556
Rakopoulos, C. D., Rakopoulos, D. C., Mavropoulos, G. C., and Kosmadakis, G. M. (2018). Investigating the EGR rate and temperature impact on diesel engine combustion and emissions under various injection timings and loads by comprehensive two-zone modeling. Energy 157, 990–1014. doi:10.1016/j.energy.2018.05.178
Rusli, M., Septyan, M. A., and Farobie, O. (2022). Effect of bio-additive derived from essential oils on particulate matter and water content of B30 (30% of biodiesel blended fuel) IOP conference series: Earth and environmental science. IOP Conf. Ser. Earth Environ. Sci. 1034 (1), 012057. doi:10.1088/1755-1315/1034/1/012057
Salaheldeen, M., Mohamed, K., Mariod, A. A., Cheng, S., Abdelrahman, M. A., and Atabani, A. E. (2015). Physicochemical characterization and thermal behavior of biodiesel and biodiesel–diesel blends derived from crude Moringa peregrina seed oil. Energy Convers. Manag. 92, 535–542. doi:10.1016/j.enconman.2014.12.087
Schirmann, J. G., Karina, G., Dekker, R. F. H., Borsato, D., and Barbosa-Dekker, A. M. (2019). 3, 3′, 5, 5′-tetramethoxybiphenyl-4, 4′-diol: A new antioxidant enhancing oxidative stability of soybean biodiesel. Fuel 237, 593–596. doi:10.1016/j.fuel.2018.10.044
Soudagar, M. E. M., Nik-Ghazali, N. N., Kalam, M. A., Badruddin, I. A., Banapurmath, N. R., Khan, T. M. Y., et al. (2019). The effects of graphene oxide nanoparticle additive stably dispersed in dairy scum oil biodiesel-diesel fuel blend on CI engine: Performance, emission and combustion characteristics. Fuel 257, 116015. doi:10.1016/j.fuel.2019.116015
Soudagar, M. E. M., Nik-Ghazali, N. N., Kalam, M. A., Badruddin, I. A., Banapurmath, N. R., Bin Ali, M. A., et al. (2020). An investigation on the influence of aluminium oxide nano-additive and honge oil methyl ester on engine performance, combustion and emission characteristics. Renew. Energy 146, 2291–2307. doi:10.1016/j.renene.2019.08.025
Stevanovic, S., Vaughan, A., FarzanehHedayat, F., Rahman, Md M., Ali, Z., Brown, R. A., et al. (2017). Oxidative potential of gas phase combustion emissions-An underestimated and potentially harmful component of air pollution from combustion processes. Atmos. Environ. 158, 227–235. doi:10.1016/j.atmosenv.2017.03.041
Subramani, L., Parthasarathy, M., Balasubramanian, D., and Ramalingam, K. M. (2018). Novel Garcinia gummi-gutta methyl ester (GGME) as a potential alternative feedstock for existing unmodified DI diesel engine. Renew. energy 125, 568–577. doi:10.1016/j.renene.2018.02.134
Sundus, F., Fazal, M. A., and Masjuki, H. H. (2017). Tribology with biodiesel: A study on enhancing biodiesel stability and its fuel properties. Renew. Sustain. Energy Rev. 70, 399–412. doi:10.1016/j.rser.2016.11.217
Uğuz, G., Atabani, A. E., Mohammed, M. N., SuthaShobana, S. U., Kumar, G., Ala'a, H., et al. (2019). Fuel stability of biodiesel from waste cooking oil: A comparative evaluation with various antioxidants using FT-IR and dsc techniques. Biocatal. Agric. Biotechnol. 21, 101283. doi:10.1016/j.bcab.2019.101283
Vaughan, A., Stevanovic, S., Morrison, L., Rahman, M., Ali, Z., Miljevic, B., et al. (2015). Removal of organic content from diesel exhaust particles alters cellular responses of primary human bronchial epithelial cells cultured at an air-liquid interface. J. Environ. Anal. Toxicol. 5, 316. Article-number.316. 25. doi:10.4172/2161-0525.1000316
Yang, J., He, Q. S., Corscadden, K., and Caldwell, C. (2017). Improvement on oxidation and storage stability of biodiesel derived from an emerging feedstock camelina. Fuel Process. Technol. 157, 90–98. doi:10.1016/j.fuproc.2016.12.005
Yaqoob, H., Teoh, Y. H., Sher, F., Ahmad Jamil, M., Ali, M., Ağbulut, Ü., et al. (2022). Energy, exergy, sustainability and economic analysis of waste tire pyrolysis oil blends with different nanoparticle additives in spark ignition engine. Energy 251 (2022), 123697. doi:10.1016/j.energy.2022.123697
Zhang, Y., Niu, S., Lu, C., Gong, Z., and Hu, X. (2020). Catalytic performance of NaAlO2/γ-Al2O3 as heterogeneous nanocatalyst for biodiesel production: Optimization using response surface methodology. Energy Convers. Manag. 203, 112263. doi:10.1016/j.enconman.2019.112263
Nomenclature
ASTM American Society For Testing Materials
B30% biodiesel 30%
Bp brake power
C.I compression ignition
Cd cadmium
Ci nickel
Clo clove oil
Cu copper
D100 diesel 100%
Db Decibel
FFA free fatty acid
kW kilowatt
Pb lead
PM particulate matter
PPM parts per million
Keywords: biodiesel, mustard oil, clove oil, CI engine, noise emission level
Citation: Bhangwar S, Liaquat AM, Luhar MR, Abbasi A, Kumar L, Rajput UA and Mastoi S (2022) Production of biodiesel and analysis of exhaust particulate emissions and metal concentration of lubricant oil of the compression ignition engine. Front. Energy Res. 10:1057611. doi: 10.3389/fenrg.2022.1057611
Received: 29 September 2022; Accepted: 04 November 2022;
Published: 24 November 2022.
Edited by:
Muhammad Jamshaid, Bahauddin Zakariya University, PakistanReviewed by:
Abdülvahap Çamak, Samsun University, TurkeyManzoore Elahi M. Soudagar, Chandigarh University, India
Alpaslan Atmanli, National Defense University, Turkey
Copyright © 2022 Bhangwar, Liaquat, Luhar, Abbasi, Kumar, Rajput and Mastoi. This is an open-access article distributed under the terms of the Creative Commons Attribution License (CC BY). The use, distribution or reproduction in other forums is permitted, provided the original author(s) and the copyright owner(s) are credited and that the original publication in this journal is cited, in accordance with accepted academic practice. No use, distribution or reproduction is permitted which does not comply with these terms.
*Correspondence: Sajjad Bhangwar, c2FqamFkYmhhbmd3YXJAcXVlc3QuZWR1LnBr