- 1Jiangsu Maritime Institute, Nanjing, China
- 2School of Energy and Environment, Southeast University, Nanjing, China
Building energy, particularly air conditioning energy, makes for a significant fraction of overall societal energy usage. The heat island effect is a common urban environmental concern that threatens human sustainable development. Roofs, which cover more than 20% of the total metropolitan area, play an essential role in mitigating the urban heat island effect and lowering building energy use. To examine the triple beneficial benefits of cool roofs and green roofs on building energy conservation in different temperature zones in China, a simulation model based on a typical residential community is established. The results reveal that minimizing direct heat acquisition from the roof, reducing heat transfer from the enclosure, and enhancing the coefficient of performance have a surprising triple effect on building energy saving. In cold areas, hot summer and cold winter areas, and hot summer and warm winter areas, cool roofs may lower the regional ambient temperatures by 2°C, 2.3°C, and 2.6°C, respectively, whereas green roofs can reduce the regional ambient temperatures by 1°C, 1.1°C, and 1.2°C. The triple saving impact of cool roofs and green roofs may accomplish 11.0%, 11.5%, 12.6%, and 9.4%, 8.1%, and 9.3%, respectively, for building energy conservation. Because of the increased solar radiation, cool roofs perform better in low-latitude zones, whereas green roofs function consistently.
1 Introduction
As a result of the degree of social urbanization, the share of building energy consumption in total social energy consumption has steadily increased, and it is presently over 30% (Cai et al., 2009; Lin and Yang, 2018; Yang et al., 2019). In building energy consumption, the energy consumption of air conditioning systems varies from 25% to 50%, according to different building types (Chua et al., 2013; Guixian Liu et al., 2021). It can be seen that the energy consumption of air conditioning systems plays an important role in building energy consumption. The building roof has grown from the fundamental structural needs to a building energy-saving platform that complements the internal thermal climate and building energy consumption as the “Fifth Facade” of the structure (Guanpeng Liu et al., 2021). Making full use of building roof resources and implementing relevant energy-saving technology will help minimize urban energy consumption and meet China’s objective of “carbon peak and carbon neutrality” as soon as possible.
At the same time, with the rapid development of the world economy and the intensification of the process of urbanization, urban land is expanding indiscriminately, the urban population is expanding on a large scale, and the regional building density is increasing, which has caused great pressure on the ecological environment of the whole city. The shift in the thermal environment caused by the heat island effect has gained increasing attention as a significant element impacting the urban environmental quality (Yang et al., 2020). The heat island effect (UHI) refers to the phenomena of island-like high temperatures in urban areas produced by the quick rise in air temperature caused by the huge quantity of radiation received by underlying surfaces such as buildings and roads in the metropolitan region and the heat radiated into the air (Deilami et al., 2018). The annual average temperature in the city can be 2°C higher than that in the suburbs due to the concentration of urban population, developed industries, traffic congestion, severe air pollution, and the fact that buildings in the city are typically built of stone and concrete with low heat capacity and high thermal conductivity. Coupled with the blocking or weakening effect of buildings on the wind, the city is like a warm island in the spatial distribution of temperature, thus forming the urban heat island effect. The scale of the heat island effect covers from the building community to the overall situation of the city, which is affected by the local urban building cluster planning and surface coverage layout. The urban heat island not only leads to changes in climate characteristics, the reduction of life comfort, and the change of living habits of species but also increases the energy consumption of building cooling and accelerates the social energy consumption. Santamouris’ research shows that in Greece, the urban heat island leads to a twice cooling load of buildings in summer, a triple peak power consumption used for cooling, and a reduction of the cooling efficiency by 25% (Santamouris, 2013b).
According to the previous literature, the roof area of buildings in the city accounts for more than 25% of the total area (Akbari and Rose, 2008), and the roof is the most intense and direct part of buildings receiving solar radiation. As a result, the roof’s performance characteristics have a direct impact on the degree of building energy consumption, as well as a major impact on the urban heat island effect. Many researchers have investigated the active and passive roles of roofs in lowering urban heat islands and building energy consumption, with cool roofs and green roofs having the most visible influence (Takebayashi and Moriyama, 2007; Rizwan et al., 2008; Susca et al., 2011; Li and Norford, 2016; Saber et al., 2019; Saber and Maref, 2019).
The research on the impact of green roofs on the heat island effect mainly focuses on green space coverage and the mitigation of green roofs on the heat island effect. Armson showed that the greening function can achieve a good result in regulating the urban climate environment. In his report, the regulation of the urban surface temperature could be reduced by 24°C. Vegetation individuals mainly use canopy shading and transpiration to consume a large amount of energy to achieve the effect of cooling (Armson et al., 2012). Saito reported that the change of green coverage from 0 to 100% could reduce the urban temperature by about 2.7°C (Saito et al., 1990). Rosenzweig‘s model in Los Angeles, United States, showed that the green roof reduces the urban peak temperature by 0.86°C and the daily average temperature by 0.3–0.55°C under the sunlight in summer (Rosenzweig et al., 2006). Sun’s experimental study in Taipei showed that the average temperature drop of buildings with green roofs can reach 0.26°C, and the maximum temperature drop during the daytime is 1.6°C (Sun et al., 2012). A number of related research reports have demonstrated that green roofs have an effect on the decreasing urban heat island and ambient temperature, although their mitigation effect is influenced by the climate features of different locations and the types of city structures.
As for another type of passive roof, the cool roof, Rosenfeld’s early test (Rosenfeld et al., 1998) in Los Angeles showed that the increase in roof reflectivity per 0.1 could lead to a decrease in the average ambient temperature of 0.51°C. Levinson and Akbari of the heat island group of the Lawrence Berkeley National Laboratory in the United States have conducted a lot of research on the urban heat island effect caused by the selection of different pavement/roof materials and the corresponding environmental and ecological impacts from the heat island effect model calculation and experimental research for many years (Akbari et al., 2016, 2001; Levinson et al., 2007; 2005a; 2005b). Santamouris conducted extensive research on the heat island effect, from the cause of the heat island to the impact on energy and the environment, and from analysis and calculation tools to the implementation of response measures, providing a comprehensive theoretical foundation for the study of the urban heat island effect (Santamouris et al., 2015, 2011; Santamouris, 2013b; Santamouris, 2013a; Santamouris, 2014).
According to the literature study, green roofs and cool roofs can reduce urban heat islands and building energy consumption, although their effectiveness is impacted by local climatic conditions and building types. At the moment, quantitative research on the heat island and roof technologies under China’s climatic conditions is lacking. At the same time, there is a threefold linkage between the impact of green roofs and cool roofs on building energy usage. In the summer, two major elements contribute to the development of the indoor cooling load: solar radiation and external heat transfer. Cool roofs and green roofs may significantly enhance the thermal properties of the roof and minimize heat uptake from solar radiation. This is the roof’s first beneficial influence on building energy usage. At the same time, cool roofs and green roofs can reduce the urban heat island effect, lower the ambient temperature around the building, reduce the temperature difference between indoor and outdoor heat transfer, and lower the heat transferred from the surrounding environment to the room through the enclosure structure, lowering the energy consumption of building air conditioning. This is the roof’s second beneficial effect on building energy usage. Finally, lowering the ambient temperature aids in lowering the condensation temperature of refrigeration operations, improving the operation efficiency of air conditioning refrigeration units, and lowering the energy consumption of building air conditioning. This is the third beneficial effect of the roof on building energy usage. The prior study focused mostly on the first two impacts, with a lack of quantitative investigation of the third beneficial impact of the roof on building energy usage.
This paper comprehensively considers the triple superposition effect of cool roofs and green roofs on building energy conservation and urban heat island mitigation. The working characteristics of cool roofs and green roofs in different climatic areas to alleviate the urban heat island effect and reduce building energy consumption are determined through iterative calculation and simulation of a typical Chinese building community in typical meteorological areas in China. This can serve as a theoretical foundation for the practical implementation of roof technology.
2 Methodology
The simulation object in this work is a multi-story high-rise composite residential complex in Weifang, Shandong Province. The community is a typical clustered Chinese residential community, with a total area of about 160,000 m2, a total construction area of about 420,000 m2, a plot ratio of 2.6, and a community greening rate of 28%. The overview of the building layout is shown in Figure 1. Phoenix software is selected to simulate the thermal environment of the community.
According to a prior study, in severe cold areas of China, the negative effect of cool roof heating in winter is larger than the beneficial effect of cooling in summer, and air conditioning is not necessary for cooling and heating in mild areas. Therefore, this study mainly compares the impact of cool roofs and green roofs on the mitigation of the heat island effect and building energy consumption in cold areas, hot summer and cold winter areas, and hot summer and warm winter areas in China in summer conditions. Under the working condition of a typical design day in summer, Phoenix software is used to compare the changes in the overall thermal environment of the community under different climate regions and different roof technologies (as shown in Figure 2 and Figure 3). The air temperature at 5 m, 10 m, 15 m, and 20 m away from the ground is obtained respectively, and the average value is taken as the corrected ambient temperature. The temperature difference between ordinary roofs, cool roofs, and green roofs is compared by the overall average temperature.
To replace the original ambient temperature, the corrected ambient temperature is entered into the EPW meteorological file, and EnergyPlus software is used to simulate the building energy usage under the new ambient temperature. In EnergyPlus, the space–time modulated cooling efficiency is calculated using the real-time temperature correction model. The energy savings are computed by comparing the hourly heat load and energy consumption output to the initial energy consumption. Figure 4 depicts the analysis and calculation diagram of the triple energy consumption impact.
In the simulation model, the form of the air conditioning system, setting temperature of cooling and heating, working strategy, personnel distribution, building lighting index, air tightness, refrigeration and heating efficiency rules, setting range of roof thermal parameters, and other indicators shall refer to the relevant provisions and requirements in the design standard for energy efficiency of public buildings GB50189-2015 (Research, 2015), as seen in Figure 5.
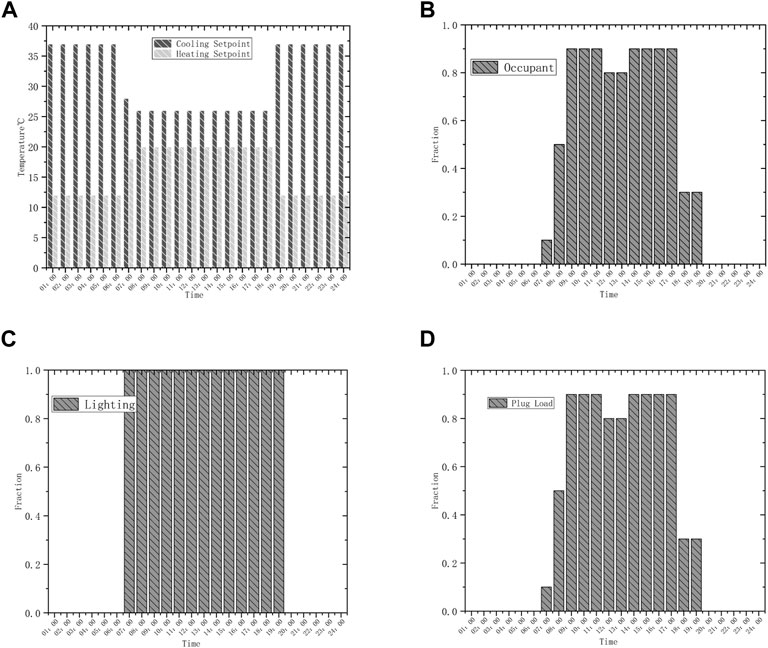
FIGURE 5. Energy simulation configuration. (A) Cooling and heating setpoint. (B) Occupant. (C) Lighting. (D) Plug load.
The building thermal characteristics are included in Table 1.
An ordinary roof is set with a reflectivity of 0.45 and an emissivity of 0.8. The reflectivity of the cool roof is 0.85, and the emissivity is 0.9. The green roof adopts the model of a 15-cm soil layer, 8-cm vegetation layer, and 2.0 leaf area index. Other settings refer to the default. The climate parameters used in this paper are taken from the official website of EnergyPlus (https://energyplus.net/weather), and Beijing, Nanjing, and Guangzhou are chosen as the representative cities, respectively, in cold regions, hot summer and cold winter regions, and hot summer and warm winter regions. The highest temperature day in the three cities is selected as the simulation date. According to the meteorological documents, Beijing is July 19, Nanjing is July 20, and Guangzhou is July 22. The weather parameters are shown in Figure 6.
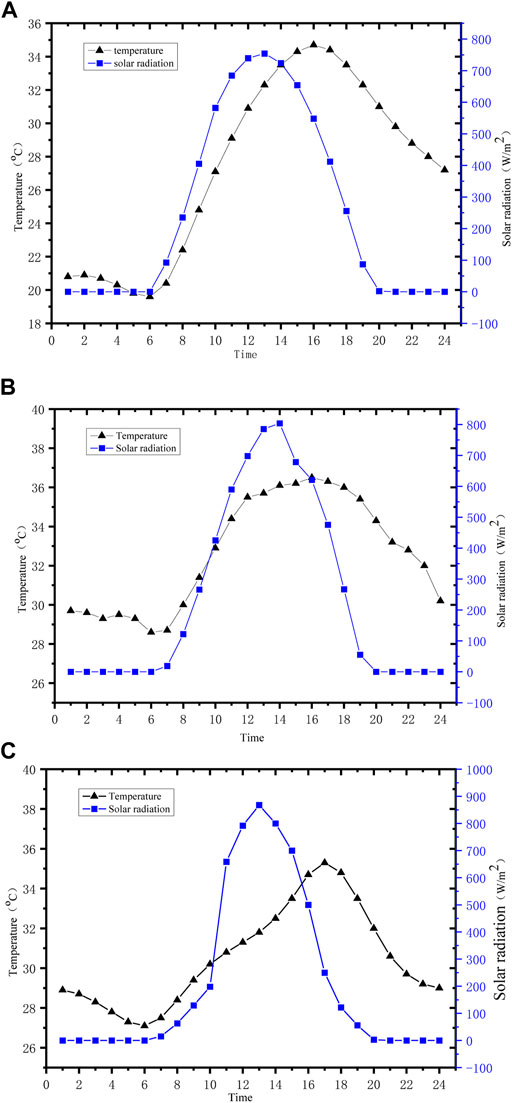
FIGURE 6. (A) Weather parameter of Beijing. (B) Weather parameter of Nanjing. (C) Weather parameter of Guangzhou.
As can be seen from Figure 6A, for Beijing, the maximum temperature is about 35°C, which occurs at around 16:00 PM. The lowest temperature is 20°C, which occurs at 06:00 AM before sunrise. The maximum solar radiation intensity is about 750 W/m2.
As can be seen from Figure 6B, for Nanjing, the maximum temperature is about 37°C, which occurs at around 16:00 PM. The lowest temperature is 28.5°C, which occurs at 06:00 AM before sunrise. The maximum solar radiation intensity is about 800 W/m2.
As can be seen from Figure 6C, for Guangzhou, the maximum temperature is about 35°C, which occurs at around 16:00 PM. The lowest temperature is 27°C, which occurs at 06:00 AM before sunrise. The maximum solar radiation intensity is about 900 W/m2.
3 Result analysis
3.1 Cold zones
Beijing is chosen as the representative city in the cold area. Beijing’s meteorological parameters are imported into the built Phoenix model to simulate the heat reflection cool roof and green roof, respectively, and the change of the community ambient temperature is obtained, as shown in Figure 7.
Figure 8 depicts the difference in the ambient temperature between the standard roof and the modified roof (the cool roof and green roof). As the numbers show, when there is solar radiation throughout the day, the neighborhood with the cool roof system has the lowest ambient temperature and the least evident heat island impact. At about 13:00 PM, when the solar irradiation intensity is the largest, the temperature difference is the most obvious: the cool roof can reduce the ambient temperature by 2°C, while the green roof can reduce the ambient temperature by 1°C. At night, cool roofs play a weak role in mitigating the heat island effect, and the ambient temperature of the community is the same as that of ordinary roofs. This is because the cool roof mainly reduces the ambient temperature by reflecting solar radiation. When there is no solar radiation at night, the high reflectivity characteristics of the cool roof cannot work as well as during the daytime. The green roof can effectively reduce the heat island effect at night because of the heat storage capacity of the soil layer and the transpiration of plants both during the day and at night. According to the calculation in Beijing, in general, the cool roof can reduce the ambient temperature by 1.26°C on average during the day and has no effect on the heat island effect at night. The green roof can reduce the ambient temperature by 0.77°C in the daytime and 0.49°C at night.
The newly converted ambient temperature was imported into the EPW data file instead of the weather file in the original EnergyPlus. The aforementioned EnergyPlus model was used to simulate the energy consumption of cool roofs and green roofs in the daytime working period (8:00 AM–18:00 PM) under the new ambient temperature. The results are shown in Figure 9.
As can be seen from Figure 9, the energy-saving effect of the cool roof is the most obvious, followed by the green roof. With the increase in the solar radiation intensity, the effect of cool roofs and green roofs on alleviating the heat island effect is more obvious, as well as its energy-saving effect. The energy-saving rate is the highest at about 14 o’clock, reaching 11.7%. The total energy consumption for the whole day is shown in Figure 10. After calculation, it can be observed that the cool roof can achieve an energy-saving percentage of 11.0% and that of the green roof is 9.4%.
3.3.1 Hot summer and cold winter zones
In hot summer and cold winter zones, Nanjing is selected as the typical city. Nanjing’s meteorological parameters are imported into the built Phoenix model to simulate the cool roof and green roof, respectively, and the change of the community ambient temperature is obtained, as shown in Figure 11. The ambient temperature difference between the ordinary roof and the modified roof (cool roof and green roof) is shown in Figure 12.
As can be seen from the figures, under the direct irradiation of the Sun, the ambient temperature of the cool roof community is the lowest, and the containment effect on the urban heat island effect is also the most obvious. At about 01:00 PM, when the solar irradiation intensity is the largest, the temperature difference is the most obvious: the cool roof can reduce the ambient temperature by 2.3°C, while the green roof can reduce the ambient temperature by 1.1°C. Cool roofs do little to mitigate the heat island effect and keep the same community ambient temperature as the ordinary roof at night. Because of the same reason as that in cold zones, when there is no solar radiation at night, the high reflectivity characteristics of the cool roof cannot work as well as during the daytime. The green roof can effectively reduce the heat island effect at night because of the heat storage capacity of the soil layer and the transpiration of plants both during the day and at night. According to the calculation in Nanjing, generally, the cool roof can reduce the ambient temperature by 1.27°C on average during the day and has no effect on the heat island effect at night. The green roof can reduce the ambient temperature by 0.79°C in the daytime and 0.52°C at night.
The cooling rate under the different roofs in hot summer and cold winter zones is shown in Figure 13.
As can be seen from Figure 13, the energy-saving effect of the cool roof is the most obvious, followed by the green roof. With the increase in the solar radiation intensity, the effect of cool roofs and green roofs on alleviating the heat island effect is more obvious, and its energy-saving effect is more obvious. The energy-saving rate meets the highest around 14 o’clock. At this time, the energy-saving rate of the cool roof is 13.6%, and that of the green roof is 11.8%. The total cooling load for the whole day is shown in Figure 14. It can be concluded that the cool roof can save energy by 11.5%, and the green roof can save energy by 8.1%.
3.3.2 Hot summer and warm winter zones
In hot summer and warm winter zones, Guangzhou is selected as the typical city. Conducted in the same way as in Beijing and Nanjing, Phoenix software is used to simulate the ambient temperature difference between the ordinary roof and the modified roof (cool roof and green roof), as shown in Figures 15 and 16.
As can be seen from the figures, under the direct irradiation of the Sun, the ambient temperature of the cool roof community is the lowest, and the containment effect on the urban heat island effect is also the most obvious. At about 13:00 p.m., when the solar irradiation intensity is the largest, the temperature difference is the most obvious: the cool roof can reduce the ambient temperature by 2.6°C, while the green roof can reduce the ambient temperature by 1.2°C. As the same reason for the aforementioned two climate zones, lack of solar reflectivity working conditions for the cool roof and the heat storage capacity of the soil layer and the transpiration of plants of the green roof contribute to the advantage of the green roof on the mitigation of the urban heat island effect. According to the calculation in Guangzhou, generally, the cool roof can reduce the ambient temperature by 1.67°C on average during the day and has no effect on the heat island effect at night. The green roof can reduce the ambient temperature by 0.92°C in the daytime and 0.59°C at night.
The cooling rate under different roofs in hot summer and warm winter zones is shown in Figure 13.
As can be seen from Figure 17, the energy-saving effect of the cool roof is the most obvious, followed by the green roof. With the increase in the solar radiation intensity, the effect of cool roofs and green roofs on alleviating the heat island effect is more obvious, and its energy-saving effect is more obvious. The energy-saving rate meets the highest around 14 o’clock. At this time, the energy-saving rate of the cool roof is 16.3% and that of the green roof is 15.2%. The total cooling load for the whole day is shown in Figure 18. It can be concluded that the cool roof can save energy by 12.6%, and the green roof can save energy by 9.3%.
Comparing the results of cold areas, hot summer and cold winter zones, and hot summer and warm winter zones, it can be seen that cool roofs and green roofs can effectively alleviate the heat island effect. The effect of the cool roof is more significant in the daytime, while the green roof can reduce the ambient temperature no matter in the daytime or at night. The greater the intensity of solar radiation, the greater is the intensity of the heat island reduced by the cool roof and green roof. With the decrease in the latitude, the effect is more and more obvious.
4 Conclusion
Given the prevalence of the heat island effect, this research proposes a threefold positive energy-saving impact of heat island effect mitigation on building air conditioning energy consumption for cool roofs and green roofs. The effect of different roof technologies on easing urban heat island effects in different climate areas is simulated using modeling and analysis, and the triple effect impact of building energy consumption is computed and examined. The conclusions are as follows:
1) Cool roofs and green roofs have a triple effect on building energy conservation: reducing the direct heat gain caused by improving the thermal characteristics of the roof, reducing the heat transfer through the enclosure structure caused by mitigating the urban heat island, and improving the efficiency of refrigeration equipment caused by reducing the ambient temperature.
2) Cool roofs and green roofs play an important role in reducing urban heat islands and enhancing the thermal environment of cities. Among these, the effect of a cool roof is more noticeable during the day. Cool roofs can lower the regional ambient temperature by 2°C, 2.3°C, and 2.6°C in cold areas, hot summer and cold winter areas, and hot summer and warm winter areas, respectively. However, reducing the consumption at night is challenging for cool roofs. Green roofs, due to their unique plant transpiration, may effectively minimize the heat island effect both during the day and at night.
3) The triple effect on building energy conservation by decreasing the heat island effect for cool roofs and green roofs is noteworthy according to energy consumption simulation studies. The triple saving impact on building energy consumption for cool roofs and green roofs may achieve 11.0 percent, 11.5 percent, and 12.6 percent, and 9.4 percent, 8.1 percent, and 9.3 percent, respectively, in cold zones, hot summer and cold winter zones, and hot summer and warm winter zones.
4) When the influence of temperature change and the energy consumption is compared, it is discovered that as latitude decreases, solar radiation gradually increases, and the role of cool roofs in mitigating the heat island effect and saving air conditioning energy consumption gradually increases, while the green roof nearly remains stable.
Data availability statement
The raw data supporting the conclusions of this article will be made available by the authors, without undue reservation.
Author contributions
XZ put forward the idea of the paper. SZ conducted the simulation work and data analysis. XZ revised the manuscript.
Funding
The research described in this paper is supported by the Changzhou Science and Technology Project (No. CJ20220032) and the National Natural Science Foundation of China (No. 51520105009).
Conflict of interest
The authors declare that the research was conducted in the absence of any commercial or financial relationships that could be construed as a potential conflict of interest.
Publisher’s note
All claims expressed in this article are solely those of the authors and do not necessarily represent those of their affiliated organizations, or those of the publisher, the editors, and the reviewers. Any product that may be evaluated in this article, or claim that may be made by its manufacturer, is not guaranteed or endorsed by the publisher.
References
Akbari, H, Cartalis, C, Kolokotsa, D, Muscio, A, Pisello, A L, Rossi, F, et al. (2016). Local climate change and urban heat island mitigation techniques–the state of the art. J. Civ. Eng. Manag. 22, 1–16. doi:10.3846/13923730.2015.1111934
Akbari, H, Pomerantz, M, and Taha, H (2001). Cool surfaces and shade trees to reduce energy use and improve air quality in urban areas. Sol. Energy 70, 295–310. doi:10.1016/s0038-092x(00)00089-x
Akbari, H., and Rose, L S (2008). Urban surfaces and heat island mitigation potentials. J. Hum. Environ. Syst. 11, 85–101. doi:10.1618/jhes.11.85
Armson, D, Stringer, P, and Ennos, A (2012). The effect of tree shade and grass on surface and globe temperatures in an urban area. Urban For. Urban Green. 11, 245–255. doi:10.1016/j.ufug.2012.05.002
Cai, W, Wu, Y, Zhong, Y, and Ren, H, (2009). China building energy consumption: Situation, challenges and corresponding measures. Energy Policy 37, 2054–2059. doi:10.1016/j.enpol.2008.11.037
Chua, K J, Chou, S K, Yang, W, and Yan, J (2013). Achieving better energy-efficient air conditioning–a review of technologies and strategies. Appl. Energy 104, 87–104. doi:10.1016/j.apenergy.2012.10.037
Deilami, K, Kamruzzaman, M, and Liu, Y (2018). Urban heat island effect: A systematic review of spatio-temporal factors, data, methods, and mitigation measures. Int. J. Appl. Earth Obs. Geoinf. 67, 30–42. doi:10.1016/j.jag.2017.12.009
Levinson, R, Berdahl, P, Akbari, H, Miller, W, Joedicke, I, Reilly, J, et al. (2007). Methods of creating solar-reflective nonwhite surfaces and their application to residential roofing materials. Sol. Energy Mat. Sol. Cells 91, 304–314. doi:10.1016/j.solmat.2006.06.062
Levinson, R, Berdahl, P, and Akbari, H (2005). Solar spectral optical properties of pigments—Part I: Model for deriving scattering and absorption coefficients from transmittance and reflectance measurements. Sol. Energy Mat. Sol. Cells 89, 319–349. doi:10.1016/j.solmat.2004.11.012
Levinson, R, Berdahl, P, and Akbari, H (2005). Solar spectral optical properties of pigments—Part II: Survey of common colorants. Sol. Energy Mat. Sol. Cells 89, 351–389. doi:10.1016/j.solmat.2004.11.013
Li, X X, and Norford, L K (2016). Evaluation of cool roof and vegetations in mitigating urban heat island in a tropical city, Singapore. Urban Clim. 16, 59–74. doi:10.1016/j.uclim.2015.12.002
Lin, Y, and Yang, W (2018). Application of multi-objective genetic algorithm based simulation for cost-effective building energy efficiency design and thermal comfort improvement. Front. Energy Res. 6, 25. doi:10.3389/fenrg.2018.00025
Liu, G, Hong, S, Wang, Y, Dai, L, and Wei, W (2021). Study of the fifth façade: Planning and controlling in the wuhan aerotropolis, in Human-centered urban planning and design in China: Volume II (Springer), 29–39. Berlin, Germany.
Liu, G, Kong, Z, Dong, J, Dong, X, Jiang, Q, Wang, K, et al. (2021). Influencing factors, energy consumption, and carbon emission of central heating in China: A supply chain perspective. Front. Energy Res. 9, 648857. doi:10.3389/fenrg.2021.648857
Research, C. A. of B. (2015). Design standard for energy efficiency of public buildings (GB50189-2015). Beijing, China: China Architecture & Building Press.
Rizwan, A. M., Dennis, L. Y. C., and Liu, C. (2008). A review on the generation, determination and mitigation of Urban Heat Island. J. Environ. Sci. 20, 120–128. doi:10.1016/S1001-0742(08)60019-4
Rosenfeld, A. H., Akbari, H., Romm, J. J., and Pomerantz, M. (1998). Cool communities: Strategies for heat island mitigation and smog reduction. Energy Build. 28, 51–62. doi:10.1016/s0378-7788(97)00063-7
Rosenzweig, C., Solecki, W., and Slosberg, R. (2006). Mitigating New York City’s heat island with urban forestry, living roofs, and light surfaces. Rep. N. Y. State Energy Res. Dev. Auth.
Saber, H. H., and Maref, W. (2019). Energy performance of cool roofs followed by development of practical design tool. Front. Energy Res. 7, 122. doi:10.3389/fenrg.2019.00122
Saber, H. H., Maref, W., and Hajiah, A. E. (2019). Hygrothermal performance of cool roofs subjected to Saudi climates. Front. Energy Res. 7, 39. doi:10.3389/fenrg.2019.00039
Saito, I., Ishihara, O., and Katayama, T. (1990). Study of the effect of green areas on the thermal environment in an urban area. Energy Build. 15, 493–498. doi:10.1016/0378-7788(90)90026-f
Santamouris, M (2013a). Using cool pavements as a mitigation strategy to fight urban heat island—a review of the actual developments. Renew. Sustain. Energy Rev. 26, 224–240. doi:10.1016/j.rser.2013.05.047
Santamouris, M., Cartalis, C., Synnefa, A., and Kolokotsa, D. (2015). On the impact of urban heat island and global warming on the power demand and electricity consumption of buildings—a review. Energy Build. 98, 119–124. doi:10.1016/j.enbuild.2014.09.052
Santamouris, M. (2014). Cooling the cities–a review of reflective and green roof mitigation technologies to fight heat island and improve comfort in urban environments. Sol. Energy 103, 682–703. doi:10.1016/j.solener.2012.07.003
Santamouris, M, Synnefa, A, and Karlessi, T (2011). Using advanced cool materials in the urban built environment to mitigate heat islands and improve thermal comfort conditions. Sol. Energy 85, 3085–3102. doi:10.1016/j.solener.2010.12.023
Sun, C Y, Lee, K P, Lin, T P, and Lee, S H (2012). “Vegetation as a material of roof and city to cool down the temperature,” in Advanced materials research (Seestrasse: Trans Tech Publ), 552–556.
Susca, T, Gaffin, S R, and Dell’Osso, G R (2011). Positive effects of vegetation: Urban heat island and green roofs. Environ. Pollut. 159, 2119–2126. doi:10.1016/j.envpol.2011.03.007
Takebayashi, H, and Moriyama, M (2007). Surface heat budget on green roof and high reflection roof for mitigation of urban heat island. Build. Environ. 42, 2971–2979. doi:10.1016/j.buildenv.2006.06.017
Yang, J, Wang, Y, Xiu, C, Xiao, X, Xia, J, and Jin, C (2020). Optimizing local climate zones to mitigate urban heat island effect in human settlements. J. Clean. Prod. 275, 123767. doi:10.1016/j.jclepro.2020.123767
Keywords: building energy, cool roof, green roof, heat island effect, simulation analysis
Citation: Zhao S and Zhang X (2023) Energy consumption and heat island effect mitigation analysis of different roofs considering superposition coupling. Front. Energy Res. 10:1047614. doi: 10.3389/fenrg.2022.1047614
Received: 18 September 2022; Accepted: 16 November 2022;
Published: 18 January 2023.
Edited by:
Runsheng Tang, Yunnan Normal University, ChinaReviewed by:
Xing Lu, Pacific Northwest National Laboratory (DOE), United StatesFeng Wang, Yangzhou University, China
Copyright © 2023 Zhao and Zhang. This is an open-access article distributed under the terms of the Creative Commons Attribution License (CC BY). The use, distribution or reproduction in other forums is permitted, provided the original author(s) and the copyright owner(s) are credited and that the original publication in this journal is cited, in accordance with accepted academic practice. No use, distribution or reproduction is permitted which does not comply with these terms.
*Correspondence: Shanguo Zhao, brozhao@126.com